Three-component selective synthesis of phenothiazines and bis-phenothiazines under metal-free conditions†
Received
11th January 2023
, Accepted 30th January 2023
First published on 1st February 2023
Abstract
An iodine-containing reagent promoted three-component method for the selective synthesis of phenothiazines and bis-phenothiazines has been developed. The present protocol starts from simple and easily available cyclohexanones, elemental sulfur, and inorganic ammonium salts, selectively producing phenothiazines and bis-phenothiazines in satisfactory yields under aerobic conditions. This method has the advantages of simple and readily available starting materials and metal-free conditions, affording a facile and practical approach for the preparation of phenothiazines and bis-phenothiazines.
Phenothiazine derivatives have a broad spectrum of pharmacological activities and are widely applied in the pharmaceutical industry.1,2 To date, more than 100 phenothiazine derivatives have been used as antipsychotic drugs in clinical settings. Moreover, phenothiazine derivatives have extensive application in the field of optoelectronic materials and dyes.3,4 As special phenothiazine derivatives, bis-phenothiazines described as thia-bridged triarylamine heterohelicenes with an aryl ring and a nitrogen atom in common forced into a helical shaped structure by four long carbon–sulfur bonds have attracted increasing attention in recent years. Bis-phenothiazines have been applied as redox-driven molecular switches, organic dyes for dye-sensitized solar cells, and ultralong room-temperature phosphorescence (URTP) materials, due to their unique structural, unusual electronic, and photochemical properties (Fig. 1).5
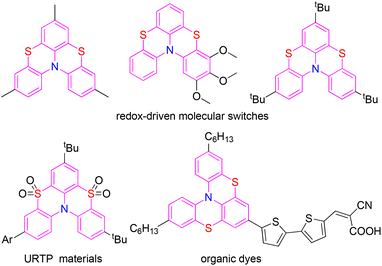 |
| Fig. 1 Some representative bis-phenothiazines. | |
On account of the significance of these compounds, a variety of methods for the synthesis of phenothiazines have been reported in the past decades.6,7 With the rapid development of transition-metal catalysts, transition-metal-catalyzed coupling reactions have proved to be the most powerful tools for the preparation of phenothiazines.8,9 However, metal contamination remains an important issue to be solved urgently, particularly in medicine and materials science. Although several metal-free approaches for the preparation of N-unsubstituted phenothiazines have been developed in recent years, the use of two kinds of ortho difunctionalized arenes as starting materials also limits their synthetic application.10 As for bis-phenothiazines, only a few methodologies have been developed due to their complex structure. And most of them require multi-step procedures or pre-functionalized complex substrates. For instance, in 2004 Okada et al. reported a four-step procedure for the preparation of bis-phenothiazines from 1,3-dichloro-2-nitrobenzenes and o-bromothiophenols (Scheme 1a).11 In 2008, Menichetti and co-workers described a two-step synthesis of bis-phenothiazines from triphenylamines and phthalimidosulfenyl chloride by four consecutive electrophilic regioselective aromatic sulfur insertions (Scheme 1b).12 In 2019, Viglianisi et al. developed a similar two-step approach for constructing bis-phenothiazines, which started from N-phenyl phenothiazines and phthalimidosulfenyl chloride.13 Despite their uses, the need for multiple steps or pre-functionalized precursors limits their synthetic application dramatically. Therefore, it is highly desirable to explore new approaches to synthesize phenothiazines and bis-phenothiazines from simple and easily available chemicals under metal-free conditions.
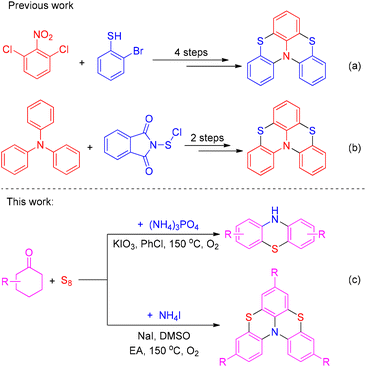 |
| Scheme 1 General synthesis of bis-phenothiazines. | |
In recent years, elemental sulfur, as a cheap, easily available, nontoxic, stable, and easy to handle reagent, has been regarded as the best source of sulfur atoms in the formation of organosulfur compounds. It has shown great potential in C–S bond formation.14 A diverse range of methods for the preparation of sulfur-containing heterocycles have been developed using elemental sulfur as the sulfur source in the last few years.15,16 Our group has been dedicated to the dehydroaromatization of cyclohexanones17 and construction of sulfur-containing heterocycles using elemental sulfur as the sulfur source in recent years.18,19 As part of our sustained research in these fields, herein we describe an iodine-containing reagent promoted three-component selective synthesis of phenothiazines and bis-phenothiazines under aerobic conditions (Scheme 1c). The present protocol starts from cheap and easily available cyclohexanones, elemental sulfur, and inorganic ammonium salts, selectively producing phenothiazines and bis-phenothiazines in satisfactory yields under metal-free conditions.
This research was initiated by using 4-methylcyclohexanone (1a), elemental sulfur, and ammonium iodide as the reactants to probe the optimal reaction conditions (Table 1). Based on our previous studies,19a this reaction was initially conducted with 0.2 equiv. of KI in chlorobenzene under an oxygen atmosphere at 150 °C. To our delight, product 2a was generated in 24% isolated yield and a trace amount of product 3a was also detected under these conditions (entry 1). Inspired by this, a number of ammonium salts were tested (entries 1–4). Among them, (NH4)3PO4 showed the best efficiency to afford product 2a in 52% isolated yield (entry 4). Then various iodine-containing reagents were screened (entries 5–9). The highest yield of 2a was obtained when KIO3 was used as the additive (entry 8). Encouragingly, the product 3a was obtained in 12% yield when 2.0 equiv. of DMSO were used as the co-oxidant (entry 10). Subsequently, a number of iodine-containing reagents were tested again (entries 11–14). The yield of 3a was enhanced to 20% isolated yield when NaI was used (entry 11). Then a few other solvents including PhMe, NMP, DMF, and ethyl acetate (EA) were screened (entries 15–18). Ethyl acetate (EA) proved to be the best solvent to provide product 3a in 26% yield (entry 18). The yield increased to 34% when the amount of NaI was increased to 0.3 equiv. (entry 19). It further increased to 55% isolated yield when 4.0 equiv. of elemental sulfur and 2.5 equiv. of NH4I were used (entry 20). Regrettably, the yield of 3a could not be further improved either by increasing or decreasing the reaction temperature (entries 21 and 22).
Table 1 Optimization of reaction conditionsa
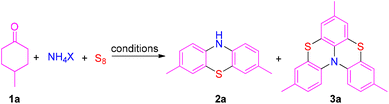
|
Entry |
NH4X |
Additive |
Oxidant |
Solvent |
Yieldb (%) |
2a
|
3a
|
Reaction conditions: 0.4 mmol of 1a for entries 1–9, 0.6 mmol of 1a for entries 10–22, S8 (0.1 mmol), NH4X (0.3 mmol), additive (0.04 mmol), DMSO (0.4 mmol), solvent (0.6 mL), 150 °C oil bath, under O2, sealed tube, 24 h.
Isolated yield.
NaI (0.06 mmol).
S8 (0.2 mmol), NH4I (0.5 mmol).
140 °C.
160 °C.
|
1 |
NH4I |
KI |
O2 |
PhCl |
24 |
Trace |
2 |
NH4OAc |
KI |
O2 |
PhCl |
10 |
0 |
3 |
(NH4)2CO3 |
KI |
O2 |
PhCl |
45 |
0 |
4 |
(NH4)3PO4 |
KI |
O2 |
PhCl |
52 |
0 |
5 |
(NH4)3PO4 |
NaI |
O2 |
PhCl |
69 |
0 |
6 |
(NH4)3PO4 |
I2 |
O2 |
PhCl |
47 |
0 |
7 |
(NH4)3PO4 |
IBr |
O2 |
PhCl |
56 |
0 |
8 |
(NH4)3PO4 |
KIO3 |
O2 |
PhCl |
80 |
0 |
9 |
(NH4)3PO4 |
NaIO4 |
O2 |
PhCl |
75 |
0 |
10 |
NH4I |
KI |
DMSO + O2 |
PhCl |
8 |
12 |
11 |
NH4I |
NaI |
DMSO + O2 |
PhCl |
10 |
20 |
12 |
NH4I |
IBr |
DMSO + O2 |
PhCl |
12 |
8 |
13 |
NH4I |
I2 |
DMSO + O2 |
PhCl |
9 |
10 |
14 |
NH4I |
KIO3 |
DMSO + O2 |
PhCl |
16 |
11 |
15 |
NH4I |
NaI |
DMSO + O2 |
PhMe |
11 |
14 |
16 |
NH4I |
NaI |
DMSO + O2 |
NMP |
0 |
0 |
17 |
NH4I |
NaI |
DMSO + O2 |
DMF |
0 |
0 |
18 |
NH4I |
NaI |
DMSO + O2 |
EA |
13 |
26 |
19c |
NH4I |
NaI |
DMSO + O2 |
EA |
11 |
34 |
20c,d |
NH4I |
NaI |
DMSO + O2 |
EA |
8 |
55 |
21c,d,e |
NH4I |
NaI |
DMSO + O2 |
EA |
7 |
50 |
22c,d,f |
NH4I |
NaI |
DMSO + O2 |
EA |
9 |
48 |
With the optimized conditions established, the scope and generality for phenothiazine formation were evaluated (Scheme 2). 4-Methylcyclohexanone offered product 2a in 80% isolated yield. However, cyclohexanone provided product 2b in 60% yield. Cyclohexanones with straight-chain alkyl or branched alkyl groups at the para-position reacted well to afford phenothiazine products in moderate to good yields (2c–2h). Cyclohexanones bearing a remote substituent at the C4 position also were able to give the corresponding products in moderate yields (2i and 2j). Regrettably, a trace amount of product 2k was generated when cyclohexanone with a strongly electron-donating group at the para-position was used as the substrate. However, the corresponding products were obtained in good yields when cyclohexanones with a strongly electron-withdrawing group were used (2l and 2m). In addition, 4-phenylcyclohexanone and 4-(4-chlorophenyl)cyclohexanone also reacted smoothly to provide target products 2n and 2o in acceptable yields. However, the desired product 2p was not detected when 4-(4-hydroxyphenyl)cyclohexanone was used. No significant steric hindrance effect was observed when 2-methylcyclohexanone was used as the substrate (2q).
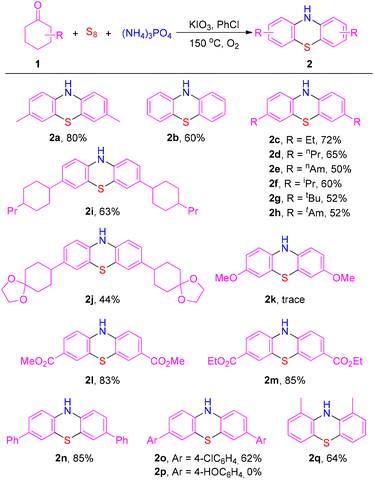 |
| Scheme 2 The substrate scope for phenothiazine formation. | |
Next, the substrate scope for the synthesis of bis-phenothiazines was investigated (Scheme 3). The product 3a was acquired in 55% isolated yield under standard conditions. It's worth noting that the structure of product 3a was further confirmed by single-crystal X-ray diffraction.20 Cyclohexanones bearing straight-chain alkyl or branched alkyl groups at the para position reacted smoothly to afford bis-phenothiazine products in moderate yields (3b–3e). However, the product was obtained in lower yield when cyclohexanone with a phenyl group at the para position was used as the substrate (3f). Unfortunately, the corresponding products were generated in trace amounts when cyclohexanones with a strongly electron-donating group or strongly electron-withdrawing group at the para position were used as the substrates (3g and 3h).
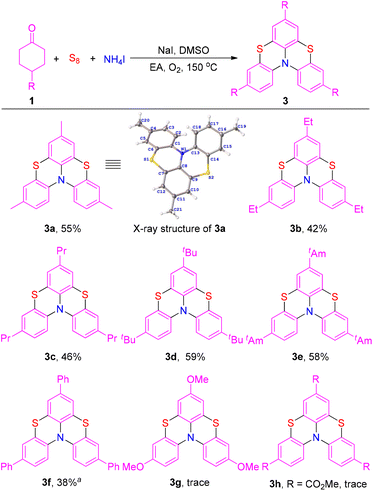 |
| Scheme 3 The substrate scope for bis-phenothiazine formation. a 160 °C. | |
To demonstrate the synthetic applicability of this three-component reaction, the large-scale and derivatization reaction carried out (Scheme 4). The product 3d was obtained in 48% yield when the reaction was scaled up to 1.0 mmol. It should be noted that product 3d could be applied as a redox-triggered chiroptical switch.5a,e Furthermore, product 3d could be oxidized into the derivative 4a in 98% isolated yield when it was treated with 3-chloroperoxybenzoic acid (m-CPBA). The derivative 4a could be applied as an ultralong room-temperature phosphorescence (URTP) material.5c
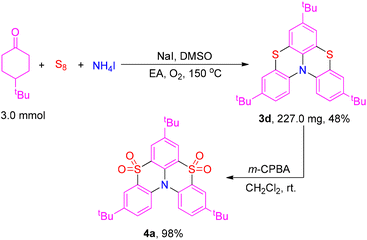 |
| Scheme 4 The large-scale and derivatization reaction. | |
To investigate the mechanism, some control experiments were carried out (Scheme 5). The phenothiazine product 2a was obtained in 80% isolated yield under the optimized conditions. The yield of 2a did not decrease significantly when 2,6-di-tert-butyl-4-methylphenol (BHT) or 2,2,6,6-tetramethyl-1-piperidinyloxy (TEMPO) was added to this reaction (Scheme 5a). Moreover, radical scavengers BHT and TEMPO also could not impede the formation of product 3a (Scheme 5b). These results indicate that this reaction probably does not involve a radical process. In addition, product 3a could not be generated when phenothiazine 2a was treated with 4-methylcyclohexanone 1a and elemental sulfur under the optimized conditions, indicating that product 3a was not transformed from phenothiazine 2a (Scheme 5c).
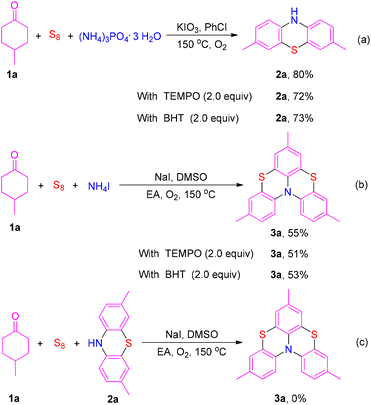 |
| Scheme 5 Control experiments. | |
Based on the above-mentioned experimental results and related literature,16d,19a,21 a plausible mechanism is proposed (Scheme 6). Condensation of ammonium salts with three equiv. of 4-methylcyclohexanone (1a) provides intermediate A, which attacks elemental sulfur via the Willgerodt–Kindler procedure to afford intermediate B. Intermediate C is formed from intermediate B by the release of sulfur (Sn−1) and a proton. Subsequently, intermediate C is oxidized into intermediate D in the presence of elemental iodine from NaI, which is further converted into intermediate Evia intramolecular addition. Deprotonation of intermediate E produces intermediate F, which is further translated into intermediate Gvia the second thionation process. Finally, oxidative dehydrogenation of intermediate G affords product 3a with the aid of a NaI/DMSO/O2 system. Moreover, product 2a is formed via a similar pathway.
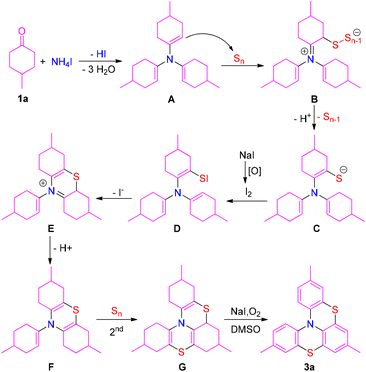 |
| Scheme 6 Possible mechanism. | |
Conclusions
In conclusion, we have disclosed an iodine-containing reagent promoted three-component method for the selective synthesis of phenothiazines and bis-phenothiazines. The present protocol starts from cheap and easily available cyclohexanones, elemental sulfur, and inorganic ammonium salts, selectively producing phenothiazines and bis-phenothiazines in satisfactory yields under aerobic conditions. In this work, multiple C–N bonds and C–S bonds were constructed in one pot under metal-free conditions. This method has the advantages of simple and readily available starting materials and metal-free conditions, affording a facile and practical approach for the preparation of phenothiazines and bis-phenothiazines.
Conflicts of interest
There are no conflicts to declare.
Acknowledgements
Financial support was provided by the National Natural Science Foundation of China (No. 22201240, 22271244 and 21871226), the Hunan Provincial Natural Science Foundation of China (No. 2020JJ5531), and the Open Research Fund of School of Chemistry and Chemical Engineering, Henan Normal University (2022C02). The Undergraduate Investigated Study and Innovated Experiment Plan from the Ministry of Education of China and the Science and Technology Innovation Program of Hunan Province (2020RC1009) are gratefully acknowledged.
References
-
(a) M. Donnier-Marechal, P. E. Larchanche, D. L. Broc, C. Furman, P. Carato and P. Melnyk, Carboline-and phenothiazine derivated heterocycles as potent SIGMA-1 protein ligands, Eur. J. Med. Chem., 2015, 89, 198–206 CrossRef CAS PubMed;
(b) S. Darvesh, K. V. Darvesh, R. S. McDonald, D. Mataija, R. Walsh, S. Mothana, O. Lockridge and E. Martin, Carbamates with differential mechanism of inhibition toward acetylcholinesterase and butyrylcholinesterase, J. Med. Chem., 2008, 51, 4200–4212 CrossRef CAS PubMed;
(c) K. Pluta, M. Jelen, B. Morak-Mlodawska, M. Zimecki, J. Artym, M. Kocieba and E. Zaczynska, Azaphenothiazines-promising phenothiazine derivatives. An insight into nomenclature, synthesis, structure elucidation and biological properties, Eur. J. Med. Chem., 2017, 138, 774–806 CrossRef CAS PubMed;
(d) Y. Gao, T. Y. Sun, W. F. Bai and C. G. Bai, Design, synthesis and evaluation of novel phenothiazine derivatives as inhibitors of breast cancer stem cells, Eur. J. Med. Chem., 2019, 183, 111692 CrossRef PubMed.
-
(a) H. Prinz, A.-K. Ridder, K. Vogel, K. J. Böhm, I. Ivanov, J. B. Ghasemi, E. Aghaee and K. Muller, N-Heterocyclic (4-phenylpiperazin-1-yl)methanones derived from phenoxazine and phenothiazine as highly potent inhibitors of tubulin polymerization, J. Med. Chem., 2017, 60, 749–766 CrossRef CAS PubMed;
(b) I. H. Gilbert, Drug discovery for neglected diseases: molecular target-based and phenotypic approaches: miniperspectives series on phenotypic screening for antiinfective targets, J. Med. Chem., 2013, 56, 7719–7726 CrossRef CAS PubMed;
(c) N. Scalacci, A. K. Brown, F. R. Pavan, C. M. Ribeiro, F. Manetti, S. Bhakta, A. Maitra, D. L. Smith, E. Petricci and D. Castagnolo, Synthesis and SAR evaluation of novel thioridazine derivatives active against drug-resistant tuberculosis, Eur. J. Med. Chem., 2017, 127, 147–158 CrossRef CAS PubMed;
(d) K. Vögerl, N. Ong, J. Senger, D. Herp, K. Schmidtkunz, M. Marek, M. Muller, K. Bartel, T. B. Shaik, N. J. Porter, D. Robaa, D. W. Christianson, C. Romier, W. Sippl, M. Jung and F. Bracher, Synthesis and biological investigation of phenothiazine-based benzhydroxamic acids as selective histone deacetylase 6 inhibitors, J. Med. Chem., 2019, 62, 1138–1166 CrossRef PubMed.
-
(a) R. Y. Lai, X. Kong, S. A. Jenekhe and A. J. Bard, Synthesis, cyclic voltammetric studies, and electrogenerated chemiluminescence of a new phenylquinoline-biphenothiazine donor-acceptor molecule, J. Am. Chem. Soc., 2003, 125, 12631–12639 CrossRef CAS PubMed;
(b) D. Sun, S. V. Rosokha and J. Kochi, Donor-acceptor (electronic) coupling in the precursor complex to organic electron transfer: intermolecular and intramolecular self-exchange between phenothiazine redox centers, J. Am. Chem. Soc., 2004, 126, 1388–1401 CrossRef CAS PubMed;
(c) E. A. Weiss, M. J. Tauber, R. F. Kelley, M. J. Ahrens, M. A. Ratner and M. R. Wasielewski, Conformationally gated switching between superexchange and hopping within oligo-p-phenylene-based molecular wires, J. Am. Chem. Soc., 2005, 127, 11842–11850 CrossRef CAS PubMed;
(d) H. W. Rhee, S. J. Choi, S. H. Yoo, Y. O. Jang, H. H. Park, R. M. Pinto, J. C. Cameselle, F. J. Sandoval, S. Roje, K. Han, D. S. Chung, J. Suh and J. I. Hong, A bifunctional molecule as an artificial flavin mononucleotide cyclase and a chemosensor for selective fluorescent detection of flavins, J. Am. Chem. Soc., 2009, 131, 10107–10112 CrossRef CAS PubMed.
-
(a) C. Quinton, L. Sicard, N. Vanthuyne, O. Jeannin and C. Poriel, Confning nitrogen inversion to yield enantiopure quinolino[3,2,1-k]phenothiazine derivatives, Adv. Funct. Mater., 2018, 28, 1803140 CrossRef;
(b) A. F. Buene, E. E. Ose, A. G. Zakariassen, A. Hagfeldt and B. H. Hoff, Auxiliary donors for phenothiazine sensitizers for dye-sensitized solar cells-how important are they really, J. Mater. Chem. A, 2019, 7, 7581–7590 RSC.
-
(a) S. Menichetti, S. Cecchi, P. Procacci, M. Innocenti, L. Becucci, L. Franco and C. Viglianisi, Thia-bridged triarylamine heterohelicene radical cations as redox-driven molecular switches, Chem. Commun., 2015, 51, 11452–11454 RSC;
(b) C. Kim, H. Choi, S. Paek, J.-J. Kim, K. Song, M.-S. Kang and J. Ko, Molecular engineering of thia-bridged triphenylamine heterohelicenes as novel organic dyes for dye-sensitized solar cells, J. Photochem. Photobiol., A, 2011, 225, 17–25 CrossRef CAS;
(c) Z. T. Ma, Z. Q. Yang, L. Mu, L. S. Deng, L. J. Chen, B. H. Wang, X. F. Qiao, D. H. Hu, B. Yang, D. G. Ma, J. B. Peng and Y. G. Ma, Converting molecular luminescence to ultralong room-temperature phosphorescence via the excited state modulation of sulfone-containing heteroaromatics, Chem. Sci., 2021, 12, 14808–14814 RSC;
(d) M. Lupi, S. Menichetti, P. Stagnaro, R. Utzeri and C. Viglianisi, Thia-bridged triarylamine[4]helicene-functionalized polynorbornenes as redox-active pH-sensitive polymers, Synthesis, 2021, 2602–2611 CAS;
(e) B. D. Gliemann, A. G. Petrovic, E. M. Zolnhofer, P. O. Dral, F. Hampel, G. Breitenbruch, P. Schulze, V. Raghavan, K. Meyer, P. L. Polavarapu, N. Berova and M. Kivala, Configurationally stable chiral dithia-bridged hetero[4]helicene radical cation: electronic structure and absolute configuration, Chem. – Asian J., 2017, 12, 31–35 CrossRef CAS PubMed.
-
(a) N. L. Smith, Synthesis of phenothiazine derivatives for use as antioxidants, J. Org. Chem., 1950, 15, 1125–1130 CrossRef CAS;
(b) M. Mayer, P. T. Lang, S. Gerber, P. B. Madrid, I. G. Pinto, R. K. Guy and T. L. James, Synthesis and testing of a focused phenothiazine library for binding to HIV-1 TAR RNA, Chem. Biol., 2006, 13, 993–1000 CrossRef CAS PubMed;
(c) P. B. Madrid, W. E. Polgar, L. Toll and M. J. Tanga, Synthesis and antitubercular activity of phenothiazines with reduced binding to dopamine and serotonin receptors, Bioorg. Med. Chem. Lett., 2007, 17, 3014–3017 CrossRef CAS PubMed.
-
(a) H. L. Yale, 3-Chloro-10-dialkylaminoalkylphenothiazines, J. Am. Chem. Soc., 1955, 77, 2270–2272 CrossRef CAS;
(b) R. S. Rathore, M. Jain, A. Gupta, V. Saraswat, V. Gupta and R. R. Gupta, Synthesis of phenothiazines via Smiles rearrangement, Pharmazie, 1992, 47, 945–946 CAS;
(c) N. Sharma, R. Gupta, M. Kumar and R. R. Gupta, Synthesis of fluorophenothiazines via Smiles rearrangement and their conversion into sulfones, J. Fluorine Chem., 1999, 98, 153–157 CrossRef CAS.
-
(a) T. Dahl, C. W. Tornøe, B. Bang-Andersen, P. Nielsen and M. Jørgensen, Palladium-catalyzed three-component approach to promazine with formation of one carbon-sulfur and two carbon-nitrogen bonds, Angew. Chem., Int. Ed., 2008, 47, 1726–1728 CrossRef CAS PubMed;
(b) D. W. Ma, Q. Geng, H. Zhang and Y. W. Jiang, Assembly of substituted phenothiazines by a sequentially controlled CuI/l-proline-catalyzed cascade C-S and C-N bond formation, Angew. Chem., Int. Ed., 2010, 49, 1291–1294 CrossRef CAS PubMed;
(c) J. Tang, B. Xu, X. Mao, H. Yang, X. Wang and X. Lv, One-pot synthesis of pyrrolo[3,2,1-kl]phenothiazines through copper-catalyzed tandem coupling/double cyclization reaction, J. Org. Chem., 2015, 80, 11108–11114 CrossRef CAS PubMed;
(d) X. Y. Rui, C. Wang, D. J. Si, X. C. Hui, K. T. Li, H. M. Wen, W. Li and J. Liu, One-pot tandem access to phenothiazine derivatives from acetanilide and 2-bromothiophenol via rhodium-catalyzed C-H thiolation and copper-catalyzed C-N amination, J. Org. Chem., 2021, 86, 6622–6632 CrossRef CAS PubMed.
-
(a) C. Dai, X. F. Sun, X. Z. Tu, L. Wu, D. Zhan and Q. L. Zeng, Synthesis of phenothiazines via ligand-free CuI-catalyzed cascade C-S and C-N coupling of aryl ortho-dihalides and ortho-aminobenzenethiols, Chem. Commun., 2012, 48, 5367–5369 RSC;
(b) W. Y. Hu and S. L. Zhang, Method for the synthesis of phenothiazines via a domino iron-catalyzed C-S/C-N cross-coupling reaction, J. Org. Chem., 2015, 80, 6128–6132 CrossRef CAS PubMed;
(c) M. Huang, D. Huang, X. Zhu and Y. Wan, Copper-catalyzed domino reactions for the synthesis of phenothiazines, Eur. J. Org. Chem., 2015, 4835–4839 CrossRef CAS.
-
(a) Y. M. Lin, G. P. Lu, R. K. Wang and W.-bin Yi, Radical route to 1,4-benzothiazine derivatives from 2-aminobenzenethiols and ketones under transition-metal-free conditions, Org. Lett., 2016, 18, 6424–6427 CrossRef CAS PubMed;
(b) S. Wu, W.-Y. Hu and S. L. Zhang, Potassium carbonate-mediated tandem C-S and C-N coupling reaction for the synthesis of phenothiazines under transition-metal-free and ligand-free condition, RSC Adv., 2016, 6, 24257–24260 RSC;
(c) Y. Zhou, Q. L. Zeng and L. Zhang, Transition-metal-free synthesis of phenothiazines from S-2-acetamidophenyl ethanethioate and ortho-dihaloarenes, Synth. Commun., 2017, 47, 710–715 CrossRef CAS.
- M. Kuratsu, M. Kozaki and K. Okada, Synthesis, structure, and electron-Donating ability of 2,2′: 6′,2′′-dioxatriphenylamine and its sulfur analogue, Chem. Lett., 2004, 33, 1174–1175 CrossRef CAS.
- G. Lamanna, C. Faggi, F. Gasparrini, A. Ciogli, C. Villani, P. J. Stephens, F. J. Devlin and S. Menichetti, Efficient thia-bridged triarylamine heterohelicenes: synthesis, resolution, and absolute configuration determination, Chem. – Eur. J., 2008, 14, 5747–5750 CrossRef CAS PubMed.
- S. Menichetti, C. Faggi, M. Onori, S. Piantini, M. Ferreira, S. Rocchi, M. Lupi, I. Marin, M. Maggini and C. Viglianisi, Thia-bridged triarylamine hetero[4]helicenes: regioselective synthesis and functionalization, Eur. J. Org. Chem., 2019, 168–175 CrossRef CAS.
- For selected examples, see:
(a) Z. T. Wang, Y. Wang, W.-X. Zhang, Z. M. Hou and Z. F. Xi, Efficient one-pot synthesis of 2,3-dihydropyrimidinthiones via multicomponent coupling of terminal alkynes, elemental sulfur, and carbodiimides, J. Am. Chem. Soc., 2009, 131, 15108–15109 CrossRef CAS PubMed;
(b) T. B. Nguyen, L. Ermolenko, P. Retailleau and A. Al-Mourabit, Elemental sulfur disproportionation in the redox condensation reaction between o-halonitrobenzenes and benzylamines, Angew. Chem., Int. Ed., 2014, 53, 13808–13812 CrossRef CAS PubMed;
(c) L. Meng, T. Fujikawa, M. Kuwayama, Y. Segawa and K. Itami, Thiophene-fused π-systems from diarylacetylenes and elemental sulfur, J. Am. Chem. Soc., 2016, 138, 10351–10355 CrossRef CAS PubMed;
(d) G. T. Zhang, C. Liu, H. Yi, Q. Y. Meng, C. L. Bian, H. Chen, J.-X. Jian, L.-Z. Wu and A. W. Lei, External oxidant-free oxidative cross-coupling: a photoredox cobalt-catalyzed aromatic C-H thiolation for constructing C-S Bonds, J. Am. Chem. Soc., 2015, 137, 9273–9280 CrossRef CAS PubMed;
(e) M. Wang, Z. H. Dai and X. F. Jiang, Design and application of α-ketothioesters as 1,2-dicarbonyl-forming reagents, Nat. Commun., 2019, 10, 2661 CrossRef PubMed;
(f) M. Saito, S. Murakami, T. Nanjo, Y. Kobayashi and Y. Takemoto, Mild and chemoselective thioacylation of amines enabled by the
nucleophilic activation of elemental sulfur, J. Am. Chem. Soc., 2020, 142, 8130–8135 CrossRef CAS PubMed.
-
(a) T. B. Nguyen, Recent advances in organic reactions involving elemental sulfur, Adv. Synth. Catal., 2017, 359, 1066–1130 CrossRef CAS;
(b) T. B. Nguyen, Recent advances in the synthesis of heterocycles via reactions involving elemental sulfur, Adv. Synth. Catal., 2020, 362, 3448–3484 CrossRef CAS;
(c) G. T. Zhang, H. Yi, H. Chen, C. L. Bian, C. Liu and A. W. Lei, Trisulfur radical anion as the key intermediate for the synthesis of thiophene via the interaction between elemental sulfur and NaOtBu, Org. Lett., 2014, 16, 6156–6159 CrossRef CAS PubMed;
(d) T. B. Nguyen and P. Retailleau, DIPEA-promoted reaction of 2-nitrochalcones with elemental sulfur: an unusual approach to 2-benzoylbenzothiophenes, Org. Lett., 2017, 19, 4858–4860 CrossRef CAS PubMed;
(e) P. Q. Zhou, Y. B. Huang, W. Q. Wu, J. M. Zhou, W. T. Yu and H. F. Jiang, Access to 2-aroylthienothiazoles via C-H/N-O bond functionalization of oximes, Org. Lett., 2019, 21, 9976–9980 CrossRef CAS PubMed.
-
(a) M. Wang, Q. L. Fan and X. F. Jiang, Transition-metal-free diarylannulated sulfide and selenide construction via radical/anion-mediated sulfur-iodine and selenium-iodine exchange, Org. Lett., 2016, 18, 5756–5759 CrossRef CAS PubMed;
(b) T. Shoji, K. Miura, A. Ohta, R. Sekeguchi, S. Ito, Y. Endo, T. Nagahata, S. Mori and T. Okujima, Synthesis of azuleno[2,1-b]thiophenes by cycloaddition of azulenylalkynes with elemental sulfur and their structural, optical and electrochemical properties, Org. Chem. Front., 2019, 6, 2801–2811 RSC;
(c) C.-K. Ran, L. Song, Y.-N. Niu, M.-K. Wei, Z. Zhang, X.-Y. Zhou and D.-G. Yu, Transition-metal-free synthesis of thiazolidin-2-ones and 1,3-thiazinan-2-ones from arylamines, elemental sulfur and CO2, Green Chem., 2021, 23, 274–279 RSC;
(d) Q. H. Chen, R. Xie, H. H. Jia, J. L. Sun, G. P. Lu, H. F. Jiang and M. Zhang, Access to phenothiazine derivatives via iodide-mediated oxidative three-component annulation reaction, J. Org. Chem., 2020, 85, 5629–5637 CrossRef CAS PubMed;
(e) X. X. Ma, X. X. Yu, H. Huang, Y. Zhou and Q. L. Song, Synthesis of thiazoles and isothiazoles via three-component reaction of enaminoesters, sulfur, and bromodifluoroacetamides/esters, Org. Lett., 2020, 22, 5284–5288 CrossRef CAS PubMed;
(f) X. Wang, X. Qiu, J. Wei, J. Liu, S. Song, W. Wang and N. Jiao, Cu-catalyzed aerobic oxidative sulfuration/annulation approach to thiazoles via multiple Csp3-H Bond Cleavage, Org. Lett., 2018, 20, 2632–2636 CrossRef CAS PubMed.
-
(a) Y. J. Xie, J. Wu, X. G. Che, Y. Chen, H. W. Huang and G. J. Deng, Efficient pyrido[1,2-a]benzimidazole formation from 2-aminopyridines and cyclohexanones under metal-free Conditions, Green Chem., 2016, 18, 667–671 RSC;
(b) Y. Liao, Y. Peng, H. Qi, G. J. Deng, H. Gong and C. J. Li, Palladium-catalyzed benzothieno[2,3-b]indole formation via dehydrative-dehydrogenative double C-H sulfuration using sulfur powder, indoles and cyclohexanones, Chem. Commun., 2015, 51, 1031–1034 RSC;
(c) P. Jiang, S. Chen, Y. Xia, Q. Zhang and G. J. Deng, Access to dibenzofurans through dimerization/trimerization of cyclohexanones followed by dehydroaromatization, Org. Lett., 2020, 22, 8076–8081 CrossRef CAS PubMed;
(d) P. Jiang, S. Chen, H. Huang, K. Hu, Y. Xia and G. J. Deng, Metal-free synthesis of indolo[2,3-b]indoles through aerobic cascade ddehydrogenative aromatization/oxidative annulation, Green Synth. Catal., 2021, 2, 78–81 CrossRef;
(e) P. Y. Jiang, Z. F. Shan, S. P. Chen, Q. Y. Wang, S. X. Jiang, H. L. Zheng and G.-J. Deng, Metal-free synthesis of benzo[a]phenanthridines from aromatic aldehydes, cyclohexanones, and aromatic amines, Chin. J. Chem., 2022, 40, 365–370 CrossRef CAS.
-
(a) Q. Y. Xing, F. H. Xiao, G. J. Mao and G.-J. Deng, A four-component reaction for the synthesis of thienopyrrolediones under transition metal free conditions, Org. Lett., 2022, 24, 4377–4382 CrossRef CAS PubMed;
(b) Z. Xu, G.-J. Deng, F. Zhang, H. Chen and H. Huang, Three-component cascade bis-heteroannulation of aryl or vinyl methylketoxime acetates toward thieno[3,2-c]isoquinolines, Org. Lett., 2019, 21, 8630–8634 CrossRef CAS PubMed;
(c) Z. Xu, H. Huang, H. Chen and G.-J. Deng, Catalyst-and additive-free annulation/aromatization leading to benzothiazoles and naphthothiazoles, Org. Chem. Front., 2019, 6, 3060–3064 RSC;
(d) J. Jiang, H. Huang and G.-J. Deng, Four-component thiazole formation from simple chemicals under metal-free conditions, Green Chem., 2019, 21, 986–990 RSC.
-
(a) J. J. Chen, G. Z. Li, Y. J. Xie, Y. F. Liao, F. H. Xiao and G.-J. Deng, Four-component approach to N-substituted phenothiazines under transition-metal-free conditions, Org. Lett., 2015, 17, 5870–5873 CrossRef CAS PubMed;
(b) P. Ni, H. Huang, F. Xiao and G.-J. Deng, Solvent-controlled highly regio-selective thieno[2,3-b]indole formation under metal-free conditions, Green Chem., 2017, 19, 5553–5558 RSC;
(c) C. Lu, H. Huang, X. Tuo, P. Jiang, F. Zhang and G.-J. Deng, Chemoselective metal-free indole arylation with cyclohexanones, Org. Chem. Front., 2019, 6, 2738–2743 RSC;
(d) X. Che, J. Jiang, F. Xiao, H. Huang and G.-J. Deng, Assembly of 2-arylbenzothiazoles through three-component oxidative annulation under transition-metal-free conditions, Org. Lett., 2017, 19, 4576–4579 CrossRef CAS PubMed.
- The CCDC number of 3a: 2112855.†.
- D. L. Priebbenow and C. Bolm, Recent advances in the Willgerodt-Kindler reaction, Chem. Soc. Rev., 2013, 42, 7870–7880 RSC.
Footnotes |
† Electronic supplementary information (ESI) available. CCDC 2112855. For ESI and crystallographic data in CIF or other electronic format see DOI: https://doi.org/10.1039/d3ob00055a |
‡ These authors contributed equally to this work. |
|
This journal is © The Royal Society of Chemistry 2023 |
Click here to see how this site uses Cookies. View our privacy policy here.