Rearrangement of thiazolidine derivatives – a synthesis of a chiral fused oxathiane–γ-lactam bicyclic system†
Received
10th September 2023
, Accepted 6th November 2023
First published on 6th November 2023
Abstract
Reaction of L-cysteine with carbonyl compounds leads to thiazolidine derivatives which undergo a stereoselective conversion to two types of chiral bicyclic products bearing two or three stereogenic centers, including the first fused oxathiane–γ-lactam system.
Introduction
Stereoselective preparation of chiral bicyclic lactams has attracted attention due to their wide application in organic synthesis and medicinal chemistry. Vince and Meyers’ lactams (A and B in Fig. 1) serve as useful building blocks for the asymmetric synthesis of bioactive heterocycles.1,2 Another important system − penam − composed of β-lactam and thiazolidine rings sharing a C–N bond (Fig. 1, C) is mainly responsible for the antibacterial activity of penicillins, while the spectrum of action can be modified by appropriate substitution.3 Hydrolytic instability hinders efficient isolation of these compounds. Thiazolidines themselves undergo ring–chain tautomerism.4 Fülöp and co-workers demonstrated that in most cases the ring form predominates in the equilibrium mixture.5,6 Further conversion to the corresponding aldehydes and aminothiols (cysteine derivatives) was observed under acidic or basic conditions.7,8 The reaction mechanism involves C–S or C–N bond breaking and formation of an iminium (III) or a sulfonium intermediate (IV), respectively (Scheme 1).
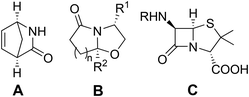 |
| Fig. 1 Examples of important chiral bicyclic lactams: A, Vince lactam (one enantiomer shown), B, Meyers’ lactams, and C, penicillins with the penam core. | |
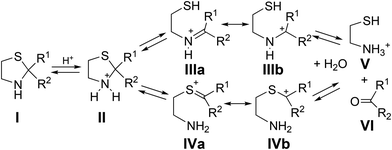 |
| Scheme 1 Equilibria involving the thiazolidine moiety under acidic conditions. | |
Inspired also by the wide therapeutic potential of thiazolidines,9 which constitute a structural motif present in many siderophores – bacterial iron-acquiring systems,10 we decided to prepare new bicyclic derivatives combining this heterocycle with the lactam moiety (penam analogs).
Results and discussion
We commenced our synthesis of thiazolidine derivatives with the preparation of β-hydroxy esters 12–16 using literature-based methodologies.11–13L-Cysteine was reacted with three different aldehydes; a subsequent Boc protection led to enantiopure (2R,4R)-thiazolidine-4-carboxylic acids 2–4 in good yields (>80%; Scheme 2).14,15 For 2,2-disubstituted derivatives 5 and 6, L-cysteine was refluxed with ketones, and after purification, Boc-protection was introduced in the presence of pyridine.11,13,16 Compound 6 was obtained as an equimolar mixture of both epimers differing in the configuration on C-2.
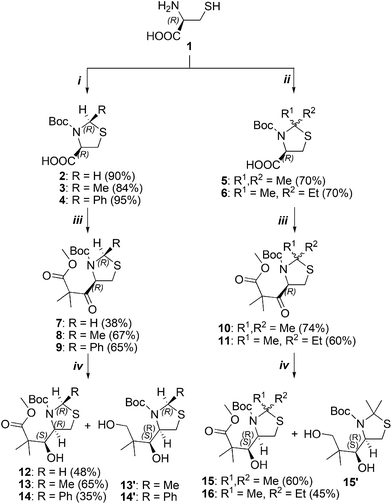 |
| Scheme 2 Synthesis of chiral β-hydroxy esters 12–16 with the thiazolidine core. Reagents and conditions: (i) 2 M NaOH(aq), aldehyde (1 eq.), acetone, NaOH, NH2OH·HCl, Boc2O (1.1 eq.). (ii) Ketone (1 eq.), reflux; then Boc2O (1.1 eq.), pyridine. (iii) CDI (1 eq.), Me2CHCO2Me (2.1 eq.), LDA (1.5 eq.), n-BuLi (1.5 eq.), DIPA (1.5 eq.). (iv) NaBH4 (1 eq.). | |
Keto-ester derivatives 7–11 were synthesized through CDI treatment and a subsequent condensation with methyl isobutyrate. A stereoselective reduction of prochiral ketones was performed using sodium borohydride in ethanol, and the desired (S)-alcohols 12–16 were obtained in agreement with Cram's rule along with trace amounts of (R) isomers and over-reduced diols (easily separable by chromatography; derivatives 13′–15′ were isolated and purified). The stereochemistry of the products was confirmed by X-ray crystallography (for compounds 3, 4, and 7, see the ESI†) and 2D NMR techniques.
Aiming to obtain bicyclic compounds by the reaction of an amine nucleophile and an ester carbonyl group, Boc removal under acidic conditions followed by neutralization was carried out. This approach enabled us to develop an efficient and stereoselective route to novel bicyclic thiazolidine derivatives. We found that substitution of the thiazolidine part has a prominent effect on the structure of the final product. Compounds 12 and 13 after deprotection and neutralization using ammonia did not rearrange to the bicyclic products. Instead, the corresponding esters 17 and 18 were afforded. Interestingly, we observed epimerization at the C-2 position in the thiazolidine part of 18 as demonstrated by two sets of signals in the 1H NMR spectra which appeared after base treatment (Fig. 2). Only one set of resonances was observed for compound 17 lacking the C-2 stereocenter, which confirmed the involvement of this position in the epimerization process. To examine the effect of the base on the observed configuration change, we checked sterically differentiated amines, such as pyridine and DIPEA. The final effect was the same: a mixture of epimers in a 70
:
30 ratio was formed (Fig. 2). Additional heating of compounds 17 and 18 in refluxing toluene was performed to check the possible rearrangement to the bicyclic product. After 48 h of reflux we observed the formation of new products (Scheme 3). An expected bicyclic structure preserving the thiazolidine core condensed with a γ-lactam system was obtained. The reaction proceeded in good yield, and only trace amounts of substrates and another bicyclic product were observed. The main products were isolated as single stereoisomers, and their configurations were established using 2D NMR as well as the X-ray structure of compound 21 (Scheme 3; see also the comparison of the 1H NMR spectra of 18 and 21, Fig. S19, in the ESI†). Interestingly, an inverted configuration of C-2 in this final product (S compared to R in the starting thiazolidine 13) was found.
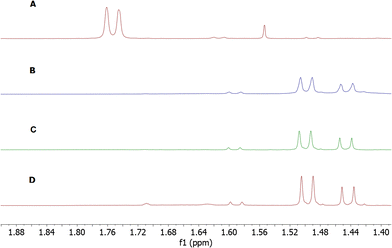 |
| Fig. 2 Fragments of 1H NMR spectra (400 MHz, CDCl3) recorded upon transformation of 13 into 18: (A) the reaction mixture after addition of TFA; effects of (B) alkalization with DIPEA, (C) alkalization with pyridine and (D) alkalization with 25% aqueous ammonia. | |
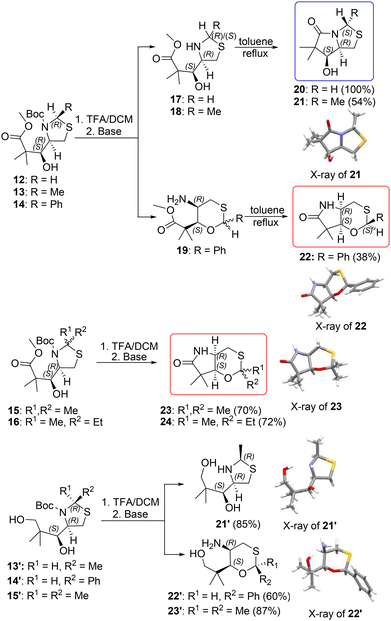 |
| Scheme 3 Ring opening–closure rearrangement of thiazolidine-based alcohols 12–16. Reagents and conditions: (i) TFA/CH2Cl2, base. (ii) Toluene, reflux. For 21′, a major epimer is shown. | |
In contrast, a 2-phenyl substituted derivative 14 underwent a two-step rearrangement to a completely different bicyclic enantiopure product composed of γ-lactam and 1,3-oxathiane 22 (Scheme 3). Again, the (S) configuration of C-2 was noted, while the stereocenters in the lactam part remained unchanged, as confirmed by the crystal structure of this derivative.
For comparison, 2,2-disubstituted thiazolidine derivatives 15 and 16 (the latter as a mixture of two epimers) were deprotected with trifluoroacetic acid at room temperature. The workup under basic conditions yielded again rearranged bicyclic products 22 and 23, respectively (Scheme 3). The formation of oxathiane derivative 22 was confirmed by X-ray diffraction measurement.
In order to explain the observed transformation a mechanism should be proposed taking into account structural factors and reaction conditions, as well as the stereochemistry of the process (Scheme 4). Retained configurations of two stereogenic centers and epimerization followed by configuration change at C-2 indicate fragments engaged in the process. This is in agreement with the formation of 1,3-oxathiane which requires breaking of the C-2–N bond.
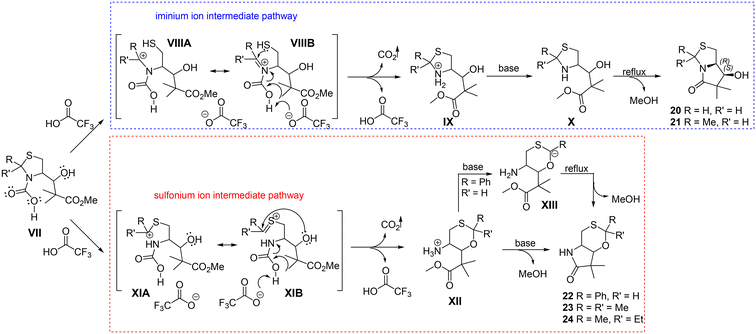 |
| Scheme 4 A proposed mechanism including iminium ion (blue pathway) and sulfonium ion intermediates (red pathway). For simplicity, the Boc removal step is omitted. | |
Under acidic conditions, ring–chain tautomerization of thiazolidine takes place including both cationic iminium and sulfonium ion intermediates as previously described.7 However, the use of this type of transformation in the stereoselective synthesis of multifunctionalized lactams has not been known so far. The simple procedure of deprotection in an acidic medium and subsequent neutralization revealed an interesting rearrangement of thiazolidine with wide synthetic possibilities.
The 2-methyl substituted thiazolidine β-hydroxy ester 13 or unsubstituted analogue 12 treated with acid resulted in cationic iminium species VIII as a predominant intermediate (blue pathway, Scheme 4). The positive charge is partially located on the neighboring carbon atom which is immediately attacked by the thiol nucleophile. This results in the reconstruction of the thiazolidine ring. After neutralization of 2-methyl substituted thiazolidine IX using different bases, epimerization occurs at the C-2 position which can be explained by α-hydrogen exchange. Upon refluxing X in toluene an intramolecular nucleophilic substitution takes place leading to the formation of the lactam ring, accompanied by the inversion of the configuration at the C-2 position of 21 leading to enantiopure products.
The 2,2-disubstituted thiazolidines 15 and 16 under acidic conditions might generate sulfonium ion XI as a predominant species due to the additional stabilization of its resonance form, the carbenium ion, via higher order of the positively charged carbon atom (red pathway, Scheme 4). Yoshida et al. revealed that the structure and properties of the sulfonium ion may be explained by the combination of the two resonance forms, i.e. the sulfonium ion and the carbenium ion stabilized by the adjacent sulfur.16 Here, the carbocation can be trapped by the hydroxyl group present in the molecule acting as a nucleophile. As a result, 1,3-oxathiane intermediate XII is formed. Direct formation of products 23 and 24 bearing 1,3-oxathiane condensed with the γ-lactam system was observed after neutralization. A similar mechanism of 1,3-oxathiane formation can be proposed for the 2-phenyl substituted derivative 22. The aromatic system at the C-2 position in the thiazolidine ring would stabilize the sulfonium ion type intermediate XI by conjugation. The observed epimerization at the C-2 position may be caused by the acidity of the H-2 atom. Lactam formation by a nucleophilic substitution reaction between the secondary amine group and the carbonyl moiety is accomplished after refluxing XIII in toluene, yielding the bicyclic product in a stereoselective manner.
Additional experiments were performed to explain the relationship between ring–chain tautomerism and nucleophilic substitution occurring during synthesis. In order to achieve this goal we used previously obtained over-reduced diols 13′–15′ (obtained as side products during reduction of keto-esters 8–10 with NaBH4; Scheme 2). The lack of electrophilic species in the structures of these thiazolidines eliminates the possibility of nucleophilic substitution and formation of a bicyclic system. After the Boc-deprotection reaction (TFA/DCM) and subsequent basification we observed a preserved thiazolidine system for compound 21′ formed from 13′, and the formation of the oxathiane cycle for 22′ and 23′ (Scheme 3). The obtained results confirmed the dependence of the formation of various intermediate ions on substitution in the C-2 position. In addition, 21′ was formed as a mixture of epimers in a 60
:
40 (R)/(S) ratio. Epimerization at C-2 proves thiazolidine ring opening during the process; on the other hand, full diastereoselectivity is not observed when a bicyclic system is not formed. Nucleophilic substitution leading to lactam formation leading to 22–24 is a subsequent transformation following the observed rearrangement.
DFT calculations were performed to shed more light on the factors determining the 13 → 21 and 16 → 24 reaction pathways. For every structure, conformational analysis was performed to identify the most stable species, and despite conformational flexibility, only one or two conformers dominated due to the intermolecular hydrogen bond interactions. In every reaction step, both epimers (differing by the configuration on C-2) were included. Two alternative paths with protonation on the nitrogen or sulfur atoms and simultaneous breaking of the N–C or S–C bonds were taken into account, respectively (Scheme S1, ESI†). The calculations confirmed that the presence of two substituents at the C-2 atom (compound 16) leads to the formation of a very stable structure in which protonation takes place at the N atom (Fig. S24,† subB_NH). Subsequently, the C-2–N bond is broken, and a simple rotation about the single C–S bond leads to the closure of the 1,3-oxathiane ring (Fig. S24,†24_H). During the 16 → 24 conversion, the C-2 configuration is preserved, yet the (R)/(S)-conformer ratio is ca. 35%/65%. However, when compound 13 is used, the protonation on the S-atom is not straightforward and takes place in the presence of excess H+. Then, the C-2–S bond breaking takes place during the protonation and CO2 release. Epimerization at C-2 occurs as the bicyclic product (S)-21 is thermodynamically and kinetically favored over the (R)-enantiomer (Fig. S26†). The activation barrier between (S)-18 and (S)-21 is lower by 2.74 kcal mol−1 than for the corresponding (R)-enantiomers (Fig. S27†). The details of the calculations (methodology, calculated geometries, and energies) are gathered in the ESI.†
Experimental
General
All commercially available reagents were purchased from Merck and used without further purification. 1H and 13C NMR spectra were recorded using 400 MHz JEOL 400YH (JEOL Ltd, Tokyo, Japan) and 600 MHz Bruker Avance II 600 (Bruker, Billerica, MA, USA) instruments. If not stated otherwise, reference solvent residual peaks were used for calibration (chloroform-d, δ(1H) = 7.28 ppm, δ(13C) = 77.00 ppm and acetonitrile-d δ(1H) = 1.94 ppm, δ(13C) = 1.32, 118.26 ppm). The reported J values are those observed from the splitting patterns in the spectrum and may not reflect the true coupling constant values. IR spectra were obtained from either potassium bromide pellets or liquid films with a PerkinElmer 2000 FTIR spectrometer (PerkinElmer, Waltham, MA, USA). High resolution mass spectra were recorded (Waters LCT Premier XE TOF spectrometer, Waters Corporation, Milford, MA, USA) utilizing the electrospray ionization mode. Melting points were determined by the open capillary method using Apotec® Schmelzpunktbestimmer melting point apparatus (WEPA Apothekenbedarf GmbH & Co. KG., Hillscheid, Germany). The optical rotation was measured on an automatic polarimeter model AA-5 (Optical Activity, Ltd, Ramsey, UK, [α]20D values are expressed in 10−1 deg cm2 g−1). Liquid chromatography purification was performed on silica gel 60 (70–230 mesh). Thin layer chromatography was carried out using silica gel 60 precoated plates (Merck).
Synthetic procedures
Synthesis of aldehyde-derived Boc-protected carboxylic acids (2–4).
General procedure A (compounds 2–4), according to ref 13.
To a 100 ml round-bottom flask containing L-cysteine (3.63 g, 30 mmol), NaOH (2 M, 1.20 g in 15 mL H2O, 30 mmol, 1.0 eq.) was added at 0 °C, followed by aldehyde (3 mmol, 0.1 eq.). The reaction mixture was stirred for 24 h at the same temperature. Then, acetone (17.5 mL), NaOH (1.2 M, 0.12 g in 2.5 mL H2O, 3 mmol, 0.1 eq.) and hydroxylamine hydrochloride (0.21 g, 3 mmol, 0.1 eq.) were added. After stirring for 15 minutes at 0 °C, (Boc)2O (7.20 g, 33 mmol, 1.1 eq.) was introduced and stirring was continued for 3 h at room temperature. The mixture was diluted with water, followed by washing with diethyl ether (2 × 100 mL). The aqueous phase was quenched with 20% citric acid solution and extracted with ethyl acetate (2 × 100 mL). The organic extracts were dried over anhydrous Na2SO4, filtered and concentrated under reduced pressure. After purification by column chromatography (silica gel, n-hexane/EtOAc = 6
:
1), the desired Boc-protected products 2–4 were obtained.
(R)-3-(tert-Butoxycarbonyl)thiazolidine-4-carboxylic acid (2).
Prepared according to the General procedure A from L-cysteine (30 mmol) and formaldehyde (3 mmol, 0.1 eq.); product 2 was obtained as a white crystalline solid (6.28 g, 26.9 mmol, 90% yield). M. p. 132–134 °C, [α]20D = −130.8 (c 0.50, DCM). 1H NMR (400 MHz, CDCl3): δ 10.40 (br s, 1H), 4.87–4.41 (m, 3H), 3.35–3.27 (m, 2H), 1.46 (s, 9H), ppm. 13C NMR (100 MHz, CDCl3): δ 176.4, 175.0, 154.0, 153.2, 82.0, 81.7, 61.4, 49.0, 48.4, 34.5, 32.9, 28.3, ppm. IR (KBr): 3433, 2971, 2935, 1746, 1635, 1419, 1217, 1197 cm−1. HRMS (ESI-TOF): m/z [M + H]+ calcd for (C9H15NO4SNa)+ 256.0620; found 256.0621.
(2R,4R)-3-(tert-Butoxycarbonyl)-2-methylthiazolidine-4-carboxylic acid (3).
Prepared according to the General procedure A from L-cysteine (30 mmol) and acetaldehyde (3 mmol, 0.1 eq.); product 3 was obtained as white needles (6.23 g, 25.2 mmol, 84% yield). M. p. 119–121 °C, [α]20D = −95.2 (c 0.69, DCM). 1H NMR (CDCl3, 400 MHz): δ 9.38 (br s, 1H), 5.20–5.14 (m, 1H), 4.85–4.67 (m, 1H), 3.40–3.31 (m, 2H), 1.57 (d, J = 5.2 Hz, 3H), 1.47 (s, 9H) ppm. 13C NMR (150 MHz, CDCl3): δ 177.2, 175.6, 153.7, 152.4, 81.8, 63.9, 60.3, 59.5, 33.1, 32.0, 28.3, 23.6 ppm. IR (KBr): 2976, 2611, 1728, 1621, 1428, 1351, 1286, 1270, 1235, 1216, 1142, 1043, 861, 839 cm−1. HRMS (ESI-TOF): m/z [M + H]+ calcd for (C10H17NO4SNa)+ 270.0776; found 270.0781.
(2R,4R)-3-(tert-Butoxycarbonyl)-2-phenylthiazolidine-4-carboxylic acid (4).
Prepared according to the General procedure A from L-cysteine (30 mmol) and benzaldehyde (3 mmol, 0.1 eq.); product 4 was obtained as a white solid (8.81 g, 28.5 mmol, 95% yield). 1H NMR (400 MHz, CDCl3) δ 7.47–7.21 (m, 5H), 5.93 (s, 1H), 4.90 (s, 1H), 3.48–3.45 (m, 1H), 3.32 (dd, J = 11.8, 6.7 Hz, 1H), 1.32 (s, 9H) ppm. The spectroscopic data are in agreement with the literature data.17
Synthesis of ketone-derived Boc-protected carboxylic acids (5–6).
General procedure B (compounds 5–6), according to refs. 12 and 15.
Step 1: To a 250 ml round-bottom flask containing L-cysteine (2.42 g, 20 mmol), 200 ml of ketone was added under an argon atmosphere. The resulting suspension was refluxed for 3 h. The reaction mixture was cooled to room temperature and the product was separated from the unreacted ketone and side products by filtration. The obtained carboxylic acid was used directly in the next step, without additional purification.
Step 2: In a 100 ml round-bottom flask under argon, carboxylic acid (20 mmol) was dissolved in pyridine (25 ml), and (Boc)2O (4.80 g, 22 mmol, 1.1 eq.) was added at −25 °C. The reaction mixture was stirred for 72 h at room temperature. Then, it was diluted with toluene and extracted with 2 N ice-cold NaOH solution (3 × 80 ml). The aqueous layers were combined and washed with toluene (3 × 100 ml) and hexane (100 ml). After bringing the pH down to 3 with solid citric acid, extraction with DCM (3 × 100 ml) was performed. The organic layers were washed with brine, dried over anhydrous Na2SO4, filtered and concentrated under reduced pressure to afford the crude product. Purification by column chromatography (silica gel, n-hexane/EtOAc: 9
:
1 → 1
:
1) yielded pure Boc-protected carboxylic acids 5–6.
(R)-3-(tert-Butoxycarbonyl)-2,2-dimethylthiazolidine-4-carboxylic acid (5).
Prepared according to the General procedure B from L-cysteine (20 mmol) and acetone (200 ml); product 5 was obtained as a white solid (3.65 g, 14.0 mmol, 70% yield over two steps). 1H NMR (400 MHz, CDCl3): δ 8.38 (br s, 1H), 5.02–4.75 (m, 1H), 3.32–3.10 (m, 2H), 1.85 (s) and 1.78 (s, 6H), 1.49 (s) and 1.41 (s) (9H) ppm. The spectroscopic data are in agreement with the literature data.12
Mixture of (2S,4R)-3-(tert-butoxycarbonyl)-2-ethyl-2-methylthiazolidine-4-carboxylic acid and (2R,4R)-3-(tert-butoxycarbonyl)-2-ethyl-2-methylthiazolidine-4-carboxylic acid (6).
Prepared according to the General procedure B from L-cysteine (20 mmol) and 2-butanone (200 ml); a mixture of products 6 was obtained as a colorless oil (3.85 g, 14.0 mmol, 70% yield over two steps). 1H NMR (400 MHz, CDCl3): δ 9.38 (br s, 1H), 4.96–4.88 (m, 1H), 3.32–3.17 (m, 2H), 2.20–2.08 (m, 2H), 1.72 (s, 3H), 1.41 (s, 9H), 0.98 (t, J = 8.0 Hz, 3H) (major diastereoisomer); 9.38 (br s, 1H), 4.83–4.76 (m, 1H), 3.16–3.08 (m, 2H), 2.34–2.21 (m, 2H), 1.72 (s, 3H), 1.49 (s, 9H), 0.95 (t, J = 8.0 Hz, 3H) (minor diastereoisomer) ppm. The spectroscopic data are in agreement with the literature data.15
Synthesis of ketones (7–11).
General procedure C (compounds 7–11), according to ref 18.
Step 1: Carboxylic acid 2–6 (12 mmol) was dissolved in dry THF (20 ml) at 0 °C, and CDI (1.95 g, 12 mmol, 1.0 eq.) was added. After stirring for 30 min, the ice bath was removed and the reaction mixture was stirred for another 2 h at room temperature. The mixture was briefly dried under reduced pressure. The residue was transferred to a separating funnel, diluted utilizing EtOAc and washed with distilled water and brine. The organic extract was dried over anhydrous Na2SO4, filtered and the solvent was evaporated to obtain activated carboxylic acid.
Step 2: LDA solution was prepared at −78 °C under an argon atmosphere, by adding DIPA (2.52 ml, 18 mmol, 1.5 eq.) to a solution of n-BuLi (2.5 M, 7.2 ml, 18 mmol, 1.5 eq.) in dry THF (15 ml). After stirring for 30 min at the same temperature, methyl isobutyrate (2.89 ml, 25.2 mmol, 2.1 eq.) was added dropwise over a period of 10 min at −78 °C and stirred at that temperature for another 30 min. Then, acylimidazole was dissolved in dry THF (10 ml) and added dropwise over 25 min. It was kept stirring for 30 min at the same temperature. The unreacted reagents were deactivated with 10% citric acid solution, followed by extraction with EtOAc (2 × 80 ml). Then, the organic extracts were combined and washed with water and brine. After drying it over anhydrous Na2SO4 and filtering, solvent evaporation in vacuo was performed to give crude products 7–11, which were then purified by column chromatography (silica gel, n-hexane/EtOAc = 9
:
1).
(R)-tert-Butyl 4-(3-methoxy-2,2-dimethyl-3-oxopropanoyl)thiazolidine-3-carboxylate (7).
Prepared according to the General procedure C from carboxylic acid 2 (2.80 g, 12 mmol); product 7 was obtained as a crystalline solid (1.45 g, 4.57 mmol, 38% yield over two steps). M. p. 66–68 °C, [α]20D = −114.0 (c 0.39, DCM). 1H NMR (400 MHz, CDCl3): δ 5.16 (br s, 1H), 4.75–4.66 (m, 1H), 4.35 (d, J = 8.0 Hz, 1H), 3.72 (s, 3H), 3.19 (br s, 1H), 3.04 (dd, J = 11.6, 4.8 Hz, 1H), 1.49 (s, 3H), 1.42 (m, 12H) ppm. 13C NMR (150 MHz, CDCl3): δ 205.6, 173.6, 153.2, 128.1, 128.0, 81.3, 63.3, 52.7, 49.6, 33.3, 28.4, 22.9, 22.7 ppm. IR (KBr): 2980, 2938, 1741, 1720, 1390, 1368, 1288, 1254, 1203, 1153 cm−1. HRMS (ESI-TOF): m/z [M + H]+ calcd for (C14H23NO5SNa)+ 340.1195; found 340.1196.
(2R,4R)-tert-Butyl 4-(3-methoxy-2,2-dimethyl-3-oxopropanoyl)-2-methylthiazolidine-3-carboxylate (8).
Prepared according to the General procedure C from carboxylic acid 3 (2.97 g, 12 mmol); product 8 was obtained as a colorless oil (2.67 g, 8.06 mmol, 67% yield over two steps). [α]20D = +55.3 (c 0.60, DCM). 1H NMR (CDCl3, 400 MHz): δ 5.13–5.09 (m, 2H), 3.73 (s, 3H), 3.44–3.39 (m, 1H), 2.98 (m, 1H), 1.53 (d, 3H), 1.51 (s, 3H), 1.42 (s, 3H), 1.41 (s, 9H) ppm. 13C NMR (150 MHz, CDCl3): δ 205.9, 173.0, 151.8, 79.9, 63.7, 58.2, 51.6, 29.9, 29.6, 27.3, 24.0, 22.3 ppm. IR (KBr): 3442, 2978, 2931, 1743, 1721, 1699, 1459, 1366, 1267, 1173, 1153, 772 cm−1. HRMS (ESI-TOF): m/z [M + H]+ calcd for (C15H25NO5SNa)+ 354.1351; found 354.1347.
(2R,4R)-tert-Butyl 4-(3-methoxy-2,2-dimethyl-3-oxopropanoyl)-2-phenylthiazolidine-3-carboxylate (9).
Prepared according to the General procedure C from carboxylic acid 4 (3.71 g, 12 mmol); product 9 was obtained as white crystals (3.07 g, 7.80 mmol, 65% yield over two steps). M. p. 73–74 °C. [α]20D = +62.9 (c 0.86, DCM). 1H NMR (CDCl3, 400 MHz): δ 7.66–7.68 (m, 2H), 7.32–7.35 (m, 2H), 7.23–7.26 (m, 1H), 5.96 (s, 1H), 5.16 (t, J = 7.6 Hz, 1H), 3.74 (s, 3H), 3.28–3.18 (m, 1H), 3.04–3.00 (m, 1H), 1.62 (s, 3H), 1.50 (s, 3H), 1.19 (s, 9H) ppm. 13C NMR (150 MHz, CDCl3): δ 204.8, 173.8, 153.1, 142.6, 128.3, 127.6, 126.5, 81.2, 65.8, 55.7, 52.7, 33.2, 31.7, 28.1, 23.1, 14.2 ppm. IR (KBr): 3502, 2981, 2926, 1717, 1700, 1683, 1381, 1120, 696 cm−1. HRMS (ESI-TOF): m/z [M + H]+ calcd for (C20H27NO5S)+ 394.1688; found 394.1694.
(R)-tert-Butyl 4-(3-methoxy-2,2-dimethyl-3-oxopropanoyl)-2,2-dimethylthiazolidine-3-carboxylate (10).
Prepared according to the General procedure C from carboxylic acid 5 (3.14 g, 12 mmol); product 10 was obtained as a crystalline solid (3.07 g, 8.89 mmol, 74% yield over two steps). 1H NMR (400 MHz, CDCl3): δ 5.26 (br s, 1H), 3.72 (s, 3H), 3.21 (br s, 1H), 2.91 (dd, J = 12.3, 4.0 Hz, 1H), 1.84 (br s, 3H), 1.75 (s, 3H), 1.53 (s, 3H), 1.44 (s, 3H), 1.42 (s, 9H) ppm. The spectroscopic data are in agreement with the literature data.18
Mixture of (2S,4R)-tert-butyl 2-ethyl-4-(3-methoxy-2,2-dimethyl-3-oxopropanoyl)-2-methylthiazolidine-3-carboxylate and (2R,4R)-tert-butyl 2-ethyl-4-(3-methoxy-2,2-dimethyl-3-oxopropanoyl)-2-methylthiazolidine-3-carboxylate (11).
Prepared according to the General procedure C from carboxylic acid 6 (3.30 g, 12 mmol); a mixture of products 11 was obtained as a colorless oil (2.58 g, 7.18 mmol, 60% yield over two steps). 1H NMR (400 MHz, acetonitrile-d3) δ (major diastereomer) 5.25–5.16 (m, 1H), 3.69 (s, 3H), 3.36–3.16 (m, 1H), 2.96–2.80 (m, 1H), 2.30–2.08 (m, 2H), 1.69 (s, 3H), 1.49 (s, 3H), 1.41 (s, 9H), 1.40 (s, 3H), 1.02 (t, J = 7.2 Hz, 3H) ppm; (minor diastereomer) 5.35–5.27 (m, 1H), 3.69 (s, 3H), 3.36–3.16 (m, 1H), 3.07–2.98 (m, 1H), 2.30–2.08 (m, 2H), 1.71 (s, 3H), 1.44 (s, 3H), 1.41 (s, 9H), 1.40 (s, 3H), 0.95 (t, J = 7.2 Hz, 3H) ppm. HRMS (ESI-TOF): m/z [M + Na]+ calcd for (C17H29NO5SNa)+ 382.1664; found 382.1671.
Synthesis of alcohols (12–16).
General procedure D (compounds 12–16), according to ref. 18.
A solution of ketone 7–11 (3.5 mmol) in EtOH (15 ml) was prepared at 0 °C, and NaBH4 (0.132 g, 3.5 mmol, 1.0 eq.) was added in small portions. The reaction mixture was stirred for 48 h at room temperature. It was then carefully transferred to a separating funnel containing ice-cold NH4Cl solution, before being extracted with EtOAc (2 × 50 ml). The combined organic layers were washed with water and brine, dried over anhydrous Na2SO4, filtered and the solvent was evaporated in vacuo to obtain the crude product. Purification by column chromatography (silica gel, DCM) resulted in alcohols 12–16 and over-reduced diols 13′–15′.
(R)-tert-Butyl 4-((S)-1-hydroxy-3-methoxy-2,2-dimethyl-3-oxopropyl)thiazolidine-3-carboxylate (12).
Prepared according to the General procedure D from ketone 7 (1.11 g, 3.5 mmol); product 12 was obtained as a crystalline solid (0.54 g, 1.69 mmol, 48% yield). M. p. 96–98 °C, [α]20D = −38.6 (c 0.45, DCM). 1H NMR (CDCl3, 400 MHz): δ 4.79 (br s, 1H), 4.61–4.57 (m, 1H), 4.17 (d, J = 9.6 Hz, 1H), 3.68 (s, 3H), 3.63 (dd, J = 6.0, 8.0 Hz, 1H), 3.11 (dd, J = 7.2, 11.2 Hz, 1H), 2.84 (dd, J = 3.2, 11.2 Hz, 1H), 1.46 (s, 9H), 1.30 (s, 3H), 1.28 (s, 3H) ppm. 13C NMR (100 MHz, CDCl3): δ 177.9, 81.6, 79.4, 60.1, 52.2, 49.8, 46.3, 34.8, 28.4, 22.7, 21.8 ppm. IR (KBr): 3435, 2986, 2948, 1728, 1677, 1401, 1368, 1253, 1162, 1133, 1117 cm−1. HRMS (ESI-TOF): m/z [M + H]+ calcd for (C14H25NO5S)+ 320.1532; found 320.1539.
(2R,4R)-tert-Butyl 4-((S)-1-hydroxy-3-methoxy-2,2-dimethyl-3-oxopropyl)-2-methylthiazolidine-3-carboxylate (13).
Prepared according to the General procedure D from ketone 8 (1.16 g, 3.5 mmol); product 13 was obtained as a colorless oil (0.76 g, 2.28 mmol, 65% yield). [α]20D = −10.6 (c 0.87, DCM). 1H NMR (CDCl3, 400 MHz): δ 5.17 (q, J = 6.0 Hz, 1H), 4.48 (m, 1H), 3.82 (m, 1H), 3.69 (s, 3H), 3.08 (dd, J = 6.8, 12.0 Hz, 1H), 2.68 (dd, J = 2.8, 12.0 Hz, 1H), 1.55 (d, J = 6.8 Hz, 3H), 1.47 (s, 9H), 1.30 (s, 3H), 1.23 (s, 3H) ppm. 13C NMR (100 MHz, CDCl3): δ 177.4, 81.7, 78.1, 62.4, 58.9, 52.1, 47.1, 33.7, 28.4, 24.0, 22.7, 20.3 ppm. IR (KBr): 3465, 2978, 1728, 1697, 1456, 1389, 1369, 1260, 1168, 860, 773 cm−1. HRMS (ESI-TOF): m/z [M + H]+ calcd for (C15H27NO5S)+ 334.1688; found 334.1680.
(2R,4R)-tert-Butyl 4-((S)-1-hydroxy-3-methoxy-2,2-dimethyl-3-oxopropyl)-2-phenylthiazolidine-3-carboxylate (14).
Prepared according to the General procedure D from ketone 9 (1.38 g, 3.5 mmol); product 14 was obtained as a colorless oil (0.49 g, 1.24 mmol, 35% yield). [α]20D = +93.8 (c 0.54, DCM). 1H NMR (CDCl3, 400 MHz): δ 7.54–7.52 (m, 2H), 7.36–7.32 (m, 2H), 7.29–7.25 (m, 1H), 5.97 (s, 1H), 4.58–4.53 (m, 1H), 4.04 (t, J = 8.8 Hz, 1H), 3.67 (s, 3H), 3.18 (dd, J = 6.4, 12.4 Hz, 1H), 2.59 (dd, J = 2.4, 12.4 Hz, 1H), 1.36 (s, 3H), 1.28 (s, 3H), 1.20 (s, 9H) ppm. 13C NMR (100 MHz, CDCl3): δ 177.3, 140.7, 128.5, 127.9, 126.7, 82.1, 78.2, 66.3, 63.6, 52.0, 47.4, 34.0, 28.0, 23.4, 19.7 ppm. IR (KBr): 3440, 2979, 2951, 1729, 1665, 1386, 1368, 1261, 1163, 1132, 858, 732 cm−1. HRMS (ESI-TOF): m/z [M + H]+ calcd for (C20H29NO5S)+ 396.1845; found 396.1852.
(R)-tert-Butyl 4-((S)-1-hydroxy-3-methoxy-2,2-dimethyl-3-oxopropyl)-2,2-dimethylthiazolidine-3-carboxylate (15).
Prepared according to the General procedure D from ketone 10 (1.21 g, 3.5 mmol); product 15 was obtained as a crystalline solid (0.73 g, 2.10 mmol, 60% yield). 1H NMR (400 MHz, CDCl3): δ 4.54 (dd, J = 8.9, 5.5 Hz, 1H), 3.88 (dd, J = 9.9, 8.9 Hz, 1H), 3.68 (s, 3H), 3.15 (dd, J = 12.3, 5.5 Hz, 1H), 2.36 (d, J = 12.3 Hz, 1H), 1.76 (s, 6H), 1.46 (s, 9H), 1.29 (s, 3H), 1.23 (s, 3H) ppm. The spectroscopic data are in agreement with the literature data.18
Mixture of (2S,4R)-tert-butyl 2-ethyl-4-((S)-1-hydroxy-3-methoxy-2,2-dimethyl-3-oxopropyl)-2-methylthiazolidine-3-carboxylate and (2R,4R)-tert-butyl 2-ethyl-4-((S)-1-hydroxy-3-methoxy-2,2-dimethyl-3-oxopropyl)-2-methylthiazolidine-3-carboxylate (16).
Prepared according to the General procedure D from ketone 11 (1.26 g, 3.5 mmol); a mixture of products 16 was obtained as a colorless oil (0.57 g, 1.58 mmol, 45% yield). De = 35%. 1H NMR (400 MHz, CD3CN): δ (major diastereomer) 4.48 (dd, J = 4.0, 8.0 Hz, 1H), 3.71 (t, J = 4.0 Hz, 1H), 3.56 (s, 3H), 3.30–3.28 (m, 1H), 3.10 (dd, J = 4.0, 8.0 Hz, 1H), 2.32 (d, J = 12.0 Hz, 1H), 2.03 (q, J = 8.0 Hz, 2H), 1.67 (s, 3H), 1.38 (s, 9H), 1.16 (s, 3H), 1.11 (s, 3H), 0.93 (t, J = 8.0 Hz, 3H) ppm; (minor diastereomer) 4.53 (dd, J = 4.0, 8.0 Hz, 1H), 3.74 (t, J = 4.0 Hz, 1H), 3.57 (s, 3H), 3.05 (dd, J = 4.0, 8.0 Hz, 1H), 2.98–2.96 (m, 1H), 2.26 (d, J = 12.0 Hz, 1H), 1.99 (q, J = 8.0 Hz, 2H), 1.63 (s, 3H), 1.37 (s, 9H), 1.17 (s, 3H), 1.12 (s, 3H), 0.86 (t, J = 8.0 Hz, 3H) ppm. 13C NMR (100 MHz, CD3CN): δ (major diastereomer) 176.9, 81.0, 75.1, 77.4, 64.6, 51.3, 47.4, 32.8, 30.8, 28.0, 27.7, 21.9, 20.1, 9.52 ppm; (minor diastereomer) 176.9, 81.0, 75.1, 64.6, 51.4, 47.4, 34.9, 32.7, 30.8, 27.8, 27.6, 21.5, 20.5, 8.9 ppm. HRMS (ESI-TOF): m/z [M + Na]+ calcd for (C17H31NO5SNa)+ 384.1821; found 384.1814.
Synthesis of bicyclic products (20–24).
General procedure E (compounds 20–24).
To a solution of alcohol 12–16 or diol 13′–15′ (0.65 mmol) in dry DCM (1.0 ml), TFA (1.0 ml, 13.0 mmol, 20 eq.) was added dropwise at 0 °C under an argon atmosphere. The reaction mixture was then stirred for 30 minutes at room temperature and subsequently ammonia solution was added portionwise (pH > 7). The neutralized mixture was washed with DCM (3 × 10 ml) and the combined organic phases were dried over Na2SO4. Solvent was removed under reduced pressure; products 23 and 24 were purified using column chromatography (silica gel, n-hexane/EtOAc: 4
:
1 → 1
:
1) and products 21′–23′ were purified by crystallization from petroleum ether. Crude intermediates 17–19 were refluxed in toluene (20 ml) for 48 hours and after solvent evaporation, purified by column chromatography (silica gel, n-hexane/EtOAc: 4
:
1 → 1
:
1) to give products 20–22.
(7S,7aR)-7-Hydroxy-6,6-dimethyltetrahydropyrrolo[1,2-c]thiazol-5(3H)-one (20).
Prepared according to the General procedure E from alcohol 12 (0.208 g, 0.65 mmol); product 20 was obtained as a crystalline solid (0.122 g, 0.65 mmol, 100% yield). M. p. 102–104 °C, [α]20D = +40.4 (c 0.45). 1H NMR (CDCl3, 400 MHz): δ 4.81 (d, J = 9.6 Hz, 1H), 4.33–4.28 (m, 1H), 4.02 (d, J = 4.4 Hz, 1H), 3.98 (d, J = 9.2 Hz, 1H), 3.28 (dd, J = 6.8, 10.8 Hz, 1H), 2.96 (dd, J = 7.2, 11.2 Hz, 1H), 2.77 (br s, 1H), 1.25 (s, 3H), 1.16 (s, 3H) ppm. 13C NMR (100 MHz, CDCl3): δ 177.8, 76.1, 64.8, 50.1, 44.8, 29.4, 23.6, 18.1 ppm. IR (KBr): 3429, 2969, 2932, 1666, 1467, 1437, 1292, 1270, 1096 cm−1. HRMS (ESI-TOF): m/z [M + H]+ calcd for (C8H13NO2S)+ 188.0745; found 188.0739.
(3S,7S,7aR)-7-Hydroxy-3,6,6-trimethyltetrahydropyrrolo[1,2-c]thiazol-5(3H)-one (21).
Prepared according to the General procedure E from alcohol 13 (0.217 g, 0.65 mmol); product 21 was obtained as white needles (0.071 g, 0.35 mmol, 54% yield). M. p. 93–95 °C. [α]20D = −90.2 (c 0.41, DCM). 1H NMR (CDCl3, 400 MHz): δ 5.22 (q, J = 6.4 Hz, 1H), 4.41–4.36 (m, 1H), 4.02–4.00 (m, 1H), 3.27 (dd, J = 8.0, 10.8 Hz, 1H), 2.97 (dd, J = 6.4, 10.8 Hz, 1H), 2.32 (br s, 1H), 1.47 (d, J = 6.4 Hz, 3H), 1.22 (s, 3H), 1.14 (s, 3H) ppm. 13C NMR (150 MHz, CDCl3): δ 176.4, 75.3, 64.3, 54.8, 49.9, 29.9, 23.7, 23.4, 18.2 ppm. IR (KBr): 3410, 2921, 1664, 1418, 1341, 1265, 1209, 1103, 870 cm−1. HRMS (ESI-TOF): m/z [M + H]+ calcd for (C9H15NO2S)+ 202.0902; found 202.0904.
(2S,4aR,7aS)-7,7-Dimethyl-2-phenyltetrahydro-[1,3]oxathiino[5,6-b]pyrrol-6(4H)-one (22).
Prepared according to the General procedure E from alcohol 14 (0.257 g, 0.65 mmol); product 22 was obtained as a white solid (0.065 g, 0.25 mmol, 38% yield). M. p. 196–198 °C. [α]20D = −19.4 (c 0.41, DCM). 1H NMR (CDCl3, 400 MHz): δ 7.43 (dd, J = 1.6, 8.4 Hz, 2H), 7.30–7.37 (m, 3H), 5.80 (br s, 1H), 5.80 (br s, 1H), 4.04 (dd, J = 1.2, 3.6 Hz, 1H), 3.90 (q, J = 4.0 Hz, 1H), 3.42 (dd, J = 4.0, 14.4 Hz, 1H), 3.00 (dd, J = 4.4, 14.8 Hz, 1H), 1.62 (m, 2H), 1.25 (s, 3H), 1.22 (s, 3H) ppm. 13C NMR (100 MHz, CDCl3): δ 181.6, 139.4, 128.6, 125.9, 81.9, 46.5, 46.4, 31.0, 29.5, 22.2, 17.5 ppm. IR (KBr): 3430, 2921, 1699, 1660, 1447, 1306, 1238, 1096, 1087, 712 cm−1. HRMS (ESI-TOF): m/z [M + H]+ calcd for (C14H17NO2S)+ 264.1058; found 264.1039.
(4aR,7aS)-2,2,7,7-Tetramethyltetrahydro-[1,3]oxathiino[5,6-b]pyrrol-6(4H)-one (23).
Prepared according to the General procedure E from alcohol 15 (0.226 g, 0.65 mmol); product 23 was obtained as a white crystalline solid (0.098 g, 0.455 mmol, 70% yield). M. p. 137–139 °C, [α]20D = +49.5 (c 0.41, DCM). 1H NMR (400 MHz, CDCl3): δ 6.13 (s, 1H), 3.89–3.79 (m, 1H), 2.85 (dd, J = 13.3, 6.4 Hz, 1H), 2.55 (dd, J = 13.3, 8.8 Hz, 1H), 1.52 (s, 1H), 1.45 (s, 1H), 1.11 (s, 1H), 1.06 (s, 1H) ppm. 13C NMR (100 MHz, CDCl3): 180.85, 80.43, 75.75, 50.41, 43.04, 30.24, 26.54, 25.28, 23.19, 16.46 δ ppm. IR (KBr): 3405, 3313, 3240, 2981, 2935, 2886, 1709, 1692, 1640, 1383, 1327, 1292, 1052 cm−1. HRMS (ESI-TOF): m/z [M + H]+ calcd for (C10H18NO2S)+ 216.1058; found 216.1059.
Mixture of (2S,4aR,7aS)-2-ethyl-2,7,7-trimethyltetrahydro-[1,3]oxathiino[5,6-b]pyrrol-6(4H)-one and (2R,4aR,7aS)-2-ethyl-2,7,7-trimethyltetrahydro-[1,3]oxathiino[5,6-b]pyrrol-6(4H)-one (24).
Prepared according to the General procedure E from alcohol 16 (0.235 g, 0.65 mmol); a mixture of products 24 was obtained as a colorless oil (0.107 g, 0.467 mmol, 72% yield). De = 35%. 1H NMR (400 MHz, CDCl3) δ (major diastereomer) 6.37–6.31 (m, 1H), 3.97–3.95 (m, 1H), 3.88–3.86 (m, 1H), 2.86 (dd, J = 8.0, 16.0 Hz, 1H), 2.59 (dd, J = 8.0, 16.0 Hz, 1H), 1.87–1.77 (m, 1H), 1.72–1.67 (m, 1H), 1.45 (s, 3H), 1.17 (s, 3H), 1.11 (s, 3H), 0.97 (t, J = 8.0 Hz, 3H) ppm; (minor diastereomer) 6.37–6.31 (m, 1H), 3.91–3.87 (m, 2H), 2.88 (dd, J = 8.0, 16.0 Hz, 1H), 2.58 (dd, J = 8.0, 16.0 Hz, 1H), 2.03–1.93 (m, 1H), 1.72–1.67 (m, 1H), 1.51 (s, 3H), 1.15 (s, 3H), 1.13 (s, 3H), 0.91 (t, J = 8.0 Hz, 3H) ppm. 13C NMR (100 MHz, CDCl3): (major diastereomer) 181.9, 84.8, 76.5, 51.8, 44.3, 36.8, 27.8, 24.5, 17.7, 8.7 ppm; (minor diastereomer) 181.9, 85.1, 76.4, 51.9, 44.1, 31.4, 27.4, 24.5, 23.6, 17.6, 8.6 ppm. HRMS (ESI-TOF): m/z [M + H]+ calcd for (C11H19NO2)+ 230.1215; found 230.1219.
Mixture of (2S,4R)-tert-butyl 4-((S)-1,3-dihydroxy-2,2-dimethylpropyl)-2-methylthiazolidine-3-carboxylate and (2R,4R)-tert-butyl 4-((S)-1,3-dihydroxy-2,2-dimethylpropyl)-2-methylthiazolidine-3-carboxylate (13′).
Obtained as a side product from ketone 8 according to the General procedure D. White solid. Yield ca. 15%. M. p. 94–95 °C, [α]20D = (c, DCM). 1H NMR (400 MHz, CDCl3) δ 5.12 (q, J = 6.1 Hz, 1H), 4.64 (t, J = 7.1 Hz, 1H), 3.61–3.48 (m, 2H), 3.44 (d, J = 8.0 Hz, 1H), 3.18 (dd, J = 11.6, 6.3 Hz, 1H), 3.04 (br s, 1H), 2.70 (dd, J = 11.6, 1.7 Hz, 1H), 1.55 (d, J = 6.1 Hz, 3H), 1.46 (s, 9H), 1.01 (s, 3H), 0.96 (s, 3H) ppm. 13C NMR (100 MHz, CDCl3) δ 155.34, 81.70, 71.49, 61.57, 58.86, 39.11, 35.79, 28.43, 23.57, 20.06. IR (KBr): 3369, 2976, 2965, 2924, 2874, 1661, 1476, 1410, 1364, 1327, 1264, 1123, 1074, 1040 cm−1. HRMS (ESI-TOF): m/z [M + H]+ calcd for (C14H27NO4S)+ 328.1559; found 328.1555.
Mixture of (2S,4R)-tert-butyl 4-((S)-1,3-dihydroxy-2,2-dimethylpropyl)-2-phenylthiazolidine-3-carboxylate and (2R,4R)-tert-butyl 4-((S)-1,3-dihydroxy-2,2-dimethylpropyl)-2-phenylthiazolidine-3-carboxylate (14′).
Obtained as a side product from ketone 9 according to the General procedure D. Crystalline solid. Yield ca. 15%. M. p. 117–119 °C, [α]20D = +43.6 (c 0.62, DCM). 1H NMR (CDCl3, 400 MHz): δ 7.61–7.56 (m, 2H), 7.35–7.25 (m, 3H), 5.90 (br s, 1H), 4.67 (ddd, J = 8.4, 6.3, 1.8 Hz, 1H), 3.67–3.58 (m, 2H), 3.54 (d, J = 11.4 Hz, 1H), 3.31 (dd, J = 12.0, 6.3 Hz, 1H), 2.80 (dd, J = 12.0, 1.8 Hz, 1H), 1.18 (s, 9H), 1.06 (s, 3H), 1.00 (s, 3H) ppm. 13C NMR (100 MHz, CDCl3) δ 156.53, 140.70, 128.42, 128.07, 127.15, 82.06, 80.28, 71.76, 66.52, 62.89, 39.49, 35.91, 28.03, 23.56, 19.92 ppm. IR (KBr): 3458, 2976, 2932, 1667, 1477, 1456, 1387, 1367, 1311, 1259, 1165, 1117, 1076, 1046 cm−1. HRMS (ESI-TOF): m/z [M + H]+ calcd for (C19H29NO4S)+ 368.1895; found 368.1889.
(R)-tert-Butyl 4-((S)-1,3-dihydroxy-2,2-dimethylpropyl)-2,2-dimethylthiazolidine-3-carboxylate (15′).
Obtained as a side product from ketone 10 according to the General procedure D. White solid. Yield ca. 15%. M. p. 129 °C, 1H NMR (400 MHz, CDCl3) δ 4.76–4.64 (m, 1H), 3.63–3.52 (m, 2H), 3.45 (d, J = 8.0 Hz, 1H), 3.27 (dd, J = 11.9, 5.3 Hz, 1H), 2.46 (d, J = 11.9 Hz, 1H), 1.78 (s) and 1.78 (s) (6H), 1.49 (s, 9H), 1.04 (s, 3H), 0.96 (s, 3H) ppm. HRMS (ESI-TOF): m/z [M + H]+ calcd for (C15H29NO4S)+ 320.1895; found 320.1895. Spectroscopic data are consistent with literature.18
Mixture of epimers (S)-2,2-dimethyl-1-((2R,4R)-2-methylthiazolidin-4-yl)propane-1,3-diol and (S)-2,2-dimethyl-1-((2S,4R)-2-methylthiazolidin-4-yl)propane-1,3-diol (21′).
Prepared according to the General procedure E from alcohol 13′ (0.199 g, 0.65 mmol); a mixture of products 21′ was obtained as a crystalline solid (0.113 g, 0.55 mmol, 85% yield). De = 20%. M. p. 139–140 °C, [α]20D = +17.9 (c 0.55, DCM). 1H NMR (CDCl3, 400 MHz): δ 4.54 (q, J = 8.0 Hz, 1H), 3.72 (d, J = 12.0 Hz, 1H), 3.44 (s, 1H), 3.37–3.33 (m, 1H), 3.03–2.97 (m, 4H), 2.93–2.56 (m, 2H), 1.53 (d, J = 8.0 Hz, 3H), 1.00 (s, 3H), 0.89 (s, 3H) ppm (major epimer); 4.72 (q, J = 8.0 Hz, 1H), 3.67 (d, J = 12.0 Hz, 1H), 3.57–3.53 (m, 1H), 3.48 (s, 1H), 3.03–2.97 (m, 4H), 2.93–2.56 (m, 2H), 1.44 (d, J = 8.0 Hz, 3H), 1.00 (s, 3H), 0.90 (s, 3H) ppm (minor epimer). IR (KBr): 3401, 2926, 2854, 1475, 1307, 1219, 1135, 1093, 1048, 916 cm−1. HRMS (ESI-TOF): m/z [M + H]+ calcd for (C9H20NO2S)+ 206.1215; found 206.1218.
2-((2R,5R,6S)-5-Amino-2-phenyl-1,3-oxathian-6-yl)-2-methylpropan-1-ol (22′).
Prepared according to the General procedure E from alcohol 14′ (0.239 g, 0.65 mmol); product 22′ was obtained as a white crystalline solid (0.104 g, 0.39 mmol, 60% yield). M. p. 175–176 °C, [α]20D = −42.2 (c 0.51, DCM). 1H NMR (CDCl3, 400 MHz): δ 7.47–7.43 (m, 2H), 7.39–7.30 (m, 3H), 5.87 (s, 1H), 3.87 (d, J = 11.7 Hz, 1H), 3.51 (dd, J = 13.5, 1.9 Hz, 1H), 3.33 (s, 1H), 3.16 (ddd, J = 4.0, 1.9, 1.1 Hz, 1H), 2.94 (d, J = 11.9 Hz, 1H), 2.70 (dd, J = 13.5, 3.9 Hz, 1H), 1.05 (s, 3H), 0.88 (s, 3H) ppm. 13C NMR (100 MHz, CDCl3) δ 139.09, 128.50, 125.74, 90.76, 85.45, 64.99, 40.66, 40.49, 40.28, 26.15, 21.25 ppm. IR (KBr): 3435, 3345, 2959, 2911, 2864, 1504, 1475, 1370, 1309, 1243, 1075, 1038, 1024, 1002, 979, 730, 699 cm−1. HRMS (ESI-TOF): m/z [M + H]+ calcd for (C14H21NO2S)+ 268.1371; found 268.1363.
2-((5R,6S)-5-Amino-2,2-dimethyl-1,3-oxathian-6-yl)-2-methylpropan-1-ol (23′).
Prepared according to the General procedure E from alcohol 15′ (0.208 g, 0.65 mmol); product 23′ was obtained as a white crystalline solid (0.125 g, 0.57 mmol, 87% yield). M. p. 115–117 °C, [α]20D = +29.2 (c 0.59, DCM). 1H NMR (CDCl3, 400 MHz) δ 3.94 (s, 2H), 3.73 (d, J = 11.5 Hz, 1H), 3.45–3.31 (m, 2H), 3.09–2.96 (m, 1H), 2.85 (d, J = 11.6 Hz, 1H), 2.56 (dd, J = 13.7, 4.0 Hz, 1H), 1.62 (s, 3H), 1.53 (s, 3H), 1.32 (s, 1H), 1.01 (s, 3H), 0.81 (s, 3H) ppm. 13C NMR (CDCl3, 100 MHz) δ 80.92, 64.58, 40.19, 39.52, 36.83, 31.56, 25.98, 25.87, 21.37 ppm. IR (KBr): 3435, 3338, 3272, 2958, 2924, 2853, 1512, 1456, 1377, 1363, 1172, 1132, 1071, 1045, 993 cm−1. HRMS (ESI-TOF): m/z [M + H]+ calcd for (C10H22NO2S)+ 220.1371; found 220.1370.
Crystallography
The data were collected on an Oxford Diffraction four-circle single crystal diffractometer equipped with a CCD detector using graphite-monochromatized MoKα radiation (λ = 0.71073 Å). The raw data were treated with the CrysAlis Data Reduction Program (version 1.171.39.46). The intensities of the reflection were corrected for Lorentz and polarization effects. The crystal structures were solved by direct methods using SHELXS-2018/3 and refined by the full-matrix least-squares method using the SHELXL-2018/3 program.19,20 The crystal structure of compound 23′ was refined as a three component twin with the respective domain contributions of 0.56, 0.23 and 0.21. Non-hydrogen atoms were refined using anisotropic displacement parameters. H-atoms were placed by geometry and allowed to refine “riding on” the parent atom with Uiso = 1.2Ueq(N/C) for NH, CH and CH2 groups and Uiso = 1.5Ueq(C/O) for CH3 and OH groups. Crystal data and structure refinement details are presented in Table S1 (ESI†). Visualization of the structures was made with Mercury, Diamond 3.2k and CrystalExplorer programs.21–23 CCDC 2180921–2180926 and 2237944–2237947 contain the supplementary crystallographic data for this paper.†
Calculations
The conformational analysis of the studied systems was performed using the Conflex program,24–26 in which the MMFF94s force field was applied and the 1 kcal mol−1 energy window was used. In the next step, the MMFF94s structures were optimized using the DFT B3LYP functional27–29 combined with the def2-TZVP basis set.30 All stationary points (minima and transition structures) were characterized by calculating the harmonic vibrational frequencies. The transition structures (TS) were found using the quadratic synchronous transit-guided quasi-Newton (QST3) method.31 In some cases, intrinsic reaction coordinate (IRC) calculations32,33 were performed for both forward and reverse directions of the vibrational mode calculations to confirm that the found transition states connect the appropriate minima. However, in most cases, one imaginary frequency with a high value and appropriate vibrational mode was enough to state that the found TS structure is the proper one. Structure minima were confirmed by ascertaining that all harmonic frequencies were real.
Duplicate conformers were removed, and the relative abundances were calculated based on the ΔE and ΔG values referred to the value of the most stable conformer. Natural bond analysis (NBO)34 was performed to estimate the charge distribution. All calculations were performed using the Gaussian 16 package,35 and the structures were visualized using GaussView.36 The computational data were processed with the help of Tesliper software.37
Conclusions
In conclusion, an efficient transformation was established leading to novel chiral sulfur-bearing bicyclic lactams. Using L-cysteine as the sole chirality source, products with up to three stereogenic centers could be obtained with high stereoselectivity. We believe that the new derivatives will find application in asymmetric synthesis and show their potential as bioactive compounds.
Conflicts of interest
There are no conflicts to declare.
Acknowledgements
MD would like to thank ILT&SR PAS for financial support through statutory activity subsidy. The computational grant for JER from the Świerk Computing Centre (CIŚ) is gratefully acknowledged.
Notes and references
- M. D. Groaning and A. I. Meyers, Tetrahedron, 2000, 56, 9843 CrossRef CAS.
- R. Singh and R. Vince, Chem. Rev., 2012, 112, 4642 CrossRef CAS PubMed.
- J. F. Fisher and S. Mobashery, Chem. Rev., 2021, 121, 3412 CrossRef CAS.
- G. W. Stacy and P. L. Strong, J. Org. Chem., 1967, 32, 1487 CrossRef CAS.
- F. Fülöp, J. Mattinen and K. Pihlaja, Tetrahedron Lett., 1988, 29, 5427 CrossRef.
- F. Fülöp, J. Mattinen and K. Pihlaja, Tetrahedron, 1990, 46, 6545 CrossRef.
- J. J. Pesek and J. H. Frost, Tetrahedron, 1975, 31, 907 CrossRef CAS.
- A. M. Davis and M. I. Page, J. Chem. Soc., Chem. Commun., 1985, 1702 RSC.
- D. S. Kemp and D. I. Carey, J. Org. Chem., 1989, 54, 3640 CrossRef CAS.
- K. Kamińska, A. Mular, E. Olshvang, N. Metzler-Nolte, H. Kozłowski, E. Wojaczyńska and E. Gumienna-Kontecka, RSC Adv., 2022, 12, 25284 RSC.
- A. Ino, Y. Hasegawa and A. Murabayashi, Tetrahedron Lett., 1998, 39, 3509 CrossRef CAS.
- R. O. Duthaler and B. Wyss, Eur. J. Org. Chem., 2011, 4667 CrossRef CAS.
- M. R. Vishnumaya and V. K. Singh, J. Org. Chem., 2009, 74, 4289 CrossRef CAS.
- Z.-C. Song, G.-Y. Mab and H.-L. Zhu, RSC Adv., 2015, 5, 24824 RSC.
- R. H. Wiley and J. F. Jeffries, J. Am. Chem. Soc., 1949, 71, 1137 CrossRef CAS.
- A. Shimizu, K. Takeda, S. Mishima, K. Saito, S. Kim, T. Nokami and J. Yoshida, Bull. Chem. Soc. Jpn., 2016, 89, 61 CrossRef CAS.
- C. Doler, M. Friess, F. Lackner, H. Weber, R. S. Fischer and R. Breinbauer, Tetrahedron, 2020, 76, 131249 CrossRef CAS.
- A. Ino, Y. Hasegawa and A. Murabayashi, Tetrahedron, 1999, 55, 10283 CrossRef CAS.
- G. M. Sheldrick, Acta Crystallogr., Sect. A: Found. Adv., 2015, 71, 3 CrossRef PubMed.
- G. M. Sheldrick, Acta Crystallogr., Sect. C: Struct. Chem., 2015, 71, 3 Search PubMed.
-
K. Brandenburg and H. Putz, Crystal Impact GbR, Bonn, Germany, 2008 Search PubMed.
- C. F. MacRae, I. Sovago, S. J. Cottrell, P. T. A. Galek, P. McCabe, E. Pidcock, M. Platings, G. S. Shields, J. S. Stevens, M. Towler and P. A. Wood, J. Appl. Crystallogr., 2020, 53, 226 CrossRef CAS.
-
M. J. Turner, J. J. McKinnon, S. K. Wolff, D. J. Grimwood, P. R. Spackman, D. Jayatilaka and M. A. Spackman, CrystalExplorer17, University of Western Australia, 2017 Search PubMed.
-
CONFLEX 7, Conflex Corp., Japan, 2012 Search PubMed.
- H. Goto and E. Osawa, J. Am. Chem. Soc., 1989, 111, 8950 CrossRef CAS.
- H. Goto and E. Osawa, J. Chem. Soc., Perkin Trans. 2, 1993, 187 RSC.
- A. D. Becke, J. Chem. Phys., 1993, 98, 5648 CrossRef CAS.
- C. Lee, W. Yang and R. G. Parr, Phys. Rev. B: Condens. Matter Mater. Phys., 1988, 37, 785 CrossRef CAS.
- P. J. Stephens, F. J. Devlin, C. F. Chabalowski and M. J. Frisch, J. Phys. Chem., 1994, 98, 11623 CrossRef CAS.
- F. Weigend and R. Ahlrichs, Phys. Chem. Chem. Phys., 2005, 7, 3297 RSC.
- C. Peng and H. B. Schlegel, Isr. J. Chem., 1993, 33, 449 CrossRef CAS.
- K. Fukui, Acc. Chem. Res., 1981, 14, 363 CrossRef CAS.
- C. Gonzales and H. B. Schlegel, J. Chem. Phys., 1989, 90, 2154 CrossRef.
-
F. Weinhold and C. R. Landis, Discovering Chemistry with Natural Bond Orbitals, John Wiley & Sons, Hoboken, NJ, 2012 Search PubMed.
-
M. J. Frisch, et al., Gaussian 09 ver. D.01, Gaussian Inc., Wallingford, CT, 2013 Search PubMed.
-
R. D. Dennington, II, T. A. Keith and J. M. Millam, GaussView 6.0.16, Semichem Inc., 2000–2016 Search PubMed.
- M. M. Więcław, J. Open Source Softw., 2022, 7, 4164 CrossRef.
Footnote |
† Electronic supplementary information (ESI) available: Synthetic protocols, characterization of compounds, copies of NMR spectra, X-ray measurement details, and results of theoretical calculations. CCDC 2180921–2180926 and 2237944–2237947. For ESI and crystallographic data in CIF or other electronic format see DOI: https://doi.org/10.1039/d3ob01454a |
|
This journal is © The Royal Society of Chemistry 2023 |
Click here to see how this site uses Cookies. View our privacy policy here.