DOI:
10.1039/D2QI02653H
(Research Article)
Inorg. Chem. Front., 2023,
10, 3195-3201
Uranyl-silicotungstate-containing hybrid building units {α-SiW9} and {γ-SiW10} with excellent catalytic activities in the three-component synthesis of dihydropyrimidin-2(1H)-ones†
Received
13th December 2022
, Accepted 28th January 2023
First published on 1st February 2023
Abstract
A novel uranyl-containing polytungstate, {K4.2Na18.9H0.9(H2O)20[K4(UO2)3(H2O)6(α-SiW9O34)(γ-SiW10O36)3]}·ca.16H2O (U3), was synthesized using the Keggin-type precursor [γ-SiW10O36]8− ({γ-SiW10}) and UO2(OAc)2 and was further characterized by single-crystal X-ray diffraction and various techniques. Single crystal X-ray diffraction analysis showed a quadrahedron-like structure of U3 with interesting tarot-like (top view) and crown-like (side view) views, among which one central {K4(UO2)3(H2O)3} cluster was surrounded by one {α-SiW9} and three {γ-SiW10} building units. In addition, a more significant aspect is that U3 shows good catalytic activity in the dehydration condensation of aldehydes, acetoacetates, and urea to green synthesize dihydropyrimidin-2(1H)-ones. The advantages of this transformation include solvent-free conditions, water as the sole by-product, available starting materials, good compatibility, and operational simplicity.
Introduction
Self-assembly of metal–oxygen clusters with definite structures makes it possible to design and synthesize metal oxide materials from micro- to macro-scale. Polyoxometalates (POMs) are a kind of molecular metal–oxygen-cluster with various structures and important properties involving applications in catalysis, photoelectric materials, medicine, etc.1–9 In general, in situ and precursor strategies are the mainstream methods used in the synthesis of 3d/4f-metal-containing POMs. Thousands of 3d/4f-metal-containing POMs have been reported and show fascinating properties. The in situ method offers more driving powers to intrinsically build new POMs by adjusting the reaction steps and conditions, while the usage of lacunary POMs precursors makes it easier to predict the structures of the obtained POMs. However, the development of actinide-containing POMs may be limited by synthetic methods, and the study still lags far behind 3d/4f-metal-containing POMs comparing the research contents and depth, which includes structure-based research and applications.10 Actinides that have unique 5f electrons and bigger atomic radii may show more abundant oxidation states and are good members to synthesize POMs.11–16 For these stable actinides, thorium and uranium are most accessible to study, and U-containing heteropolytungstates (U-POWs) are the most explored. But it is disappointing to note that less than ten cases were in situ synthesized and dozens of U-POWs were all synthesized using lacunary POM precursors among these U-POWs.17–22 Thus, the knowledge of the structural diversities of actinide-containing POMs still needs exploitation.
It should be noted that the synthesis of POMs that contain more than one lacunary POM building unit is quite challenging due to the complexity of the self-assembly. Most of the U-POWs are based on one type of building unit and there are only four cases that contain more than one type of lacunary POM building unit, named hybrid structures. The first structure reported by Pope in 2001 was [(UO2)3(H2O)5As3W29O104]19−, which consisted of a trivacant Keggin-{AsW9} and a Dawson-like {(AsW9)2(W2)} building units.23 The second one, [(UO2)3(H2O)4As3W26O94]17−, was based on Keggin-{AsW9} and a rare Dawson-like {(AsW8)2W} building units.24 The third showed an iso-heteropolytungstate hybrid structure, [(H3SbIIIW17O59)UIV(HW5O18)]11−, which was in situ constructed from simple materials with monovacant Lindqvist-{W5} and monovacant Dawson-{SbW17} building units.18 Recently, Zhang's and our group have reported the fourth hybrid U-POW, K1.5Na13.25[(UO2)3(SeO3)3Na5(H2O)6(SeW6O21)(SeW9O33)3]·26H2O, which was in situ synthesized with one Anderson-{SeW6} and three Keggin-{SeW9} building units.22
The usage of lacunary POM precursors indeed makes it efficient to synthesize new POMs, but the relatively strong stabilities of lacunary POM precursors also reduce the structural uncertainties of the obtained new POMs.25–27 Thus, these changeful and relatively unstable lacunary POM precursors with low stabilities are preferred to build new U-POWs.28–33 For example, Kortz reported a horseshoe-like U-POW, e.g., K6Li19[Li(H2O)K4(H2O)3{(UO2)4(O2)4(H2O)2}2(PO3OH)2P6W36O136]·74H2O, which was synthesized using the precursor [H6P4W24O94]18− ({P4W24}).34 {P4W24} transformed into a rare {P2W12}-trimeric {P6W36} building unit in an LiAc buffer in the synthetic procedure. Another representative case is the third hybrid U-POW, (NH4)17[(UO2)3(H2O)4As3W26O94]·16H2O, as mentioned earlier, which was synthesized from {AsW9} (Na9[AsW9O33]·27H2O).24 A part of {AsW9} lost another {WO6} and assembled into a rare Dawson-like {(AsW8)2W} building unit. {γ-SiW10} is one of the most changeful lacunary POM precursors and could undergo complicated transformations into monovacant {SiW11}, trivacant {SiW9}, and other species in solution.35 Thus, new U-POWs may be obtained using {γ-SiW10} under specific conditions.
Regarding the aforementioned cases, we report a new U-POW with hybrid polyanions: {K4.2Na18.9H0.9(H2O)20[K4(UO2)3(H2O)6(α-SiW9O34)(γ-SiW10O36)3]}·ca.16H2O (U3), which is synthesized by a one-pot method using K8[γ-SiW10O36]·12H2O and UO2(OAc)2. A part of {γ-SiW10} transformed into trivacant {α-SiW9} in the aqueous solution and joined in the assembly of the polyanion. The polyanion of U3 shows a quadrahedron-like configuration based on a {K4(UO2)3(H2O)6} cluster chromophore and two kinds of lacunary silicotungstate building units, e.g., trivacant {α-SiW9} and bivacant {γ-SiW10}. This work provides the first case of U-POW that is constructed from two kinds of Keggin-type lacunary building units. Besides, U3 shows good catalytic activity in the synthesis of dihydropyrimidin-2(1H)-ones (DHPMs) via the dehydration condensation of aldehydes, acetoacetates, and urea. The solvent-free conditions, water as the sole by-product, and simple starting materials make this method suitable for the green synthesis of DHPMs.
Experimental
Materials and methods
The precursor K8[γ-SiW10O36]·12H2O was synthesized according to the method reported by Hervéa.36 Other reagents were purchased from the reagent manufacturers and used without further purification. CAUTION! Although the uranyl salt used is depleted, it is still radioactive and toxic. Appropriate protective measures are still necessary for handling all radioactive materials.
Synthesis of U3
In a NaCl aqueous solution (0.25 M, 20 mL), UO2(OAc)2·2H2O (0.1 mmol, 0.0424 g) and K8[γ-SiW10O36]·12H2O (0.4 mmol, 1.1884 g) were dissolved successively. The pH value of the mixture was then adjusted to 5.9 using 1 M HCl. The cloudy solution was heated and stirred at 85 °C for 30 min. During the heating procedure, this cloudy solution would transform from cloudy to clear and then cloudy again. After cooling down to room temperature, NaCl (4 g) was added to the solution and stirred for 5 min. The resulting solution was filtered and the filtrate was left to evaporate at room temperature. A cold room temperature (below 10 °C) is necessary for the formation of U3. Unknown and cubic crystals will appear if the room temperature is higher.37 Yellow-green crystals of U3 with rod-like shapes were collected after two weeks (yield: 11% based on UO2(OAc)2·2H2O). Elemental analysis (ICP-OES, %) for H84.9K8.2Na18.9O190Si4U3W39, found: Na, 3.37; K, 2.49; W, 55.68. IR (cm−1): 3388 (s), 1624 (m), 1005 (w), 945 (m), 847 (vs), 787 (s), 722 (vs), 689 (vs), 558 (m).
Typical procedure of dehydration condensation reaction catalyzed by U3
Benzaldehyde (1 mmol), ethyl acetoacetate (1.5 mmol), urea (1 mmol), and U3 (0.2 mol%) were added to a 4 mL reaction vial. Then the reaction was carried out at 100 °C for 3 h. After cooling down to room temperature, the crude product was dissolved in hot EtOH (10 mL). After simple filtration, the filtrate was cooled in an ice bath to promote the recrystallization of the desired product. The purified products were all characterized by NMR and the corresponding data are provided in the ESI.†
Results and discussion
Analysis of U3
The single-crystal analysis demonstrates that U3 crystallizes in the P
space group. The polyanion in U3 consists of three parts, including one {K4(UO2)3(H2O)6} chromophore at the centre of the polyanion, one {α-SiW9} and three {γ-SiW10} moieties (Fig. 1A). In the triangular {K4(UO2)3(H2O)6} chromophore, all the three U(VI) atoms (U1/U2/U3) show a similar 7-coordinated distorted pentagonal bipyramidal geometry at the peak of the triangle. The polar oxygen atoms from the U(VI) pentagonal bipyramid could be divided into two groups based on the positions relative to U(VI) atoms (Fig. S1†). The bond lengths of three inner polar oxygen atoms and the corresponding U(VI) atoms (O1 for U1, O3 for U2, and O5 for U3) are in the range of 1.795(18) to 1.811(17) Å, which are slightly longer than the typical O
U
O bonds (1.75–1.79 Å), while the outside polar oxygen atoms (O2 for U1, O4 for U2 and O6 for U3) show normal U
O bond lengths (1.736(16) Å for O2–U1, 1.794(18) Å for O4–U2 and 1.781(17) for O6–U3). The bond angles for O
U
O bonds are also distorted compared with a standard uranyl ion (178.048(13)° for U1, 179.418(10)° for U2 and 178.997(11)° for U3). For the five equatorial oxygen atoms, four oxygen atoms coordinated with K(I) ions are offered by {α-SiW9} and {γ-SiW10}, while the other oxygen atoms (O7/O8/O9) are coordinated with water molecules. The five equatorial oxygen atoms (O7/O10/O11/O16/O17) for U1 are almost coplanar and the deviation is less than 0.05 Å. But O8 is 0.0848 Å away from the plane constructed by O12/O13/O18 for U2. For U3, the four equatorial oxygen atoms (O14/O15/O20/O21) offered by polyanions are almost coplanar and O9 is 0.1433 Å away from the plane of O14/O15/O20.
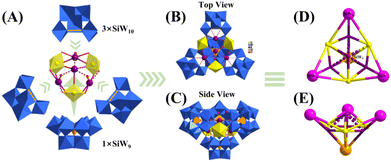 |
| Fig. 1 (A) Schematic depiction of the units in the polyanion of U3; (B) top view and (C) side view of the polyanion in U3; and (D) and (E) the simplified schematic depiction of the polyanion in U3. | |
In the typical coordination bond lengths of K–O bonds (2.9 Å), K1 is coordinated by four equatorial oxygen atoms (O10/O12/O17/O18), one coordinated water molecule (O151), and two other oxygen atoms (bridging oxygen atoms offered by {γ-SiW10}) (Fig. S1†). K2 is coordinated by three equatorial oxygen atoms (O14/O19/O20), one coordinated water molecule (O152), and one oxygen atom offered by {γ-SiW10}. Similarly, K3 is also coordinated by three equatorial oxygen atoms (O11/O16/O21), one coordinated water molecule (O153) and the other two oxygen atoms offered by {γ-SiW10}. Thus, a triangular arrangement is constructed by the connection of three U(VI) pentagonal bipyramids and three K(I) ions. K1 to K3 have weak coordination interactions with adjacent oxygen with distances from 2.9161(3) to 3.2179(3) Å. K4 is located at the position above the centre of this triangle and is coordinated by two inner polar oxygen atoms (O1/O5) from U1 and U3 with bond lengths of 2.8974(2) and 2.8993(2) Å, respectively. The distance from K4 to the plane of U1/U2/U3 is 2.3507 Å. Besides, K4 also has weak coordination interactions with adjacent oxygen atoms and two halves of water molecules (O154/O155) in the range of 2.9188(3)–3.2768(3) Å. In short, a triangular {K4(UO2)3(H2O)6} chromophore is constructed dexterously by the connection of U(VI) and K(I) ions and oxygen atoms offered by {α-SiW9} and {γ-SiW10}. Three U(VI) ions are at the peaks of the triangle and K1 to K3 are located at the centre of the edges.
In addition, one {α-SiW9} offers all the six peak oxygen atoms (O16–O21) to coordinate with three U(VI) and K(I) ions from the direction perpendicular to the plane of the triangle at the opposite side from K4. All the three {γ-SiW10} building units that act as inorganic multidentate ligands only offer two terminal oxygen atoms and one or two bridging oxygen atoms to coordinate with U(VI) and K(I) ions from directions perpendicular to the edges of the triangle, respectively. Thus, the three {γ-SiW10} units are all unsaturated. The plane constructed by Si2 to Si4 from three {γ-SiW10} units is not parallel to the plane formed by U1 to U3; the dihedral angle is 2.768°, while the distance between K4 and plane Si2 to Si4 is only 0.0930 Å. As a result, a hybrid quadrahedron-like polyanion in U3 is constructed dexterously from a one {α-SiW9}, three {γ-SiW10} and one {K4(UO2)3(H2O)6} cluster with a formula of [K4(UO2)3(H2O)6(α-SiW9O34)(γ-SiW10O36)3]24− (Fig. 1B–E and 2A). Besides, adjacent [K4(UO2)3(H2O)6(α-SiW9O34)(γ-SiW10O36)3]24− ions are alternately connected by K(I) and Na(I) ions and coordinating water molecules to form a one-dimensional chain in the typical coordination bond lengths of K–O bonds (2.9 Å) and Na–O bonds (2.6 Å) (Fig. S2†).
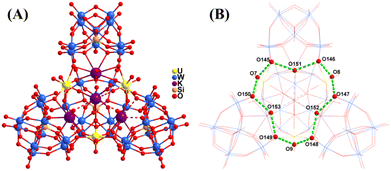 |
| Fig. 2 (A) Ball-and-stick view of the polyanion in U3 and (B) schematic depiction of the hydrogen bonds in U3. | |
It is worth noting that there are strong hydrogen bonds between the six coordinated water molecules (O7/O8/O9/O151/O152/O153) and six uncoordinated peak oxygen atoms (O145–O150) from three {γ-SiW10} (Fig. 2B). The distances between the donors and the acceptors are in the range of 2.67(3)–3.17(3) Å (Table S4†). Twelve oxygen atoms are connected in a zigzag manner by hydrogen bonds to form a heart-like arrangement. These hydrogen bonds help stabilize the unsaturated coordination structure.
The formula of U3 was determined by various characterization methods. The ICP-OES results reveal that the mass fractions of K, Na, and W in U3 were 2.49%, 3.37%, and 55.68%, respectively. Thus, the number of K
:
Na
:
W should be 8.2
:
18.9
:
39 and there was 0.9 hydrogen ion for charge balance. The TGA curve of U3 gives a 5.86% weight loss from room temperature to 300 °C, which corresponds to about 42 water molecules (Fig. S3†). The number of lattice water molecules removed by the SQUEEZE procedure is 16. Thus, the formula of U3 should be {K4.2Na18.9H0.9(H2O)20[K4(UO2)3(H2O)6(α-SiW9O34)(γ-SiW10O36)3]}·ca.16H2O. The elementary composition of U3 was also confirmed by energy dispersive spectroscopy (EDS) mapping results (Fig. S4†). Electrospray ionization mass spectrometry (ESI-MS) results demonstrated the chemical behaviours of U3 in water (Fig. S5 and Table S6†). The unsaturated coordination structure of U3 partly decomposed in electrospray ionization, but the polyanion of U3 could also maintain a degree of stability. U3 could be used as a catalyst under mild conditions. Besides, Fourier transform infrared, Raman, and solid-state luminescence spectra of U3 were also recorded to assess the properties of U3 (Fig. S7–S9†).
Evaluation of the catalytic activity of U3
The evaluation of the catalytic activity of U3 was performed in the synthesis of DHPMs through the dehydration condensation of aldehydes, acetoacetates, and urea.37–40 DHPMs play an extremely important role in organic synthesis and pharmaceutical chemistry, and have received great attention from organic chemists.41–45 Certain derivatives have good effects in anti-tumour, anti-inflammatory, and anti-bacterial activities, etc., and especially, some functional DHPMs are considered to have great development potential in the clinical treatment of calcium channel block, adrenergic antagonism, neuropeptide Y antagonism, and so on.46–49 This research would provide a promising catalytic system for the synthesis of DHPMs in good yields through a green method.
The reaction was carried out with benzaldehyde (1a), ethyl acetoacetate (2a), and urea (3a) as the model substrates, U3 as the catalyst, and various reaction conditions were optimized including the solvent, reaction temperature and time, the dosage of the catalyst, and the proportion of substrates (Fig. 3A). From Fig. 3B, there is no doubt that the catalytic activity of U3 is better under solvent-free conditions than in other solvents. Besides, from Fig. 3C–F, it is obvious that the product 4a yield increases with an increase of temperature, time, the dosage of the catalyst, and the proportion of the substrates. By screening, the optimum reaction conditions of the catalytic system were obtained as follows: 0.2 mol% U3, solvent-free, 100 °C, 3 h, and 1a
:
2a
:
3a = 1
:
1.5
:
1.
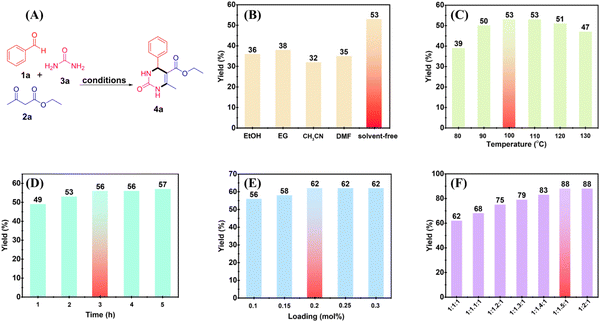 |
| Fig. 3 (A) Model reaction: benzaldehyde (1a, 1 mmol), ethyl acetoacetate (2a, 1 mmol), urea (3a, 1 mmol), U3 (0.1 mol%), solvent (1 mL), 100 °C, and 2 h; (B) screening of the reaction solvent (EG: ethylene glycol and DMF: N, N-dimethylformamide); (C and D) optimizing reaction temperature and time; (E) exploring the dosage of the catalyst; and (F) investigating the proportion of substrates (1a : 2a : 3a). | |
Having established the optimal reaction conditions, the scope of this transformation was further investigated (Table 1). A range of benzaldehyde derivatives (1) having both electron-donating groups (p-Me, m-Me, o-Me, p-iPr, p-OMe, and p-OEt) and electron-withdrawing groups (p-F, p-Cl, p-Br, and p-N(Me)2) were tested with ethyl acetoacetate (2a) and urea (3a). Generally, all the reactions proceeded smoothly with good yields of the corresponding products (4b–4j, and 4m). In addition, it was found that 2-naphthaldehyde and 5-methyl furfural can also react with ethyl acetoacetate (2a) and urea (3a) to generate the desired products 4k and 4l in moderate yields. Subsequently, we studied the reaction of benzaldehyde (1a), methyl acetoacetate (2b), and urea (3a), giving the product 4n in a yield of 86%. More interestingly, our protocol is applicable to the reaction of benzaldehyde (1a), ethyl acetoacetate (2a), and thiourea (3b), delivering the corresponding product 4o in a yield of 87%.
Table 1 Synthesis of DHPMs catalyzed by U3
a
Reaction conditions: benzaldehydes (1, 1.0 mmol), ethyl acetoacetates (2, 1.5 mmol), urea (3, 1.0 mmol), U3 (0.2 mol%), solvent-free conditions, 100 °C, and 3 h.
|
|
Conclusions
In summary, we have synthesized and fully characterized a new hybrid U-POW based on two kinds of Keggin-type building units. A part of {γ-SiW10} transformed into trivacant {α-SiW9} in the aqueous solution and joined in the assembly to access the polyanion. U3 represents a rare hybrid U-POW that is built based on one {α-SiW9} and three {γ-SiW10} building units. Besides, U3 was demonstrated to be a good catalyst for the synthesis of dihydropyrimidin-2(1H)-ones via the condensation of aldehydes, acetoacetates, and urea. Importantly, the solvent-free conditions, water as the sole by-product, and available starting materials mean that this protocol provides a green and efficient multicomponent reaction for the synthesis of DHPMs. This work provides another case of rare hybrid U-POWs and the application of U-POWs in catalytic synthesis chemistry and may benefit the development of related research.
Conflicts of interest
There are no conflicts to declare.
Acknowledgements
This work was supported by the National Natural Science Foundation of China (22001034), the Jiangxi Provincial Natural Science Foundation (20212BAB213001), and the Natural Science Foundation of Guangxi (2022GXNSFGA035003).
References
- J. C. Liu, J. W. Zhao, C. Streb and Y. F. Song, Recent advances on high-nuclear polyoxometalate clusters, Coord. Chem. Rev., 2022, 471, 214734 CrossRef CAS.
- D. J. Zang and H. Q. Wang, Polyoxometalate-based nanostructures for electrocatalytic and photocatalytic CO2 reduction, Polyoxometalates, 2022, 1, 9140006 CrossRef.
- L. B. Ni, G. P. Yang, Y. Liu, Z. Wu, Z. Y. Ma, C. Shen, Z. X. Lv, Q. Wang, X. X. Gong, J. Xie, G. W. Diao and Y. G. Wei, Self-assembled polyoxometalate nanodots as bidirectional cluster catalysts for polysulfide/sulfide redox conversion in lithium–sulfur batteries, ACS Nano, 2021, 15, 12222–12236 CrossRef CAS PubMed.
- Y. Q. Gu, Q. Li, D. J. Zang, Y. C. Huang, H. Yu and Y. G. Wei, Light–induced efficient hydroxylation of benzene to phenol by quinolinium and polyoxovanadate–based supramolecular catalysts, Angew. Chem., Int. Ed., 2021, 60, 13310–13316 CrossRef CAS PubMed.
- H. H. Zeng, Y. J. Qi, Z. Y. Zhang, C. T. Liu, W. J. Peng and Y. J. Zhang, Nanomaterials toward the treatment of Alzheimer's disease: Recent advances and future trends, Chin. Chem. Lett., 2021, 32, 1857–1868 CrossRef CAS.
- H. B. He, G. Wang, S. C. Chai, X. X. Li, L. Zhai, L. X. Wu and H. L. Li, Self-assembled lamellar nanochannels in polyoxometalate-polymer nanocomposites for proton conduction, Chin. Chem. Lett., 2021, 32, 2013–2016 CrossRef CAS.
- H. L. Li, M. X. Zhang, C. Lian, Z. L. Lang, H. J. Lv and G. Y. Yang, Ring-shaped polyoxometalate built by {Mn4PW9} and PO4 units for efficient visible-light-driven hydrogen evolution, CCS Chem., 2020, 2, 2095–2103 Search PubMed.
- Q. B. Shen, C. J. Gómez-García, W. L. Sun, X. Y. Lai, H. J. Pang and H. Y. Ma, Improving the photocatalytic H2 evolution activity of Keggin polyoxometalates anchoring copper-azole complexes, Green Chem., 2021, 23, 3104–3114 RSC.
- X. Guo, X. Wan, Q. T. Liu, Y. C. Li, W. W. Li and J. L. Shui, Phosphated IrMo bimetallic cluster for efficient hydrogen evolution reaction, eScience, 2022, 2, 304–310 CrossRef.
- G. P. Yang, K. Li and C. W. Hu, Recent advances in uranium-containing polyoxometalates, Inorg. Chem. Front., 2022, 9, 5408–5433 RSC.
- T. Auvray and E. M. Matson, Polyoxometalate-based complexes as ligands for the study of actinide chemistry, Dalton Trans., 2020, 49, 13917–13927 RSC.
- M. Dufaye, S. Duval and T. Loiseau, Trends and new directions in the crystal chemistry of actinide oxo-clusters incorporated in polyoxometalates, CrystEngComm, 2020, 22, 3549–3562 RSC.
- D. X. Gui, W. C. Duan, J. Shu, F. W. Zhai, N. Wang, X. X. Wang, J. Xie, H. Li, L. H. Chen, J. Diwu, Z. F. Chai and S. A. Wang, Persistent superprotonic conductivity in the order of 10–1 S cm−1 achieved through thermally induced structural transformation of a uranyl coordination polymer, CCS Chem., 2019, 1, 197–206 CAS.
- J. Xie, Y. X. Wang, W. Liu, X. M. Yin, L. H. Chen, Y. M. Zou, J. Diwu, Z. F. Chai, T. E. Albrecht-Schmitt, G. K. Liu and S. A. Wang, Highly sensitive detection of ionizing radiations by a photoluminescent uranyl organic framework, Angew. Chem., Int. Ed., 2017, 56, 7500–7504 CrossRef CAS PubMed.
- L. W. Cheng, C. Y. Liang, W. Liu, Y. X. Wang, B. Chen, H. L. Zhang, Y. L. Wang, Z. F. Chai and S. A. Wang, Three-dimensional polycatenation of a uranium-based metal–organic cage: Structural complexity and radiation detection, J. Am. Chem. Soc., 2020, 142, 16218–16222 CrossRef CAS PubMed.
- Y. X. Wang, X. M. Yin, W. Liu, J. Xie, J. F. Chen, M. A. Silver, D. P. Sheng, L. H. Chen, J. Diwu, N. Liu, Z. F. Chai, T. E. Albrecht-Schmitt and S. A. Wang, Emergence of uranium as a distinct metal center for building intrinsic X–ray scintillators, Angew. Chem., Int. Ed., 2018, 57, 7883–7887 CrossRef CAS PubMed.
- A. J. Gaunt, I. May, R. Copping, A. I. Bhatt, D. Collison, O. D. Fox, K. T. Holman and M. T. Pope, A new structural family of heteropolytungstate lacunary complexes with the uranyl, UO22+, cation, Dalton Trans., 2003, 3009–3014 RSC.
- Y. Jeannin, Synthesis and crystallographic study of a new composite of assymetric coordination of uranium(IV) linked to two ligands of the polytungstate type [((H3SbIIIW17O59))UIV(HW5O18)]11−, C. R. Chim., 2005, 8, 999–1004 CrossRef CAS.
- R. Khoshnavazi, H. Eshtiagh-Hossieni, M. H. Alizadeh and M. T. Pope, Syntheses and structures determination of new polytungstoarsenates [Na2As2W18U2O72]12− and [MAs2W18U2O72]13− (M = NH4+ and K+), Polyhedron, 2006, 25, 1921–1926 CrossRef CAS.
- H. Y. Wang, X. Y. Zheng, L. S. Long, X. J. Kong and L. S. Zheng, Sandwich-type uranyl phosphate–polyoxometalate cluster exhibiting strong luminescence, Inorg. Chem., 2021, 60, 6790–6795 CrossRef CAS PubMed.
- M. Y. Cheng, Y. F. Liu, W. X. Du, J. W. Shi, J. H. Li, H. Y. Wang, K. Li, G. P. Yang and D. D. Zhang, Two Dawson-type U(VI)-containing selenotungstates with sandwich structure and its high-efficiency catalysis for pyrazoles, Chin. Chem. Lett., 2022, 33, 3899–3902 CrossRef CAS.
- M. Y. Cheng, H. Y. Wang, Y. F. Liu, J. W. Shi, M. Q. Zhou, W. X. Du, D. D. Zhang and G. P. Yang, Bouquet-like uranium-containing selenotungstate consisting of two different Keggin-/Anderson-type units with excellent photoluminescence quantum yield, Chin. Chem. Lett., 2023, 34, 107209 CrossRef CAS.
- K.-C. Kim and M. T. Pope, New plenary and lacunary polyoxotungstate structures assembled from nonatungstoarsenate(III) anions and uranyl cations, J. Chem. Soc., Dalton Trans., 2001, 986–990 RSC.
- K.-C. Kim, A. Gaunt and M. T. Pope, New heteropolytungstates incorporating dioxouranium(VI). derivatives of α-[SiW9O34]10−, α-[AsW9O33]9−, γ-[SiW10O36]8−, and [As4W40O140]28−, J. Cluster Sci., 2002, 13, 423–436 CrossRef CAS.
- Y. Koizumi, K. Yonesato, K. Yamaguchi and K. Suzuki, Ligand-protecting strategy for the controlled construction of multinuclear copper cores within a ring-shaped polyoxometalate, Inorg. Chem., 2022, 61, 9841–9848 CrossRef CAS PubMed.
- H. F. Li, W. J. Chen, Y. J. Zhao, Y. Zou, X. Zhao, J. P. Song, P. T. Ma, J. Y. Niu and J. P. Wang, Regulating the catalytic activity of multi-Ru-bridged polyoxometalates based on differential active site environments with six-coordinate geometry and five-coordinate geometry transitions, Nanoscale, 2021, 13, 8077–8086 RSC.
- J. Goura, A. Sundar, B. S. Bassil, G. Ćirić-Marjanović, D. Bajuk-Bogdanović and U. Kortz, Peroxouranyl-containing W48 wheel: synthesis, structure, and detailed infrared and Raman spectroscopy study, Inorg. Chem., 2020, 59, 16789–16794 CrossRef CAS PubMed.
- J. H. Liu, R. T. Zhang, J. Zhang, D. Zhao, X. X. Li, Y. Q. Sun and S. T. Zheng, A series of 3D porous lanthanide-substituted polyoxometalate frameworks based on rare hexadecahedral {Ln6W8O28} heterometallic cage-shaped clusters, Inorg. Chem., 2019, 58, 14734–14740 CrossRef CAS PubMed.
- J. C. Jiao, X. M. Yan, S. Z. Xing, T. Zhang and Q. X. Han, Design of a polyoxometalate-based metal–organic framework for photocatalytic C(sp3)–H oxidation of toluene, Inorg. Chem., 2022, 61, 2421–2427 CrossRef CAS PubMed.
- L. B. Ni, H. J. Xu, H. F. Li, H. X. Zhao and G. W. Diao, 3D-Architecture via self-assembly of Krebs-type polyoxometalate {[Na10(H2O)26][Sb2W20Zn2O20(H2O)6]}, Polyhedron, 2018, 155, 59–65 CrossRef CAS.
- P. Y. Zhang, Y. L. Wang, L. Y. Yao and G. Y. Yang, Hepta-Zr-incorporated polyoxometalate assembly, Inorg. Chem., 2022, 61, 10410–10416 CrossRef CAS PubMed.
- H. L. Li, C. Lian and G. Y. Yang, {Ti6}/{Ti10} Wheel cluster substituted silicotungstate aggregates, Inorg. Chem., 2021, 60, 16852–16859 CrossRef CAS PubMed.
- H. L. Li, C. Lian and G. Y. Yang, A ring-shaped 12-Ti-substituted poly(polyoxometalate): synthesis, structure, and catalytic properties, Sci. China: Chem., 2022, 65, 892–897 CrossRef CAS.
- S. S. Mal, M. H. Dickman and U. Kortz, Actinide polyoxometalates: incorporation of uranyl–peroxo in U–shaped 36-tungsto-8-phosphate, Chem. – Eur. J., 2008, 14, 9851–9855 CrossRef CAS PubMed.
- J. Cao, C. Liu and Q. D. Jia, Complex solution chemistry behind the simple “one–pot” synthesis of vanadium–substituted polyoxometalates unraveled by electrospray ionization mass spectrometry, Rapid Commun. Mass Spectrom., 2016, 30, 14–19 CrossRef CAS PubMed.
- A. Téazéa, G. Hervéa, R. G. Finke and D. K. Lyon, α–, β–, and γ–Dodecatungstosilicic acids: isomers and related lacunary compounds, Inorg. Synth., 1990, 27, 85–96 Search PubMed.
- K. Li, X. L. Lin, K. Zeng, X. F. Gao, W. Cen, Y. F. Liu and G. P. Yang, Effect of Na(I)-H2O clusters on self-assembly of sandwich-type U(VI)-containing silicotungstates and the efficient catalytic activity for the synthesis of substituted phenylsulfonyl-1H-pyrazoles, Tungsten, 2022, 4, 149–157 CrossRef.
- G. P. Yang, X. L. Zhang, Y. F. Liu, D. D. Zhang, K. Li and C. W. Hu, Self-assembly of Keggin-type U(VI)-containing tungstophosphates with a sandwich structure: an efficient catalyst for the synthesis of sulfonyl pyrazoles, Inorg. Chem. Front., 2021, 8, 4650–4656 RSC.
- X. Q. Huang, Y. N. Cui, J. H. Zhou, Y. L. Zhang, G. D. Shen, Q. X. Yao, J. K. Li, Z. C. Xue and G. P. Yang, Self-assembly of three Ag-polyoxovanadates frameworks for their efficient construction of CN bond and detoxification of simulant sulfur mustard, Chin. Chem. Lett., 2022, 33, 2605–2610 CrossRef CAS.
- J. Zhou, T. Yu, K. Li, K. Zeng, G. P. Yang and C. W. Hu, Two U(VI)-containing silicotungstates with sandwich structures: lewis acid–base synergistic catalyzed synthesis of benzodiazepines and pyrazoles, Inorg. Chem., 2022, 61, 3050–3057 CrossRef CAS PubMed.
- M. Aslam and S. Verma, Biological activity of newly synthesized substituted dihydropyrimidinone and thione, Int. J. ChemTech Res., 2012, 4, 109–111 CAS.
- L. Saher, M. Makhloufi-Chebli, L. Dermeche, B. Boutemeur-Khedis, C. Rabia, A. M. S. Silva and M. Hamdi, Keggin and Dawson-type polyoxometalates as efficient catalysts for the synthesis of 3,4-dihydropyrimidinones: experimental and theoretical studies, Tetrahedron Lett., 2016, 57, 1492–1496 CrossRef CAS.
- S. Rezayati, F. Kalantari, A. Ramazani, S. Sajjadifar, H. Aghahosseini and A. Rezaei, Magnetic silica-coated picolylamine copper complex [Fe3O4@SiO2@GP/picolylamine-Cu(II)]-catalyzed Biginelli annulation reaction, Inorg. Chem., 2021, 61, 992–1010 CrossRef PubMed.
- W. G. Fan, Y. Queneau and F. Popowycz, HMF in multicomponent reactions: utilization of 5-hydroxymethylfurfural (HMF)
in the Biginelli reaction, Green Chem., 2018, 20, 485–492 RSC.
- P. Noppawan, S. Sangon, N. Supanchaiyamat and A. J. Hunt, Vegetable oil as a highly effective 100% bio-based alternative solvent for the one-pot multicomponent Biginelli reaction, Green Chem., 2021, 23, 5766–5774 RSC.
- S. R. Kumar, A. Idhayadhulla, A. J. A. Nasser and J. Selvin, Synthesis and antimicrobial activity of a new series 1,4-dihydropyridine derivatives, J. Serb. Chem. Soc., 2011, 76, 1–11 CrossRef.
- J. Lloyd, H. J. Finlay, W. Vacarro, T. Hyunh, A. Kover, R. Bhandaru, L. Yan, K. Atwal, M. L. Conder and T. Jenkins-West, Pyrrolidine amides of pyrazolodihydropyrimidines as potent and selective Kv1.5 blockers, Bioorg. Med. Chem. Lett., 2010, 20, 1436–1439 CrossRef CAS PubMed.
- Â. de Fátima, T. C. Braga, L. d. S. Neto, B. S. Terra, B. G. F. Oliveira, D. L. da Silva and L. V. Modolo, A mini-review on biginelli adducts with notable pharmacological properties, J. Adv. Res., 2015, 6, 363–373 CrossRef PubMed.
- C. O. Kappe, Biologically active dihydropyrimidones of the biginelli-type—a literature survey, Eur. J. Med. Chem., 2000, 35, 1043–1052 CrossRef CAS PubMed.
Footnotes |
† Electronic supplementary information (ESI) available: Bond lengths/angles, tables, and additional figures. CCDC 2132862. For ESI and crystallographic data in CIF or other electronic format see DOI: https://doi.org/10.1039/d2qi02653h |
‡ These authors contributed equally to this work. |
|
This journal is © the Partner Organisations 2023 |
Click here to see how this site uses Cookies. View our privacy policy here.