DOI:
10.1039/D2QM01205G
(Research Article)
Mater. Chem. Front., 2023,
7, 1093-1099
Exciplex-induced TADF, persistent RTP and ML in a host–guest doping system†
Received
21st November 2022
, Accepted 23rd January 2023
First published on 26th January 2023
Abstract
The development of multifunctional luminescent materials is of great importance but really difficult, since different luminescent properties have different requirements for molecular structure. Herein, a universal exciplex platform was reported to construct multifunctional luminescent materials, including thermally-activated delayed fluorescence (TADF), persistent room temperature phosphorescence (RTP) and mechanoluminescence (ML). With compound PTZ-CN as an electron donor and its derivative of OPTZ-CN as an electron acceptor, the resultant exciplex system demonstrated efficient TADF with the quantum yield up to 61%, persistent RTP lasting for more than 3 minutes and bright blue ML emission. Thus, this work opens a new way to construct multifunctional luminescent materials, which will largely promote the further development of this research area.
Introduction
The research of organic luminescent materials has drawn great attention, because of their wide applications in bio-imaging, sensors, anti-counterfeiting and displays, etc.1–7 With different luminescence properties, luminogens generally present different photophysical processes and applicable scenarios. For instance, thermally-activated delayed fluorescence (TADF) indicates a radiative transition of singlet excitons after undergoing intersystem crossing (ISC) and reverse intersystem crossing (RISC) between excited singlet and triplet states.8,9 On account of this unique excited process, both singlet and triplet excitons can be utilized in TADF materials. Also, the corresponding emission lifetime could be much prolonged from nanoseconds in normal fluorescence to microseconds in TADF. When the excitons decay from the excited triplet state, phosphorescence occurs. To achieve persistent organic room temperature phosphorescence (RTP), the triplet excitons should be stabilized by specific external environments, such as crystallization, polymerization, supramolecular assembly, and host–guest doping.10–16 Accordingly, the lifetime of persistent RTP could even reach seconds. The advantages of long emission lifetime and full utilization of excitons in TADF and RTP materials offer them great potential in the applications of time-resolved bio-imaging, anti-counterfeiting and organic light-emitting diodes (OLEDs).17–19 Besides, some organic systems were found to present mechanoluminescence (ML), another important type of luminescence induced by mechanical stimulation such as grinding, rubbing, or shaking.20–24 Systematic studies indicated that ML was heavily related to the piezoelectric effect, in which the singlet and triplet excitons could be formed through the recombination of positive and negative charges at the broken interfaces. An ML material sensitive to mechanical force could be utilized in the applications of pressure sensors and heartbeat monitors etc.25–27 Thus, regardless of the different emissive properties, the behaviors of TADF and RTP, in addition to ML, are all heavily related to the singlet and triplet excitons; therefore, the inherent mechanism could be considered much more deeply if there were some luminogens having all these three different emitting properties.
To date, the designs of organic luminescent materials are mostly based on a single property, since different luminescent properties have different requirements for molecular structure. In the design of TADF materials, separated HOMO and LUMO orbitals in space are needed, thus minimizing the energy gap (ΔEST) between the S1 and T1 states.28 To prolong the emission lifetime (τp) in RTP materials, specific intermolecular interaction forms should be presented to minimize both radiative (kp,r) and non-radiative (kp,nr) transitions of triplet excitons, according to the equation τp = 1/(kp,r + kp,nr).29 As for organic ML materials, a non-centrosymmetric crystal packing with large dipole moment is of great importance.30 Therefore, it is difficult to construct multifunctional luminescent materials due to the lack of a universal platform to meet all of their different requirements. Nevertheless, if these luminescent properties could be successfully integrated into one system, multiple advances can be expected. On the one hand, the properties and applications of luminescent materials could be largely enriched. On the other hand, different luminescent properties might have a mutually promoting relationship. For example, the exciton transfer between TADF and RTP could largely prolong their corresponding lifetimes.31 Also, the full utilization of the excited state energy in TADF and RTP will contribute much to the realization of bright ML emission.32 Thus, it will be of great significance to develop multifunctional luminescent materials.
In previous works, we have found that phenothiazine (PTZ) and phenothiazine 5,5-dioxide (OPTZ) groups were good candidates to construct RTP materials.10,33 When they were mixed together to form a doping system, a stable exciplex with a new energy level could be formed in some cases.34 To further adjust the energy level of the exciplex and construct multifunctional luminescence materials, the cyano group (–CN) with electron-withdrawing ability was introduced in this work (Fig. 1). Indeed, multifunctional luminescent properties were successfully achieved with the formation of the exciplex. For the exciplex, compound PTZ-CN acted as an electron donor (D), while its derivative of OPTZ-CN was the electron acceptor (A). Firstly, separated HOMO and LUMO orbitals in space could be realized in the exciplex, thus minimizing the energy gap (ΔEST) between the S1 and T1 states. Secondly, the exciton transfer between the exciplex and other components would prolong the exciton lifetime. Thirdly, an increased dipole moment could be expected in the D–A exciplex. Thus, although the individual PTZ-CN and OPTZ-CN crystals just showed a moderate RTP effect, multifunctional luminescent properties could be achieved for their host–guest doping system with the formation of an exciplex, including thermally-activated delayed fluorescence (TADF), persistent room temperature phosphorescence (RTP) and mechanoluminescence (ML).
 |
| Fig. 1 Exciplex-induced thermally-activated delayed fluorescence (TADF), persistent room temperature phosphorescence (RTP) and mechanoluminescence (ML) in a doping system with OPTZ-CN as the host and PTZ-CN as the guest. | |
Results and discussion
As shown in Fig. 2(A) and Fig. S1–S5 (ESI†), the individual OPTZ-CN and PTZ-CN crystals only show a moderate RTP effect. For OPTZ-CN, two phosphorescence peaks exist, in which, one peak is at 425 nm with the lifetime of 110 ms and the other one is at 485 nm with a relatively longer lifetime of 133 ms. As for PTZ-CN, its RTP peak locates at about 500 nm with a much shorter lifetime of 43 ms. Also, their PL efficiencies are relatively low, at just 4% and 16%, respectively. Interestingly, when they are mixed together to crystallize with the mass ratio of 99
:
1, the resultant doping system of M-CN shows a much boosted PL efficiency of 61%. Furthermore, the steady-state and delayed PL spectra of M-CN are almost exactly overlapped with the emission peak at about 485 nm. Besides, the excitation spectrum of M-CN is different from those of PTZ-CN and OPTZ-CN crystals, indicating the formation of new energy levels (Fig. S4, ESI†). Careful measurements of PL decay curves, coupled with the luminescent photos after UV-off, demonstrated that two decay components exist in M-CN (Fig. 2(B)). Among them, the former component is strong but relatively short, which shows the characteristic of TADF with a delayed fluorescence lifetime of 37 ms.35 As for the later component, a weak but long emission lasting for more than 3 min could be observed, which should be ascribed to the persistent RTP effect (Fig. 2(C) and Fig. S5, ESI†). Thus, the integration of TADF and persistent RTP was successfully realized in the host–guest doping system of M-CN.
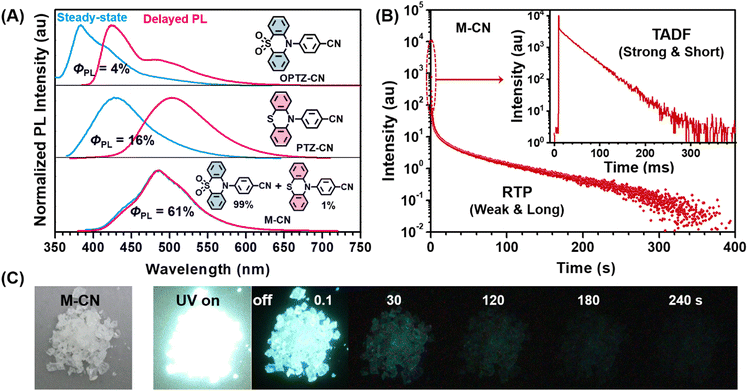 |
| Fig. 2 (A) The steady-state and delayed photoluminescence (PL) spectra of the OPTZ-CN crystal, PTZ-CN crystal and corresponding host–guest doping system (M-CN); (B) the PL decay curves of M-CN; (C) the luminescent behavior of M-CN (λex = 365 nm). | |
To make the internal mechanism clear, the UV-Vis absorption spectra of OPTZ-CN, PTZ-CN and M-CN were firstly measured. As shown in Fig. 3(A), an additional absorption band (∼370 nm) appears in the long wavelength region of M-CN, in comparison with OPTZ-CN and PTZ-CN. This indicates the occurrence of intermolecular charge transfer between OPTZ-CN and PTZ-CN.36 Also, this new absorption band well corresponded to the excitation spectrum of M-CN (@485 nm), suggesting the formation of an exciplex (Fig. S6, ESI†).37 Coupled with the HOMO/LUMO energy levels of compounds OPTZ-CN and PTZ-CN, it could be inferred that OPTZ-CN acts as an acceptor and PTZ-CN as a donor in the exciplex (Fig. S7 and S8, ESI†).38 Further on, the temperature-dependent PL behaviors were studied for the M-CN crystal (Fig. 3 and Table S1, Fig. S9, ESI†). As shown in Fig. 3(B) and (C), its PL intensity and lifetime both decrease with the temperature increasing, due to the accelerated non-radiative transition. Nevertheless, the delayed portion in the PL decay curves (bright & short component) presents obvious enhancement in the process of heating up, showing the typical characteristic of TADF.39 Also, the PL decay curve of the persistent phosphorescence (weak & long component) was measured at 77 K, which showed much slower decay than the one at room temperature for the restricted non-radiative transition (Fig. S10, ESI†). Thus, based on the temperature-dependent PL behaviors of the M-CN crystal, the existence of TADF and persistent RTP effects could be further certified.
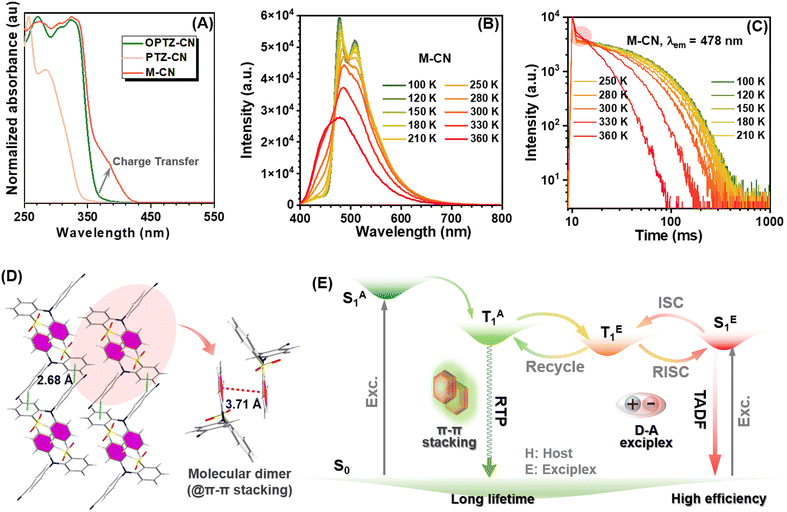 |
| Fig. 3 (A) Normalized UV-Vis absorption spectra of the OPTZ-CN crystal, PTZ-CN solution and M-CN crystal; (B) temperature-dependent PL spectra of the M-CN crystal; (C) temperature-dependent PL decay curves of the M-CN crystal at 478 nm; (D) single crystal structure of the OPTZ-CN crystal; (E) proposed mechanism for the unique luminescent behaviors of the M-CN crystal. | |
Further on, the phosphorescence behaviors of OPTZ-CN and PTZ-CN were studied at 77 K. As shown in Fig. S11 (ESI†), just one main phosphorescence band at about 430 nm could be observed for OPTZ-CN in both the solution and crystal states at 77 K, which corresponds to the RTP one of its crystal at short wavelength (425 nm). Coupled with the single crystal structure of OPTZ-CN (Fig. 3(D)), in which, an obvious molecular dimer with strong intermolecular π–π stacking (3.71 Å) exists, it could be inferred that the RTP peak at 425 nm should originate from the OPTZ-CN monomer, while the longer one at 485 nm is from the dimer-based excimer (Fig. S12, ESI†), just like other phenothiazine-5,5-dioxide derivatives in previous work.40 As for PTZ-CN, the phosphorescence bands of its solution and crystal at 77 K both well correspond to the RTP one of the crystal (Fig. S13, ESI†). On the other hand, the intermolecular π–π distances in the PTZ-CN crystal were as long as 4.59 and 5.18 Å, suggesting the absence of π–π interaction (Fig. S14, ESI†). Thus, it could be concluded that the RTP emission of PTZ-CN is from its monomer. When OPTZ-CN and PTZ-CN are mixed together with the mass ratio of 99
:
1 to form M-CN, the resultant molecular packing is still dominated by the OPTZ-CN crystal, as indicated by their powder X-ray diffraction (PXRD) patterns in Fig. S15 (ESI†).
Based on these PL behaviors and crystal structures, the excited process of M-CN in PL was proposed. As shown in Fig. 3(E), upon 365 nm UV irradiation, the excimer based on the OPTZ-CN dimer and exciplex based on OPTZ-CN/PTZ-CN would be both excited and jump from the S1 to T1 states through ISC transition. Because of the small energy gap (ΔEST) between S1 and T1 in the exciplex, RISC could happen, then leading to efficient TADF emission from it. On the other hand, the T1 states for the excimer based on the OPTZ-CN dimer and exciplex based on OPTZ-CN/PTZ-CN are both at about 2.56 eV, which allows their corresponding triplet excitons to make a cyclic transfer between them. Thus, their exciton lifetimes are much prolonged, thus resulting in an abnormal long lifetime of TADF (37 ms) from the exciplex (OPTZ-CN/PTZ-CN) and persistent RTP lasting for more than 3 min from the excimer (OPTZ-CN dimer). Thus, the exciplex as a good platform to construct TADF and persistent RTP materials could be well certified, in which, the TADF and RTP properties have a mutually promoting relationship in emission lifetime.
It is worth emphasizing that long-lived TADF and RTP are unusual and may result from a combination of causes. Besides the cyclic transfer of different excitons to prolong their lifetimes, and rigid molecular stacking of the M-CN crystal should also be crucial.41–44OPTZ-CN and PTZ-CN have similar molecular structures/conformations, so the M-CN crystal containing a small amount of PTZ-CN can still maintain good rigidity, which is beneficial for the suppression of non-radiative transitions. This is corroborated by the afterglow behaviours of the M-CN crystals with different mass ratios of PTZ-CN (Fig. S16, ESI†). In these doping systems, the afterglow shortens significantly with higher PTZ-CN ratios, indicating that the exciton lifetime decreases with lower crystal structure integrity. From another perspective, we can also assume that the excitons can be effectively blocked by the acceptor at low donor concentration, which reduces the quenching probability and prolongs the excitons’ lifetime.
Further on, the M-CN crystal was found to show bright blue mechanoluminescence (ML) under the mechanical stimulation of a glass rod, such as grinding and rubbing, while both PTZ-CN and OPTZ-CN were ML in-active (Fig. 4(A)). As shown in Fig. S17 (ESI†), the ML intensity of M-CN decreased with the increase of the doping concentration for PTZ-CN, and there was almost no ML after the mass ratio of PTZ-CN was higher than 33%. Additionally, the ML spectrum of M-CN was well consistent with the PL one, indicating that the ML emission of M-CN is also from the excited state of the exciplex. According to the previous reports, most ML emissions came from the piezoelectric effect of the crystal, which was heavily related to the non-centrosymmetric molecular packing with large dipole moment (Fig. S18, ESI†).45 For the host molecule of OPTZ-CN, its crystal presents a centrosymmetric space group of P
. Correspondingly, the dipole moment for the OPTZ-CN dimer with π–π stacking was calculated to be nearly zero, which is not conducive to the ML emission (Fig. 4(B)). When one OPTZ-CN molecule in its dimer was replaced by PTZ-CN to form an exciplex (OPTZ-CN/PTZ-CN), the dipole moment could be largely increased to 4.5418 D. Thus, after PTZ-CN was doped in OPTZ-CN to form an exciplex, the centrosymmetric molecular packing of the OPTZ-CN crystal was broken, which then contributed much to the piezoelectric effect and resultant ML emission. Based on this mechanism, the singlet and triplet excitons were formed through the recombination of positive and negative charges at the interface, just like electroluminescence (EL).46 Therefore, the TADF effect induced by the exciplex would also contribute much to the promotion of bright ML emission, as it could make full use of the excited state energy from both singlet and triplet excitons. Thus, the exciplex as a good platform to construct ML materials could also be well confirmed. Also, the promotion of exciplex-induced TADF on ML effect could be realized, demonstrating the great advances in multifunctional luminescent materials.
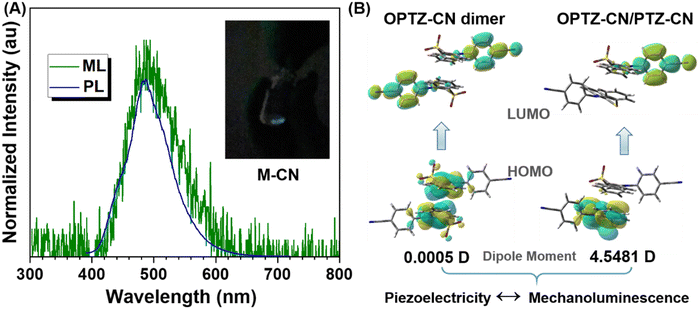 |
| Fig. 4 (A) Normalized PL and ML spectra of the M-CN crystal; (B) theoretical calculations of the HOMO/LUMO orbitals and dipole moments for the OPTZ-CN dimer and OPTZ-CN/PTZ-CN exciplex. | |
Conclusions
In this work, a host–guest doping system was developed with compound PTZ-CN as the guest and its derivative of OPTZ-CN as the host. Although just moderate RTP effects were presented for the individual OPTZ-CN and PTZ-CN crystals, their doping system of M-CN was found to show excellent multifunctional luminescent properties, including TADF, persistent RTP and ML. Experimental results showed that the formation of an exciplex between OPTZ-CN and PTZ-CN played a significant role, as it could lead to the minimized energy gap (ΔEST) between the S1 and T1 states, prolonged exciton lifetime and increased dipole moment. Also, these different luminescent properties can promote each other, showing the great advances in multifunctional luminescent materials. Thus, an exciplex as a universal platform to construct multifunctional luminescent materials was well demonstrated.
Author contributions
Jie Yang and Zhen Li conceived and planned this research; Yusheng Wang, Mingxue Gao, Jia Ren, Jiayi Liang and Yao Zhao performed the experiments; Jie Yang, Yunsheng Wang and Manman Fang performed data analysis; Yunsheng Wang, Jie Yang and Zhen Li wrote the manuscript; Zhen Li and Manman Fang gave the suggestions. All authors discussed the results and approved the final version of the manuscript.
Conflicts of interest
There are no conflicts to declare.
Acknowledgements
We are grateful to the National Natural Science Foundation of China (No. 52273191, 22235006, 51903188), the Open Project Program of Wuhan National Laboratory for Optoelectronics (No. 2020WNLOKF013), the starting Grants of Tianjin University and the Tianjin Government for financial support.
Notes and references
- G. Feng and B. Liu, Aggregation-Induced Emission (AIE) Dots: Emerging Theranostic Nanolights, Acc. Chem. Res., 2018, 51, 1404–1414 CrossRef CAS PubMed.
- M. Fang, J. Yang and Z. Li, Light emission of organic luminogens: Generation, mechanism and application, Prog. Mater. Sci., 2022, 125, 100914 CrossRef CAS.
- Q. Li and Z. Li, Molecular Packing: Another Key Point for the Performance of Organic and Polymeric Optoelectronic Materials, Acc. Chem. Res., 2020, 53, 962–973 CrossRef CAS PubMed.
- J. Ma, Y. Zhou, H. Gao, F. Zhu and G. Liang, Full-type photoluminescence from a single organic molecule for multi-signal temperature sensing, Mater. Chem. Front., 2021, 5, 2261–2270 RSC.
- Y. Huang, J. Xing, Q. Gong, L. Chen, G. Liu, C. Yao, Z. Wang, H. Zhang, Z. Chen and Q. Zhang, Reducing aggregation caused quenching effect through co-assembly of PAH chromophores and molecular barriers, Nat. Commun., 2019, 10, 169 CrossRef PubMed.
- M. Han, Z. Xu, J. Lu, Y. Xie, Q. Li and Z. Li, Intramolecular-locked triphenylamine derivatives with adjustable room temperature phosphorescence properties by the substituent effect, Mater. Chem. Front., 2022, 6, 33–39 RSC.
- Z. Cong, M. Han, Y. Fan, Y. Fan, K. Chang, L. Xiao, Y. Zhang, X. Zhen, Q. Li and Z. Li, Ultralong blue room-temperature phosphorescence by cycloalkyl engineering, Mater. Chem. Front., 2022, 6, 1606–1614 RSC.
- X. Li, S. Shen, C. Zhang, M. Liu, J. Lu and L. Zhu, Small-molecule based thermally activated delayed fluorescence materials with dual-emission characteristics, Sci. China: Chem., 2021, 64, 534–546 CrossRef CAS.
- H. Chen, H. Liu, Y. Xiong, J. He, Z. Zhao and B. Z. Tang, New aggregation-induced delayed fluorescent materials for efficient OLEDs with high stabilities of emission color and efficiency, Mater. Chem. Front., 2022, 6, 924–932 RSC.
- J. Yang, X. Zhen, B. Wang, X. Gao, Z. Ren, J. Wang, Y. Xie, J. Li, Q. Peng, K. Pu and Z. Li, The influence of the molecular packing on the room temperature phosphorescence of purely organic luminogens, Nat. Commun., 2018, 9, 840 CrossRef PubMed.
- X. K. Ma and Y. Liu, Supramolecular purely organic room-temperature phosphorescence, Acc. Chem. Res., 2021, 54, 3403–3414 CrossRef CAS PubMed.
- Y. Wang, J. Yang, M. Fang, Y. Yu, B. Zou, L. Wang, Y. Tian, J. Cheng, B. Z. Tang and Z. Li, Förster resonance energy transfer: an efficient way to develop stimulus-responsive room-temperature phosphorescence materials and their applications, Matter, 2020, 3, 449–463 CrossRef.
- D. Li, J. Yang, M. Fang, B. Z. Tang and Z. Li, Stimulus-responsive room temperature phosphorescence materials with full-color tunability from pure organic amorphous polymers, Sci. Adv., 2022, 8, eabl8392 CrossRef CAS PubMed.
- Y. Wang, J. Yang, Y. Gong, M. Fang, Z. Li and B. Z. Tang, Host–guest materials with room temperature phosphorescence: Tunable emission color and thermal printing patterns, SmartMat, 2020, 1, e1006 Search PubMed.
- J. Yang, M. Fang and Z. Li, Stimulus-Responsive Room Temperature Phosphorescence Materials: Internal Mechanism, Design Strategy, and Potential Application, Acc. Mater. Res., 2021, 2, 644–654 CrossRef CAS.
- Y. Gong, J. Yang, M. Fang and Z. Li, Room-temperature phosphorescence from metal-free polymer-based materials, Cell Rep. Phy. Sci., 2022, 3, 100663 CrossRef CAS.
- X. Zhen, R. Qu, W. Chen, W. Wu and X. Jiang, The development of phosphorescent probes for in vitro and in vivo bioimaging, Biomater. Sci., 2021, 9, 285–300 RSC.
- C. Zhou, C. Cao, D. Yang, X. Cao, H. Liu, D. Ma, C. Lee and C. Yang, Highly efficient red thermally activated delayed fluorescence emitters by manipulating the molecular horizontal orientation, Mater. Chem. Front., 2021, 5, 3209–3215 RSC.
- J. Yang, M. Fang and Z. Li, Stimulus-responsive room temperature phosphorescence in purely organic luminogens, InfoMat, 2020, 2, 791–806 CrossRef CAS.
- H. Zhang, B. Ma, F. Lin, Z. Yang, G. Huang, B. S. Li and B. Z. Tang, New shoots from old roots: multiple stimuli-responsive properties of a common tetraphenylethene derivative, Mater. Chem. Front., 2022, 6, 176–181 RSC.
- S. Li, Y. Xie, A. Li, X. Li, W. Che, J. Wang, H. Shi and Z. Li, Different Molecular Conformation and Packing Determining Mechanochromism and Room Temperature Phosphorescence, Sci. China Mater., 2021, 64, 2813–2823 CrossRef CAS.
- J. Yang, Z. Ren, Z. Xie, Y. Liu, C. Wang, Y. Xie, Q. Peng, B. Xu, W. Tian, F. Zhang, Z. Chi, Q. Li and Z. Li, AIEgen with Fluorescence–Phosphorescence Dual Mechanoluminescence at Room Temperature, Angew. Chem., Int. Ed., 2017, 3, 880–884 CrossRef PubMed.
- W. Li, Q. Huang, Z. Mao, Q. Li, L. Jiang, Z. Xie, R. Xu, Z. Yang, J. Zhao, T. Yu, Y. Zhang, M. P. Aldred and Z. Chi, Alkyl Chain Introduction: In Situ Solar-Renewable Colorful Organic Mechanoluminescence Materials, Angew. Chem., Int. Ed., 2018, 57, 12727–12732 CrossRef CAS PubMed.
- Y. Xie and Z. Li, The development of mechanoluminescence from organic compounds: breakthrough and deep insight, Mater. Chem. Front., 2020, 4, 317–331 RSC.
- C. Wang, Y. Yu, C. Ren, Q. Liao, J. Wang, Z. Chai, Q. Li and Z. Li, Heartbeat-sensing mechanoluminescent device based on a quantitative relationship between pressure and emissive intensity, Matter, 2019, 2, 181–193 CrossRef.
- Y. Xie and Z. Li, Triboluminescence: recalling interest and new aspects, Chem, 2018, 4, 943–971 CAS.
- Y. Zhuang and R. Xie, Mechanoluminescence rebrightening the prospects of stress sensing: a review, Adv. Mater., 2021, 33, 2005925 CrossRef CAS PubMed.
- Q. Xue and G. Xie, Thermally Activated Delayed Fluorescence beyond Through-Bond Charge Transfer for High-Performance OLEDs, Adv. Opt. Mater., 2021, 9, 2002204 CrossRef CAS.
- A. D. Nidhankar, Goudappagouda, V. C. Wakchaure and S. S. Babu, Efficient metal-free organic room temperature phosphors, Chem. Sci., 2021, 12, 4216–4236 RSC.
- J. I. Zink, Triboluminescence, Acc. Chem. Res., 1978, 11, 289–295 CrossRef CAS.
- B. Zhou and D. Yan, Hydrogen-Bonded Two-Component Ionic Crystals Showing Enhanced Long-Lived Room-Temperature Phosphorescence via TADF-Assisted Förster Resonance Energy Transfer, Adv. Funct. Mater., 2019, 29, 1807599 CrossRef.
- D. Li, J. Yang, Y. Wang, X. Li, D. Zhu, M. Fang and Z. Li, Bright mechanoluminescent luminogens even in daylight through close intermolecular interaction with the characteristic of hybridized local and charge transfer (HLCT), J. Mater. Chem. C, 2020, 8, 10852–10858 RSC.
- Y. Wang, J. Yang, M. Fang, Y. Gong, J. Ren, L. Tu, B. Z. Tang and Z. Li, New Phenothiazine Derivatives That Exhibit Photoinduced Room-Temperature Phosphorescence, Adv. Funct. Mater., 2021, 31, 2101719 CrossRef CAS.
- Y. Wang, H. Gao, J. Yang, M. Fang, D. Ding, B. Z. Tang and Z. Li, High Performance of Simple Organic Phosphorescence Host–Guest Materials and their Application in Time Resolved Bioimaging., Adv. Mater., 2021, 33, 2007811 CrossRef CAS PubMed.
- J. Jin, H. Jiang, Q. Yang, L. Tang, Y. Tao, Y. Li, R. Chen, C. Zheng, Q. Fan, K. Y. Zhang, Q. Zhao and W. Huang, Thermally activated triplet exciton release for highly efficient tri-mode organic afterglow, Nat. Commun., 2020, 11, 842 CrossRef CAS PubMed.
- L. Sun, W. Zhu, W. Wang, F. Yang, C. Zhang, S. Wang, X. Zhang, R. Li, H. Dong and W. Hu, Intermolecular Charge-Transfer Interactions Facilitate Two-Photon Absorption in Styrylpyridine–Tetracyanobenzene Cocrystals, Angew. Chem., Int. Ed., 2017, 56, 7831–7835 CrossRef CAS PubMed.
- E. Lim, Molecular triplet excimers, Acc. Chem. Res., 1987, 20, 8–17 CrossRef CAS.
- M. Sarma and K. Wong, Exciplex: An Intermolecular Charge-Transfer Approach for TADF, ACS Appl. Mater. Interfaces, 2018, 10, 19279–19304 CrossRef CAS PubMed.
- I. S. Park, H. Min and T. Yasuda, Phenyl-triggered photophysical switching between normal fluorescence and delayed fluorescence in phthalonitrile-based luminophores, Aggregate, 2021, 2, 145–150 CrossRef.
- Z. Liu, Y. Tian, J. Yang, A. Li, Y. Wang, J. Ren, M. Fang, B. Z. Tang and Z. Li, Direct demonstration of triplet excimer in purely organic room temperature phosphorescence through rational molecular design, Light: Sci. Appl., 2022, 11, 142 CrossRef CAS PubMed.
- A. Huang, Q. Li and Z. Li, Molecular Uniting Set Identified Characteristic (MUSIC) of Organic Optoelectronic Materials, Chin. J. Chem., 2022, 40, 2359–2370 Search PubMed.
- J. Wang, Q. Dang, Y. Gong, Q. Liao, G. Song, Q. Li and Z. Li, Precise Regulation of Distance between Associated Pyrene Units and Control of Emission Energy and Kinetics in Solid State, CCS Chem., 2021, 3, 274–286 CrossRef CAS.
- Y. Fan, S. Liu, M. Wu, L. Xiao, Y. Fan, M. Han, K. Chang, Y. Zhang, X. Zhen, Q. Li and Z. Li, Mobile Phone Flashlight Excited Red Afterglow Bioimaging, Adv. Mater., 2022, 34, 202201280 Search PubMed.
- Y. Xie and Z. Li, Approaching aggregated state chemistry accelerated by aggregation-induced emission, Nat. Sci. Rev., 2021, 8, nwaa199 CrossRef PubMed.
- Y. Tian, X. Yang, Y. Gong, Y. Wang, M. Fang, J. Yang, Z. Tang and Z. Li, The initial attempt to reveal the emission processes of both mechanoluminescence and room temperature phosphorescence with the aid of circular dichroism in solid state, Sci. China: Chem., 2021, 64, 445–451 CrossRef CAS.
- S. Ying, J. Lv, Y. Li, Y. Huo, Y. Liu, D. Ma, L. Peng and S. Yan, A large-scale deep-blue tetraphenylbenzene-bridged hybridized local and charge transfer fluorophore exhibiting small efficiency roll-off and low amplified spontaneous emission threshold, Mater. Chem. Front., 2022, 6, 2085–2094 RSC.
Footnote |
† Electronic supplementary information (ESI) available: Syntheses, photophysical properties and single crystal structures of the target compounds and the corresponding doping system. CCDC 2235978, 2216776 and 2216826. For ESI and crystallographic data in CIF or other electronic format see DOI: https://doi.org/10.1039/d2qm01205g |
|
This journal is © the Partner Organisations 2023 |
Click here to see how this site uses Cookies. View our privacy policy here.