DOI:
10.1039/D2QO01529C
(Research Article)
Org. Chem. Front., 2023,
10, 62-67
A substrate-controlled Ru(II)-catalyzed C–H activation/[5 + 2] annulation cascade and unusual acyl migration to synthesize diverse indoline scaffolds†
Received
26th September 2022
, Accepted 5th November 2022
First published on 8th November 2022
Abstract
A substrate-controlled Ru(II)-catalyzed C–H activation/[5 + 2] annulation cascade and unusual acyl migration to synthesize diverse indoline scaffolds are reported. The most pronounced advantage is that this strategy can offer a highly selective C–H activation of N-benzamidine indoline with two reaction sites prone to C–H activation to selectively prepare a 1,7-fused indoline scaffold and a C7-alkylated/N′-acylated indoline ring by varying its diazo synthons. More importantly, the NH directing group of N-aryl benzamidines is fully utilized and atom-economically integrated into the target products with a good yield and broad substrate scope.
Introduction
Indolines are classical privileged scaffolds that exist widely in natural products and drug molecules,1 among which fused indolines with a seven-membered ring and alkylated indolines have attracted more attention because of their various pharmacological activities (Fig. 1), such as anti-inflammatory2 and anti-tumor activities,3 regulation of appetite and feeding,4 treatment of aldosteronism5 and metabolic disorders,6 and neuroprotection.7 However, only a limited number of synthetic methods have been reported for these intriguing seven-membered 1,7-fused indoline backbones.8 Thus, the development of facile and atom-economical methods for their rapid construction is highly desired.
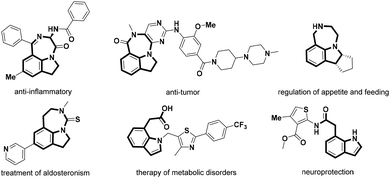 |
| Fig. 1 Related bioactive products. | |
Transition-metal catalyzed C–H activation and functionalization have recently been playing an important role in the construction of diverse heterocyclic frameworks, wherein most start with the cleavage of the C–H bond induced by an eligible directing group (DG), followed by an annulation with different one-carbon or two-carbon coupling partners.9 Literature surveys indicated that imidamides as a DG can couple with different synthons to construct indoles,10 benzimidazoles,11 quinolines,12 quinazolines,13 benzodiazepines,14 and isoquinolines.15 However, most of these require the introduction of an N-alkyl or a C-alkyl group to overcome the selectivity problem of C–H bond activation sites from different aryl groups. For example, Ackermann16 and Cheng17 reported the C–H activation of N-tBu benzamidines/[4 + 2] annulation with diazo compounds to construct substituted isoquinolines (Scheme 1a). Li18 and Fan19 constructed interesting indole skeletons from N-aryl benzamidines through a C–H activation/[4 + 1] annulation strategy. Interestingly, Fan20 had also used the two substrates to realize the construction of complex spiroheterocycles through a C–H activation/[4 + 1 + 1] annulation cascade (Scheme 1b). As part of our consistent efforts to explore new strategies for the construction of diverse drug-like scaffolds,21 we have attempted to introduce N-aryl benzamidines with two C–H activation sites as the substrate in the hope of overcoming the selectivity problem to obtain distinct heterocyclic scaffolds. Surprisingly, when introducing phenyl amidine into the indoline ring as one of the substrates, we could selectively form a 1,7-fused indoline scaffold and a C-7 alkylated/N′-acylated indoline ring by controlling different substituted diazo-substrates via a Ru(II)-catalyzed C–H activation/[5 + 2] annulation cascade and an unusual acyl migration, respectively (Scheme 1). It is particularly worth mentioning that this strategy can offer a highly selective C–H activation of N-benzamidine indoline containing two sites prone to C–H activation, and obtain two kinds of intriguing heterocyclic scaffolds by a C–H activation/[5 + 2] annulation cascade or an unusual acyl migration with a broad substrate scope; in particular, the NH group of imine is fully utilized and blended into the target products, showing good atom economy. Herein, we report these new findings.
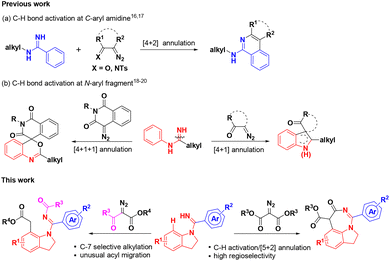 |
| Scheme 1 Transition-metal catalyzed C–H activation. | |
Results and discussion
Initially, indolin-1-yl(phenyl) methanimine (1a, 0.1 mmol) and dimethyl diazomalonate (2a, 0.15 mmol) as the model substrates were treated with [Ru(p-cymene)Cl2]2 (4 mol%), AgSbF6 (20 mol%), and KOAc (0.2 mmol) in 1,2-dichloroethane (DCE) at 80 °C for 12 h, and interestingly the [5 + 2] annulation 1,7-fused indoline heterocycle 3aa, which is not a routinely obtained [5 + 1] annulation product, was afforded in 33% yield and its structure was unambiguously confirmed by X-ray crystallographic analysis (Table 1, entry 1).22 With this intriguing structural scaffold in hand, we further screened different additives, such as Zn(OAc)2, CsOAc, PivOH, succinic acid, benzoic acid, and HOAc (Table 1, entries 2–7), and the results indicated that HOAc was the best choice for this transformation. Two other transition-metal catalysts ([Cp*RhCl2]2 and [Cp*IrCl2]2) were subsequently explored and [Ru(p-cymene)Cl2]2 was still the optimal catalyst for the formation of 3aa (Table 1, entries 8 and 9). Although the investigation of different Ag salts showed that AgOAc could give good catalytic efficiency (Table 1, entries 10–13), the removal of the Ag salt seemed to be able to provide a better yield (up to 86%, Table 1, entry 14), which indicated that the Ag salt was not necessary. We next explored the reaction solvents, and the results demonstrated that DCE was superior to acetone, toluene, DMF, CH3CN, and THF (Table 1, entries 15–19). Based on these results, the effects of temperature and time on this reaction were also scrutinized (Table 1, entries 20–22). We found that the reaction could proceed smoothly at 60 °C with a 97% yield in only 2 h (Table 1, entry 22). However, the control experiment showed that the target product could not be obtained in the absence of [Ru(p-cymene)Cl2]2 (Table 1, entry 23).
Table 1 Optimization of reaction conditionsa

|
Entry |
Ag salt |
Additive |
Solvent |
Yieldb (%) |
General reaction conditions: 1a (0.1 mmol), 2a (0.15 mmol), [Ru(p-cymene)Cl2]2 (4 mol%), additive (0.2 mmol), Ag salt (20 mol%) in solvent (2 mL) at 80 °C in an oil bath, under air, for 12 h.
Determined by 1H NMR spectroscopy using CH2Br2 as the internal standard.
[Cp*RhCl2]2 (4 mol%).
[Cp*IrCl2]2 (4 mol%).
At 60 °C.
At 100 °C.
At 60 °C in an oil bath for 2 h.
Without [Ru(p-cymene)Cl2]2. DCE: 1,2-dichloroethane. THF: tetrahydrofuran. |
1 |
AgSbF6 |
KOAc |
DCE |
33 |
2 |
AgSbF6 |
Zn(OAc)2 |
DCE |
21 |
3 |
AgSbF6 |
CsOAc |
DCE |
12 |
4 |
AgSbF6 |
PivOH |
DCE |
40 |
5 |
AgSbF6 |
Succinic acid |
DCE |
40 |
6 |
AgSbF6 |
Benzoic acid |
DCE |
54 |
7 |
AgSbF6 |
HOAc |
DCE |
55 |
8c |
AgSbF6 |
HOAc |
DCE |
37 |
9d |
AgSbF6 |
HOAc |
DCE |
18 |
10 |
AgOAc |
HOAc |
DCE |
77 |
11 |
AgOTf |
HOAc |
DCE |
72 |
12 |
AgOPiv |
HOAc |
DCE |
71 |
13 |
AgBF4 |
HOAc |
DCE |
69 |
14 |
— |
HOAc |
DCE |
86 |
15 |
— |
HOAc |
Acetone |
40 |
16 |
— |
HOAc |
Toluene |
45 |
17 |
— |
HOAc |
DMF |
Trace |
18 |
— |
HOAc |
CH3CN |
28 |
19 |
— |
HOAc |
THF |
25 |
20e |
— |
HOAc |
DCE |
91 |
21f |
— |
HOAc |
DCE |
86 |
22g |
— |
HOAc
|
DCE
|
97
|
23h |
— |
HOAc |
DCE |
0 |
With the optimized reaction conditions identified, we first investigated the scope of indolin-1-yl(phenyl) methanimine (1a–1w) by performing the reactions with diazomalonate compounds 2a–2f (Table 2). Generally, this catalytic conversion proved to be very compatible with a wide variety of N-substituted indolines and provided the corresponding [5 + 2] annulation 1,7-fused indoline products in good yields. For example, separately introducing different electron-donating groups, electron-withdrawing groups, and halogen groups at the R1 position of substrate 1a could smoothly deliver the products in moderate to excellent yields, sometimes in up to 91% yield (3ba–3ja); however, the substituents at the C6-position would result in a little decreased yield, probably due to steric hindrance (3ka and 3la). Thus, it is not difficult to conclude that introducing two methoxyl groups at the C5- and C6-position of 1a would be detrimental to the yield of product (3ma), but surprisingly, disubstituted indolines with two halogen atoms were well tolerated and gave good yields of the target products (3na and 3oa). Interestingly, when we introduced a cyclopentane at the C3-position of 1a to form an intriguing spiroindoline, the corresponding spiroheterocycle product 3pa was obtained in 75% yield. Additionally, we have investigated the R2 substituents of substrate 1a and the products could be obtained with good to excellent efficiencies when the substituents on R2 were 4-OMe, 4-F, and 3,5-dimethyl, respectively (3qa–3sa). When thienyl was used instead of C-phenyl, 3ta was still obtained in a good yield. However, the products could not be obtained by replacing C-phenyl with methyl or cyclopropyl (3ua and 3va). Moreover, N-methyl-N-phenylbenzimidamide (1w) could not react with 2a, indicating that the indoline skeleton was crucial for [5 + 2] annulation. Meanwhile, the tolerance of substrate 2 was also tested and the results demonstrated that different substituents introduced at the R3 moiety of diazomalonate compounds, such as ethyl, isopropyl, n-butyl, and benzyl groups, could smoothly react with 1a to give the desired products in 70–92% yields, except for that substituted by the t-butyl group owing to large steric hindrance (3ab–3af).
Table 2 Substrate scope of N-substituted indolines and diazo compoundsa,b
Reaction conditions: 1a (0.4 mmol), 2a (0.6 mmol), [Ru(p-cymene)Cl2]2 (4 mol%), HOAc (0.8 mmol) in DCE (4 mL) at 60 °C, under air, 2 h.
Isolated yields are reported.
For 12 h.
|
|
Very surprisingly, when we introduced an asymmetric diazo coupling partner (diazoketoester, 4a) to this transformation under the above optimal reaction conditions, an unanticipated new product 5aa was detected in 32% yield, which was identified to undergo a selective C-7 alkylation instead of the desired [5 + 2] annulation, accompanied by the simultaneous migration of the acyl group of the diazo partner to the NH group of the directing group. Further investigations on the reaction conditions by altering different catalysts, solvents, additives, and the equivalent amount indicated that [Ru(p-cymene)Cl2]2/AgSbF6 (4 mol%/20 mol%) with KOAc (3 equiv.) in DCE (2 mL) was the best catalytic system for this transformation to construct C-7 alkylated and N′-acylated indolines (see the ESI† for details about the screening of the reaction conditions). Consequently, we further investigated the generality of this new transformation. Moderate to good yields of the target products 5ba–5sa could be achieved by the treatment of diverse indoline substrates containing a fluoro, chloro, bromo, methyl, nitro, difluoro, dimethyl, and heterocyclic substitution at different positions (1b–1s) with the asymmetric diazo partner diazoketoester 4a (Table 3), in which compound 5sa was also verified by X-ray crystallographic analysis.22 Nonetheless, the yields of 5ua and 5va were very low when C-phenyl was replaced with methyl or cyclopropyl. Similarly, N-methyl-N-phenylbenzimidamide (1w) could not react with 4a, showing that the indoline scaffold was also important for the reaction of acyl migration. Besides, the scope of diazoketoester substrates was explored and a series of various substituted diazoketoesters (4b–4f) were found to be good reaction partners for this reaction, and products 5ab–5af were obtained in 50–74% yields, which indicated good substrate tolerance.
Table 3 Substrate scope of N-substituted indolines and diazo compoundsa,b
Reaction conditions: 1a (0.4 mmol), 2a (0.6 mmol), [Ru(p-cymene)Cl2]2 (4 mol%), AgSbF6 (20 mol%), KOAc (1.2 mmol) in DCE (4 mL) at 80 °C, under air, 12 h.
Isolated yields are reported.
|
|
To assess the efficiency and potential for utilization of these methods, gram-scale experiments of products 3aa and 5aa were conducted with 85% and 65% isolated yields, respectively (Scheme 2a). Besides, we investigated the further transformation potential of 3aa, such as the successful introduction of a side chain into the seven-membered ring of 3aa using allyl bromide as the alkylation reagent to prepare product 6 in 36% yield. Similarly, the active fragment cinnamyl group was also introduced into 3aa to obtain product 7 in 32% yield (Scheme 2b).
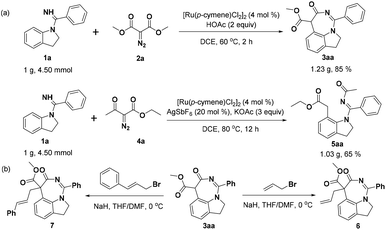 |
| Scheme 2 Gram-scale preparation and conversion of the product. | |
To shed light on the preliminary mechanism, several mechanistic experiments have been performed. In the H/D exchange experiments, the reaction of 1a with CD3COOD in the absence of synthon 2a gave 42% deuterium atom at the C7-position of indoline 1a, indicating that the C–H bond activation was reversible. Carrying out the same reaction in the presence of diazo compound 2a showed that 45% of hydrogen at the tertiary carbon of 3aa was deuterated. Meanwhile, 1H NMR analysis revealed 85% deuteration at the α-methylene group of 5aa (Scheme 3a). Furthermore, the competition experiments between the electron-withdrawing group substituted 1h and electron-donating group substituted 1f and 2a were performed, and the results indicated that the electron-poor arene reacted faster. Likewise, the competition experiments were also performed between substrates with different electronic properties and ethyl diazoacetate 4a and it was seen that the electron-rich substrate had better reactivity (Scheme 3b).
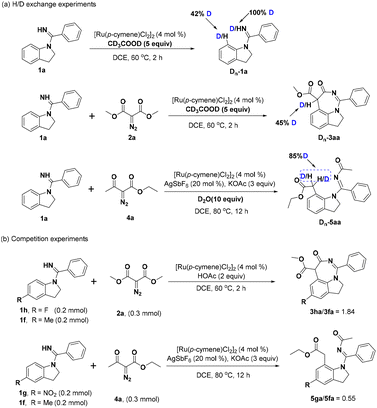 |
| Scheme 3 Mechanism studies. | |
Based on the preliminary mechanistic studies and previous reports,18 a plausible reaction mechanism is proposed (Scheme 4). Initially, the active catalyst is afforded through anion exchange, which then coordinates with 1a to form the ruthenacyclic intermediate A. Subsequently, the diazo substrate is coordinated with intermediate A by denitrogenation to produce the ruthenium carbene species B. Next, the Ru(II)–aryl bond experiences migratory insertion into the carbene unit to provide the seven-membered Ru(II) species C, which undergoes protonolysis and demetallization to afford intermediate E. For diazomalonate substrates, intermediate F is obtained through an intramolecular nucleophilic attack, followed by the elimination of alcohol to deliver the desired product 3aa. For diazoketoesters, intermediate G may undergo C–C bond cleavage to give the C–H alkylation/acyl group migration product 5aa.
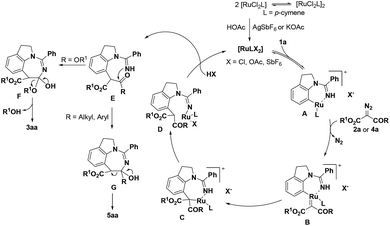 |
| Scheme 4 Proposed reaction mechanism. | |
Conclusions
In summary, we have successfully developed a substrate-controlled synthetic protocol to selectively establish two kinds of indoline scaffolds. The advantage of this approach is the ability to implement a highly selective C–H activation of N-benzamidine indolines involving two sites prone to C–H activation to further obtain two kinds of intriguing indoline scaffolds by a successive [5 + 2] annulation cascade or an unusual acyl migration with a broad substrate scope; in particular, the NH group of imine is fully utilized and blended into the target products, showing good atom economy. What is noteworthy is that the synthesized structural motifs widely exist in drug molecules and have potential biological activities.
Conflicts of interest
There are no conflicts to declare.
Acknowledgements
We are grateful to the National Natural Science Foundation of China (82173656, 21977106 and 82151219) and the Strategic Priority Research Program of Chinese Academy of Sciences (SIMM010109 and SIMM010111) for financial support.
References
-
(a) T. Kawasaki and K. Higuchi, Simple indole alkaloids and those with a nonrearranged monoterpenoid unit, Nat. Prod. Rep., 2005, 22, 761 RSC;
(b) A. J. Kochanowska-Karamyan and M. T. Hamann, Marine Indole Alkaloids: Potential New Drug Leads for the Control of Depression and Anxiety, Chem. Rev., 2010, 110, 4489 CrossRef CAS PubMed.
- C. Burnouf, E. Auclair, N. Avenel, B. Bertin, C. Bigot, A. Calvet, K. Chan, C. Durand, V. Fasquelle, F. Féru, R. Gilbertsen, H. Jacobelli, A. Kebsi, E. Lallier, J. Maignel, B. Martin, S. Milano, M. Ouagued, Y. Pascal, M.-P. Pruniaux, J. Puaud, M.-N. Rocher, C. Terrasse, R. Wrigglesworth and A. M. Doherty, Synthesis, Structure-Activity Relationships, and Pharmacological Profile of 9-Amino-4-oxo-1-phenyl-3,4,6,7-tetrahydro[1,4]diazepino[6,7,1-hi]indoles: Discovery of Potent, Selective Phosphodiesterase Type 4 Inhibitors, J. Med. Chem., 2000, 43, 4850 CrossRef CAS PubMed.
- J. M. Elkins, J. Wang, X. Deng, M. J. Pattison, J. S. C. Arthur, T. Erazo, N. Gomez, J. M. Lizcano, N. S. Gray and S. Knapp, X-ray Crystal Structure of ERK5 (MAPK7) in Complex with a Specific Inhibitor, J. Med. Chem., 2013, 56, 4413 CrossRef CAS.
- A. Ren, X. Zhu, K. Feichtinger, J. Lehman, M. Kasem, T. O. Schrader, A. Wong, H. Dang, M. Le, J. Frazer, D. J. Unett, A. J. Grottick, K. T. Whelan, M. E. Morgan, C. R. Sage and G. Semple, Discovery of a lead series of potent benzodiazepine 5-HT2C receptor agonists with high selectivity in functional and binding assays, Bioorg. Med. Chem. Lett., 2020, 30, 126929 CrossRef CAS PubMed.
-
R. W. Hartmann, R. Heim and S. Lucas, 2009, WO2009135651.
-
J. W. Malecha, C. Zhao, C. K. M. Robinson, S. G. Duron, M. Sertic and S. A. Noble, 2005, WO2005060958.
- S. Bowers, A. P. Truong, R. J. Neitz, M. Neitzel, G. D. Probst, R. K. Hom, B. Peterson, R. A. Galemmo, A. W. Konradi, H. L. Sham, G. Tóth, H. Pan, N. Yao, D. R. Artis, E. F. Brigham, K. P. Quinn, J.-M. Sauer, K. Powell, L. Ruslim, Z. Ren, F. Bard, T. A. Yednock and I. Griswold-Prenner, Design and synthesis of a novel, orally active, brain penetrant, tri-substituted thiophene based JNK inhibitor, Bioorg. Med. Chem. Lett., 2011, 21, 1838 CrossRef CAS.
-
(a) X. Wang, H. Tang, H. Feng, Y. Li, Y. Yang and B. Zhou, Access to Six- and Seven-Membered 1,7-Fused Indolines via Rh(III)-Catalyzed Redox-Neutral C7-Selective C–H Functionalization of Indolines with Alkynes and Alkenes, J. Org. Chem., 2015, 80, 6238 CrossRef CAS PubMed;
(b) T. Zhou, B. Li and B. Wang, Rhodium-catalyzed C-H activation of 3-(indolin-1-yl)-3-oxopropanenitriles with diazo compounds and tandem cyclization leading to hydrogenated azepino[3,2,1-hi]indoles, Chem. Commun., 2016, 52, 14117 RSC.
-
(a) X.-X. Guo, D.-W. Gu, Z. Wu and W. Zhang, Copper-Catalyzed C–H Functionalization Reactions: Efficient Synthesis of Heterocycles, Chem. Rev., 2015, 115, 1622 CrossRef CAS;
(b) T. Gensch, M. N. Hopkinson, F. Glorius and J. Wencel-Delord, Mild metal-catalyzed C–H activation: examples and concepts, Chem. Soc. Rev., 2016, 45, 2900 RSC;
(c) J. R. Hummel, J. A. Boerth and J. A. Ellman, Transition-Metal-Catalyzed C–H Bond Addition to Carbonyls, Imines, and Related Polarized π Bonds, Chem. Rev., 2017, 117, 9163 CrossRef CAS PubMed;
(d) Y. Park, Y. Kim and S. Chang, Transition Metal-Catalyzed C–H Amination: Scope, Mechanism, and Applications, Chem. Rev., 2017, 117, 9247 CrossRef CAS;
(e) P. Gandeepan, T. Muller, D. Zell, G. Cera, S. Warratz and L. Ackermann, 3d Transition Metals for C-H Activation, Chem. Rev., 2019, 119, 2192 CrossRef CAS PubMed;
(f) Y. Wu, C. Pi, Y. Wu and X. Cui, Directing group migration strategy in transition-metal-catalysed direct C–H functionalization, Chem. Soc. Rev., 2021, 50, 3677 RSC.
-
(a) R. Lai, X. Wu, S. Lv, C. Zhang, M. He, Y. Chen, Q. Wang, L. Hai and Y. Wu, Synthesis of indoles and quinazolines via additive-controlled selective C-H activation/annulation of N-arylamidines and sulfoxonium ylides, Chem. Commun., 2019, 55, 4039 RSC;
(b) C. Wu, J. Zhou, G. He, H. Li, Q. Yang, R. Wang, Y. Zhou and H. Liu, Ruthenium(II)-catalyzed selective C–H bond activation of imidamides and coupling with sulfoxonium ylides: an efficient approach for the synthesis of highly functional 3-ketoindoles, Org. Chem. Front., 2019, 6, 1183 RSC;
(c) H. B. Xu, Y. Y. Zhu and L. Dong, Specific Synthesis of 3H-Indole Derivatives via Rh(III)-Catalyzed Cascade Annulation between N-Phenylbenzimidamides and Pyridotriazoles, J. Org. Chem., 2019, 84, 16286 CrossRef CAS;
(d) Q. Yang, C. Wu, J. Zhou, G. He, H. Liu and Y. Zhou, Highly selective C–H bond activation of N-arylbenzimidamide and divergent couplings with diazophosphonate compounds: a catalyst-controlled selective synthetic strategy for 3-phosphorylindoles and 4-phosphorylisoquinolines, Org. Chem. Front., 2019, 6, 393 RSC;
(e) J. Zhou, J. Li, Y. Li, C. Wu, G. He, Q. Yang, Y. Zhou and H. Liu, Direct Synthesis of 3-Acylindoles through Rhodium(III)-Catalyzed Annulation of N-Phenylamidines with α-Cl Ketones, Org. Lett., 2018, 20, 7645 CrossRef CAS PubMed.
-
(a) Y. Li, C. Jia, H. Li, L. Xu, L. Wang and X. Cui, Rh(III)-Catalyzed Synthesis of 2-Alkylbenzimidazoles from Imidamides and N-Hydroxycarbamates, Org. Lett., 2018, 20, 4930 CrossRef CAS PubMed;
(b) Y. Hu, T. Wang, Y. Liu, R. Nie, N. Yang, Q. Wang, G.-B. Li and Y. Wu, Practical Synthesis of Benzimidazo[1,2-a]quinolines via Rh(III)-Catalyzed C–H Activation Cascade Reaction from Imidamides and Anthranils, Org. Lett., 2020, 22, 501 CrossRef CAS;
(c) X. Song, X. Cai, X. Zhang and X. Fan, Synthesis of N-acylbenzimidazoles through [4 + 1] annulation of N-arylpivalimidamides with dioxazolones, Org. Chem. Front., 2021, 8, 6265 RSC;
(d) L. Xu, L. Wang, Y. Feng, Y. Li, L. Yang and X. Cui, Iridium(III)-Catalyzed One-Pot Access to 1,2-Disubstituted Benzimidazoles Starting from Imidamides and Sulfonyl Azides, Org. Lett., 2017, 19, 4343 CrossRef CAS;
(e) S. Huang, H. Li, X. Sun, L. Xu, L. Wang and X. Cui, Rh(III)-Catalyzed Sequential C-H Amination/Annulation Cascade Reactions: Synthesis of Multisubstituted Benzimidazoles, Org. Lett., 2019, 21, 5570 CrossRef CAS.
- X. Zhou, Z. Qi, S. Yu, L. Kong, Y. Li, W.-F. Tian and X. Li, Synthesis of 2-Substituted Quinolines via Rhodium(III)-Catalyzed C-H Activation of Imidamides and Coupling with Cyclopropanols, Adv. Synth. Catal., 2017, 359, 1620 CrossRef CAS.
- F. Xu, W. F. Kang, Y. Wang, C. S. Liu, J. Y. Tian, R. R. Zhao and M. Du, Rhodium(III)-Catalyzed Cascade [5 + 1] Annulation/5-exo-Cyclization Initiated by C-H Activation: 1,6-Diynes as One-Carbon Reaction Partners, Org. Lett., 2018, 20, 3245 CrossRef CAS.
- X. Song, Q. Zhou, J. Zhao, Y. Jiang, X. Zhang, X. Zhang and X. Fan, Synthesis of 1,3-Benzodiazepines through [5 + 2] Annulation of N-Aryl Amidines with Propargylic Esters, Org. Lett., 2020, 22, 9506 CrossRef CAS PubMed.
-
(a) X. Wei, M. Zhao, Z. Du and X. Li, Synthesis of 1-Aminoisoquinolines via Rh(III)-Catalyzed Oxidative Coupling, Org. Lett., 2011, 13, 4636 CrossRef CAS;
(b) J. Li, M. John and L. Ackermann, Amidines for versatile ruthenium(II)-catalyzed oxidative C-H activations with internal alkynes and acrylates, Chem. – Eur. J., 2014, 20, 5403 CrossRef CAS;
(c) J. Li, Z. Zhang, M. Tang, X. Zhang and J. Jin, Selective Synthesis of Isoquinolines by Rhodium(III)-Catalyzed C-H/N-H Functionalization with alpha-Substituted Ketones, Org. Lett., 2016, 18, 3898 CrossRef CAS;
(d) Y. Zuo, X. He, Y. Ning, Y. Wu and Y. Shang, Selective Synthesis of Aminoisoquinolines via Rh(III)-Catalyzed C–H/N–H Bond Functionalization of N-Aryl Amidines with Cyclic 2-Diazo-1,3-diketones, J. Org. Chem., 2018, 83, 13463 CrossRef CAS PubMed;
(e) X. Huang, Y. Xu, J. Li, R. Lai, Y. Luo, Q. Wang, Z. Yang and Y. Wu, Synthesis of aminoisoquinolines via Rh-catalyzed [4 + 2] annulation of benzamidamides with vinylene carbonate, Chin. Chem. Lett., 2021, 32, 3518 CrossRef CAS;
(f) J. Ren, C. Pi, X. Cui and Y. Wu, Rhodium(III)-Catalyzed [4 + 2] Annulation of N-Arylbenzamidines with Propargyl Alcohols: Highly Regioselective Synthesis of 1-Aminoisoquinolines Controlled by Noncovalent Interaction, Org. Lett., 2021, 23, 6628 CrossRef CAS.
- J. Li, M. Tang, L. Zang, X. Zhang, Z. Zhang and L. Ackermann, Amidines for Versatile Cobalt(III)-Catalyzed Synthesis of Isoquinolines through C–H Functionalization with Diazo Compounds, Org. Lett., 2016, 18, 2742 CrossRef CAS.
- S. Xu, S. Qiao, S. Sun, J. T. Yu and J. Cheng, Rhodium-catalyzed C-H activation/annulation of amidines with 4-diazoisochroman-3-imines toward isochromeno[3,4-c]isoquinolines, Org. Biomol. Chem., 2019, 17, 8417 RSC.
-
(a) Y. Li, Z. Qi, H. Wang, X. Yang and X. Li, Ruthenium(II)-Catalyzed C−H Activation of Imidamides and Divergent Couplings with Diazo Compounds: Substrate-Controlled Synthesis of Indoles and 3H-Indoles, Angew. Chem., Int. Ed., 2016, 55, 11877 CrossRef CAS;
(b) Z. Qi, S. Yu and X. Li, Rh(III)-Catalyzed Synthesis of N-Unprotected Indoles from Imidamides and Diazo Ketoesters via C–H Activation and C–C/C–N Bond Cleavage, Org. Lett., 2016, 18, 700 CrossRef CAS PubMed.
- Q. Zhou, X. Song, X. Zhang and X. Fan, Synthesis of 3-spirooxindole 3H-indoles through Rh(iii)-catalyzed [4 + 1] redox-neutral spirocyclization of N-aryl amidines with diazo oxindoles, Org. Chem. Front., 2021, 8, 4131 RSC.
- Q. Zhou, X. Song, X. Zhang and X. Fan, Synthesis of Spiro[benzo[d][1,3]oxazine-4,4′-isoquinoline]s via [4 + 1 + 1] Annulation of N-Aryl Amidines with Diazo Homophthalimides and O2, Org. Lett., 2022, 24, 1280 CrossRef CAS.
-
(a) Y. Li, J. Li, X. Wu, Y. Zhou and H. Liu, Rh(III)-Catalyzed C–H Cyclization of Arylnitrones with Diazo Compounds: Access to 3-Carboxylate Substituted N-Hydroxyindoles, J. Org. Chem., 2017, 82, 8984 CrossRef CAS PubMed;
(b) F. Fang, C. Zhang, C. Zhou, Y. Li, Y. Zhou and H. Liu, Rh(III)-Catalyzed C–H Activation of Benzoylacetonitriles and Tandem Cyclization with Diazo Compounds to Substituted Benzo[de]chromenes, Org. Lett., 2018, 20, 1720 CrossRef CAS PubMed;
(c) Z. Shu, J. Zhou, J. Li, Y. Cheng, H. Liu, D. Wang and Y. Zhou, Rh(III)-Catalyzed Dual C–H Functionalization/Cyclization Cascade by a Removable Directing Group: A Method for Synthesis of Polycyclic Fused Pyrano[de]Isochromenes, J. Org. Chem., 2020, 85, 12097 CrossRef CAS;
(d) F. Fang, S. Hu, C. Li, Q. Wang, R. Wang, X. Han, Y. Zhou and H. Liu, Catalytic System-Controlled Divergent Reaction Strategies for the Construction of Diversified Spiropyrazolone Skeletons from Pyrazolidinones and Diazopyrazolones, Angew. Chem., Int. Ed., 2021, 60, 21327 CrossRef CAS PubMed;
(e) Z. Jiang, J. Zhou, H. Zhu, H. Liu and Y. Zhou, Rh(III)-Catalyzed [5 + 1] Annulation of Indole-enaminones with Diazo Compounds To Form Highly Functionalized Carbazoles, Org. Lett., 2021, 23, 4406 CrossRef CAS.
-
(a)
Y. Zhou
, CCDC 2195446† for 3aa;
(b)
Y. Zhou
, CCDC 2195448† for 5sa.
Footnote |
† Electronic supplementary information (ESI) available: Experimental details and characterization data. CCDC 2195446 and 2195448. For ESI and crystallographic data in CIF or other electronic format see DOI: https://doi.org/10.1039/d2qo01529c |
|
This journal is © the Partner Organisations 2023 |
Click here to see how this site uses Cookies. View our privacy policy here.