DOI:
10.1039/D2QO01591A
(Research Article)
Org. Chem. Front., 2023,
10, 157-162
Visible-light mediated allylation of thiols with allylic alcohols†
Received
10th October 2022
, Accepted 12th November 2022
First published on 15th November 2022
Abstract
Herein, we report a mild metal-free visible-light-mediated allylation of thiols using an inexpensive photocatalyst (eosin Y). Photocatalytically generated sulfenyl radicals were coupled with allylic alcohols to obtain allylic sulfides in good to excellent yields. The reaction was found to be scalable and the products could be further functionalized. Furthermore, preliminary studies were carried out to gain insights into the reaction mechanism.
Over the past five decades, the unique reactivity of free radicals has allowed facile access to a variety of complex organic molecules from fairly simple precursors.1 Radical reactions being mild and selective have become a mainstay in synthetic chemistry for accessing value added chemicals like drugs, natural products, agrochemicals, polymers etc.2 Typically, radicals are generated by employing stoichiometric amounts of redox mediators or by photoexcitation with UV light. Consequently, these reactions suffer from a sustainability point of view, limiting their large-scale applications. As a result, the past decade has seen the emergence of visible-light photocatalysis as a superior and sustainable catalytic platform for generating radicals.3 These reactions rely on the use of photocatalysts which are neither oxidants nor reductants in the ground state, but upon photoexcitation by visible light, they become good single electron donors or acceptors. Based on the substrate, the photocatalyst in the excited state would act either as a single-electron acceptor or donor, thereby generating radical intermediates, avoiding the need for stochiometric initiators or UV irradiation. In addition, these reactions proceed under extremely mild conditions at ambient temperature, typically employing energy efficient LEDs as the energy source. All these advantages have made visible-light photocatalysis one of the greenest platforms to carry out radical reactions.4
On the other hand, allyl sulfides are one of the most celebrated classes of organosulfur compounds, both commercially and synthetically.5 They are found in the core of a variety of important molecules like drugs, natural products, agrochemicals and functional materials. In addition, they are also valuable building blocks for the synthesis of diverse classes of other organosulfur compounds.6 As a result, the synthesis of allyl sulfides bearing diverse substituents is of great interest. Efforts in this direction have led to the development of various protocols towards their syntheses like allylation of thiols,7 allylic substitution,8 allene/alkyne hydrothiolation9 and allylic C–H sulfenylation.10 However, these protocols suffer from several drawbacks, such as the requirement of harsh reaction conditions, rare/expensive transition metal catalysts, stoichiometric additives, and specialized pre-functionalized precursors, all of which compromise the sustainability of these protocols. As a result, the development of efficient methods towards allylic sulfides under mild conditions (room temperature) avoiding the need for stoichiometric additives using readily available organic catalysts would be of great interest.
The quest for efficient and green carbon–sulfur bond forming reactions has led to seminal reports by Yoon and Stephenson on visible-light-mediated thiol–ene reactions (Scheme 1a).11 These reactions proceed via the generation of sulfenyl radicals by single electron oxidation of thiols by a photocatalyst followed by their addition to alkenes. These methods marked a renaissance in green protocols towards visible-light mediated synthesis of organosulfur compounds using thiols.12,13 In spite of all these elegant reports, to the best of our knowledge, visible-light-mediated organocatalyzed allylation of thiols to furnish allylic sulfides remains unreported. Our group has had a long-standing interest in radical chemistry,14 especially visible-light photocatalysis.15 We had previously reported a visible-light-mediated sulfenylative cascade cyclization of alkyne-tethered cyclohexadienones using thiols (Scheme 1b).15a In continuation of our interest, herein we report a visible-light-mediated protocol towards allylic sulfides by the reaction of thiols with diaryl allylic alcohols (Scheme 1c). The reaction delivers allylic sulfides via the addition of photocatalytically generated sulfenyl radicals to allylic alcohols. The desired allyl sulfides were obtained in good to excellent yields at room temperature using an inexpensive and non-toxic photocatalyst, eosin Y.
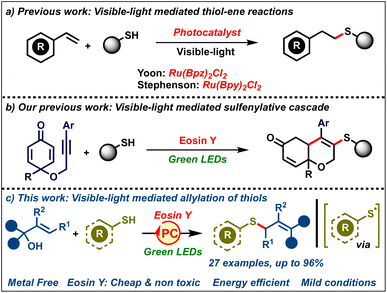 |
| Scheme 1 Overview of the work. | |
We began our preliminary investigations with the reaction of diphenyl allylic alcohol 1a and 1.5 equiv. of 4-chlorothiophenol 2a in the presence of 5 mol% of rhodamine B in DMF under irradiation with green LEDs. To our delight, the desired allylic sulfide 3a was obtained in 26% yield (Table 1, entry 1). Motivated by this, we carried out extensive screening of other photocatalysts and solvents for improving the yield of 3a. Among various organic as well as metal-based-photocatalysts, eosin Y was found to be the most optimal, delivering 40% of 3a (entries 2–6). The reaction displayed a high solvent dependency and acetonitrile was found to be the best solvent, providing 86% of 3a. The absence of either the photocatalyst or the light source proved deleterious as the reactions failed to deliver 3a in both the cases (entries 11 and 12). Upon switching the light source from green LEDs to blue or white LEDs, reductions in the yield of 3a to 80% and 47%, respectively, were observed (entries 13 and 14). Carrying out the reaction under an air atmosphere led to a slight increase in yield and the following were identified as the optimized reaction conditions, i.e. 1 equiv. of 1a, 1.5 equiv. of 2a, and 5 mol% of eosin Y in acetonitrile under an air atmosphere upon irradiation with green LEDs to obtain 3a in 89% (isolated yield of 87%).
Table 1 Reaction optimizationa
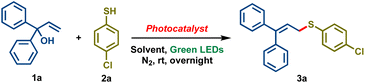
|
No. |
Photocatalyst (5 mol%) |
Solvent |
Yieldb (%) |
Reaction conditions: 1a (0.2 mmol), 2a (0.3 mmol), photocatalyst (5 mol%), solvent (2 mL) at room temperature for 12 h under the irradiation of green LEDs under nitrogen atmosphere.
NMR yields using 1,2,3-trimethoxybenzene as the internal standard.
Isolated yield.
Reaction in the dark.
Reaction under blue LED irradiation.
Reaction under white LED irradiation.
Reaction under air atmosphere.
|
1 |
Rhodamine B |
DMF |
26 |
2 |
Eosin Y |
DMF |
40 |
3 |
Eosin B |
DMF |
14 |
4 |
Rose Bengal |
DMF |
36 |
5 |
Fluorescein |
DMF |
Traces |
6 |
Ru(bpy)3Cl2 |
DMF |
37 |
7 |
Eosin Y |
DMSO |
27 |
8 |
Eosin Y |
DCM |
72 |
9 |
Eosin Y |
MeOH |
35 |
10 |
Eosin Y |
MeCN |
86 (83)c |
11 |
— |
MeCN |
Traces |
12d |
Eosin Y |
MeCN |
Traces |
13e |
Eosin Y |
MeCN |
80 |
14f |
Eosin Y |
MeCN |
47 |
15g
|
Eosin Y
|
MeCN
|
89 (87)c
|
With these optimized conditions in hand, our attention was then turned towards broadening the scope of the developed protocol, and various aromatic as well as aliphatic thiols 2a-p were screened (Table 2). Benzene thiol 2b reacted smoothly under the reaction conditions to furnish the desired allylic sulfide 3b in 88% yield. Thiophenols bearing para-substituents such as p-tolyl 2c, p-bromo 2d and p-fluoro gave the corresponding sulfides in 87%, 79% and 83% yields, respectively. m-Fluorothiophenol also furnished the corresponding allylic sulfide 3f in 83% yield. Thiophenols bearing o-substituents such as 2-MeO, 2-Me and 2-COOMe reacted smoothly to deliver the corresponding products 3g–3i in 82%, 89% and 81%, respectively. Electron-poor 2-mercaptopyridine led to 3j in a lower yield of 42%. Pleasingly, aliphatic thiols like benzyl thiol 2k, n-dodecane thiol 2l, cyclohexanethiol 2m, and t-butyl thiol 2n gave the corresponding sulfide products 3k–3n in good to excellent yields (75–96%). To our delight, biologically relevant thiols like methyl thioglycolate 2o and methyl ester of cysteine 2p delivered the corresponding allylic sulfide derivatives 3o and 3p in excellent yields. Interestingly, the reaction of 1a with thioacetic acid 2q afforded the thiol–ene addition product 4 in 98% yield and the allylic sulfide was not observed.
Table 2 Scope of thiolsa,b
Isolated yields.
Conditions: 1a (0.2 mmol), 2 (0.3 mmol), eosin Y (5 mol%), MeCN (2 mL), green LEDs, air.
|
|
In order to expand the scope of the transformation further, we probed the reactivity of different allylic alcohols with thiol 2a (Table 3). A variety of 4,4′-disubstituted diaryl allylic alcohols were found to be compatible, delivering good to excellent yields of the corresponding allylic sulfides 3q–3u. Allylic alcohols bearing two different aryl rings such as 1v and 1w gave good yields of the desired products 3v and 3walbeit as a 1
:
1 mixture of E
:
Z isomers. The reaction also tolerated 3-phenyl substituted allylic alcohol (cinnamyl alcohol derivative) 1x furnishing the desired product 3x in 86% yield. Allylic alcohol bearing a methyl substituent on olefin (iso-propylidene) 1y led to the desired tetra-substituted allylic sulfide derivative 3y in 84% yield. 1,3-Diphenyl allylic alcohol 1z and aryl methyl substituted allylic alcohol 1aa gave marginally lower yields of the desired sulfides 3z and 3aa, 63% and 42% respectively.
Table 3 Scope of allylic alcoholsa,b
Isolated yields.
Conditions: 1 (0.2 mmol), 2a (0.3 mmol), eosin Y (5 mol%), MeCN (2 mL), green LEDs, air.
|
|
Furthermore, the reaction was found to be scalable as 1.0 g of 1a and 1.03 g of 2a under the standard conditions delivered 1.4 g of 3a in 62% yield (Scheme 2a). Sulfide 3a was oxidized with m-CPBA to either the corresponding sulfoxide 5 (94%) or sulfone 6 (60%) by controlling the amount of oxidant (Scheme 2b). We also found that treating 3a with TBAB and PIDA led to the bis-acetoxylated derivative 7 in 54% yield. Interestingly, the Pummerer fragmentation product 9 was isolated in 58% yield upon reacting sulfoxide 5 with β-naphthol 8 and trifluoroacetic anhydride. Subsequently, our attention was turned towards the mechanistic investigation of the established protocol. When the reaction was carried out in the presence of radical scavengers like TEMPO and BHT, no allylic sulfide 3a was detected, indicating that the reaction might be proceeding via a radical intermediate (Scheme 2c). Upon carrying out the reaction in the presence of CuCl2, a singlet electron quencher, a drastic reduction in the reaction yield was observed (25% vs. 87%), indicating that the reaction might proceed via single electron transfer. In addition, when the reaction was carried out in the presence of a catalase (a reactive oxygen species quencher), 3a was obtained in 80% yield, indicating that reactive oxygen species might not play a significant role in our reaction. In a control experiment, when the reaction was performed with disulfide 10, 3a was isolated in 58% yield, hinting that disulfide might be an intermediate in our reaction (Scheme 2d).
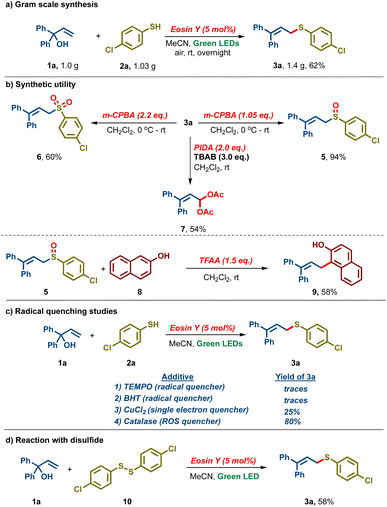 |
| Scheme 2 Gram scale synthesis and functionalization. | |
To further probe the reaction mechanism, fluorescence quenching studies were carried out. We observed that the fluorescence emission intensity of eosin Y upon excitation at 500 nm was drastically quenched with increasing concentrations of thiophenol 2b (see Fig. 1 and 2). On the other hand, no significant quenching was observed in the case of allylic alcohol 1a (see the ESI†). This suggested that an electron transfer process exist between the exited state of eosin Y and thiol, while no such process occurs with allylic alcohol.
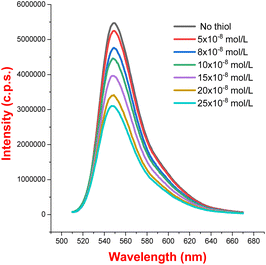 |
| Fig. 1 Fluorescence quenching of eosin Y with thiophenol. | |
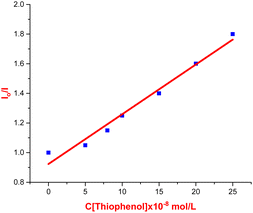 |
| Fig. 2 Stern–Volmer plot. | |
We propose the following mechanism in accordance with the previous literature and mechanistic studies (Scheme 3). Eosin Y on visible light irradiation undergoes photoexcitation forming eosin Y*, which subsequently undergoes reductive quenching by thiol 2via single electron transfer forming the eosin Y radical anion and the sulfenyl radical cation A. The key sulfenyl radical B is formed subsequently via deprotonation, which adds onto the olefin of allylic alcohol resulting in the radical intermediate C. This intermediate abstracts a hydrogen radical from thiol 2, forming the thiol–ene addition product D and sulfenyl radical B resulting in chain propagation. Intermediate D undergoes spontaneous water elimination furnishing allylic sulfide 3.
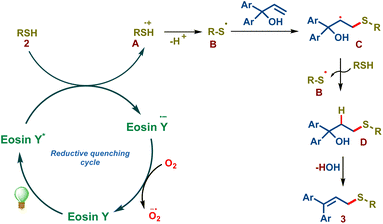 |
| Scheme 3 Plausible mechanism. | |
In conclusion, we have developed a mild metal- and additive-free protocol towards the synthesis of allylic sulfides via a visible-light-mediated sulfenylative condensation of thiols with diaryl allylic alcohols in excellent yields. The reaction proceeds at room temperature by employing the non-toxic, inexpensive and commercially available photocatalyst, eosin Y. The synthetic utility of the reaction was highlighted by carrying out gram-scale synthesis and further functionalization. Furthermore, control studies were carried out to get insights into the reaction mechanism.
Conflicts of interest
There are no conflicts to declare.
Acknowledgements
This activity is supported by the Science and Engineering Research Board (SERB), India (CRG/2019/005059). S. K. would like to thank UGC for a fellowship. The authors are also grateful to DST-FIST (SR/FST/CS-II/2017/37).
References
-
(a) M. Yan, J. C. Lo, J. T. Edwards and P. S. Baran, Radicals: Reactive Intermediates with Translational Potential, J. Am. Chem. Soc., 2016, 138, 12692 CrossRef CAS;
(b) D. P. Curran and D. M. Rakiewicz, Tandem radical approach to linear condensed cyclopentanoids. Total synthesis of (.+-.)-hirsutene, J. Am. Chem. Soc., 1985, 107, 1448 CrossRef CAS;
(c) D. P. Curran and D. M. Rakiewicz, Radical-initiated polyolefinic cyclizations in linear triquinane synthesis. model studies and total synthesis of (±)-hirsutene, Tetrahedron, 1985, 41, 3943 CrossRef CAS.
-
(a) M. D. Karkas, C. R. J. Stephenson and J. A. Porco Jr., Photochemical Approaches to Complex Chemotypes: Applications in Natural Product Synthesis, Chem. Rev., 2016, 116, 9683 CrossRef CAS;
(b) N. Hoffmann, Photochemical Reactions as Key Steps in Organic Synthesis, Chem. Rev., 2008, 108, 1052 CrossRef CAS PubMed.
-
(a) C. K. Prier, D. A. Rankic and D. W. C. MacMillan, Visible Light Photoredox Catalysis with Transition Metal Complexes: Applications in Organic Synthesis, Chem. Rev., 2013, 113, 5322 CrossRef CAS;
(b) D. C. Fabrey and M. Rueping, Merging Visible Light Photoredox Catalysis with Metal Catalyzed C–H Activations: On the Role of Oxygen and Superoxide Ions as Oxidants, Acc. Chem. Res., 2016, 49, 1969 CrossRef PubMed;
(c) I. Ghosh, L. Marzo, A. Das, R. Shaikh and B. König, Visible Light Mediated Photoredox Catalytic Arylation Reactions, Acc. Chem. Res., 2016, 49, 1566 CrossRef CAS PubMed;
(d) T. P. Yoon, M. A. Ischay and J. Du, Visible light photocatalysis as a greener approach to photochemical synthesis, Nat. Chem., 2010, 2, 527 CrossRef CAS PubMed;
(e) J. M. R. Narayanam and C. R. J. Stephenson, Visible light photoredox catalysis: applications in organic synthesis, Chem. Soc. Rev., 2011, 40, 102 RSC.
- G. E. M. Crisenza and P. Melchiorre, Chemistry glows green with photoredox catalysis, Nat. Commun., 2020, 11, 803 CrossRef.
-
(a) M. Ahmed, A. E. I. Doaa, Y. E. Rasha and M. A. A. Hayam, Design and Synthesis of Novel Thioethers Derived from 1,5-Diphenyl-6-thioxo-6,7-dihydro-1H-pyrazolo[3,4-d]pyrimidin-4(5H)-ones as Antiangiogenic Agents, Lett. Drug Des. Discovery, 2019, 16, 200 Search PubMed;
(b) P. S. S. Rao, M. M. Narasimha, D. M. Duane, C. Subhash, K. Anil and K. Santosh, Diallyl Sulfide: Potential Use in Novel Therapeutic Interventions in Alcohol, Drugs, and Disease Mediated Cellular Toxicity by Targeting Cytochrome P450 2E1, Curr. Drug Metab., 2015, 16, 486 CrossRef CAS;
(c) M. J. Wargovich, Diallylsulfide and Allylmethylsulfide Are Uniquely Effective among Organosulfur Compounds in Inhibiting CYP2E1 Protein in Animal Models, J. Nutr., 2006, 136, 832S CrossRef CAS;
(d) A. Arunkumar, M. R. Vijayababu, P. Venkataraman, K. Senthilkumar and J. Arunakaran, Chemoprevention of Rat Prostate Carcinogenesis by Diallyl Disulfide, an Organosulfur Compound of Garlic, Biol. Pharm. Bull., 2006, 29, 375 CrossRef CAS PubMed.
-
(a) D. Chen, G. Xing, J. Yao and H. Zhou, Applicable β-sulfonium carbanions: facile construction of thiophene derivatives, Org. Chem. Front., 2017, 4, 1042 RSC;
(b) Y. A. Lin, J. M. Chalker, N. Floyd, G. J. L. Bernardes and B. G. Davis, Allyl Sulfides Are Privileged Substrates in Aqueous Cross-Metathesis: Application to Site-Selective Protein Modification, J. Am. Chem. Soc., 2008, 130, 9642 CrossRef CAS;
(c) S. Barluenga, P. Lopez, E. Moulin and N. Winssinger, Modular Asymmetric Synthesis of Pochonin C, Angew. Chem., Int. Ed., 2004, 43, 3467 CrossRef CAS.
-
(a) L. Herkert, S. L. J. Green, G. Barker, D. G. Johnson, P. C. Young, S. A. Macgregor and A.-L. Lee, Gold(I)-Catalysed Direct Thioetherifications Using Allylic Alcohols: an Experimental and Computational Study, Chem. – Eur. J., 2014, 20, 11540 CrossRef CAS;
(b) Y. Yatsumonji, Y. Ishida, A. Tsubouchi and T. Takeda, Nickel(0) Triethyl Phosphite Complex-Catalyzed,
Allylic Substitution with Retention of Regio- and Stereochemistry, Org. Lett., 2007, 9, 4603 CrossRef CAS;
(c) T. Kondo, Y. Morisaki, S.-Y. Uenoyama, K. Wada and T.-A. Mitsudo, Ruthenium-Catalyzed S-Propargylation of Thiols Enables the Rapid Synthesis of Propargylic Sulfides, J. Am. Chem. Soc., 1999, 121, 8657 CrossRef CAS;
(d) J. E. Gómez, W. Guo and A. W. Kleij, Palladium-Catalyzed Stereoselective Formation of Substituted Allylic Thioethers and Sulfones, Org. Lett., 2016, 18, 6042 CrossRef;
(e) G. Guo, Y. Yuan, S. Wan, X. Cao, Y. Sun and C. Huo, K2S2O8 promoted dehydrative cross-coupling between α,α-disubstituted allylic alcohols and thiophenols/thiols, Org. Chem. Front., 2021, 8, 2990 RSC.
-
(a) M. Roggen and E. M. Carreira, Enantioselective Allylic Thioetherification: The Effect of Phosphoric Acid Diester on Iridium-Catalyzed Enantioconvergent Transformations, Angew. Chem., Int. Ed., 2012, 51, 8652 CrossRef PubMed;
(b) N. Gao, S. Zheng, W. Yang and X. Zhao, Carbon−Sulfur Bond Formation via Iridium-Catalyzed Asymmetric Allylation of Aliphatic Thiols, Org. Lett., 2011, 13, 1514 CrossRef CAS;
(c) S. Zheng, N. Gao, W. Liu, D. Liu, X. Zhao and T. Cohen, Regio- and Enantioselective Iridium-Catalyzed Allylation of Thiophenol: Synthesis of Enantiopure Allyl Phenyl Sulfides, Org. Lett., 2010, 12, 4454 CrossRef CAS PubMed;
(d) S. Tanaka, P. K. Pradhan, Y. Maegawa and M. Kitamura, Highly efficient catalytic dehydrative S-allylation of thiols and thioic S-acids, Chem. Commun., 2010, 46, 3996 RSC.
-
(a) X.-H. Yang, R. T. Davison, S.-Z. Nie, F. A. Cruz, T. M. McGinnis and V. M. Dong, Catalytic Hydrothiolation: Counterion-Controlled Regioselectivity, J. Am. Chem. Soc., 2019, 141, 3006 CrossRef CAS;
(b) X.-H. Yang, R. T. Davison and V. M. Dong, Catalytic Hydrothiolation: Regio- and Enantioselective Coupling of Thiols and Dienes, J. Am. Chem. Soc., 2018, 140, 10443 CrossRef CAS;
(c) A. B. Pritzius and B. Breit,
Z-Selective Hydrothiolation of Racemic 1,3-Disubstituted Allenes: An Atom-Economic Rhodium-Catalyzed Dynamic Kinetic Resolution, Angew. Chem., Int. Ed., 2015, 54, 15818 CrossRef CAS PubMed;
(d) A. B. Pritzius and B. Breit, Asymmetric Rhodium-Catalyzed Addition of Thiols to Allenes: Synthesis of Branched Allylic Thioethers and Sulfones, Angew. Chem., Int. Ed., 2015, 54, 3121 CrossRef CAS PubMed.
- J. Kim, B. Kang and S. H. Hong, Direct Allylic C(sp3)–H Thiolation with Disulfides via Visible Light Photoredox Catalysis, ACS Catal., 2020, 10, 6013 CrossRef CAS.
-
(a) E. L. Tyson, Z. L. Niemeyer and T. P. Yoon, Redox Mediators in Visible Light Photocatalysis: Photocatalytic Radical Thiol–Ene Additions, J. Org. Chem., 2014, 79, 1427 CrossRef CAS PubMed;
(b) E. L. Tyson, M. S. Ament and T. P. Yoon, Transition Metal Photoredox Catalysis of Radical Thiol-Ene Reactions, J. Org. Chem., 2013, 78, 2046 CrossRef CAS PubMed;
(c) M. H. Keylor, J. E. Park, C. J. Wallentin and C. R. J. Stephenson, Photocatalytic initiation of thiol–ene reactions: synthesis of thiomorpholin-3-ones, Tetrahedron, 2014, 70, 4264 CrossRef CAS.
-
(a) A. Wimmer and B. König, Photocatalytic formation of carbon–sulfur bonds, Beilstein J. Org. Chem., 2018, 14, 54 CrossRef CAS PubMed;
(b) Y. Wang, Y. Li and X. Jiang, Sulfur-Center-Involved Photocatalyzed Reactions, Chem. – Asian J., 2018, 13, 2208 CrossRef CAS PubMed;
(c) W. Guo, K. Tao, W. Tan, M. Zhao, L. Zheng and X. Fan, Recent advances in photocatalytic C–S/P–S bond formation via the generation of sulfur centered radicals and functionalization, Org. Chem. Front., 2019, 6, 2048 RSC.
-
(a) H. Cui, W. Wei, D. Yang, Y. Zhang, H. Zhao, L. Wang and H. Wang, Visible-light-induced selective synthesis of sulfoxides from alkenes and thiols using air as the oxidant, Green Chem., 2017, 19, 3520 RSC;
(b) S. S. Zalesskiy, N. S. Shlapakov and V. P. Ananikov, Visible light mediated metal-free thiol–yne click reaction, Chem. Sci., 2016, 7, 6740 RSC;
(c) Y. Li, M. Wang and X. Jiang, Controllable Sulfoxidation and Sulfenylation with Organic Thiosulfate Salts via Dual Electron- and Energy-Transfer Photocatalysis, ACS Catal., 2017, 7, 7587 CrossRef CAS;
(d) Q. Liu, L. Wang, H. Yue, J.-S. Li, Z. Loud and W. Wei, Catalyst-free visible-light-initiated oxidative coupling of aryldiazo sulfones with thiols leading to unsymmetrical sulfoxides in air, Green Chem., 2019, 21, 1609 RSC;
(e) J.-G. Sun, H. Yang, P. Li and B. Zhang, Metal-Free Visible-Light-Mediated Oxidative Cross-Coupling of Thiols with P(O)H Compounds Using Air as the Oxidant, Org. Lett., 2016, 18, 5114 CrossRef CAS PubMed;
(f) W. Wei, H. Cui, D. Yang, Y. Zhang, H. Yue, C. He, Y. Zhang and H. Wang, Visible-light-enabled spirocyclization of alkynes leading to 3-sulfonyl and 3-sulfenyl azaspiro[4,5]trienones, Green Chem., 2017, 19, 5608 RSC;
(g) W. Wei, P. Bao, H. Yue, S. Liu, L. Wang, Y. Li and D. Yang, Visible-Light-Enabled Construction of Thiocarbamates from Isocyanides, Thiols, and Water at Room Temperature, Org. Lett., 2018, 20, 5291 CrossRef CAS PubMed;
(h) L.-Y. Xie, Y.-L. Chen, L. Qin, Y. Wen, J.-W. Xie, J.-X. Tan, Y. Huang, Z. Cao and W.-M. He, Visible-light-promoted direct C-H/S-H cross coupling of quinoxalin-2(1H)-ones with thiols leading to 3-sulfenylated quinoxalin-2(1H)-ones in air, Org. Chem. Front., 2019, 6, 3950 RSC;
(i) D.-S. Yang, Q.-L. Yan, E.-J. Zhu, J. Lv and W.-M. He, Carbon–sulfur bond formation via photochemical strategies: An efficient method for the synthesis of sulfur-containing compounds, Chin. Chem. Lett., 2021, 33, 1798 CrossRef;
(j) Z. Gan, G. Li, X. Yang, Q. Yan, G. Xu, G. Li, Y.-Y. Jiang and D.-S. Yang, Visible-light-induced regioselective cross-dehydrogenative coupling of 2-isothiocyanatonaphthalenes with amines using molecular oxygen, Sci. China: Chem., 2020, 63, 1652 CrossRef CAS.
-
(a) A. M. Nair, A. H. Shinde, S. Kumar and C. M. R. Volla, Metal-free spirocyclization of N-arylpropiolamides with glyoxylic acids: access to complex azaspiro-fused tricycles, Chem. Commun., 2020, 56, 12367 RSC;
(b) A. M. Nair, I. Halder, S. Khan and C. M. R. Volla, Metal Free Sulfonylative Spirocyclization of Alkenyl and Alkynyl Amides via Insertion of Sulfur Dioxide, Adv. Synth. Catal., 2020, 362, 224 CrossRef CAS;
(c) A. M. Nair, I. Halder and C. M. R. Volla, A metal-free four-component sulfonylation, Giese cyclization, selenylation cascade via insertion of sulfur dioxide, Chem. Commun., 2022, 58, 6950 RSC;
(d) N. Thrimurtulu, A. Dey, K. Pal, A. Nair, S. Kumar and C. M. R. Volla, Metal–Free Remote C–H Bond Acetoxylation of 8−Aminoquinolines on the C5−Position, ChemistrySelect, 2017, 2, 7251 CrossRef CAS.
-
(a) A. M. Nair, S. Kumar and C. M. R. Volla, Visible Light Mediated Sulfenylation-Annulation Cascade of Alkyne Tethered Cyclohexadienones, Adv. Synth. Catal., 2019, 361, 4983 CrossRef CAS;
(b) A. M. Nair, S. Kumar, I. Halder and C. M. R. Volla, Visible-light mediated sulfonylation of thiols via insertion of sulfur dioxide, Org. Biomol. Chem., 2019, 17, 5897 RSC;
(c) R. Rahaman, A. M. Nair and C. M. R. Volla, Visible-Light Mediated Arbuzov-Like Reaction with Thiophenols, Chem. – Eur. J., 2022, 28, e202201290 CrossRef CAS;
(d) S. Kumar, A. M. Nair and C. M. R. Volla, Dual Photoredox Cobalt Catalyzed [4+1] Annulation and C−H Alkoxylation, Chem. – Asian J., 2022, e202200801 CAS.
|
This journal is © the Partner Organisations 2023 |
Click here to see how this site uses Cookies. View our privacy policy here.