DOI:
10.1039/D2QO01938H
(Research Article)
Org. Chem. Front., 2023,
10, 970-976
A metal-free protocol for the preparation of amines using ammonia borane under mild conditions†
Received
7th December 2022
, Accepted 29th December 2022
First published on 29th December 2022
Abstract
A mild and convenient reductive amination protocol for the synthesis of structurally diverse secondary and tertiary amines as well as N-methylated products under metal-free conditions is presented. This one-pot protocol works efficiently at room temperature using ammonia borane as the reductant and is applicable to a wide range of substrates, including chiral amines and amino acid derivatives. The synthetic utility and practicability of this methodology is demonstrated by gram-scale reactions and selected synthesis of current drug molecules.
Introduction
Metal-catalyzed reactions have become one of the most powerful and straightforward methods for the synthesis of organic molecules.1–4 However, the involvement of metals in organic reactions also raises many issues. For example, noble metals, such as Ru, Rh, Pd, Ir, are effective catalysts for a variety of organic reactions, but are expensive and often comparably toxic.5,6 For many applications, especially in the production of pharmaceuticals, the removal of metal is a crucial issue, which requires additional efforts and costs.7,8 In case of more abundant metals such as Fe, Co, Ni, Cu, Zn, frequently sophisticated ligands are commonly required to achieve the desired reactivity.9,10 Notably, in such cases the ligands can be more expensive than the metals themselves. In addition, some metal–ligand complexes are sensitive to moisture and air, which causes operational difficulty and safety issues.11–14 To address these problems, the development of metal-free reactions is interesting.
Amines represent a privileged class of compounds in organic chemistry, that is used extensively in fine chemicals, pharmaceuticals, agrochemicals and materials.15–18 More specifically, the majority (80%) of the 200 top selling small molecule drugs of 2021 contained amine and/or nitrogen moieties.19 Regarding their synthesis and functionalization, direct reductive amination is still one of the most used and convenient protocols to access different kinds of amines.20–24 The general reaction pathway for reductive aminations is first to generate an imine intermediate in situ through condensation of carbonyl compounds with amines, and subsequent reduction to produce the corresponding amines.25–28 In general, this latter reduction step is performed in the presence of metal catalysts under (high) pressure of H2. Transfer hydrogenation offers an alternative pathway that avoids the use of pressurized gas or the need of specialized equipment.29,30 In this respect, great efforts have been made using hydrosilanes,31–36 borohydrides37,38 or formic acid and derivatives39–42 as the hydrogen source for reductive amination reactions. Although these procedures offer many benefits and show interesting substrate scope, there remain challenges such as the use of water-soluble substrates, highly functionalized compounds, metal contamination of products and so on. In addition, ammonia borane is considered as a promising material for hydrogen transfer. The low molecular weight (30.87 g mol−1), high hydrogen content (19.6 wt%), and ease of handling as a bench-stable crystalline solid makes ammonia borane a particularly attractive reagent.43–45 Thus, in the last decades ammonia borane has been employed as a reducing agent for C
O, C
N and C
C bonds, but only a few reports are known for reductive amination reactions.46–48 Ramachandran group49 and Hu group50 described reductive amination procedure employing boron amine complex as a reductant. However, a sub-stoichiometric promoter or heating conditions are necessary to obtain amine product in good yields. In addition, the substrates of chiral drug molecules or biologically relevant amino acid derivatives are not involved in these protocols. Despite all these elegant works, the development of a mild and general reductive amination procedure remains desirable, specifically for more demanding substrates such as amino acid derivatives, chiral amines or advanced substrates with sensitive functional groups, e.g. nitro, olefin, iodo, etc.
For some time, our group is interested to improve existing catalytic hydrogenation and reductive amination reactions.16,26,51,52 Based on our previous works, we recently became interested in exploring the use of ammonia borane for various hydrogenation reactions. Herein, we report a general reductive amination of aldehydes with primary and secondary amines using ammonia borane as a reductant (Scheme 1). This protocol could be performed at room temperature without using any metal catalyst, inert atmosphere, dry solvent, base, and water scavenger. The mild reaction conditions, broad substrate scope, high product yield, simple operation and workup and ease of scale-up constitute the key features of the protocol and makes it attractive for many potential applications in organic synthesis and life sciences.
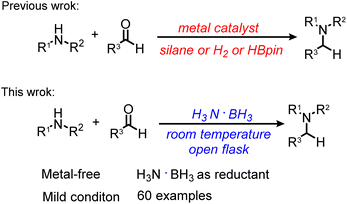 |
| Scheme 1 Reductive amination of aldehydes with amines. | |
Results and discussion
Reaction design and optimization
As a starting point of our present investigation, we have chosen reductive amination of benzaldehyde 1 and aniline 2 as a benchmark reaction to explore the influence of critical reaction parameters. In the absence of any reducing agent, the model reaction provided a 93% conversion to imine 4 but no desired amine 3 was formed (Table 1, entry 1). Testing different reducing agents, such as HCOOH, HCOONa, Et3SiH, Ph2SiH2, and N2H4·H2O gave poor results without providing any desired amine product (Table 1, entries 2–6). Next, we tested several borohydrides reducing agents (Table 1, entries 7–11) and to our delight, a 72% yield of 3 was obtained while using H3N·BH3 (Table 1, entry 9). To improve the product yield, the influence of various organic solvents was examined. The model reaction only provided moderate product yield of 3 in EtOH, i-PrOH, THF, CH3CN, toluene, or (CF3)2CHOH (HFIP) as solvent (Table 1, entries 12–17). Surprisingly, using trifluoroethanol (TFE) as a solvent, an 84% yield of 3 could be reached (Table 1, entry 18). Even under open flask conditions the reaction still gave a yield of 83% (Table 1, entry 19). Interestingly, a 73% yield of 3 was observed using water as a sole solvent, showing that this reaction protocol is not sensitive to water (Table 1, entry 20). While mixing TFE and water, the product yield increased to 79% (Table 1, entry 21). Notably, it is possible to run the reaction also under solvent-free conditions, which can be advantageous for certain applications (Table 1, entry 22). Finally, the best product yield (86%) was obtained in TFE following a stepwise reductive amination procedure (Table 1, entry 23). It is worth mentioning that neither an inert atmosphere nor carefully dried solvents are necessary for this protocol.
Table 1 Reductive amination of benzaldehyde with aniline: optimization of the reaction conditions

|
Entrya |
Reducing agent |
Solvent |
Conv. (%) |
Yield (%) |
3
|
4
|
Reaction conditions: reactions were conducted in the closed pressure tube. Benzaldehyde (0.6 mmol), aniline (0.5 mmol), reducing agent (0.75 mmol), solvent (2 mL), 25 °C, 4 h.
Reactions were conducted in open flask condition.
Stepwise reductive amination procedure: benzaldehyde (0.6 mmol) reacted with aniline (0.5 mmol) for 3 h, then H3N·BH3 (0.75 mmol) was added and continued the reaction for another 1 h. Conversions and yields were determined based aniline by GC using mesitylene as a standard.
|
1 |
–– |
MeOH |
93 |
0 |
93 |
2 |
HCOOH |
MeOH |
75 |
0 |
75 |
3 |
HCOONa |
MeOH |
72 |
0 |
72 |
4 |
Et3SiH |
MeOH |
90 |
0 |
90 |
5 |
Ph2SiH2 |
MeOH |
70 |
0 |
70 |
6 |
N2H4·H2O |
MeOH |
48 |
0 |
48 |
7 |
NaHB4 |
MeOH |
58 |
52 |
6 |
8 |
BH3·THF |
MeOH |
94 |
58 |
36 |
9 |
H3N·BH3 |
MeOH |
76 |
72 |
4 |
10 |
(CH3)2NH·BH3 |
MeOH |
53 |
49 |
4 |
11 |
(CH3)3N·BH3 |
MeOH |
95 |
55 |
40 |
12 |
H3N·BH3 |
EtOH |
48 |
46 |
2 |
13 |
H3N·BH3 |
i-PrOH |
52 |
50 |
2 |
14 |
H3N·BH3 |
THF |
58 |
54 |
4 |
15 |
H3N·BH3 |
CH3CN |
48 |
45 |
3 |
16 |
H3N·BH3 |
Toluene |
82 |
47 |
35 |
17 |
H3N·BH3 |
HFLP |
72 |
69 |
3 |
18 |
H3N·BH3 |
TFE |
86 |
84 |
2 |
19b |
H3N·BH3 |
TFE |
87 |
83 |
4 |
20b |
H3N·BH3 |
H2O |
78 |
73 |
5 |
21b |
H3N·BH3 |
TFE/H2O |
81 |
79 |
2 |
22b |
H3N·BH3 |
–– |
75 |
72 |
3 |
23b,c |
H3N·BH3 |
TFE |
88 |
86 |
2 |
To understand the reasons for the high specificity of the ammonia borane and the mechanism of this novel reductive amination protocol, a kinetic experiment for the model reaction of benzaldehyde and aniline was carried out. As shown in Scheme 2, in the first 15 min, aniline 2 is expeditiously consumed and converted mainly to N-benzylaniline 3. In addition, a small amount of N-benzylideneanilinea 4 is formed. Within the next 4 hours, 2 and 4 are continuously reduced and converted to 3. Further control experiments are shown in Scheme S1.† As expected, in the absence of ammonia borane, 1 reacted with 2 to produce 4 in 84% yield in 4 h. Subsequently, adding ammonia borane to the reaction mixture, 4 was fully converted to 3 in 0.5 h. Thus, the reaction profile and control experiments support that the progress of the reaction via an imine intermediate and further reduction to the desired amine using ammonia borane. Furthermore, the 11B NMR analyses (∼22.4/21.3 ppm) indicated the formation of polyaminoborane in the reaction mixtures (Scheme S2†). The main side reaction in this protocol is the reduction of aldehyde. If no amine is added to the reaction mixture, the aldehyde will be reduced to the corresponding alcohol. Therefore, 1.2 equivalents of aldehyde were used to ensure an efficient reductive amination reaction. Apparently, TFE (pKa = 12.4) can promote the decomposition of ammonia borane to form a transient and highly reactive aminoborane (H2B=NH2), which can effectively reduce the imine intermediate formed from the aldehyde and amine to give the desired secondary amine.
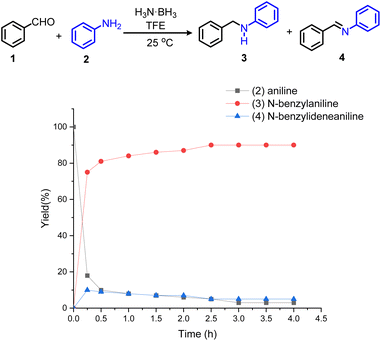 |
| Scheme 2 Time-dependent profile for the reduction amination reaction of benzaldehyde with aniline. Reaction conditions: benzaldehyde (0.6 mmol), aniline (0.5 mmol), ammonia borane (0.75 mmol), TFE (2 mL), 25 °C, open flask, yield was determined based aniline by GC using mesitylene as an internal standard. | |
Reductive amination of aldehydes and amines
Having an optimal procedure in hand, we explored its general applicability for the synthesis of diverse secondary amines. First, the reactivity of various aryl aldehydes with aniline was investigated (Scheme 3). As expected, benzaldehydes with different electro-donating groups, such as methyl, ethyl, t-butyl, 1,3-dioxolanemethyl, reacted with aniline to provide the corresponding secondary amines in up to 91% yield (Scheme 3, 5–9). Notably, pentamethylbenzaldehyde as sterically hindered substrate also proceeded well to offer a 65% yield of 10. Furthermore, aldehydes bearing halide substituents (F, Cl, Br, I) provided the corresponding products in high yields (85–91%, Scheme 3, 11–15). Aryl aldehydes with other sensitive functional groups such as nitro or carboxyl provided the corresponding reductive amination products in 75% and 68% yield, respectively (Scheme 3, 16 and 17). This protocol can be easily applied to heteroaryl aldehydes. Hence, a range of heteroaromatic aldehydes such as 2-pyridinecarboxaldehyde, 5-chloropicolinaldehyde, 2-thiophene-carboxaldehyde, and furfural afforded the target products in 71–92% yields (Scheme 3, 18–21). Notably, not only aromatic and heteroaromatic aldehydes, but also aliphatic aldehydes, which are less reactive and more prone to aldol side reactions, can be employed. As an example, under the same conditions, cyclic substrates reacted well with aniline and gave the corresponding products in up to 82% yield (Scheme 3, 22–25). Interestingly, even more challenging unsaturated aldehydes as well as chloral hydrate, which is used in the short-term treatment of insomnia, reacted smoothly with aniline to provide the corresponding products (Scheme 3, 26 and 27). The scope of amines was next explored, and methyl, methoxy, halide-substituted substrates provided the corresponding products in up to 85% yield (Scheme 3, 28–34). Likewise, benzylic amines reacted well to give the desired products in up to 90% yield (Scheme 2, 35–38). Similarly, racemic 1-phenylethylamine was tested with different aryl aldehydes and afforded 70–81% yields (Scheme 3, 39–42). From the viewpoint of chemoselectivity, the reaction of 4-iodobenzaldehyde with 1,2-diphenylethan-1-amine is interesting due to the high reactivity of the C–I bond in this substrate (Scheme 3, 43). Lastly, two examples of aliphatic amines were examined in the reaction, and both tert-butylamine and neopentylamine gave good results (Scheme 3, 44 and 45).
 |
| Scheme 3 Reductive amination reaction using ammonia borane as a reductant. Reaction conditions: aldehyde (0.6 mmol), amine (0.5 mmol), ammonia borane (0.75 mmol), TFE (2 mL), 25 °C, open flask, 24 h, isolated yields corresponding to aniline. | |
In comparison to the tested primary amines, we also tried various secondary amines, including piperidine, morpholine, and diphenylamine, but only trace amounts of products were observed by GC-MS. As this protocol works well at room temperature, the formation of the corresponding imine intermediates at this temperature seems to be more difficult.
Synthesis of N-methylamines
N-methylated amine derivatives play a vital role in regulating many biological and pharmaceutical properties of life-science molecules.18,53 Thus, we investigated the reductive N-methylation of amines using aqueous formaldehyde (37 wt% in water) in the presence of ammonia borane at room temperature. As shown in Scheme 4, phenyl and benzylic amines reacted well and provided the corresponding methylated products in moderate to good yields (Scheme 4, 46–53).
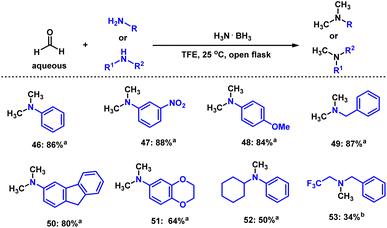 |
| Scheme 4 Reductive N-methylation of amines using aqueous formaldehyde. a Reaction conditions: amine (0.5 mmol), formaldehyde 37 wt% in water (2 mmol), ammonia borane (2 mmol), TFE (2 mL), 25 °C, open flask, 24 h, isolated yield corresponding to amine. b Yield was determined by GC-MS. | |
Reductive amination of chiral amines and amino acid derivatives
Chiral phenylethylamines are interesting intermediates for the synthesis of various drugs and biologically active compounds. So, we explored the scope of these chiral amines with a series of substituted aryl aldehydes. In the case of (S)-1-phenylethan-1-amine as substance, the desired transformation took place smoothly with various aldehydes and afforded both good product yields and ee values (Scheme 5, 54–56). As another example (S)-1-(naphthalen-1-yl)ethan-1-amine reacted with 4-bromobenzaldehyde to give the corresponding product in 70% yield and 98% ee (Scheme 5, 57). Notably, N-alkylated amino acid derivatives are widely distributed in nature, and such molecules are used in modern pharmaceuticals such as Cilazapril, Enalapril, as they trigger many biological processes. Compared with standard amines, the reductive alkylation of this class of compounds is more demanding for several reasons: (a) the reaction should be performed under mild conditions (ambient temperature, no strong base or acid) to avoid partial racemization of the inherent stereocenter(s); (b) as a reaction medium polar ideally aqueous media are desired for solubility reasons; and (c) the close presence of the carboxyl and amino groups constitutes a specific challenge with respect to chemoselectivity.54,55 To our delight, benzaldehyde and 4-fluorobenzaldehyde reacted with methyl L-phenylalaninate to provide the N-alkylated amino acid esters in 61–63% yield and 70–79% ee. (Scheme 4, 58 and 59). Thus, this benign reductive amination process shows feasibility for selective late-stage manipulation of amine-based life-science molecules.
 |
| Scheme 5 Reductive amination chiral amines and amino acid derivatives. Reaction conditions: amine (0.5 mmol), aldehyde (1.5 mmol), ammonia borane (2.0 mmol), TFE (3 mL), 25 °C, open flask, 24 h, isolated yield corresponding to amine. | |
Selected applications (synthesis of drugs, scale up) and current limitations
To demonstrate the practical utility of this protocol, reductive amination reactions on gram-scale were carried out using different substrates (Scheme 6). As expected, the selected substrates produced the corresponding amines in good yields, which proved that this methodology could be easily scaled up. To showcase further the applicability, the drug molecule amitriptyline was successfully methylated in good yields (Scheme 6, 62). Finally, (S)-1-(naphthalen-1-yl)ethan-1-amine provided the drug cinacalcet in 70% yield and 98% ee (Scheme 6, 64). This reaction system also has certain substrate limitations. The active group in aryl aldehyde, such as carbonyl, and cyano group, could be reduced in the reaction, resulting in complex products. As mentioned before, secondary amines were not active enough in the system to afford any products (Scheme S3†). We next performed a controlled experiment. The substrates of 4-fluorobenzaldehyde, 4-nitrophenylethanone, aniline, piperidine, benzamide, were mixed with ammonia borane in TFE at room temperature. After 12 hours, the protocol afforded 11 in 84% yield, and a 76% yield of 1-(4-nitrophenyl)ethanol was obtained from the reduction of 4-nitrophenylethanone (Scheme S4†). This result indicated that the present protocol is selective to the aldehyde functionality over the ketone functionality.
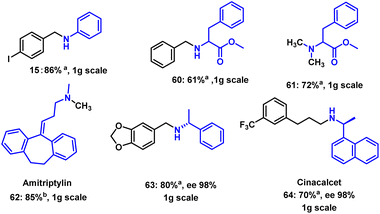 |
| Scheme 6 Reductive amination reactions on a gram-scale. a Reaction conditions: aryl aldehyde (6 mmol), amine (7.2 mmol), ammonia borane (9 mmol), TFE (20 mL), 25 °C, open flask, 24 h. b Amine (6 mmol), formaldehyde 37 wt% in water (12 mmol), ammonia borane (12 mmol), TFE (20 mL), 25 °C, open flask, 24 h. Isolated yield corresponding to amine. | |
Conclusions
In conclusion, we developed a convenient and mild reductive amination protocol. This novel methodology makes use of air-stable ammonia borane as the reducing agent and does not need any metal catalyst. In general, this reaction can be performed in both organic and aqueous solvents and is applicable to synthesize diverse amines, including (hetero)aromatic and aliphatic secondary and tertiary amines as well as N-methylated products with excellent functional group tolerance and high yields. Notably, chiral amines and amino acid derivatives can also be used in this system. In this respect, the synthesis of a plethora of interesting bio-active compounds is possible. In addition, the present methodology can be used to prepare drugs on gram-scale with good yields demonstrating the potential applications in the synthesis of pharmaceuticals.
Author contributions
RVJ and MB supervised the project. XL, RVJ and MB planned and developed the project. XL performed catalytic experiments. YH, BZ, ZM, and JG co-performed catalytic experiments and reproduced the results. AMA involved in the development of this project. XL, MB and RVJ wrote the paper.
Conflicts of interest
There are no conflicts to declare.
Acknowledgements
We gratefully acknowledge funding of the Deutsche Forschungsgemeinschaft (DFG; Project number 447724917), European Research Council (EU project 670986-NoNaCat) and the State of Mecklenburg-Vorpommern. We are grateful to the Deputyship for Research & Innovation, Ministry of Education in Saudi Arabia for financial support through the project number “375213500”. Xinmin Li thank the Chinese Scholarship Council (CSC) and National Natural Science Foundation of China (No. 22267025) for support. Yue Hu, Zhuang Ma and Jie Gao thank Chinese Scholarship Council (CSC) for fellowship. We thank the analytical team of the Leibniz-Institut für Katalyse e.V. for their excellent service.
References
- X.-F. Wu, H. Neumann and M. Beller, Palladium-catalyzed carbonylative coupling reactions between Ar–X and carbon nucleophiles, Chem. Soc. Rev., 2011, 40, 4986–5009 RSC.
- Z. Shi, C. Zhang, C. Tang and N. Jiao, Recent advances in transition-metal catalyzed reactions using molecular oxygen as the oxidant, Chem. Soc. Rev., 2012, 41, 3381–3430 RSC.
- D. Formenti, F. Ferretti, F. K. Scharnagl and M. Beller, Reduction of nitro compounds using 3d-non-noble metal catalysts, Chem. Rev., 2019, 119, 2611–2680 CrossRef CAS PubMed.
- H. M. Huang, P. Bellotti and F. Glorius, Transition metal-catalysed allylic functionalization reactions involving radicals, Chem. Soc. Rev., 2020, 49, 6186–6197 RSC.
- T. Dalton, T. Faber and F. Glorius, C–H activation: toward sustainability and applications, ACS Cent. Sci., 2021, 7, 245–261 CrossRef CAS PubMed.
- J. D. Lasso, D. J. Castillo-Pazos and C.-J. Li, Green chemistry meets medicinal chemistry: a perspective on modern metal-free late-stage functionalization reactions, Chem. Soc. Rev., 2021, 50, 10955–10982 RSC.
- J. C. Hermann, Y. Chen, C. Wartchow, J. Menke, L. Gao, S. K. Gleason, N.-E. Haynes, N. Scott, A. Petersen, S. Gabriel, B. Vu, K. M. George, A. Narayanan, S. H. Li, H. Qian, N. Beatini, L. Niu and Q.-F. Gan, Metal Impurities Cause False Positives in High-Throughput Screening Campaigns, ACS Med. Chem. Lett., 2013, 4, 197–200 CrossRef CAS PubMed.
- K. S. Egorova and V. P. Ananikov, Toxicity of metal compounds: knowledge and myths, Organometallics, 2017, 36, 4071–4090 CrossRef CAS.
- T. Senthamarai, K. Murugesan, J. Schneidewind, N. V. Kalevaru, W. Baumann, H. Neumann, P. C. J. Kamer, M. Beller and R. V. Jagadeesh, Simple ruthenium-catalyzed reductive amination enables the synthesis of a broad range of primary amines, Nat. Commun., 2018, 9, 4123 CrossRef PubMed.
- C. Bäumler, C. Bauer and R. Kempe, The synthesis of primary amines through reductive amination employing an iron catalyst, ChemSusChem, 2020, 13, 3110–3114 CrossRef PubMed.
- S. Rana, J. P. Biswas, S. Paul, A. Paik and D. Maiti, Organic synthesis with the most abundant transition metal–iron: from rust to multitasking catalysts, Chem. Soc. Rev., 2021, 50, 243–472 RSC.
- E. Peris and R. H. Crabtree, Key factors in pincer ligand design, Chem. Soc. Rev., 2018, 47, 1959–1968 RSC.
- A. Haque, L. Xu, R. A. Al-Balushi, M. K. Al-Suti, R. Ilmi, Z. Guo, M. S. Khan, W.-Y. Wong and P. R. Raithby, Cyclometallated tridentate platinum(II) arylacetylide complexes: old wine in new bottles, Chem. Soc. Rev., 2019, 48, 5547–5563 RSC.
- S.-T. Bai, G. De Smet, Y. Liao, R. Sun, C. Zhou, M. Beller, B. U. W. Maes and B. F. Sels, Homogeneous and heterogeneous catalysts for hydrogenation of CO2 to methanol under mild conditions, Chem. Soc. Rev., 2021, 50, 4259–4298 RSC.
- V. Froidevaux, C. Negrell, S. Caillol, J.-P. Pascault and B. Boutevin, Biobased amines: from synthesis to polymers; present and future, Chem. Rev., 2016, 116, 14181–14224 CrossRef CAS PubMed.
- R. V. Jagadeesh, A.-E. Surkus, H. Junge, M.-M. Pohl, J. Radnik, J. Rabeah, H. Huan, V. Schünemann, A. Brückner and M. Beller, Nanoscale Fe2O3-based catalysts for selective hydrogenation
of nitroarenes to anilines, Science, 2013, 342, 1073–1076 CrossRef CAS PubMed.
- R. V. Jagadeesh, T. Stemmler, A.-E. Surkus, H. Junge, K. Junge and M. Beller, Hydrogenation using iron oxide–based nanocatalysts for the synthesis of amines, Nat. Protoc., 2015, 10, 548–557 CrossRef CAS PubMed.
- K. Murugesan, T. Senthamarai, V. G. Chandrashekhar, K. Natte, P. C. J. Kamer, M. Beller and R. V. Jagadeesh, Catalytic reductive aminations using molecular hydrogen for synthesis of different kinds of amines, Chem. Soc. Rev., 2020, 49, 6273–6328 RSC.
-
M. H. Qureshi, Top 200 small molecule drugs by sales in 2021, University of Arizona, https://njardarson.lab.arizona.edu/content/top-pharmaceuticals-poster.
- O. I. Afanasyev, E. Kuchuk, D. L. Usanov and D. Chusov, Reductive amination in the synthesis of pharmaceuticals, Chem. Rev., 2019, 119, 11857–11911 CrossRef CAS PubMed.
- K. Natte, H. Neumann, R. V. Jagadeesh and M. Beller, Convenient iron-catalyzed reductive aminations without hydrogen for selective synthesis of N-methylamines, Nat. Commun., 2017, 8, 1344 CrossRef PubMed.
- T. Senthamarai, K. Murugesan, K. Natte, N. V. Kalevaru, H. Neumann, P. C. J. Kamer and R. V. Jagadeesh, Expedient synthesis of N-methyl- and N-alkylamines by reductive amination using reusable cobalt oxide nanoparticles, ChemCatChem, 2018, 10, 1235–1240 CrossRef CAS.
- D. Wei, A. Bruneau-Voisine, D. A. Valyaev, N. Lugan and J.-B. Sortais, Manganese catalyzed reductive amination of aldehydes using hydrogen as a reductant, Chem. Commun., 2018, 54, 4302–4305 RSC.
- G. Hahn, P. Kunnas, N. de Jonge and R. Kempe, General synthesis of primary amines via reductive amination employing a reusable nickel catalyst, Nat. Catal., 2019, 2, 71–77 CrossRef CAS.
- S. Gomez, J. A. Peters and T. Maschmeyer, The reductive amination of aldehydes and ketones and the hydrogenation of nitriles: mechanistic aspects and selectivity control, Adv. Synth. Catal., 2002, 344, 1037–1057 CrossRef CAS.
- K. Murugesan, Z. Wei, V. G. Chandrashekhar, H. Neumann, A. Spannenberg, H. Jiao, M. Beller and R. V. Jagadeesh, Homogeneous cobalt-catalyzed reductive amination for synthesis of functionalized primary amines, Nat. Commun., 2019, 10, 5443 CrossRef PubMed.
- D. Chusov and B. List, Reductive amination without an external hydrogen source, Angew. Chem., Int. Ed., 2014, 53, 5199–5201 CrossRef CAS PubMed.
- M. Sharma, J. Mangas-Sanchez, S. P. France, G. A. Aleku, S. L. Montgomery, J. I. Ramsden, N. J. Turner and G. Grogan, A mechanism for reductive amination catalyzed by fungal reductive aminases, ACS Catal., 2018, 8, 11534–11541 CrossRef CAS.
- C. Gelis, A. Heusler, Z. Nairoukh and F. Glorius, Catalytic transfer hydrogenation of arenes and heteroarenes, Chem. – Eur. J., 2020, 26, 14090–14094 CrossRef CAS PubMed.
- D. Wang and D. Astruc, The golden age of transfer hydrogenation, Chem. Rev., 2015, 115, 6621–6686 CrossRef CAS PubMed.
- S. Enthaler, Synthesis of secondary amines by iron-catalyzed reductive amination, ChemCatChem, 2010, 2, 1411–1415 CrossRef CAS.
- R. R. Thakore, B. S. Takale, G. Casotti, E. S. Gao, H. S. Jin and B. H. Lipshutz, Chemoselective reductive aminations in aqueous nanoreactors using parts per million level Pd/C catalysis, Org. Lett., 2020, 22, 6324–6329 CrossRef CAS PubMed.
- S. E. Varjosaari, V. Skrypai, P. Suating, J. J. M. Hurley, A. M. D. Lio, T. M. Gilbert and M. J. Adler, Simple metal-free direct reductive amination using hydrosilatrane to form secondary and tertiary amines, Adv. Synth. Catal., 2017, 359, 1872–1878 CrossRef CAS.
- O. S. Nayal, M. S. Thakur, V. Bhatt, M. Kumar, N. Kumar, B. Singh and U. Sharma, Synthesis of tertiary arylamines: Lewis acid-catalyzed direct reductive N-alkylation of secondary amines with ketones through an alternative pathway, Chem. Commun., 2016, 52, 9648–9651 RSC.
- O. S. Nayal, V. Bhatt, S. Sharma and N. Kumar, Chemoselective reductive amination of carbonyl compounds for the synthesis of tertiary amines using SnCl2·2H2O/PMHS/MeOH, J. Org. Chem., 2015, 80, 5912–5918 CrossRef CAS PubMed.
- K. Kuciński and G. Hreczycho, Hydrosilylation and hydroboration in a sustainable manner: from earth-abundant catalysts to catalyst-free solutions, Green Chem., 2020, 22, 5210–5224 RSC.
- V. K. Pandey, S. Bauri and A. Rit, Catalyst- and solvent-free efficient access to N-alkylated amines via reductive amination using HBpin, Org. Biomol. Chem., 2020, 18, 3853–3857 RSC.
- A. Shokrolahi, A. Zali and M. H. Keshavarz, Reductive amination of aldehydes and ketones by NaBH4 using carbon-based solid acid (CBSA) as catalyst, Green Chem. Lett. Rev., 2011, 4, 195–203 CrossRef CAS.
- Z. Luo, Y. Pan, Z. Yao, J. Yang, X. Zhang, X. Liu, L. Xu and Q.-H. Fan, BF3·Et2O as a metal-free catalyst for direct reductive amination of aldehydes with amines using formic acid as a reductant, Green Chem., 2021, 23, 5205–5211 RSC.
- Q. Zhang, S.-S. Li, M.-M. Zhu, Y.-M. Liu, H.-Y. He and Y. Cao, Direct reductive amination of aldehydes with nitroarenes using bio-renewable formic acid as a hydrogen source, Green Chem., 2016, 18, 2507–2513 RSC.
- S. Liang, P. Monsen, G. B. Hammond and B. Xu, Au/TiO2 catalyzed reductive amination of aldehydes and ketones using formic acid as reductant, Org. Chem. Front., 2016, 3, 505–509 RSC.
- L. Jiang, P. Zhou, Z. Zhang, S. Jin and Q. Chi, Synthesis of secondary amines from one-pot reductive amination with formic acid as the hydrogen donor over an acid-resistant cobalt catalyst, Ind. Eng. Chem. Res., 2017, 56, 12556–12565 CrossRef CAS.
- B. L. Davis, D. A. Dixon, E. B. Garner, J. C. Gordon, M. H. Matus, B. Scott and F. H. Stephens, Efficient regeneration of partially spent ammonia borane fuel, Angew. Chem., Int. Ed., 2009, 48, 6812–6816 CrossRef CAS PubMed.
- Q. Zhao, R. D. Dewhurst, H. Braunschweig and X. Chen, A new perspective on borane chemistry: the nucleophilicity of the B−H bonding pair electrons, Angew. Chem., Int. Ed., 2019, 58, 3268–3278 CrossRef CAS PubMed.
- A. L. Colebatch and A. S. Weller, Amine–Borane dehydropolymerization: challenges and opportunities, Chem. – Eur. J., 2019, 25, 1379–1390 CrossRef CAS PubMed.
- D. Han, F. Anke, M. Trose and T. Beweries, Recent advances in transition metal catalysed dehydropolymerisation of amine boranes and phosphine boranes, Coord. Chem. Rev., 2019, 380, 260–286 CrossRef CAS.
- C. Faverio, M. F. Boselli, F. Medici and M. Benaglia, Ammonia borane as a reducing agent in organic synthesis, Org. Biomol. Chem., 2020, 18, 7789–7813 RSC.
- S. Lau, D. Gasperini and R. L. Webster, Amine–Boranes as transfer hydrogenation and hydrogenation reagents: A mechanistic perspective, Angew. Chem., Int. Ed., 2021, 60, 14272–14294 CrossRef CAS PubMed.
- P. V. Ramachandran, S. Choudhary and A. Singh, Trimethyl borate-catalyzed, solvent-free reductive amination, J. Org. Chem., 2021, 86, 4274–4280 CrossRef CAS PubMed.
- Q. Zou, F. Liu, T. Zhao and X. Hu, Reductive amination of ketones/aldehydes with amines using BH3N(C2H5)3 as a reductant, Chem. Commun., 2021, 57, 8588–8591 RSC.
- R. V. Jagadeesh, K. Murugesan, S. A. Ahmad, H. Neumann, M.-M. Pohl, J. Radnik and M. Beller, MOF-derived cobalt nanoparticles catalyze a general synthesis of amines, Science, 2017, 358, 326–332 CrossRef CAS PubMed.
- K. Murugesan, M. Beller and R. V. Jagadeesh, Reusable nickel nanoparticles-catalyzed reductive amination for selective synthesis of primary amines, Angew. Chem., Int. Ed., 2019, 58, 5064–5068 CrossRef CAS PubMed.
- J. Leonard, A. J. Blacker, S. P. Marsden, M. F. Jones, K. R. Mulholland and R. Newton, A Survey of the borrowing hydrogen approach to the synthesis of some pharmaceutically relevant intermediates, Org. Process Res. Dev., 2015, 19, 1400–1410 CrossRef CAS.
- T. Yan, B. L. Feringa and K. Barta, Direct catalytic N-alkylation of α-amino acid esters and amides using alcohols with high retention of stereochemistry, ChemSusChem, 2021, 14, 2303–2307 CrossRef CAS PubMed.
- T. Yan, B. L. Feringa and K. Barta, Direct N-alkylation of unprotected amino acids with alcohols, Sci. Adv., 2017, 3, eaao6494 CrossRef PubMed.
|
This journal is © the Partner Organisations 2023 |
Click here to see how this site uses Cookies. View our privacy policy here.