DOI:
10.1039/D2QO02023H
(Research Article)
Org. Chem. Front., 2023,
10, 1393-1398
Fluorometric detection of volatile amines using an indanonalkene platform†
Received
22nd December 2022
, Accepted 5th January 2023
First published on 10th January 2023
Abstract
Excessive volatile amines are considered to cause environmental pollution and harm to human health, and especially, ammonia has been reported to be associated with a variety of human diseases. In this article, we developed a gas sensor named CA-SCH3 based on an indanonalkene structure, for real-time/on-site and quantitative detection of volatile amines. The non-luminescent probe containing a bis-vinylogous thioester receptor exhibited high reactivity towards volatile amines, e.g., ammonia, via an amine–thiol scrambling reaction. A turn-on fluorescence signal and a blue shift in the UV-vis absorbance signal were observed for this chemosensor in the presence of ammonia. A paper-based gas sensor loaded with the probe exhibited fluorescence enhancement for the quantification of ammonia gas. Volatile amines from fish spoilage were also captured and detected via the paper sensor. Furthermore, we prepared a polyethylene glycol film interlinked by the CA-SCH3 reagent which exhibited a dual response of macroscopic degradation and fluorescence readout towards ammonia vapors. The fluorescent probe (CA-SCH3) with a novel amine receptor offers extensive possibilities for developing additional photoluminescent detection systems.
Introduction
Amines are an important class of chemical substances in nature and industrial production.1,2 Many of them are volatile, corrosive and dangerous.3,4 Consequently, sensing and monitoring of such highly toxic and volatile amine substances are required for early-stage warning systems and to protect the environment and human health.5,6 The detection of volatile amines is thus critical in many fields.6–9 In particular, endogenous volatile biogenetic amines in exhaled breath and amine compounds in urine and sweat are considered to be analytes for the early diagnosis of diseases.10 Moreover, in the process of food spoilage, microorganisms decompose free amino acids into biogenic amines such as histamine, cadaverine, putrescine, dimethylamine, trimethylamine, etc., which can cause serious harm to human health.11,12 Therefore, it is important to develop detection methods for volatile amines with high sensitivity for real world applications.
The gas sensors reported previously include electrochemical sensors, semiconductor sensors and photochemical sensors.13–15 They are generally based on selected metal oxides, which endow them with good sensitivity but limited selectivity,16 and instability and loss of sensitivity after exposure to moisture or long operating time.17,18 In recent years, gas sensors based on semiconductors have been reported to detect ammonia.18–20 However, ammonia sensors based on these oxide chemical resistors have to work at high temperatures, thus causing energy consumption and requiring thermal insulation. In addition, a variety of composite gas sensors based on conjugated polymers of metal oxide carbon materials have been reported,21–23 and they can sense amine VOCs (volatile organic compounds) at room temperature. However, their response rates are undesirable for further practical applications.
For fluorescent chemical sensors, multiple luminescent materials have been reported for the detection of amines.24–26 Koner et al.15 synthesized a luminescent probe containing a cyclized anhydride with molecular aggregation induced emission (AIE). Tang et al.27 used the O-acetyl bond as an amine molecular trap to detect the presence of amines to produce a fluorescence indicator with AIE characteristics. Although different systems have been constructed to detect amine vapors, these systems are limited by the number of reactive amine receptors available. With this in mind, the development of chemosensors using a new receptor to capture amine vapors, along with easy operation and structural simplicity, would be beneficial for further applications and could provide an alternative fluorescence detection tool.
Previously, we established a photoluminescent platform based on indanonalkene luminogens (Scheme 1A).28,29 On this basis, we employed CA-SCH3, an indanonalkene molecule containing a bis-vinylogous thioester group, as an amine receptor for the fluorescence detection of volatile amines via amine–thiol scrambling (Scheme 1B).30–32 As such, the luminescent precursor CA-SCH3 was turned on in the presence of ammonia in both solution and solid states to generate the luminophore CA-NH2, exhibiting a strong green emission (Scheme 1B). In addition, multiple volatile amines can be detected using a paper-based probe loaded with CA-SCH3, and further used to detect amine vapor in rotting fish. Furthermore, a non-luminescent soft material, i.e., hydrogel, was prepared, which can be degraded by NH3(g) through an amine–thiol decoupling reaction and the macroscopic degradation process can be tracked visually on spatial and time scales by following the changes in luminescence as a result of the generation of the indicator. Thus, we developed a new amine fluorescent chemosensor with ease of operation and structural simplicity, which demonstrated promise for the construction of portable devices.
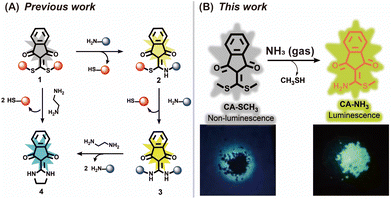 |
| Scheme 1 Schematic illustration of the previous and current work. (A) Previous work: indanonalkene photoluminescent platform; (B) this work: fluorescence detection of volatile amines. Conjugate acceptor CA-SCH3 as the chemosensor and its reaction with NH3 to generate luminogen CA-NH2. Inset pictures: non-luminescent solid CA-SCH3 and luminescent solid CA-NH2. | |
Results and discussion
UV-Vis and fluorescence responses
The luminescent precursor CA-SCH3 was prepared according to the previous method.33 The molecule contains bis-vinylogous thioester active moieties, which are reactive towards amine derivatives.32,34 The chemical reaction enables the design of a reaction-based chemosensor through amine–thiol scrambling, with the release of methylmercaptan. Firstly using UV-Vis absorbance spectroscopy, kinetic tracking of the photophysicochemical reaction between CA-SCH3 (10 μM) and ammonia (0.0125%) over 0–180 min resulted in a ratiometric change, with a decrease at λabs = 366 nm an increase at λabs = 336 nm and isosbestic point at 345 nm in a H2O/DMSO (1%/99%) system (Fig. 1A and B). As can be observed by the naked eye, the test solution exhibited a colorimetric change (Fig. 1C) from yellowish to colorless. Secondly, significant fluorescence turn-on was observed for the luminescent precursor CA-SCH3 (10 μM) in the presence of ammonia (0.0125%), resulting in a more than 32-fold fluorescence enhancement at λem = 495 nm (Fig. 1D–F and S1†). To confirm the reaction product and optical properties, we prepared an independent sample of CA-NH2 and studied its absorbance and fluorescence spectra (Fig. S2 and S3†), which showed a good agreement with the absorbance and fluorescence spectra mentioned above. In addition, it was observed that the water fraction (1–99%) could affect the reaction between CA-SCH3 and NH3·H2O in DMSO. With an increase in the water fraction, the fluorescence was significantly quenched and the emission wavelength red shifted (Fig. S4†), which was probably due to the hydrogen bonding induced internal energy conversion of luminescent CA-NH2.
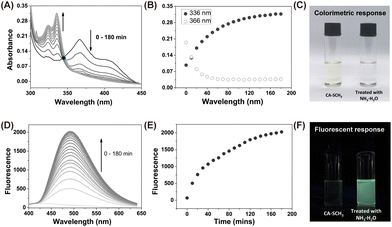 |
| Fig. 1 UV-Vis and fluorescence responses for the CA-SCH3 probe. (A) Time-dependent UV-Vis absorbance spectra of CA-SCH3 (10 μM) upon addition of NH3·H2O (0.0125%) in a H2O/DMSO = 1% system over 180 min. (B) Time course of absorbance at λabs = 336 nm (●) and λabs = 366 nm (○), respectively, referred to (A). (C) Colorimetric photograph before and after NH3·H2O treatment. (D) Time-dependent fluorescence spectra of CA-SCH3 (10 μM) upon addition of NH3·H2O (0.0125%) in a H2O/DMSO = 1% system. λex = 336 nm; λem = 495 nm. (E) Time course of fluorescence intensity at 495 nm, referred to as (D). (F) Fluorescence photograph before and after NH3·H2O treatment under 365 nm UV light. | |
Proposed mechanism of the optical sensing
Next, the spatial conformation optimization and energy level difference calculation of the HOMO–LUMO orbitals for CA-SCH3 and CA-NH2 were analysed using density functional theory (DFT) software. According to the calculation results (Fig. 2), the HOMO–LUMO gap of CA-NH2 was found to be 4.35 eV while that of CA-SCH3 was 3.93 eV, which matched the experimental blue shift in absorbance. As reported in our previous work,28 the fluorescence quenching due to vibrational energy loss from the bis-vinylogous thioester of CA-SCH3 accounted for the initial state of the probe. When the thiol group was substituted by the amine, the quenching factor was diminished which truned on the photoluminescence output. The mechanism to explain the fluorescence performance of CA-NH2 was proposed as excited state electronic charge distribution from the double bond that was stabilized by the amine, which led to the UV-Vis absorbance shifting to a shorter wavelength with higher energy and fluorescence enhancement.
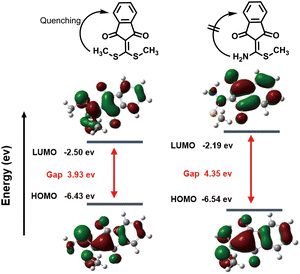 |
| Fig. 2 Optimized DFT molecular orbital (LUMO and HOMO) for CA-SCH3 and CA-NH2 at the B3LYP/6-31G (d) level (parameter settings are shown in the ESI†). | |
Paper-based sensor for the detection of ammonia vapors
Next, we prepared test papers loaded with CA-SCH3 as a portable sensor for the real-time detection of amine vapors. A solution of CA-SCH3 (10 mM) in CH2Cl2 was dropped on to star-shaped paper and dried by evaporation. As shown in Fig. 3A, the paper-based sensor CA-SCH3 was non-emissive under 365 nm UV irradiation, while treatment with ammonia vapor (produced by NH3·H2O) led to luminescence turn-on over 1 h in a dose-dependent manner. As the concentration of ammonia vapor ranged from 0 to 624 ppm, the emission brightness and fluorescence intensity of the paper enhanced. The solid fluorescence emission spectra of the CA-SCH3-loaded paper after exposure to ammonia vapor are shown in Fig. 3B. A 16.5-fold signal enhancement in the presence of ammonia vapor produced from 5% ammonia at λem = 336 nm was observed. With the concentration of ammonia vapor increasing from 0 to 624 ppm (NH3·H2O concentrations from 0 to 0.125%), a moderate linear relationship (R2 = 0.918) between I/I0 at 495 nm and analyte concentration was observed (Fig. 3C), which can be potentially used as a calibration curve for the detection of unknown samples. Based on the signal-to-noise method, the detection limit (3σ/S) for ammonia vapor was calculated to be 36 ppm (see the ESI†). These experimental results demonstrate that CA-SCH3-loaded filter paper can be potentially used as a portable real-time sensor for the tracking and quantification of volatile amines.
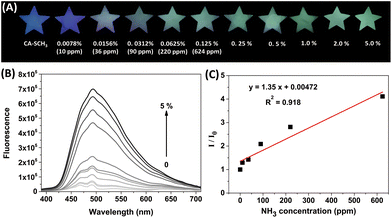 |
| Fig. 3 (A) Photographs of paper-based sensor CA-SCH3 treated with various concentrations of NH3·H2O for 1 h were taken under UV light (365 nm). (B) Solid state fluorescence spectra of the filter papers in (A). (C) Linear fitting of I/I0 with ammonia concentrations ranging from 0 to 624 ppm. I0 and I show the fluorescence intensities of CA-SCH3 at 495 nm and that upon exposure to various concentrations of amine vapor. | |
Paper-based sensor for the detection of multiple amines
In addition to ammonia, diverse amine species can be found in nature, as well as in industrial manufacturing. Thus, the capability of the sensor to detect various amine vapors was then investigated. As shown in Fig. 4B, the fluorescence emission of the paper sensors was turned on and enhanced in the presence of different amine vapors, including ammonia (2.34-fold), hydrazine (2.31-fold), aliphatic amine (benzylamine, 4.65-fold), EtNH2 (2.58-fold), putrescine (1.57-fold), 1,3-propylene diamine (1.48-fold), cadaverine (1.39-fold), trimethylamine (1.59-fold), and dimethylamine (5.35-fold) vapors (produced by using an aqueous or methanol solution (0.08 M) of amine compounds), respectively over the initial CA-SCH3 (Fig. 4C). The signal enhancement indicated that these amine vapors led to emissive amine substituted products after amine–thiol scrambling with CA-SCH3 (Fig. 4A). However, aromatic amine derivatives, i.e. aniline and p-bromoaniline, did not exhibit any fluorescence signal response, probably due to reduced nucleophilicity.27 In addition, histamine, as a kind of biogenic amine, may not produce fluorescence response most probably due to low volatility.35 Therefore the paper-based CA-SCH3 sensor is capable of selective luminescence detection of volatile amine vapors, and is promising for future applications (see below).
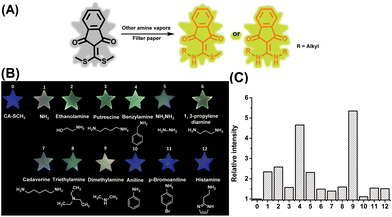 |
| Fig. 4 (A) Schematic diagram of the reaction of CA-SCH3 with volatile amines. (B) Selectivity of CA-SCH3 for a variety of volatile amine compounds (numbers 1 to 12 represent NH3, ethanolamine, putrescine, benzylamine, NH2NH2, 1,3-propylene diamine, cadaverine, triethylamine, dimethylamine, aniline, p-bromoaniline and histamine respectively). The test paper was exposed to a variety of amine vapors released from an aqueous or methanol solution (0.08 M) of amine compounds for 2 h and then photographed under 365 nm UV light irradiation. (C) Relative fluorescence intensity at 495 nm of the paper-based probe CA-SCH3 after exposure to the 12 amine compounds in (B). λex = 336 nm. | |
Real-world application of the amine sensor
The generation of biogenic amines by microbial fermentation in food is used to help evaluate freshness.36 As such, the development of a simple portable detector for reliable, fast, and real-time assessment of food freshness would be particularly beneficial. Therefore, we decided to evaluate food spoilage utilizing the newly developed chemosensor. When a cod fillet was sealed in a plastic box and stored at 4 °C for 5 days, almost no fluorescence enhancement was observed for the paper sensor after placing it with the cod fillet for 2 h (Fig. 5A). However, a strong fluorescence emission was observed by the naked eye when the cod fillet was kept at room temperature (25 °C) for 5 days (Fig. 5A), indicating that the cod fillet has seriously deteriorated, and was not suitable for consumption. These results demonstrate that the paper-based CA-SCH3 sensor can be employed for food spoilage detection by reporting the release of biogenic amine vapor from rotting food.
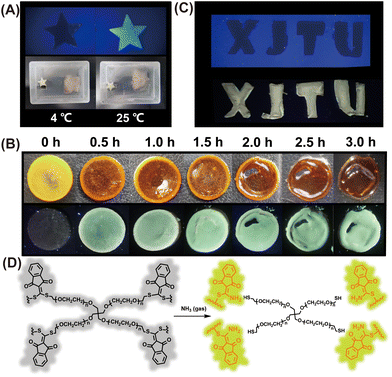 |
| Fig. 5 (A) Paper-based sensor CA-SCH3 was placed in a box for 2 hours for the detection of amine vapors generated from cod fillets, stored at 4 °C and 25 °C for 5 days, respectively. (B) Fluorescence responses and degradation of the CA-PEG gel by ammonia vapor produced by 2% NH3·H2O for 3 hours. (C) Fluorescence responses of the gel pattern treated with ammonia vapor (from 5% NH3·H2O) under 365 nm UV light. (D) Sensing mechanism of the gel constructed from the reaction of CA-SCH3 with 4-arm PEG thiol in the presence of ammonia (gas). Fluorescence turn-on and gel degradation after amine–thiol replacement. | |
Hydrogel film sensor for volatile amine detection
In addition, typical fluorescent chemosensors mainly work in solution states.37,38 Solid-state fluorescence sensory systems, such as test papers and polymeric films, are attractive because of their portability, operational simplicity, and stability.39 The recognition mode or sensitivity between the chemosensor and the target relies on chemical reactivity or binding affinity.40 Nevertheless, they suffer from restricted sensitivity and relatively long equilibrium response time because of the hindered diffusion of the analyte within low-porosity dense solid systems.41 As such, polymeric hydrogels could be a promising alternative as chemical sensing systems owing to their high porosity, 3D network, and tissue-like mechanical properties, which in principle facilitate analyte absorption and diffusion.
As such we designed and developed a polymeric gel film named CA-PEG (Fig. S5†), through linking four-arm poly(ethylene glycol) thiol with CA-SCH3 through thiol–thiol scrambling and high cross linking.29 The scanning electron microscopic (SEM) images of CA-PEG are given in Fig. S6.† The pore diameter distribution of the CA-PEG gel was analyzed using pore diameter analysis software, and the average pore size was determined to be ca. 53 μm. On this basis, when the non-luminescent gel film (radius × thickness = 5 mm × 1 mm) was exposed to amine vapor (produced by 2% NH3·H2O), CA-PEG exhibited fluorescence enhancement within 30 min (Fig. 5B), and after 1 h exposure, the hydrogel underwent macroscopic degradation with the appearance of wrinkles (Fig. 5B). Then after 2 h, more degradation and further enhanced fluorescence could be observed. The mechanism of degradation was attributed to the amine–thiol scrambling reaction between the amines and CA-SCH3 linkers (Fig. 5D) while the fluorescence enhancement was due to the generation of CA-NH2 derivatives as the indicator. Moreover, as shown in Fig. 5C, the letters “XJTU” were carved out of the gel and were non-fluorescent under a UV lamp. Then, exposure to ammonia vapors led to a significant fluorescence enhancement, which indicated that the hydrogel film can be applied to dynamic anti-counterfeiting patterns by fluorescence turn-on of the hydrogel film when it encounters volatile amine gases.
Conclusions
In summary, based on the indanonalkene photoluminescent platform and an active amine receptor, we developed a fluorescent chemosensor, which produced a fluorescence indicator after an amine–thiol substitution reaction, thereby generating an optical signal suitable for the detection of volatile amines. The chemosensor was then used to prepare portable paper-based sensors and a polymeric sensor film. When exposed to volatile organic amine compounds, the physical adsorption and chemical reaction led to enhanced fluorescence emission. Furthermore, the real-time quantitative detection of amines was achieved. The sensor also exhibits high sensitivity towards aliphatic amine vapors over aromatic amines. Due to its easy preparation, portability, simple operation and good sensitivity, the sensor and field-deployable device could be potentially used for the detection of volatile environmental amines.
Author contributions
XLS conceptualization, supervision and writing – review & editing. TDJ and JZ checked the validation and manuscript writing – review & editing. HC collaborated in funding acquisition. THW supported the experimental methodology. PZ performed the experiment, collected all the data and wrote the original draft. All authors have given approval to the final version of the manuscript.
Conflicts of interest
The authors declare no competing conflicts of interest.
Acknowledgements
XLS thanks the National Natural Science Foundation of China (no. 21907080 and 22278330) and Youth Innovative Team (no. xtr052022012) from Xi'an Jiaotong University. JZ thanks the National Natural Science Foundation of China no. 31770909. T. D. J. wishes to thank the Royal Society for a Wolfson Research Merit Award and the Open Research Fund of the School of Chemistry and Chemical Engineering, Henan Normal University for support (2020ZD01).
References
- S. Körsten and G. J. Mohr, Star-Shaped Tripodal Chemosensors for the Detection of Aliphatic Amines, Chem. – Eur. J., 2011, 17, 969–975 CrossRef PubMed
.
- J. Kumpf, S. T. Schwaebel and U. H. F. Bunz, Amine Detection with Distyrylbenzenedialdehyde-Based Knoevenagel Adducts, J. Org. Chem., 2015, 80, 5159–5166 CrossRef CAS PubMed
.
- R. S. Andre, Q. P. Ngo, L. Fugikawa-Santos, D. S. Correa and T. M. Swager, Wireless Tags with Hybrid Nanomaterials for Volatile Amine Detection, ACS Sens., 2021, 6, 2457–2464 CrossRef CAS PubMed
.
- Z. Tian, P. Song, Z. Yang and Q. Wang, Reduced graphene oxide-porous In2O3 nanocubes hybrid nanocomposites for room-temperature NH3 sensing, Chin. Chem. Lett., 2020, 31, 2067–2070 CrossRef CAS
.
- T. Lin, X. Lv, Z. Hu, A. Xu and C. Feng, Semiconductor Metal Oxides as Chemoresistive Sensors for Detecting Volatile Organic Compounds, Sensors, 2019, 19, 233–233 CrossRef PubMed
.
- Y. Liu, B. Sang, H. Wang, Z. Wu, Y. Wang, Z. Wang, Z. Peng and W. Chen, High ammonia sensitive ability of novel Cu12Sb4S13 quantum dots@reduced graphene oxide nanosheet composites at room temperature, Chin. Chem. Lett., 2020, 31, 2109–2114 CrossRef CAS
.
- B. Timmer, W. Olthuis and A. van den Berg, Ammonia sensors and their applications—a review, Sens. Actuators, B, 2005, 107, 666–677 CrossRef CAS
.
- Q. Feng, Y. Zeng, P. Xu, S. Lin, C. Feng, X. Li and J. Wang, Tuning the electrical conductivity of amorphous carbon/reduced graphene oxide wrapped-Co3O4 ternary nanofibers for highly sensitive chemical sensors, J. Mater. Chem. A, 2019, 7, 27522–27534 RSC
.
- S. Sentellas, Ó. Núñez and J. Saurina, Recent Advances in the Determination of Biogenic Amines in Food Samples by (U)HPLC, J. Agric. Food Chem., 2016, 64, 7667–7678 CrossRef CAS PubMed
.
- J.-L. Tan, Z.-X. Yong and C.-K. Liam, Using a chemiresistor-based alkane sensor to distinguish exhaled breaths of lung cancer patients from subjects with no lung cancer, J. Thorac. Dis., 2016, 8, 2772–2783 CrossRef PubMed
.
- K. B. Biji, C. N. Ravishankar, R. Venkateswarlu, C. O. Mohan and T. K. S. Gopal, Biogenic amines in seafood: a review, Int. J. Food Sci. Technol., 2016, 53, 2210–2218 CrossRef CAS PubMed
.
- V. Feddern, H. Mazzuco, F. N. Fonseca and G. J. M. M. de Lima, A review on biogenic amines in food and feed: toxicological aspects, impact on health and control measures, Anim. Prod. Sci., 2019, 59, 608–618 CrossRef
.
- L. Spinelle, M. Gerboles, G. Kok, S. Persijn and T. Sauerwald, Review of Portable and Low-Cost Sensors for the Ambient Air Monitoring of Benzene and Other Volatile Organic Compounds, Sensors, 2017, 17, 1520–1520 CrossRef PubMed
.
- A. Gliszczyńska-Świgło and J. Chmielewski, Electronic Nose as a Tool for Monitoring the Authenticity of Food. A Review, Food Anal. Methods, 2017, 10, 1800–1816 CrossRef
.
- R. Roy, N. R. Sajeev, V. Sharma and A. L. Koner, Aggregation Induced Emission Switching Based Ultrasensitive Ratiometric Detection of Biogenic Diamines Using a Perylenediimide-Based Smart Fluoroprobe, ACS Appl. Mater. Interfaces, 2019, 11, 47207–47217 CrossRef CAS PubMed
.
- T. Romih, E. Menart, V. Jovanovski, A. Jerič, S. Andrenšek and S. B. Hočevar, Sodium-Polyacrylate-Based Electrochemical Sensors for Highly Sensitive Detection of Gaseous Phenol at Room Temperature, ACS Sens., 2020, 5, 2570–2577 CrossRef CAS PubMed
.
- Z. Li, H. Li, Z. Wu, M. Wang, J. Luo, H. Torun, P. Hu, C. Yang, M. Grundmann, X. Liu and Y. Fu, Advances in designs and mechanisms of semiconducting metal oxide nanostructures for high-precision gas sensors operated at room temperature, Mater. Horiz., 2019, 6, 470–506 RSC
.
- H. G. Moon, Y. Jung, S. D. Han, Y.-S. Shim, W.-S. Jung, T. Lee, S. Lee, J. H. Park, S.-H. Baek, J.-S. Kim, H.-H. Park, C. Kim and C.-Y. Kang, All villi-like metal oxide nanostructures-based chemiresistive electronic nose for an exhaled breath analyzer, Sens. Actuators, B, 2018, 257, 295–302 CrossRef CAS
.
- C. Zamani, O. Casals, T. Andreu, J. R. Morante and A. Romano-Rodriguez, Detection of amines with chromium-doped WO3 mesoporous material, Sens. Actuators, B, 2009, 140, 557–562 CrossRef CAS
.
- A. T. Güntner, M. Righettoni and S. E. Pratsinis, Selective sensing of NH3 by Si-doped α-MoO3 for breath analysis, Sens. Actuators, B, 2016, 223, 266–273 CrossRef
.
- Y. Yan, M. Zhang, C. H. Moon, H.-C. Su, N. V. Myung and E. D. Haberer, Viral-templated gold/polypyrrole nanopeapods for an ammonia gas sensor, Nanotechnology, 2016, 27, 325502 CrossRef PubMed
.
- F. Schütt, V. Postica, R. Adelung and O. Lupan, Single and Networked ZnO–CNT Hybrid Tetrapods for Selective Room-Temperature High-Performance Ammonia Sensors, ACS Appl. Mater. Interfaces, 2017, 9, 23107–23118 CrossRef PubMed
.
- L. T. Duy, D.-J. Kim, T. Q. Trung, V. Q. Dang, B.-Y. Kim, H. K. Moon and N.-E. Lee, High Performance Three-Dimensional Chemical Sensor Platform Using Reduced Graphene Oxide Formed on High Aspect-Ratio Micro-Pillars, Adv. Funct. Mater., 2015, 25, 883–890 CrossRef CAS
.
- A. Weißenstein, V. Grande, C. R. Saha-Möller and F. Würthner, Water-soluble naphthalene diimides: synthesis, optical properties, and colorimetric detection of biogenic amines, Org. Chem. Front., 2018, 5, 2641–2651 RSC
.
- S. Yu, W. Plunkett, M. Kim, E. Wu and L. Pu, 1,1′-Bi-2-naphthol-fluoroacetyl compounds in fluorescent recognition of amines, Org. Chem. Front., 2014, 1, 395–404 RSC
.
- S. Kuwahara, M. Nakamura, A. Yamaguchi, M. Ikeda and Y. Habata, Combination of a New Chiroptical Probe and Theoretical Calculations for Chirality Detection of Primary Amines, Org. Lett., 2013, 15, 5738–5741 CrossRef CAS PubMed
.
- M. Gao, S. Li, Y. Lin, Y. Geng, X. Ling, L. Wang, A. Qin and B. Z. Tang, Fluorescent Light-Up Detection of Amine Vapors Based on Aggregation-Induced Emission, ACS Sens., 2016, 1, 179–184 CrossRef CAS
.
- T. Wu, W. Bian, C. Wang, J. Qiu, X. Sun and X. Qian, A ring-controlled fluorescent platform for visualizing polymer degradation, Aggregate, 2022, e295, DOI:10.1002/agt2.295
.
- X. Feng, T. Wu, X. Sun and X. Qian, “Indanonalkene” Photoluminescence Platform: Application in Real-Time Tracking the Synthesis, Remodeling, and Degradation of Soft Materials, J. Am. Chem. Soc., 2021, 143, 21622–21629 CrossRef CAS PubMed
.
- X. Sun, M. Chwatko, D.-H. Lee, J. L. Bachman, J. F. Reuther, N. A. Lynd and E. V. Anslyn, Chemically Triggered Synthesis, Remodeling, and Degradation of Soft Materials, J. Am. Chem. Soc., 2020, 142, 3913–3922 CrossRef CAS PubMed
.
- X. Feng, M. Du, H. Wei, X. Ruan, T. Fu, J. Zhang and X. Sun, Chemically triggered life control of “smart” hydrogels through click and declick reactions, Front. Chem. Sci. Eng., 2022, 16, 1399–1406 CrossRef CAS
.
- T. Wu, T. Liang, W. Hu, M. Du, S. Zhang, Y. Zhang, E. V. Anslyn and X. Sun, Chemically
Triggered Click and Declick Reactions: Application in Synthesis and Degradation of Thermosetting Plastics, ACS Macro Lett., 2021, 10, 1125–1131 CrossRef CAS PubMed
.
- B. M. El-Zaatari, J. S. A. Ishibashi and J. A. Kalow, Cross-linker control of vitrimer flow, Polym. Chem., 2020, 11, 5339–5345 RSC
.
- L. Chang, C. Wang, S. Han, X. Sun and F. Xu, Chemically Triggered Hydrogel Transformations through Covalent Adaptable Networks and Applications in Cell Culture, ACS Macro Lett., 2021, 10, 901–906 CrossRef CAS PubMed
.
- A. K. Anderson, Biogenic and volatile amine-related qualities of three popular fish species sold at Kuwait fish markets, Food Chem., 2008, 107, 761–767 CrossRef CAS
.
- C. Ruiz-Capillas and A. Moral, Production of Biogenic Amines and Their Potential Use as Quality Control Indices for Hake (Merluccius merluccius, L.) Stored in Ice, J. Food Sci., 2001, 66, 1030–1032 CrossRef CAS
.
- F. Lai, J. Yang, R. Huang, Z. Wang, J. Tang, M. Zhang, R. Miao and Y. Fang, Nondestructive Evaluation of Fish Freshness through Nanometer-Thick Fluorescence-Based Amine-Sensing Films, ACS Appl. Nano Mater., 2021, 4, 2575–2582 CrossRef CAS
.
- X. Sun, A. A. Boulgakov, L. N. Smith, P. Metola, E. M. Marcotte and E. V. Anslyn, Photography Coupled with Self-Propagating Chemical Cascades: Differentiation and Quantitation of G- and V-Nerve Agent Mimics via Chromaticity, ACS Cent. Sci., 2018, 4, 854–861 CrossRef CAS PubMed
.
- H. Tai, Z. Duan, Y. Wang, S. Wang and Y. Jiang, Paper-Based Sensors for Gas, Humidity, and Strain Detections: A Review, ACS Appl. Mater. Interfaces, 2020, 12, 31037–31053 CrossRef CAS PubMed
.
- X. Sun and T. D. James, Glucose Sensing in Supramolecular Chemistry, Chem. Rev., 2015, 115, 8001–8037 CrossRef CAS PubMed
.
- S. K. Mahadeva, K. Walus and B. Stoeber, Paper as a Platform for Sensing Applications and Other Devices: A Review, ACS Appl. Mater. Interfaces, 2015, 7, 8345–8362 CrossRef CAS PubMed
.
Footnote |
† Electronic supplementary information (ESI) available: Fluorescence images for the reaction; DFT calculations; optical properties for CA-SCH3 and CA-NH2; calculation of detection limit; synthesis of hydrogels; scanning electron microscopy (SEM) image of CA-PEG gel; NMR and HRMS spectra. See DOI: https://doi.org/10.1039/d2qo02023h |
|
This journal is © the Partner Organisations 2023 |
Click here to see how this site uses Cookies. View our privacy policy here.