DOI:
10.1039/D2RA06988A
(Paper)
RSC Adv., 2023,
13, 2004-2009
N-Cyano sulfilimine functional group as a nonclassical amide bond bioisostere in the design of a potent analogue to anthranilic diamide insecticide†
Received
4th November 2022
, Accepted 23rd December 2022
First published on 11th January 2023
Abstract
To explore the potential of the N-cyano sulfilimine group as an amide bond isostere, a derivative of the blockbuster anthranilic diamide, chlorantramiliprole, was synthesized and evaluated with regard to its physicochemical properties, permeability, and biological activity. Given the combination of N-cyano sulfilimine chlorantraniliprole 1 and its strong hydrogen bond acceptor character, high permeability, and excellent insecticidal activity, the N-cyano sulfilimine functional group could be considered as an amide bond isostere.
Introduction
The introduction of a bioisostere, a functional group with similar physicochemical and biological properties, is a common strategy for the enhancing the properties of biologically active compounds, such as their potency, selectivity and suitable pharmacokinetic (PK) or pharmacodynamic (PD) parameters.1,2 A representative example of such a strategy is replacement of an amide bond with a surrogate structure.3–5 Indeed, amide bonds are one of the most important functional groups in medicinal chemistry and play a crucial role in the composition of numerous biologically active molecules.6,7 However, amide bonds have a permeation problem arising from hydrolysis and polarity.3,4 In addition, resonance stabilization of amides, mainly caused by differences in electronegativity between oxygen and nitrogen, can leads to their inertness. Consequently, the carbonyl functional group of amides is less electrophilic than the carbonyl of other carboxylic acid derivatives (Fig. 1A).8,9 Under such circumstances, attempting to replace the amide moiety with a surrogate structure may be valuable. Among the most commonly applied isosteres of the amide group, sulfonamides are known to be useful for increasing water solubility and providing additional hydrogen bond acceptors (HBAs).3 Sulfonamides, the most common S(VI) functional groups, have attracted considerable attention, and intensified research activities with regard to crop science and pharmaceutical industry studies have resulted in many marketed products.10–12 Distinguishing features of the sulfur–nitrogen (S–N) bond, such as its polarized character13,14 and the ability to adopt a wide variety of oxidation states of sulfur, has led to important breakthroughs in many areas.15–19
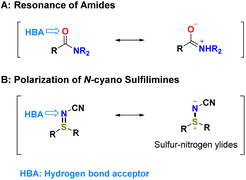 |
| Fig. 1 (A) Resonance forms of amides,8,9 (B) polarized forms of N-cyano sulfilimines20–24. | |
Recently, exploration of sulfilimidoyl S(IV) and sulfonimidoyl S(VI) functional groups, such as sulfilimines25–30 (Fig. 1B) and sulfoximines,31–34 has facilitated the discovery of important agrochemicals25–30,35–38 as well as clinical candidates of the kinase inhibitors.39,40 The unique hydrogen bond donor and acceptor properties of the sulfonimidoyl groups have led to their widespread application in drug design as bioisosteres for carboxylic acids, alcohols and sulfones.41–45
N-Cyano sulfilimines have generally been applied as building blocks in the synthesis of biologically active N-cyano and NH-sulfoximines.25–28,30,46–54 The S
N bond of S(IV) functional group is stabilized by electron-withdrawing groups and can be considered as a sulfur–nitrogen ylide in its ionic form (Fig. 1B).20–24 Furthermore, there has been increased application with regard to drug development46,53–55 and agrochemical research.25–28 For a particular example, researchers at Pfizer studied the discovery of new types of clinically useful β-lactam drugs, such as penicillin an N-cyano sulfilimine. The resulting introduction of an N-cyano sulfilimine moiety led to formation of intramolecular N–H⋯N hydrogen bonding (Fig. 2A).46 Intramolecular hydrogen bonds (IHBs) play crucial role in absorption, distribution, metabolism, and excretion (ADME)-toxicology profiles.56,57 It has been suggested that IHB can improve intrinsic membrane permeability and intestinal absorption beyond the rule of five chemical space.58,59
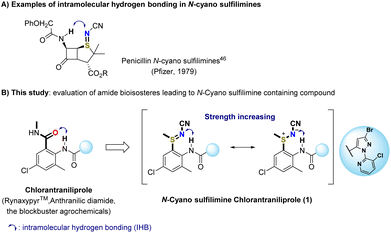 |
| Fig. 2 (A) Penicillin N-cyano sulfilimine,46 (B) N-cyano sulfilimine chlorantraniliprole 1: example of this study. | |
In this context, introduction of an N-cyano sulfilimine group, as a suitably positioned HBA functionality, seemed to be attractive based on the possible role of IHB in facilitating permeability (Fig. 1B). Consequently, we reasoned that N-cyano sulfilimines may hold considerable promise as non-classical amide isosteres.
In order to apply the aforementioned approach in our discovery program, we decided to study the N-cyano sulfilimine group-substituted chlorantraniliprole analog 1 based on our previous research.49–52 As the reference molecule, chlorantraniliprole has captured a significant market share in the insect control business. This insecticide belong to the anthranilic diamide class of chemistry and provides excellent crop protection.60 To determine the changes in physicochemical properties caused by the presence of IHB, the amide group was replaced with an N-cyano sulfilimine moiety (Fig. 2). We assumed that the formation of a sulfur–nitrogen ylide ionic form of N-cyano-substituted sulfilimine 1 could increase the strength of N–H⋯N–CN hydrogen bonding, and provide enhanced permeability via IHB while maintaining potency.
Results and discussion
Synthesis
Referring to the previous observation that it was better to perform sulfoximination of sulfide at the late-stage,61 chlorantraniliprole sulfide 6 was prepared (Scheme 1). Starting from the commercially available 4-chloro-2-methylaniline 2, 6-chloro-4-methylbenzo[d]thiazol-2-amine 3 was formed through reaction with ammonium thiocyanate (NH4SCN) in the presence of bromine in acetic acid. 2-Aminothiophenol 4 was successfully prepared by the reaction of benzothiazole 3 with hydrazine monohydrate62 and was readily converted to thiomethylated aniline 5 by alkylation with methyl iodide.63 Then, aniline 5 was coupled with 3-bromo-1-(3-chloropyridin-2-yl)-1H-pyrazole-5-carboxylic acid to give the desired chlorantraniliprole sulfide 6 with an excellent yield (41% for 4 steps).49,50,64 Impressively, the synthesis of the sulfide 6 relied only on recrystallization and did not require column chromatography methods. This protocol could be readily scaled up to the 100 g scale and applied to production of organosulfur-substituted chlorantraniliprole analogs.
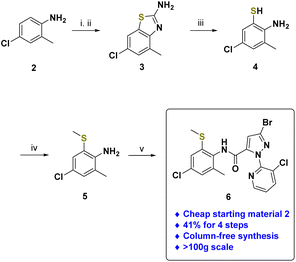 |
| Scheme 1 Reagents and conditions: (i) NH4SCN, AcOH, RT, 0.5 h, (ii) Br2, AcOH, RT, 24 h, (iii) hydrazine monohydrate, 2-methoxyethanol, (iv) tBuOK, MeI, THF, RT, 2 h, (v) 3-bromo-1-(3-chloropyridin-2-yl)-1H-pyrazole-5-carboxylic acid, 3-picoline, MsCl, MeCN, RT, 24 h. | |
More recently, we successfully developed a practical and scalable method for introduction of an N-cyano sulfilimine moiety into reactive functional group-substituted thioanisols.51,52 Following our thionium-mediated reaction method, the desired N-cyano sulfilimine chlorantraniliprole 1 was successfully synthesized (Scheme 2).65 It should be highlighted that this practical synthetic method of N-cyano sulfilimine 1 is column chromatography-free, with high yield, and performed on a 10 g scale.
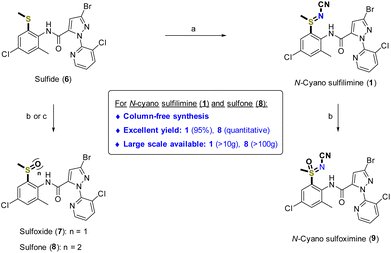 |
| Scheme 2 (a) Thionium-mediated synthesis of N-cyano sulfilimine chlorantraniliprole 1, reagents and conditions: PhI(OAc)2, H2NCN, DMF, 0 °C, 1 h; (b) synthesis of sulfoxide 7 and sulfoximine 9, reagents and conditions: mCPBA, K2CO3, MeOH; (c) synthesis of sulfone 8, reagents and conditions: MMPP·6H2O, MeOH/CH2Cl2. | |
To study the effect of IHB by means of introducing different functional groups, organosulfur group-substituted chlorantraniliprole derivatives (7–9) were also prepared (Scheme 2). For synthesis of 2-sulfoxide 7 and -sulfone 8, oxidation methods using mCPBA and MonoPeroxyPhthalate hexahydrate (MMPP·6H2O) easily provided the desired compounds 7 and 8, respectively. Oxidation of sulfilimine 1 readily produced the N-cyano sulfoximine 9 with an excellent yield. The column chromatography-free strategy was also successfully applied to prepare sulfone-substituted derivative 8. In addition, oxidation of sulfide 1 with MMPP·6H2O was suitable to manufacture a large amount of highly pure compound 8.
Intramolecular hydrogen bonding
1H NMR analysis and pKa value measurements were employed to investigate IHBs in N-cyano sulfilimine chlorantraniliprole 1 and a series of organo sulfur-substituted chlorantraniliprole derivatives 7–9. To determine the intramolecular interactions between organosulfur functionalities and the amide moiety, CO–NH (amide) proton chemical shift of each compound were divided by the corresponding pKa value.66 As shown in Fig. 3, organosulfur derivatives 1 and 7–9 appeared to have stronger interaction than the diamide compound chlorantraniliprole. In particular, S(IV) compounds 1 and 7 (highlighted in blue) exhibited superior hydrogen bonding interactions to the S(VI) compounds 8 and 9 (highlighted in red) and were slightly affected by the polarity of NMR solvents. These results clearly imply that sulfoxide 7 and sulfilimine 1 had a strong hydrogen bonding properties.
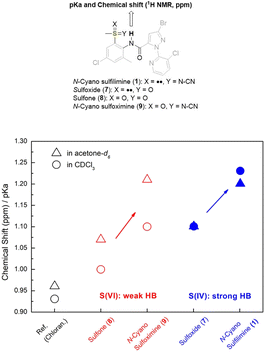 |
| Fig. 3 1H NMR and pKa analyses of organosulfur compounds 1 and 7–9. | |
The FT-IR spectra of organosulfur compounds 1 and 7–9 were illustrated in Fig. 4. The spectrum of each compound showed different spectroscopic characteristics at 1660–1700 cm−1 (amide C
O bond) and 3100–3400 cm−1 (amide N–H bond). If strong hydrogen bonding was present, the absorbance peaks of the C
O and N–H bonds tended to appear at small wavenumbers (cm−1).67 Consequently, the FT-IR study suggested that S(IV) compounds 1 and 7 were the best moieties for the formation of IHB.
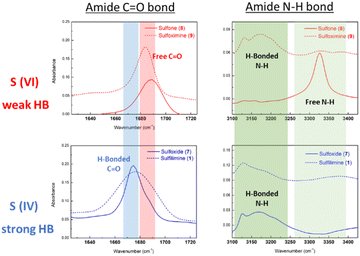 |
| Fig. 4 FT-IR spectra of the organosulfur derivatives 1 and 7–9. | |
Permeability
The synthesized organosulfur compounds 1 and 7–9 were evaluated for their permeability using parallel artificial membrane permeability assay (PAMPA). Interestingly, a similar trend was observed that the S(IV) compounds possessed superior properties to S(VI) compounds. As shown in Fig. 5, N-cyano sulfilimine 1, S(IV) compound, displayed the highest permeability. It was clearly demonstrated that the presence of a sulfur–nitrogen ylide in the ionic form of N-cyano sulfilimine could increase the permeability of the molecule. For physicochemical properties of organosulfur compounds (1, 7–9) such as equilibrium solubility, pKa, logP, and PAMPA values are reported in supplemental Table S1.†
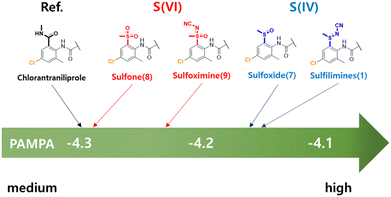 |
| Fig. 5 Comparison of experimental PAMPA permeability values of organosulfur compounds 1 and 7–9, and chlorantraniliprole. | |
Potency
N-Cyano sulfilimine chlorantraniliprole 1 and a series of organosulfur-substituted chlorantraniliprole derivatives 7–9 were measured for their insecticidal activities against the third instar larvae of Spodoptera litura according to the reported leaf-dip method.68 Importantly, an N-cyano sulfilimine 1 showed excellent activity with a high inhibition of feeding behavior (eating area: 0–5%, ref.: 0–5%, Table 1) (for images, please see the ESI†). According to the data in Table 1, the N-cyano sulfilimine group could be considered an amide bioisostere.
Table 1 Insecticidal activities of N-cyano sulfilimine chlorantraniliprole 1 and a series of organo sulfur-substituted chlorantraniliprole derivatives 7–9 against the 3rd instar larvae of Spodoptera litura
Entry |
Derivatives |
Against the 3rd instar larvae of Spodoptera litura |
Functionality |
Larvicidal activity (%) |
Eating area (%) |
Concentration (ppm) |
At time (h) |
96 h |
72 h |
96 h |
1 |
N-Cyano sulfilimine (1) |
12.5 |
100 |
100 |
0–5 |
6.25 |
100 |
100 |
0–5 |
2 |
Sulfoxide (7) |
50 |
93.3 |
100 |
5–10 |
3 |
Sulfone (8) |
12.5 |
96.7 |
100 |
0–5 |
6.25 |
96.7 |
100 |
0–5 |
4 |
N-Cyano sulfoximine (9) |
50 |
10 |
16.7 |
10–30 |
5 |
Chlorantraniliprole |
12.5 |
96.7 |
100 |
0–5 |
6.25 |
90 |
100 |
0–5 |
Field test
An open-field test was conducted to evaluate the efficacy of N-cyano sulfilimine chlorantraniliprole 1 against Spodoptera litura and Diamondback moth (Fig. 6, for a description of the tests, please see the ESI†). High control of pests was observed at least 3 days after treatment. The consistent high activity of N-cyano sulfilimine 1 was assumed to be due to its high permeability, good water solubility and relatively low logP, leading to high systemicity in plants and good distribution in the soil.69
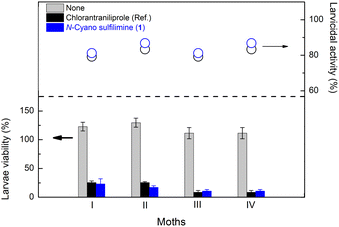 |
| Fig. 6 An open-field test with N-cyano sulfilimine chlorantraniliprole 1 [(I) Diamondback moth (after 3 days), (II) Diamondback moth (after 7 days), (III) Spodoptera litura (after 3 days), (IV) Spodoptera litura (after 7 days)]. | |
Conclusions
In summary, the studies described here demonstrated that the N-cyano sulfilimine group comprised a viable new surrogate for the amide moiety with potential applications in bioactive molecule design. The replacement of an amide bond with an N-cyano sulfilimine moiety could successfully produce the compound 1 which showed the permeability enhancement with an increase in hydrogen bond strength. N-Cyano sulfilimine chlorantraniliprole 1 displayed high activity against a range of insects in the laboratory and in open-field tests. As a result, the N-cyano sulfilimine could be considered as a valuable addition to the existing amide bond isosteres.
Conflicts of interest
There are no conflicts to declare.
Acknowledgements
This research was supported by the Korea Drug Development Fund funded by Ministry of Science and ICT, Ministry of Trade, Industry and Energy, and Ministry of Health and Welfare (HN21C1078, Republic of Korea), by KRICT (SI2231-30, TS171-09R, and TS211-07R), and by the Korean government (2019R1A2C2087018 and 2022R1A6C101A751). This research was funded by the Ministry of Trade, Industry and Energy, Republic of Korea, grant number 10052734 and 10077494.
Notes and references
- G. A. Patani and E. J. LaVoie, Chem. Rev., 1996, 96, 3147 CrossRef CAS PubMed.
- N. A. Meanwell, J. Med. Chem., 2011, 54, 2529 CrossRef CAS PubMed.
- S. Kumari, A. V. Carmona, A. K. Tiwari and P. C. Trippier, J. Med. Chem., 2020, 63, 12290 CrossRef CAS PubMed.
- A. K. Ecker, D. A. Levorse, D. A. Victor and M. J. Mitcheltree, ACS Med. Chem. Lett., 2022, 13, 964 CrossRef CAS PubMed.
- M. P. Huestis and J. A. Terrett, Nat. Chem., 2022, 14, 120 CrossRef CAS PubMed.
- V. Pattabiraman and J. Bode, Nature, 2011, 480, 471 CrossRef CAS PubMed.
- R. M. de Figueiredo, J. S. Suppo and J. M. Campagne, Chem. Rev., 2016, 116, 12029 CrossRef CAS PubMed.
- L. Pauling, J. Am. Chem. Soc., 1931, 53, 3225 CrossRef CAS.
- J. R. Cabrero-Antonino, R. Adam, V. Papa and M. Beller, Nat. Commun., 2020, 11, 3893 CrossRef PubMed.
- C. Zhao, K. P. Rakesh, L. Ravidar, W.-Y. Fang and H.-L. Qin, Eur. J. Med. Chem., 2019, 162, 679 CrossRef CAS PubMed.
- P. Devendar and G.-F. Yang, Top. Curr. Chem., 2017, 375, 82 CrossRef PubMed.
- K. A. Scott and J. T. Njardarson, Top. Curr. Chem., 2018, 376, 5 CrossRef PubMed.
- D. Leusser, J. Henn, N. Kocher, B. Engels and D. Stalke, J. Am. Chem. Soc., 2004, 126, 1781 CrossRef CAS PubMed.
- F. Pichierri, Chem. Phys. Lett., 2010, 487, 315 CrossRef CAS.
- L. Craine and M. Raban, Chem. Rev., 1989, 89, 689 CrossRef CAS.
- E. A. Ilardi, E. Vitaku and J. T. Njardarson, J. Med. Chem., 2014, 57, 2832 CrossRef CAS PubMed.
- J. J. Petkowski, W. Bains and S. Seager, J. Nat. Prod., 2018, 81, 423 CrossRef CAS PubMed.
- C. Zhao, K. P. Rakesh, L. Ravidar, W.-Y. Fang and H.-L. Qin, Eur. J. Med. Chem., 2019, 162, 679 CrossRef CAS PubMed.
- H. Mutlu and P. Theato, Macromol. Rapid Commun., 2020, 41, 2000181 CrossRef CAS PubMed.
- T. L. Gilchrist and C. J. Moody, Chem. Rev., 1977, 77, 409 CrossRef CAS.
- S. Oae, Sulfoxides and Sulfilimines, Organic Chemistry of Sulfur, Plenum Press, New York, 1977, p. 394 Search PubMed.
- N. Furukawa and S. Oae, Ind. Eng. Chem. Prod. Res. Dev., 1981, 20, 260 CrossRef CAS.
- V. Bizet, C. M. M. Hendriks and C. Bolm, Chem. Soc. Rev., 2015, 44, 3378 RSC.
- M. Klein and S. R. Waldvogel, Angew. Chem., Int. Ed., 2021, 60, 23197 CrossRef CAS PubMed.
- S. Zhou, Z. Jia, L. Xiong, T. Yan, N. Yang, G. Wu, H. Song and Z. Li, J. Agric. Food Chem., 2014, 62, 6269 CrossRef CAS PubMed.
- S. Zhou, Y. Gu, M. Liu, C. Wu, S. Zhou, Y. Zhao, Z. Jia, B. Wang, L. Xiong, N. Yang and Z. Li, J. Agric. Food Chem., 2014, 62, 11054 CrossRef CAS PubMed.
- S. Zhou, T. Yan, Y. Li, Z. Jia, B. Wang, Y. Zhao, Y. Qiao, L. Xiong, Y. Li and Z. Li, Org. Biomol. Chem., 2014, 12, 6643 RSC.
- X. Hua, W. Mao, Z. Fan, X. Li, F. Ji, G. Zong, H. Song, J. Li, L. Zhou, L. Zhou, X. Liang, G. Wang and X. Chen, Aust. J. Chem., 2014, 67, 1491 CrossRef CAS.
- K. Koerber, J. Wach, F. Kaiser, M. Pohlman, P. Deshmukh, D. L. Culbertson, W. D. Rogers, K. Gunjima, M. David, F. J. Braun and S. Thompson, Method of controlling ryanodine–modulator insecticide resistant insect, (BASF SE), WO Patent WO2014053406A1, 2014 Search PubMed.
- X. Yu, Y. Zhang, Y. Liu, Y. Li and Q. Wang, J. Agric. Food Chem., 2019, 67, 4224 CrossRef CAS PubMed.
- P. Mäder and L. Kattner, J. Med. Chem., 2020, 63, 14243 CrossRef PubMed.
- U. Lücking, Angew. Chem., Int. Ed., 2013, 52, 9399 CrossRef PubMed.
- J. A. Sirvent and U. Lücking, ChemMedChem, 2017, 12, 487 CrossRef CAS PubMed.
- U. Lücking, Org. Chem. Front., 2019, 6, 1319 RSC.
- Y. Zhu, M. R. Loso, G. B. Watson, T. C. Sparks, R. B. Rogers, J. X. Huang, B. C. Gerwick, J. M. Babcock, D. Kelley, V. B. Hegde, B. M. Nugent, J. M. Renga, I. Denholm, K. Gorman, G. J. DeBoer, J. Hasler, T. Meade and J. D. Thomas, J. Agric. Food Chem., 2011, 59, 2950 CrossRef CAS PubMed.
- J. M. Babcock, C. B. Gerwick, J. X. Huang, M. R. Loso, G. Nakamura, S. P. Nolting, R. B. Rogers, T. C. Sparks, J. Thomas, G. B. Watson and Y. Zhu, Pest Manage. Sci., 2011, 67, 328 CrossRef CAS PubMed.
- G. B. Watson, M. R. Loso, J. M. Babcock, J. M. Hasler, T. J. Letherer, C. D. Young, Y. Zhu, J. E. Casida and T. C. Sparks, Insect Biochem. Mol. Biol., 2011, 41, 432 CrossRef CAS PubMed.
- C. Longhurst, J. M. Babcock, I. Denholm, K. Gorman, J. D. Thomas and T. C. Sparks, Pest Manage. Sci., 2013, 69, 809 CrossRef CAS PubMed.
- K. M. Foote, J. W. M. Nissink, T. McGuire, P. Turner, S. Guichard, J. W. T. Yates, A. Lau, K. Blades, D. Heathcote, R. Odedra, G. Wilkinson, Z. Wilson, C. M. Wood and P. J. Jewsbury, J. Med. Chem., 2018, 61, 9889 CrossRef CAS PubMed.
- G. Siemeister, U. Luecking, A. M. Wengner, P. Lienau, W. Steinke, C. Schatz, D. Mumberg and K. Ziegelbauer, Mol. Cancer Ther., 2012, 11, 2265 CrossRef CAS PubMed.
- N. A. Meanwell, J. Med. Chem., 2011, 54, 2529 CrossRef CAS PubMed.
- N. Pemberton, H. Garden, E. Evertsson, E. Bratt, M. Lepistö, P. Johannesson and P. H. Svensson, ACS Med. Chem. Lett., 2012, 3, 574 CrossRef CAS PubMed.
- C. Ballatore, D. M. Huryn and A. B. Smith III, ChemMedChem, 2013, 8, 385 CrossRef CAS PubMed.
- N. Nishimura, M. H. Norman, L. Liu, K. C. Yang, K. S. Ashton, M. D. Bartberger, S. Chmait, J. Chen, R. Cupples and C. Fotsch, J. Med. Chem., 2014, 57, 3094 CrossRef CAS PubMed.
- S. R. Borhade, R. Svensson, P. Brandt, P. Artursson, P. I Arvidsson and A. Sandstroem, ChemMedChem, 2015, 10, 455 CrossRef CAS PubMed.
- J. E. G. Kemp, D. Ellis and M. D. Closier, Tetrahedron Lett., 1979, 39, 3781 CrossRef.
- O. G. Mancheño and C. Bolm, Org. Lett., 2007, 9, 2951 CrossRef PubMed.
- Y. Han, K. Xing, J. Zhang, T. Tong, Y. Shi, H. Cao, H. Yu, Y. Zhang, D. Liu and L. Zhao, E. J. Med. Chem., 2021, 209, 112885 CrossRef CAS PubMed.
- H. J. Lim, W. H. Lee and S. J. Park, Molecules, 2019, 24, 3451 CrossRef PubMed.
- S. J. Park, H. J. Lim and B. T. Kim, Pyrazole carboxamide compound containing organosulfur group and pesticide composition containing pyrazole carboxamide compound, WO Patent WO2019156425 A1, 2019 Search PubMed.
- S. M. Kim, O.-Y. Kang, H. J. Lim and S. J. Park, ACS Omega, 2020, 5, 10191 CrossRef CAS PubMed.
- I. S. Oh, Y. J. Seo, J. Y. Hyun, H. J. Lim, D.-H. Lee and S. J. Park, ACS Omega, 2022, 7, 2160 CrossRef CAS PubMed.
- X. Y. Chen, H. Buschmann and C. Bolm, Synlett, 2012, 23, 2808 CrossRef CAS.
- A.-D. Steinkamp, N. Seling, S. Lee, E. Boedtkjer and C. Bolm, Med. Chem. Commun., 2015, 6, 2163 RSC.
- T. Horn, W. Bettray, U. Noll, F. Krauskopf, M.-R. Huang, C. Bolm, A. J. Slusarenko and M. C. H. Gruhlke, Antioxidants, 2020, 9, 1086 CrossRef CAS PubMed.
- G. Caron, J. Kihlberg and G. Ermondi, Med. Res. Rev., 2019, 39, 1707 CrossRef CAS PubMed.
- D. Herschlag and M. M. Pinney, Biochemistry, 2018, 57, 3338 CrossRef CAS PubMed.
- A. Alex, D. S. Millan, M. Perez, F. Wakenhut and G. A. Whitlock, Med. Chem. Commun., 2011, 2, 669 RSC.
- B. Over, P. McCarren, P. Artursson, M. Foley, F. Giordanetto, G. Groenberg, C. Hilgendorf, M. D. Lee, P. Matsson, G. Muncipinto, M. Pellisson, M. W. W. Perry, R. Svensson, J. R. Duvall and J. Kihlberg, J. Med. Chem., 2014, 57, 2746 CrossRef CAS PubMed.
- A. Jeanguenat, Chapter 36 Diamide Insecticides as Ryanodine Receptor Activators, in Bioactive Carboxylic Compound Classes: Pharmaceuticals and Agrochemicals, ed. C. Lamberth and J. Dinges, Wiley-VCH Verlag GmbH & Co. KGaA, 1st edn, 2016 Search PubMed.
- S. J. Park, Sulfilimine- and Sulfoximine-Based Bioactives: Syntheses, COX Inhibition, and Anticancer Activity, PhD Thesis, RWTH Aachen University, Aachen, Germany, 2013 Search PubMed.
- A. Tsuruoka, Y. Kaku, H. Kakinuma, I. Tsukada, M. Yanagisawa, K. Nara and T. Naito, Chem. Pharm. Bull., 1998, 46, 623 CrossRef CAS PubMed.
- S. J. Hodson, M. J. Bishop, J. D. Speake, F. Navas, D. T. Garrison, E. C. Bigham, D. L. Saussy Jr, J. A. Liacos, P. E. Irving, M. J. Gobel and B. W. Sherman, J. Med. Chem., 2002, 45, 2229 CrossRef CAS PubMed.
- S. Y. Chang, J. N. Heo, H. Lee, H. J. Lim, B. T. Kim, J. K. Kim and J. Kim, Diaminoaryl Derivatives Substituted by Carbamate and Pesticidal Composition Containing Same, WO Patent WO2013168967 A1, 2013 Search PubMed.
- The desired N-cyano sulfilimine 1 could only be obtained when N,N-dimethylformamide (DMF) was used as a solvent. In the case of using other solvents such as CH3CN and CH2Cl2, it could not provide the desired product 1.
- P. A. Sigala, E. A. Ruben, C. W. Liu, P. M. B. Piccoli, E. G. Hohenstein, T. J. Martínez, A. J. Schultz and D. Herschlag, J. Am. Chem. Soc., 2015, 137, 5730 CrossRef CAS PubMed.
- G. Giubertoni, O. O. Sofronov and H. J. Bakker, Commun. Chem., 2020, 3, 84 CrossRef CAS.
- According to the literature 49, 50, and 64 methods, the insecticidal assays were performed by Kyung Nong Co. Ltd, Korea, http://www.knco.co.kr/company/en_aboutus/, In detail, please see the ESI.†.
- C. Gnamm, A. Jeanguenat, A. C. Dutton, C. Grimm and D. P. Kloer, Bioorg. Med. Chem. Lett., 2012, 22, 3800 CrossRef CAS PubMed.
Footnotes |
† Electronic supplementary information (ESI) available. See DOI: https://doi.org/10.1039/d2ra06988a |
‡ Current address: Chemical Business Unit, Pharmicell Co. Ltd, 249, Gongdan-ro, Onsan-eup, Ulju-gun, Ulsan 45009, Republic of Korea. |
|
This journal is © The Royal Society of Chemistry 2023 |
Click here to see how this site uses Cookies. View our privacy policy here.