DOI:
10.1039/D2RA07231A
(Paper)
RSC Adv., 2023,
13, 1434-1445
Enzymatic hydrolysis of low temperature alkali pretreated wheat straw using immobilized β-xylanase nanoparticles
Received
14th November 2022
, Accepted 15th December 2022
First published on 5th January 2023
Abstract
A low temperature alkali (LTA) pretreatment method was used to treat wheat straw. In order to obtain good results, different factors like temperature, incubation time, NaOH concentration and solid to liquid ratio for the pretreatment process were optimized. Wheat straw is a potential biomass for the production of monomeric sugars. The objective of the current study was to observe the saccharification (%) of wheat straw with immobilized magnetic nanoparticles (MNPs). For this purpose, immobilized MNPs of purified β-xylanase enzyme was used for hydrolysis of pretreated wheat straw. Wheat straw was pretreated using the LTA method and analyzed by SEM analysis. After completion of the saccharification process, saccharification% was calculated by using a DNS method. Scanning electron micrographs revealed that the hemicellulose, cellulose and lignin were partially removed and changes in the cell wall structure of the wheat straw had caused it to become deformed, increasing the specific surface area, so more fibers of the wheat straw were exposed to the immobilized β-xylanase enzyme after alkali pretreatment. The maximum saccharification potential of wheat straw was about 20.61% obtained after pretreatment with optimized conditions of 6% NaOH, 1/10 S/L, 30 °C and 72 hours. Our results indicate the reusability of the β-xylanase enzyme immobilized magnetic nanoparticles and showed a 15% residual activity after the 11th cycle. HPLC analysis of the enzyme-hydrolyzed filtrate also revealed the presence of sugars like xylose, arabinose, xylobiose, xylotriose and xylotetrose. The time duration of the pretreatment has an important effect on thermal energy consumption for the low-temperature alkali method.
Introduction
Recently sustainable energy and biofuel production from renewable resources have attained global attention. These resources are lignocellulosic biomass, residues from wood, the agro-industry and energy crops, and are the most plentiful renewable resources on earth and require less agricultural effort for their production.1 Lignocellulose is a heterogeneous polymeric material consisting of cellulose, lignin and hemicelluloses.2 The exploitation of such renewable resources in biofuel production has developed four different generations of biofuels. The first and second generation biofuels are produced by utilizing grain or food resources and non-grain or non-food resources such as lignocellulosic biomass respectively. Third generation biofuels are produced from algal biomass that has a unique growth yield in contrast to classical lignocellulosic biomass.3,4 However, fourth-generation biofuels are produced by coupling the use of genetically engineered feedstock and genetically modified microorganisms like cyanobacteria. Cyanobacteria possess the ability to produce bioenergy at a high rate. However, the competition amongst energy crops and food has created a food-over-fuel conflict which is worsened because of higher food prices.5,6
The annual global yield of the lignocellulosic biomass including agriculture, forestry residues and agro-industries is around 100 to 500 million tons that comprise roughly half of the total global biomass production. Also its availability to massive quantities at low cost, carbohydrate content of lignocellulosic biomass is high, such a reason has encouraged the use of lignocellulosic biomass as a resustained and unique source for sugar platform based organic chemicals and fuels.7,8
Cellulose and hemicellulose are the abundant polysaccharides that are long utilized for the purpose of ethanol production. Lignin is a tough aromatic polymer that protect plant cell from damage by bacteria and fungi.9 The actual steps involved in bioethanol production from lignocellulosic biomasses are biomass pretreatment, saccharification of hemicellulose and cellulose, fermentation of sugar and product separation.10 Hence, an operative pretreatment process is essential to enhance the accessibility to hemicellulose and cellulose and also to eliminate lignin content present in the biomass.11
Varieties of physical, biological and chemical pretreatment methods are being used to remove lignins and soften the cellulosic contents which facilitate the removal of sugars from lignocellulosic biomasses. These methods possess different deficiencies that include low conversion efficiency, high energy consumption and technological impasses.12,13 The choice of a suitable pretreatment method acts an essential part in enhancing the efficiency of lignocellulosic biomasses saccharification. The features that are extreme important during chemical pre-treatment methods consists of temperature, energy requirements, solid loading and time of incubation.14
Physical methods of pretreatment of biomass include the size reduction of particles to expose more surface area and reduction in the degree of polymerization.15 Chemical methods of biomass pretreatment include various acids and alkalis treatment in which concentrated and diluted chemicals are used to expose the cellulosic contents of plant biomass.16–18 Biological pretreatment methods include the utilization of biocatalyst (microorganisms or enzymes) to dignify the lignocellulosic biomass for efficient polymerization into fermentable sugars.19 Chemical and physical methods of biomass pretreatment resulted into good yield as compared to biological methods but there are some limitations associated with these methods such as environment degradation as well as expensive equipment are required.20 On the other hand, biological methods of pretreatment are eco-friendly as well as low energy consumption but the main limitation of these include the low enzymatic activity, long time duration as well as high cost.21
Another pretreatment method of lignocellulosic biomass, is the physico-chemical method which is a combination of physical and chemical pretreatment methods. Different types of physico-chemical pretreatment methods include steam pretreatment, liquid hot water pretreatment, dilute acid treatment, ammonia explosion, lime pretreatment in combination with wet oxidative pretreatment process, organo-solvent pretreatment, carbon dioxide explosion and ionic liquid pretreatment.22 Low temperature alkali pretreatment method is another effective physico-chemical pretreatment methods widely used for the delignification of plant biomass.
Alkali pretreatment process is one of the best methods which are widely explored as it uses less energy and is relatively inexpensive. The main feature of alkali pretreatment process is the effective delignification of lignocellulose, therefore increasing the release of the residual carbohydrates.23 Another important feature of this method is the breakdown of the intermolecular hydrogen bonding between lignocellulosic materials. Alkaline pretreatment process is performed by dipping the biomass in sodium hydroxide (NaOH) under mild conditions, at moderate temperature (room temperature to 50 °C) with different incubation period.24,25
Wheat is one of the main crops of the whole world. Pakistan has rich agricultural potential with around 25 million tonnes of annual wheat production in large amount but unfortunately these residues of wheat straw burned in the fields resulting in the environmental pollution according to Pakistan economic survey, 2021. Wheat straw is abundant and inexpensive that could be used as source for the renewable energy.26
The objective of this work was to analyze various factors of pretreatment like time of incubation, NaOH concentration and solid to liquid (S/L) ratio resulted in increased enzymatic saccharification of wheat straw by utilization of β-xylanase enzyme immobilized with magnetic nanoparticles. The reusability aspect of immobilized β-xylanase enzyme is a main novelty and concern for industrial applicability. Thus, the useful lifetime of immobilized enzyme as a novelty in this research work was assessed by subjecting the mixture to 11 successive cycles. It also present many advantages of the immobilized enzyme over enzymes in solution, including higher stability, economic convenience, and the possibility to be easily removed from the reaction mixture leading to pure product isolation.
Methodology
Materials
The wheat straw was collected from agricultural sites of Lahore, Pakistan. Wheat straw was dried and grind using grinder named as Thomas-Wiley Mini Mill fitted with a 40-mesh screen to separate the fine particles (Model 3383-L10 Arthur H. Thomas Co., Philadelphia, PA, USA). About 1 g biomass (wheat straw) was grinded. Grinding of wheat straw was carried out for 10 minutes. Analytical grade chemicals were used.
Cloning and expression of β-xylanase gene in pET-21a(+) and its purification
β-Xylanase gene from T. naphthophila was cloned and expressed into E. coli BL21(+)-xylanase-IIB previously.27 Double restricted pET-21a(+) vector and β-xylanase with HindIII and NdeI were ligated with T4 DNA ligase enzyme and transformed into the competent cells of E. coli BL21 (DE3). After transformation, colonies were selected randomly and tested for the presence of ligated product (pET-21a(+)/β-xylanase gene) through colony PCR as well as double restriction of isolated recombinant plasmid. The cloned E. coli BL21(+)-xylanase-IIB strain was grown in LB broth in the presence of isopropyl β-d-1-thiogalactopyranoside (IPTG) of about 0.5 mM IPTG and incubated at 37 °C for 4 hours. The optimized conditions of IPTG concentration, time of induction, temperature and pH factors were already studied.27 The inoculated culture of E. coli cells were centrifuged for 10 minutes at 10
000 rpm and the pellet was suspended into sodium citrate phosphate buffer (50 mM, pH 7.0). E. coli cells further go through 30 cycles of sonication, each cycle with 60% amplitude with 30 seconds on/off turn in a sonication system at 4 °C (Bandelin, Sonoplus). The sonicated sample was put on ice for 30 minutes and centrifuged at 10
000 rpm at 4 °C for 15 minutes.
The β-xylanase produced was purified using heat treatment method for 1 hour at 70 °C and immobilized metal anionic chromatography technique using Protino® Ni-TED kit.
Immobilization of recombinant β-xylanase enzyme on MNPs
β-Xylanase enzyme immobilization on magnetic nanoparticles (MNPs) was also performed and presented in previous study.28 Magnetic nanoparticles were obtained from Dr Muller, Germany. β-Xylanase enzyme was immobilized onto MNPs by following the method of Jordan et al.29 with some modifications. For β-xylanase binding, magnetic nanoparticles (50 mg) were transferred into 5 ml (8 mg ml−1) of 1-(3-dimethylaminopropyl)-3-ethylcarbodiimide hydrochloride (EDC). The reaction mixture was allowed to sonicate for 3 minutes and then incubated on ice for 30 minutes. 1 ml of enzyme solution (0–25 mg ml−1 in water) was then added and followed with sonication for an additional 3 minutes. The reaction contents were again sonicated for 3 minutes and incubated at 4 °C for 2 hours. The reaction contents were sonicated repeatedly for 3 minutes after every 1 hour of interval until uniform dispersion was achieved. After complete dispersion, the reaction contents were sonicated and incubated at 30 °C for 10 minutes. A strong magnet was used to isolate the magnetic nanoparticles immobilized with β-xylanase. MNPs were washed with distilled water and then enzyme activity was estimated. About 1 minute after the application of magnetic field, magnetic nanoparticles separated. And the magnet was obtained from a local market in Lahore, Pakistan and then checked the magnetic field that was between 40–50 kOe.
Enzyme activity assay
The enzyme assay was carried out by utilizing the method of Miller30 using dinitrosalicylic acid (DNS) that was specific for reducing sugar. Xylanase enzyme activity was resolved through mixing the enzyme with xylan (1%) in citrate–phosphate buffer (50 mM, pH 7.0) at 80 °C. Reducing sugar was analyzed by observing with spectrophotometer at optical density of 540 nm. Xylose was used as standard. One unit of enzyme activity was defined as “one enzyme unit that is needed to liberate one μmole of reducing sugar per minute from substrate under standard conditions of enzyme assay”.
Pretreatment of biomass
In this study, pretreatment of lignocellulosic biomass was performed by following our previous methods with some modifications.31,32 Optimization of alkali pretreatment process includes parameters like temperature, incubation time, NaOH concentration and solid–liquid ratio. The pretreatment of biomass was performed in a 250 ml conical flask placed in a shaking incubator with a rotation speed of 200 rpm. 2 g wheat straw biomass was weighed and put in a 250 ml conical flask in a ratio of 1/10 S/L immersed in a solution of 6% (w/v) NaOH. The contents were incubated for 72 hours at 200 rpm at 30 °C. The biomass was then filtered, washed with double distilled water and set to dry for overnight at room temperature.
Optimization of alkali pretreatment process
In order to obtain the good results of alkali pretreatment of biomass, various factors were optimized. The effect of different temperatures (25–45 °C), duration (24–96 hours), different NaOH concentration (2–10%) and solid/liquid ratios (1/5–1/20) of pretreatment was determined by incubating the wheat straw at these conditions. It was performed in one factor at a time. First, the effect of pretreatment temperature (25–40 °C) on the wheat straw was observed using 48 hours, 4.0% NaOH and 1/10 for the S/L ratio. Further, wheat straw was treated with different concentrations of best pretreatment time (24–96 hours) and S/L ratios (w/v) of 1/5, 1/10, 1/15 and finally the different concentrations of NaOH (2.0%, 4.0%, 6.0%, 8.0% and 10.0% (w/v)) was investigated using the other optimized conditions. The total reducing sugars obtained was calculated by dinitrosalicylic acid (DNS) method.30 All reactions were run in triplicate. Removal of lignin and hemicellulose was also determined reported.
SEM analysis of the pretreated samples
To evaluate the changes in the structure of untreated, pretreated and enzymatic-hydrolyzed pretreated wheat straw, SEM analysis was performed (MAIA3, Tescan). The SEM analysis was performed in the Bartin University, Turkey. Before SEM analysis, a thin layer of gold was layered on all samples in order to avoid any degradation and fiber conductivity and to promote the emission of secondary electrons so that the specimen conducts evenly, and to provide a homogeneous surface for imaging.
Reusability of immobilized MNPs
In a 250 ml conical flask, the process of saccharification was performed. Pretreated plant biomass (wheat straw 0.5 g) was added along with immobilized magnetic nanoparticles and incubated at 50 °C. Total reaction volume of experimental flask (25 ml of sodium citrate phosphate buffer (50 mM, pH 7.0)) was placed in a shaking incubator for 6 hours at 150 rpm. Pretreated biomass was hydrolyzed using immobilized magnetic nanoparticles. Tetracycline (10 μg ml−1) was added in each flask in order to prevent any contamination by microorganisms. All experiments were run in triplicate. A control was also run parallel to the experiment. Miller method was used to evaluate the reducing sugars released during saccharification process. After the completion of process, MNPs were isolated using an external magnetic source. Immobilized MNPs then again used in saccharification with pre-treated wheat straw. The β-xylanase-MNPs were used repetitively in saccharification. The reaction was continued until enzyme activity was significantly reduced.
HPLC analysis of wheat straw filtrate after saccharification
The hydrolysis of pretreated wheat straw under optimal standard conditions was observed using HPLC utilizing Hi-plex H column. Product details of Hi-plex H column with an UltiMate 3000 system (Agilent, Santa Clara, US) is that this HPLC system uses water or dilute acid in isocratic conditions and up to 30% acetonitrile as a modifier. The particle size of this system is 10 μM. About 30 μl filtrate sample was inserted into the system. HPLC was using grade water as the mobile phase having flow rate of about 1 ml min−1 with a retention time of about 20 minutes at room temperature. Carbohydrates including monosaccharide and disaccharides were examined with a RefractoMax 520 refractive index detector.
Results
Expression of recombinant enzyme
The isolated recombinant plasmid was restricted and colony PCR to confirm the cloning process (Fig. 1). The recombinant β-xylanase enzyme activity was calculated in the intracellular fraction to be 4.5 ± 0.23 U ml−1 min−1. Total proteins were estimated to be 4.25 ± 0.27 mg ml−1.
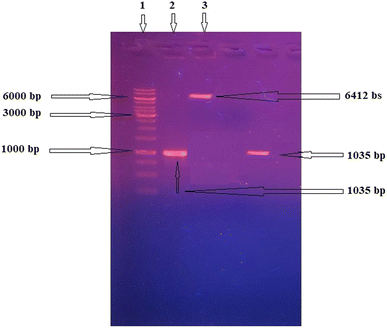 |
| Fig. 1 Amplified T. naphthophila β-xylanase gene is shown on agarose gel; DNA marker in lane 1, amplified β-xylanase gene in lane 2, single restricted recombinant pET-21a(+) with β-xylanase gene in lane 3 and β-xylanase product after colony PCR in lane 4. | |
Immobilization of β-xylanase enzyme on MNPs
β-Xylanase enzyme was immobilized onto magnetic nanoparticles through establishment of covalent bond between enzyme and magnetic nanoparticles through 1-(3-dimethylaminopropyl)-3-ethylcarbodiimide hydrochloride (EDC). These magnetic nanoparticles exhibited an additional benefit as these particles are recovered by an external magnetic source and reused several times. MNPs were washed using distilled water and enzyme activity was calculated to be 6.5 U ml−1 min−1.
Wheat straw composition
The general composition of wheat straw is mentioned in Table 1. The lignin exhibited an important part of the total biomass raw material. The contents of cellulose and hemicelluloses in wheat straw are 22–39% and 23–24%. Lignin amount in wheat straw is about 11–25%.
Table 1 Composition of raw wheat straw
Components |
This study (raw wheat straw) (%) |
This study (pretreated wheat straw) (%) |
Values from literature (%) |
References |
Cellulose |
14 |
12.2 |
22–39 |
33 |
Hemicellulose |
21 |
18.5 |
23–24 |
34 |
Xylan |
17 |
13.56 |
19–22 |
14 |
Glucose |
12 |
9.98 |
16–40 |
35 |
Arabinan |
2.5 |
1.06 |
3.63–2.17 |
36 |
Lignin |
13 |
8.65 |
11–25 |
36 |
Ash |
1.1 |
0.6 |
1.4–1.1 |
37 |
Optimization of alkali pretreatment process
Following parameters were optimized in order to improve the pretreatment of the wheat straw.
Temperature and time
The effect of different temperatures (25–45 °C) and time periods (24–96 hours) on biomass was evaluated. Maximum total reducing sugars (3.21 mg ml−1) was calculated after pretreated biomass was incubated at 30 °C for 72 hours (Fig. 2a and b). At various temperatures and time periods, calculated sugar contents were less in comparison to the optimized conditions. In Table 2, the hemicellulose, cellulose and lignin content were determined (Table 2) in the pretreated samples according to the experimental protocols of the NREL (National Renewable Energy Laboratory).37 Increasing pretreatment temperature and time of incubation effected in higher concentrations of total reducing sugar (Fig. 2a and b). Maximum total reducing sugar (3.21 mg ml−1 ± 0.015, 3.51 ± 0.032) was observed at the highest temperature 30 °C and 72 hours of incubation respectively. However, further increase result in the decrease of total sugars. The highest lignin removal was also observed at 30 °C and 72 hours of incubation of about 37 ± 0.005 and 40 ± 0.03 respectively in pretreated solid.
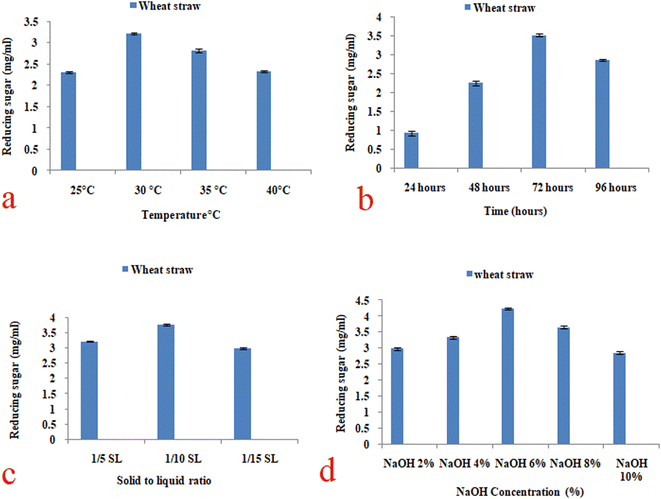 |
| Fig. 2 Optimization of various parameters to determine its effect of alkali pre-treatment on wheat straw. (a) Effect of temperature (°C) (b) effect of time (hours) (c) effect of solid to liquid ratio (d) effect of NaOH concentration (%). | |
Table 2 Experimental data on removal of cellulose, hemicellulose and lignin as means of ±SDs of three replicates
|
Hemicellulose removal (%) |
Lignin removal (%) |
Cellulose removal (%) |
Pretreatment temperature (°C): 2.0% NaOH, 48 hours, 1/5 S/L |
25 |
26 ± 0.001 |
26 ± 0.0031 |
56 ± 0.003 |
30 |
30 ± 0.004 |
37 ± 0.0054 |
61 ± 0.001 |
35 |
24 ± 0.003 |
28 ± 0.002 |
62 ± 0.021 |
40 |
22 ± 0.002 |
23 ± 0.0012 |
65 ± 0.031 |
![[thin space (1/6-em)]](https://www.rsc.org/images/entities/char_2009.gif) |
Pretreatment time (hours): 30 °C, 2.0% NaOH, 1/5 S/L |
24 |
28 ± 0.003 |
27 ± 0.0021 |
78 ± 0.005 |
48 |
30 ± 0.0021 |
31 ± 0.019 |
70 ± 0.011 |
72 |
35 ± 0.0421 |
40 ± 0.0321 |
66 ± 0.023 |
96 |
27 ± 0.0023 |
34 ± 0.0023 |
62 ± 0.003 |
![[thin space (1/6-em)]](https://www.rsc.org/images/entities/char_2009.gif) |
Solid to liquid ratio (S/L): 72 hours, 30 °C, 2.0% NaOH |
1/5 |
36 ± 0.018 |
46 ± 0.014 |
75 ± 0.041 |
1/10 |
45 ± 0.0341 |
52 ± 0.0319 |
71 ± 0.003 |
1/15 |
31 ± 0.013 |
41 ± 0.023 |
68 ± 0.037 |
![[thin space (1/6-em)]](https://www.rsc.org/images/entities/char_2009.gif) |
NaOH concentration (%): 1/10 S/L, 30 °C, 72 hours |
2 |
45 ± 0.016 |
42 ± 0.0012 |
80 ± 0.145 |
4 |
50 ± 0.178 |
55 ± 0.013 |
72 ± 0.003 |
6 |
65 ± 0.2 56 |
58 ± 0.280 |
72 ± 0.031 |
8 |
52 ± 0.145 |
53 ± 0.164 |
74 ± 0.176 |
10 |
35 ± 0.003 |
48 ± 0.004 |
81 ± 0.003 |
NaOH concentration and solid/liquid ratio
Different NaOH concentration (2–10%) and solid/liquid ratio (1/5–1/20) was used to determine the effect on pretreated biomass. Total reducing sugars were calculated after using the NaOH concentration of 6% (w/v) and at a solid/liquid ratio of 1/10 as shown in Fig. 2c and d. At other NaOH concentrations and solid/liquid ratio, the calculated sugar contents were less as compared to the optimize concentration. In Table 2, hemicellulose, cellulose and lignin contents were determined (Table 2) in the pretreated sample.37 Total reducing sugar were maximum released at 1/10 SL and 6% of NaOH of about 3.76 ± 0.03 and 4.23 ± 0.037 respectively, however, more increase in the SL ratio and NaOH concentration go to a decline for total sugar (Fig. 2c and d). The highest lignin elimination was also observed at 1/10 SL and 6% NaOH of about 52 ± 0.03 and 58 ± 0.28 respectively in pretreated solid.
At last, from the data acquired in this work, pretreatment with 6% NaOH at 1/10 S/L for 72 h would be chosen as the standard conditions to enhance the glucose yield.
SEM analysis of pretreated biomass
The wheat straw was pre-treated as stated in the methodology section. SEM was performed to evaluate the structures of untreated, pre-treated and enzyme-hydrolyzed wheat straw. Electron micrograph of native sample showed regular, highly compressed, woody, smooth and compact surface, representing the extremely well-organized surface structure (Fig. 3a), while pretreated sample revealed an irregular and uneven structure and demonstrated loosened inner zones of hemicellulose and cellulose content. Delignification of wheat straw after pretreatment leads to increased accessibility of enzyme to hemicellulose. Delignification because of sodium hydroxide pretreatment enhanced the surface area that increased the convenience for enzyme as well as resulted in the dissociation of fibers because of loosening of the fibrous network (Fig. 3b).
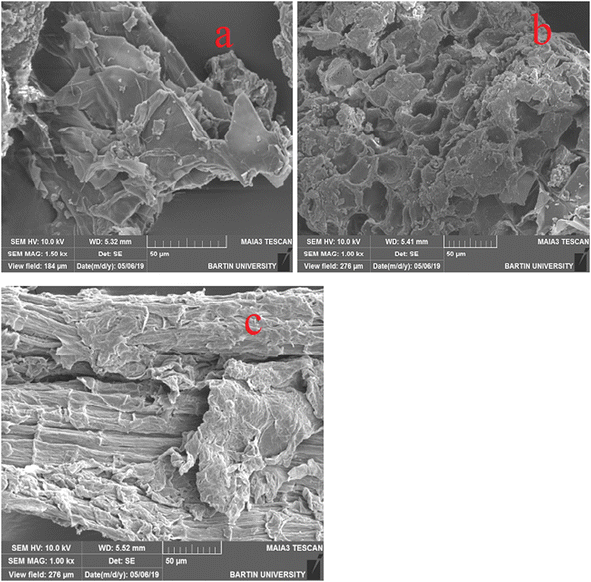 |
| Fig. 3 Scanning electron micrographs of (a) untreated wheat straw (b) pretreated wheat straw (c) enzyme-hydrolyzed wheat straw. | |
Enzyme-hydrolyzed sample revealed the good enzyme digestibility of the hemicellulose contents. The accessible surface area was improved after alkali pretreatment that is encouraging for action of immobilized β-xylanase enzyme. The rigorous degradation seen on the surface of the enzyme-hydrolyzed sample, which represents the lignin frame, was again reduced and more cracks and holes were seen on the sample surface demonstrating that most of the hemicellulose was degraded by the β-xylanase enzyme as shown in Fig. 3c. Alkali pretreatment improved the surface area of biomass that present enzyme with better convenience for saccharification of hemicellulosic content of plant biomass.
Reusability of immobilized MNPs in saccharification
The reusability of the immobilized MNPs (with β-xylanase) was examined. Maximum saccharification potential of enzyme immobilized MNPs was calculated to be 20.61% with wheat straw. It was observed that immobilized MNPs could be reused for 11th time for saccharification process. Residual activity up to 81% was calculated after 4th time of usage of immobilized MNPs. However, 72% residual activity was present after 5th cycle of usage. The residual activity was dropped to 15% after 11th time use of MNPs. The saccharification potential of immobilized MNPs was observed to be 19.48% after 5th cycle. Table 3 represents the saccharification potential and residual activity of MNPS-β-xylanase enzyme.
Table 3 Saccharification potential of immobilized MNPs
Reuse of immobilized MNPs in saccharification |
Residual activity (%) |
Saccharification (%) |
Reducing sugar (mg ml−1) |
1st cycle |
100 |
20.61 |
4.58 |
2nd cycle |
96 |
20.46 |
4.43 |
3rd cycle |
90 |
20.18 |
4.37 |
4th cycle |
81 |
19.79 |
4.19 |
5th cycle |
72 |
19.48 |
4.06 |
6th cycle |
64 |
19.21 |
3.97 |
7th cycle |
57 |
18.72 |
3.91 |
8th cycle |
39 |
18.19 |
3.90 |
9th cycle |
27 |
17.60 |
3.42 |
10th cycle |
23 |
17.23 |
3.10 |
11th cycle |
15 |
16.49 |
2.89 |
HPLC analysis of filtrate after saccharification process
Existence of different reducing sugars in filtrate of enzyme-hydrolysate of sodium hydroxide pretreated wheat straw was analyzed by HPLC that visibly showed the releasing of high quantity of xylotrioses 3.75 mg, xylose 5.1 mg, xylotriose 4.8 mg, arabinose 1 mg and xylobiose 1.24 mg etc. Improved saccharification of pretreated wheat straw by immobilized MNPs is because of the elimination of lignin and hemicellulose after sodium hydroxide pretreatment. This obviously exposed the existence of lignin as barricade for the enzymatic hydrolysis of hemicellulose. Arabinose and xylose were the predominant monosaccharide sugars in wheat straw according to the HPLC analysis. The ratio of produced xylose and arabinose from pretreated wheat straw is approximately 5.1
:
1.
Discussion
In this research work, recombinant thermostable β-xylanase gene from Thermotoga naphthophila was cloned and expressed in E. coli BL21(+)-xylanase-IIB and then immobilized on to magnetic nanoparticles.27,28 The formation of stable activated MNPs-β-xylanase complex was prepared due to the formation of an amide bond between an amine group on the magnetic nanoparticle surface (conversion of the C–O to C–N) and the carboxyl group on the β-xylanase enzyme. Catalytic activity of the MNPs bound enzyme was examined by DNS method.30
The NaOH is considered as one of the strong bases which solubilizes lignin and hemicellulose significantly under definite conditions.38–40 By using the NaOH pretreatment process, it is verified that better efficiency of saccharification was achieved as compared to other alkaline pretreatment processes. NaOH efficiently breaks the bond between lignin and hemicellulose in lignin carbohydrate complexes (LCC); particularly, the ester and ether linkages in the LCC complex are broken. Sodium hydroxide is also utilized for breakdown of the carbon-to-carbon (C–C) and ester linkages in lignin molecules (ferulic acid).34,41,42
Alkali (NaOH) can deform cell wall polymers by cleaving hydrogen and covalent bonds, and eliminate lignin competently.43,44 Surface area of wheat straw biomass is also increased by removal of lignin and hemicelluloses. After pretreatment, hemicelluloses and cellulose are hydrolyzed into fermentable sugars.45
The advantage of usage of thermostable enzymes as a catalyst in various industrial procedures, as high temperature is often employed in many industries. Further recovery of costly enzymes used in industrial processes needs to be developed. Carriers or supportive materials for enzymes immobilization were developed recently.46,47 These materials also include nanoparticles immobilized with zinc-superoxide dismutase and superoxide dismutase enzymes through carrier molecules. Magnetic nanoparticles (MNPs) are unique in nature which can be reutilized several times.
For pretreatment of biomass, various methods are used; one is alkali treatment which is less toxic as compared to the acidic chemicals, sulfite and sulfuric acid. Alkaline pretreatment methods are performed under mild conditions at normal temperature utilizing sodium hydroxide or ammonium hydroxide. These processes eliminate the requirement of expensive chemicals and use of unique designs to manage difficult reaction conditions and corrosion.48,49 Recently, researchers have combined the alkaline method with ultrasound waves and determined the delignification after the pretreatment method for the enzyme hydrolysis of wheat waste biomass.50 In another strategy, carbonate–oxygen pretreatment was used which have several advantages like economical processes, easiness of chemical recycling and sustainable pretreatment of biomass.51,52
The structural composition of untreated wheat straw biomass is presented in Table 1. The composition is determined by accumulative work of many workers. Cellulose and hemicelluloses contents in raw wheat straw are 37, 41 and from literature wheat straw 32.28% and 45% respectively. The difference in contents could be attributed to different locations, soil conditions and different times of harvest.53
Temperature, incubation time, S/L ratio and NaOH concentration are involved in the alkali pretreatment of wheat straw method. The structural changes in the biomass during pre-treatment have great influence during the saccharification process. The present study showed that the wheat straw was effectively pretreated when incubated at 30 °C for 72 hours. Total reducing sugar determined after process of pretreatment varies in between 0.9 and 4.23 mg ml−1 at standard conditions (Fig. 1).
However, S/L ratio has an immense effect on the cellulose, lignin and hemicellulose elimination. Higher NaOH concentration and more volume of liquid resulted in carbohydrate loss, particularly cellulose; maximum hemicellulose removal (40.08%) was observed with 1/10 of S/L and 6.0% NaOH. In different studies, alkali pretreatment process mostly affected the hemicellulose solubilization as compared to cellulose.54–56 Chaudhary et al.57 also described the removal of 50% hemicellulose at 7% NaOH concentration during alkali pretreatment of S. spontaneum. Li et al.58 also reported the removal of hemicellulose content from corn stover with NaOH concentration up to 4% and S/L ratio of about 1/10.
As it is observed from Table 2, 6% NaOH pretreatment resulted in degradation of 65% hemicellulose. Similarly, lignin contents after the alkali pretreatment were relatively low, indicating that lignin was effectively removed (58%) by splitting the ester bonds. In the alkaline pretreatment, porosity of the biomass is maximum. Similar results are narrated by Zheng et al.,59 who reported alkaline pretreatment with 2% NaOH for 1 hour, lignin and hemicellulose degradation by 84% and 31.4% respectively. Xin et al.60 also reported the use of NaOH in the range of 3 to 9%, at low temperature (28 °C) up to 7 days for the rice straw delignification.
Lignin is made up of cross-linked polymers of the phenolic components; representing high degree of complexity in the molecular structure of biomass. Hence, it is obvious that breakdown of lignocellulosic content resulted in the production of different phenolic compounds present in liquid phase.34 Ho et al.61 reported the maximum delignification of the biomass, probably because of the successive oxidation reactions of the phenolic compounds after using the hydroxyl radicals produced in the hydrolysis with hydrogen peroxide.
To observe the structural changes in the wheat straw samples after pretreatment and saccharification, SEM was performed (Fig. 3a–c). The hemicellulose and lignin of the pretreated wheat straw sample was partially removed. The wheat straw became irregular in structure, and showed fiber porosity on its surface in contrast to the control wheat straw sample. These outcomes are quite related to Du et al.62 who reported the alkaline pretreatment of cotton stalk and stated that alkaline pretreatment had removed the cellulose–hemicellulose–lignin network, thus eliminating certain external fibers.
The loosening of the internal structures in the pretreated wheat straw sample increases the accessibility of immobilized β-xylanase enzyme to the cellulosic and hemicellulosic contents, thus enhancing the saccharification process and resulted in change in hemicellulose structure. Surface of the enzyme-hydrolyzed wheat straw sample indicates the efficient removal of the lignin/hemicellulose and a good enzymatic digestibility of the hemicellulose in the pre-treated wheat straw. Our findings are quite similar to the Jiang et al.63 and Zheng et al.59 who observed the changes in the reed and wheat straw respectively after the SEM analysis. Yu et al.64 also determined the SEM analysis of control and pretreated wheat straw samples after ammonium sulphite based pretreatment method and showed the better efficiency of saccharification.
Saccharification potential (20.66%) was observed when 4.52 mg ml−1 sugar was produced from pretreated wheat straw using the immobilized MNPs. It was found that immobilized enzyme-MNPs were used for 11 times in enzyme hydrolysis process. After completion of the 5th cycle, β-xylanase bound MNPs showed 72% residual activity. However, after the 7th and 8th cycle, the residual activity was reduced to 57% and 39% respectively. This fall in the activity was due to the washing process in which β-xylanase was separated from MNPs and also some MNPs were also washed away from the reaction mixture. Another researcher determined the enzymatic saccharification in hemicelluloses-rich Miscanthus species under alkali pretreatment process using 4% NaOH.65 Wu et al.66 reported the 3.5 times more saccharification potential of the rice straw as compared to the untreated rice straw. Zafar et al.67 also reported the enzymatic hydrolysis by using xylanase enzyme from Bacillus licheniformis. In another study, maximum saccharification potential was checked against sugarcane bagasse using the recombinant xylanase enzyme.68 The saccharification efficiency obtained here be compared and discussed with saccharification efficiencies measured by other researchers are enlisted in Table 4.
Table 4 Saccharification potential of different enzymes on wheat straw pretreated by different methods
S. no. |
Enzymes |
Chemical method used |
Saccharification (%) |
References |
1 |
Cloned β-xylanase |
Low-temperature alkali (LTA) |
20.66 |
This study |
2 |
Xylanase |
KOH pretreatment |
20.17 |
69 |
3 |
Cellulase |
Microwave oven |
|
80 |
4 |
Cellulase |
Dilute acid pretreatment |
17.8 |
70 |
5 |
β-Glucosidase |
Alkaline peroxide pretreated |
15.1 |
70 |
The analysis from the reusability in the present work present an estimation of the minimum number of recycles of the immobilized enzyme to make the process economically advantageous compared to the use of a native enzyme. Use of the immobilize enzyme is a prominent tool that is cost-effective mainly when applied on a large scale because of increasing enzyme stability, reusability and activity.
HPLC analysis of filtrate clearly show the release of high amount of arabinose, cellobiose, xylose, glucose, and other sugars. Increased saccharification of pretreated wheat straw using β-xylanase enzymes is because of the elimination of lignin and hemicellulose after sodium hydroxide pretreatment. This clearly indicates that lignin hinders in the enzymatic hydrolysis of lignocellulosic biomass. Lignin has been reported as the main inhibitor for cellulolytic enzymes because of irreversible binding to enzymes and non-productive matters as well as a protective covering that restrict the enzymes accessibility to cellulose.71,72 Enzymatic hydrolysis of pretreated wheat straw leads to release of monomeric sugars i.e. glucose can be used as sugar feedstock in microbial fermentation for biofuels and in further economically demanding products74 (Table 5).
Table 5 Monomeric sugar yield after saccharification analysisa
Biomass |
Glucose (mg g−1) |
Xylose (mg g−1) |
Mannose (mg g−1) |
Arabinose (mg g−1) |
Total (mg g−1) |
References |
ND = not detected. |
Wheat straw |
7.3 |
13.4 |
1.04 |
ND |
21.74 |
73 |
Sugar cane bagasse |
1.12 |
2.18 |
ND |
0.5 |
3.8 |
75 |
Rice straw |
1.75 |
1.05 |
ND |
1.25 |
4.05 |
76 |
Sago palm bark |
4.26 |
ND |
ND |
1.49 |
5.68 |
77 |
Wheat straw |
0.5 |
5.3 |
ND |
6.3 |
12.1 |
78 |
Wheat straw |
1.06 |
3.01 |
ND |
0.05 |
4.12 |
79 |
Conclusion
Alkali pretreatment method has reflected one of the most operative method since it has several benefits. These comprise operative delignification, mild reaction conditions and insignificant interaction with hemicellulose. LTA method used for pretreatment of wheat straw increase the subsequence enzymatic hydrolysis step because of its ability to disturb lignin, hemicellulose and cellulose structure. The residual activity of immobilized enzyme was 57% for 7th cycles and then from 10–11th cycles it start to decline during saccharification. About saccharification 20.61% was obtained with optimized conditions. Furthermore, it is possible to recover and reuse β-xylanase enzyme immobilized on MNPs. Alkali pretreatment process is highly selective for removal of cellulose, hemicellulose and lignin. It is expected that alkali pretreatment could play an important part in the bio-refinery industry since it can be used not merely as a pretreatment reagent but also as a process for fractionation.
Author contributions
All authors have contributed to the manuscript. Attia Hamid: original manuscript writing, conceptualization. Asma Zafar: investigation. Sabahat Latif: methodology, software analysis. Liangcai Peng: resources (provision of reagent). Yanting Wang: visualization. Iram Liaqat: formal analysis. Muhammad Sohail Afzal: validation. Ikram Ul Haq: resources (provision of instrumentation). Muhammad Nauman Aftab: supervision (writing-reviewing and editing), project administration.
Conflicts of interest
The authors declare that they have no conflict of interest.
Acknowledgements
This research was funded by the Higher Education Commission of Pakistan for Biofuel project no. 5535 and the Pak-Turk Researchers Mobility Program. Sincere gratitude is expressed to the Higher Education Commission of Pakistan (project no. 5535) and the Pak-Turk Researchers Mobility Program funded by Higher Education Commission Pakistan and Council of Higher Education Turkey.
References
- O. J. Sanchez and C. A. Cardona, Trends in biotechnological production of fuel ethanol from different feedstocks, Bioresour. Technol., 2008, 99(13), 5270–5295, DOI:10.1016/j.biortech.2007.11.013.
- C. L. Tri and I. Kamei, The improvement of sodium hydroxide pretreatment in bioethanol production from Japanese bamboo Phyllostachys edulis using the white rot fungus Phlebia sp. MG-60, Int. Biodeterior. Biodegrad., 2018, 133, 86–92, DOI:10.1016/j.ibiod.2018.06.010.
- L. Brennan and P. Owende, Biofuels from microalgae—a review of technologies for production, processing, and extractions of biofuels and co-products, Renewable Sustainable Energy Rev., 2010, 14, 557–577, DOI:10.1016/j.rser.2009.10.009.
- A. A. Modenbach and S. E. Nokes, Saccharification of biomass at high-solids loadings–a review, Biomass Bioenergy, 2013, 56, 526–544, DOI:10.1016/j.biombioe.2013.05.031.
- K. Jaisamut, L. Paulová, P. Patáková, S. Kotúčová and M. Rychtera, Effect of sodium sulfite on acid pretreatment of wheat straw with respect to its final conversion to ethanol, Biomass Bioenergy, 2016, 95, 1–7, DOI:10.1016/j.biombioe.2016.08.022.
- C. Vasmara, S. Cianchetta, R. Marchetti and S. Galletti, Biogas production from wheat straw pre-treated with hydrolytic enzymes or sodium hydroxide, Environ. Eng. Manag. J., 2017, 16(8), 1827–1835, DOI:10.30638/eemj.2017.199.
- S. Ethaib, R. Omar, M. S. Mazlina, A. D. Radiah and S. L. Zubaidi, Toward sustainable processes of pretreatment technologies of lignocellulosic biomass for enzymatic production of biofuels and chemicals: a review, BioResources, 2020, 15(4), 10063, DOI:10.15376/biores.15.4.10063-10088.
- S. Ethaib, R. Omar, M. S. Mazlina and A. D. Radiah, Comparison of sodium hydroxide and sodium bicarbonate pretreatment methods for characteristic and enzymatic hydrolysis of sago palm bark, Energy Sources, Part A, 2020, 1–11, DOI:10.1080/15567036.2020.1753857.
- W. Wang, X. Zhuang, X. Tan, Q. Wang, X. Chen, Q. Yu, W. Qi, Z. Wang and Z. Yuan, Dual effect of nonionic surfactants on improving the saccharification of lignocelluloses, Energy Fuels, 2018, 32(5), 5951–5959, DOI:10.1021/acs.energyfuels.8b00225.
- Y. L. Cha, J. Yang, S. I. Seo, G. H. An, Y. H. Moon, G. D. You, J. E. Lee, J. W. Ahn and K. B. Lee, Alkaline twin-screw extrusion pretreatment of Miscanthus with recycled black liquor at the pilot scale, Fuel, 2016, 164, 322–328, DOI:10.1016/j.fuel.2015.10.006.
- W. Wang, X. Chen, X. Tan, Q. Wang, Y. Liu, M. He, Q. Yu, W. Qi, Y. Luo, X. Zhuang and Z. Yuan, Feasibility of reusing the black liquor for enzymatic hydrolysis and ethanol fermentation, Bioresour. Technol., 2017, 228, 235–240, DOI:10.1016/j.biortech.2016.12.076.
- D. P. Maurya, A. Singla and S. Negi, An overview of key pretreatment processes for biological conversion of lignocellulosic biomass to bioethanol, Biotech, 2015, 5(5), 597–609, DOI:10.1007/s13205-015-0279-4.
- Ö. Özyürek and A. V. Heiningen, Formic acid reinforced autohydrolysis of wheat straw for high yield production of monosugars and minimal lignin precipitation, Ind. Crops Prod., 2018, 112, 320–326, DOI:10.1016/j.indcrop.2017.12.026.
- H. Liu, B. Pang, J. Zhou, Y. Han, J. Lu, H. Li and H. Wang, Comparative study of pretreated corn stover for sugar production using cotton pulping black liquor (CPBL) instead of sodium hydroxide, Ind. Crops Prod., 2016, 84, 97–103, DOI:10.1016/j.indcrop.2016.01.047.
- O. J. Sanchez and C. A. Cardona, Trends in biotechnological production of fuel ethanol from different feedstocks, Bioresour. Technol., 2008, 99, 5270–5295 CrossRef CAS PubMed.
- D. Fu, G. Mazza and Y. Tamaki, Lignin extraction from straw by ionic liquids and
enzymatic hydrolysis of the cellulosic residues, J. Agric. Food Chem., 2010, 58, 2915–2922 CrossRef CAS PubMed.
- D. Fu and G. Mazza, Optimization of processing conditions for the pretreatment of wheat straw using aqueous ionic liquid, Bioresour. Technol., 2011, 102, 8003–8010 CrossRef CAS PubMed.
- D. Fu and G. Mazza, Aqueous ionic liquid pretreatment of straw, Bioresour. Technol., 2011, 102, 7008–7011 CrossRef CAS PubMed.
- C. Sánchez, Lignocellulosic residues: biodegradation and bioconversion by fungi, Biotechnol. Adv., 2009, 27, 185–194 CrossRef PubMed.
- B. Saha, L. B. Iten, M. A. Cotta and Y. V. Wu, Dilute acid pretreatment, enzymatic saccharification, and fermentation of rice hulls to ethanol, Biotechnol. Prog., 2005, 21, 816–822 CrossRef CAS PubMed.
- M. J. Taherzadeh and K. Karimi, Pretreatment of lignocellulosic wastes to improve ethanol and biogas production: a review, Int. J. Mol. Sci., 2008, 9, 1621–1651 CrossRef CAS PubMed.
- V. B. Agbor, N. Cicek, R. Sparling, A. Berlin and D. B. Levin, Biomass pretreatment: fundamentals toward application, Biotechnol. Adv., 2011, 29(6), 675–685 CrossRef CAS PubMed.
- J. S. Kim, Y. Y. Lee and T. H. Kim, A review on alkaline pretreatment technology for bioconversion of lignocellulosic biomass, Bioresour. Technol., 2016, 199, 42–48, DOI:10.1016/j.biortech.2015.08.085.
- K. Karimi and M. J. Taherzadeh, A critical review on analysis in pretreatment of lignocelluloses: degree of polymerization, adsorption/desorption, and accessibility, Bioresour. Technol., 2016, 203, 348–356, DOI:10.1016/j.biortech.2015.12.035.
- J. Xu and J. J. Cheng, Pretreatment of switchgrass for sugar production with the combination of sodium hydroxide and lime, Bioresour. Technol., 2011, 102(4), 3861–3868, DOI:10.1016/j.biortech.2010.12.038.
- M. Sajid, M. Asad, A. Akram, M. Yasir, M. Arshad and R. Raza, Sustainability of villages through electricity from wheat straw in Pakistan, AAAFM Energy, 2020, 1(1), 27–35, DOI:10.1021/aaafmenergy.8b01488.
- A. Hamid and M. N. Aftab, Cloning, purification, and characterization of recombinant thermostable β-xylanase Tnap_0700 from Thermotoga naphthophila, Appl. Biochem. Biotechnol., 2019, 189(4), 1274–1290, DOI:10.1007/s12010-019-03068-0.
- A. Hamid, A. Zafar, I. Liaqat, M. S. Afzal, L. Peng, M. K. Rauf, I. ul Haq, A. U. Rehman, S. Ali and M. N. Aftab, Effective utilization of magnetic nano-coupled cloned β-xylanase in saccharification process, RSC Adv., 2022, 12(11), 6463–6475, 10.1039/D1RA09275H.
- J. Jordan, C. S. Kumar and C. Theegala, Preparation and characterization of cellulase-bound magnetite nanoparticles, J. Mol. Catal. B: Enzym., 2011, 68, 139–146, DOI:10.1016/j.molcatb.2010.09.010.
- G. L. Miller, Use of dinitrosalicylic acid reagent for determination of reducing sugar, Anal. Chem., 1959, 31(3), 426–428, DOI:10.1021/ac60147a030.
- A. Zafar, M. N. Aftab, A. Asif, A. Karadag, L. Peng, H. U. Celebioglu, M. S. Afzal, A. Hamid and I. Iqbal, Efficient biomass saccharification using a novel cellobiohydrolase from Clostridium clariflavum for utilization in biofuel industry, RSC Adv., 2021, 11(16), 9246–9261, 10.1039/D1RA00545F.
- E. Z. Hoşgün, D. Berikten, M. Kıvanç and B. Bozan, Ethanol production from hazelnut shells through enzymatic saccharification and fermentation by low-temperature alkali pretreatment, Fuel, 2017, 196, 280–287, DOI:10.1016/j.fuel.2017.01.114.
- F. Carvalheiro, T. Silva-Fernandes, L. C. Duarte and F. M. Gírio, Wheat straw autohydrolysis: process optimization and products characterization, Appl. Biochem. Biotechnol., 2009, 153(1), 84–93, DOI:10.1007/s12010-008-8448-0.
- B. Shrivastava, K. K. Jain, A. Kalra and R. C. Kuhad, Bioprocessing of wheat straw into nutritionally rich & digested cattle feed, Sci. Rep., 2014, 4, 6360, DOI:10.1038/srep06360.
- Y. Çöpür, A. Tozluoglu and M. Özkan, Evaluating pretreatment techniques for converting hazelnut husks to bioethanol, Bioresour. Technol., 2013, 129, 182–190, DOI:10.1016/j.biortech.2012.11.058.
- Z. Zhao, X. Chen, M. F. Ali, A. A. Abdeltawab, S. M. Yakout and G. Yu, Pretreatment of wheat straw using basic ethanolamine-based deep eutectic solvents for improving saccharification, Bioresour. Technol., 2018, 263, 325–333, DOI:10.1016/j.biortech.2018.05.016.
- N. Kontogianni, E. M. Barampouti, S. Mai, D. Malamis and M. Loizidou, Effect of alkaline pretreatments on the saccharification of wheat straw, Environ. Sci. Pollut. Res., 2019, 26(35), 35648–35656, DOI:10.1007/s11356-019-06822-3.
- A. Sluiter, B. Hames, R. Ruiz, C. Scarlata, J. Sluiter, D. Templeton and D. Crocker, Determination of structural carbohydrates and lignin in biomass, Laboratory Analytical Procedure, 2008, vol. 1617, iss. 1, pp. 1–16 Search PubMed.
- Y. Çöpür, C. Güler, C. Taşçıoğlu and A. Tozluoğlu, Incorporation of hazelnut shell and husk in MDF production, Bioresour. Technol., 2008, 99(15), 7402–7406, DOI:10.1016/j.biortech.2008.01.021.
- K. Minu, K. K. Jiby and V. V. N. Kishore, Isolation and purification of lignin and silica from the black liquor generated during the production of bioethanol from rice straw, Biomass Bioenergy, 2012, 39, 210–217, DOI:10.1016/j.biombioe.2012.01.007.
- G. Nong, Z. Zhou and S. Wang, Generation of hydrogen, lignin and sodium hydroxide from pulping black liquor by electrolysis, Energies, 2015, 9(1), 1–11, DOI:10.3390/en9010013.
- W. Wang, Q. Wang, X. Tan, W. Qi, Q. Yu, G. Zhou, X. Zhuang and Z. Yuan, High conversion of sugarcane bagasse into monosaccharides based on sodium hydroxide pretreatment at low water consumption and wastewater generation, Bioresour. Technol., 2016, 218, 1230–1236, DOI:10.1016/j.biortech.2016.07.074.
- M. Balat, Production of bioethanol from lignocellulosic materials via the biochemical pathway: a review, Energy Convers. Manage., 2011, 52(2), 858–875, DOI:10.1016/j.enconman.2010.08.013.
- L. Feng, L. Qin, Z. H. Liu, C. Y. Dong, B. Z. Li and Y. J. Yuan, Combined severity during pretreatment chemical and temperature on the saccharification of wheat straw using acids and alkalis of differing strength, Bioresour. Technol., 2014, 9(1), 24–38 CAS , ISSN: 1930-2126, http://ncsu.edu/bioresources.
- Y. Çöpür, A. Tozluoglu and Ö. Özyürek, Sodium borohydrate (NaBH4) pretreatment for efficient enzymatic saccharification of wheat straw, Bioresour. Technol., 2012, 107, 258–266, DOI:10.1016/j.biortech.2011.12.076.
- A. Salimi, A. Noorbakhsh, H. A. Rafiee-Pour and H. Ghourchian, Direct Voltammetry of Copper, Zinc-Superoxide Dismutase Immobilized onto Electrodeposited Nickel Oxide Nanoparticles: Fabrication of Amperometric Superoxide Biosensor, Electroanalysis, 2011, 23(3), 683–691, DOI:10.1002/elan.201000519.
- M. Falahati, L. Ma'mani, A. Saboury, A. Shafiee, A. Foroumadi and A. R. Badiei, Aminopropyl-functionalized cubic Ia3d mesoporous silica nanoparticle as an efficient support for immobilization of superoxide dismutase, Biochim. Biophys. Acta, Proteins Proteomics, 2011, 1814(9), 1195–1202, DOI:10.1016/j.bbapap.2011.04.005.
- H. T. Kim, E. J. Yun, D. Wang, J. H. Chung, I. G. Choi and K. H. Kim, High temperature and low acid pretreatment and agarase treatment of agarose for the production of sugar and ethanol from red seaweed biomass, Bioresour. Technol., 2013, 136, 582–587, DOI:10.1016/j.biortech.2013.03.038.
- H. Zabed, J. N. Sahu, A. Suely, A. N. Boyce and G. Faruq, Bioethanol production from renewable sources: current perspectives and technological progress, Renewable Sustainable Energy Rev., 2017, 71, 475–501, DOI:10.1016/j.rser.2016.12.076.
- G. D. Saratale, R. G. Saratale, S. Varjani, S. K. Cho, G. S. Ghodake, A. Kadam, S. I. Mulla, R. N. Bharagava, D. S. Kim and H. S. Shin, Development of ultrasound aided chemical pretreatment methods to enrich saccharification of wheat waste biomass for polyhydroxybutyrate production and its characterization, Ind. Crops Prod., 2020, 150, 112425, DOI:10.1016/j.indcrop.2020.112425.
- V. S. Thite and A. S. Nerurkar, Valorization of sugarcane bagasse by chemical pretreatment and enzyme mediated deconstruction, Sci. Rep., 2019, 9, 15904, DOI:10.1038/s41598-019-52347-7.
- H. Chen, J. Mao, B. Jiang, W. Wu and Y. Jin, Carbonate-oxygen pretreatment of waste wheat straw for enhancing enzymatic saccharification, Process Biochem., 2021, 104, 117–123, DOI:10.1016/J.PROCBIO.2021.03.016.
- U. Qasim, Z. Ali, M. S. Nazir, S. U. Hassan, S. Rafiq, F. Jamil, A. H. Al-Muhtaseb, M. Ali, M. B. K. Niazi, M. Nasir, M. Ahmad, S. Ullah, A. Mukhtar and S. Saqib, Isolation of Cellulose from Wheat Straw Using Alkaline Hydrogen Peroxide and Acidified Sodium Chlorite Treatments: Comparison of Yield and Properties, Adv. Polym. Technol., 2020, 2020, 1–7, DOI:10.1155/2020/9765950.
- Y. Zhao, Y. Wang, J. Y. Zhu, A. Ragauskas and Y. Deng, Enhanced saccharification of spruce by alkaline pretreatment at low temperature, Biotechnol. Bioeng., 2008, 99(6), 1320–1328, DOI:10.1002/bit.21712.
- X. Zhao, K. Cheng and D. Liu, Organosolv pretreatment of lignocellulosic biomass for saccharification, Appl. Microbiol. Biotechnol., 2009, 82(5), 815–827, DOI:10.1007/s00253-009-1883-1.
- S. Zhang, H. Guo, L. Du, J. Liang, X. Lu, N. Li and K. Zhang, Influence of NaOH and thermal pretreatment on dewatered activated sludge solubilisation and subsequent anaerobic digestion: focused on high-solid state, Bioresour. Technol., 2015, 185, 171–177, DOI:10.1016/j.biortech.2015.02.050.
- G. Chaudhary, L. K. Singh and S. Ghosh, Alkaline pretreatment methods followed by acid hydrolysis of Saccharum spontaneum for bioethanol production, Bioresour. Technol., 2012, 124, 111–118, DOI:10.1016/j.biortech.2012.08.067.
- Q. Li, Y. Gao, H. Wang, B. Li, C. Liu, G. Yu and X. Mu, Comparison of different alkali-based pretreatments of corn stover for improving enzymatic saccharification, Bioresour. Technol., 2012, 125, 193–199, DOI:10.1016/j.biortech.2012.08.095.
- Q. Zheng, T. Zhou, Y. Wang, X. Cao, S. Wu, M. Zhao, H. Wang, M. Xu, B. Zheng, J. Zheng and X. Guan, Pretreatment of wheat straw leads to structural changes and improved enzymatic hydrolysis, Sci. Rep., 2018, 8, 1321, DOI:10.1038/s41598-018-19517-5.
- L. Xin, Z. Guo, X. Xiao, C. Peng, P. Zeng, W. Feng and W. Xu, Feasibility of anaerobic digestion on the release of biogas and heavy metals from rice straw pretreated with sodium hydroxide, Environ. Sci. Pollut. Res., 2019, 26(19), 19434–19444, DOI:10.1007/s11356-019-05195-x.
- M. C. Ho, V. Z. Ong and T. Y. Wu, Potential use of alkaline hydrogen peroxide in lignocellulosic biomass pretreatment and valorization–a review, Renewable Sustainable Energy Rev., 2019, 112, 75–86, DOI:10.1016/j.rser.2019.04.082.
- S. K. Du, X. Su, W. Yang, Y. Wang, M. Kuang, L. Ma, D. Fang and D. Zhou, Enzymatic saccharification
of high pressure assist-alkali pretreated cotton stalk and structural characterization, Carbohydr. Polym., 2016, 140, 279–286, DOI:10.1016/j.carbpol.2015.12.056.
- D. Jiang, X. Ge, Q. Zhang and Y. Li, Comparison of liquid hot water and alkaline pretreatments of giant reed for improved enzymatic digestibility and biogas energy production, Bioresour. Technol., 2016, 216, 60–68, DOI:10.1016/j.biortech.2016.05.052.
- G. Yu, S. Liu, X. Feng, Y. Zhang, C. Liu, Y. J. Liu, B. Li, Q. Cui and H. Peng, Impact of ammonium sulfite-based sequential pretreatment combinations on two distinct saccharifications of wheat straw, RSC Adv., 2020, 10(29), 17129–17142, 10.1039/D0RA01759K.
- S. Si, Y. Chen, C. Fan, H. Hu, Y. Li, J. Huang, H. Liao, B. Hao, Q. Li, L. Peng and Y. Tu, Lignin extraction distinctively enhances biomass enzymatic saccharification in hemicelluloses-rich Miscanthus species under various alkali and acid pretreatments, Bioresour. Technol., 2015, 183, 248–254, DOI:10.1016/j.biortech.2015.02.031.
- H. Wu, X. Dai, S. L. Zhou, Y. Y. Gan, Z. Y. Xiong, Y. H. Qin, J. Ma, L. Yang, Z. K. Wu, T. L. Wang and W. G. Wang, Ultrasound-assisted alkaline pretreatment for enhancing the saccharification of rice straw by using the heat energy dissipated from ultrasonication, Bioresour. Technol., 2017, 241, 70–74, DOI:10.1016/j.biortech.2017.05.090.
- A. Zafar, M. N. Aftab, Z. U. Din, S. Aftab, I. Iqbal, A. Shahid, A. Tahir and I. U. Haq, Cloning, Expression, and Purification of Xylanase Gene from Bacillus licheniformis for Use in Saccharification of Plant Biomass, Appl. Biochem. Biotechnol., 2016, 178(2), 294–311, DOI:10.1007/s12010-015-1872-z.
- M. N. Aftab, A. Zafar, I. Iqbal, A. Kaleem, K. M. Zia and A. R. Awan, Optimization of saccharification potential of recombinant xylanase from Bacillus licheniformis, Bioengineered, 2018, 9(1), 159–165, DOI:10.1080/21655979.2017.1373918.
- N. D. Huy, T. Saravanakumar, S. H. Ha and S. M. Park, Enhanced enzymatic saccharification of wheat flour arabinoxylan and barley straw using recombinant hemicellulases, Biotechnol. Bioprocess Eng., 2020, 25(3), 431–441 CrossRef CAS.
- B. C. Saha and M. A. Cotta, Alkaline peroxide pretreatment, enzymatic saccharification and fermentation of wheat straw to ethanol, in Proceedings of the 34th Meeting of the United States-Japan Cooperative Program in Natural Resources (UJNR) Food and Agriculture Panel, Susoni, Shizuoka, Japan, October 23–29, 2005, pp. 168–172 Search PubMed.
- H. Khaleghian, K. Karimi and T. Behzad, Ethanol production from rice straw by sodium carbonate pretreatment and Mucor hiemalis fermentation, Ind. Crops Prod., 2015, 76, 1079–1085, DOI:10.1016/j.indcrop.2015.08.008.
- A. Bala and B. Singh, Development of an environmental-benign process for efficient pretreatment and saccharification of Saccharum biomasses for bioethanol production, Renewable Energy, 2019, 130, 12–24, DOI:10.1016/j.renene.2018.06.033.
- A. Cornejo, I. Alegria-Dallo, Í. García-Yoldi, Í. Sarobe, D. Sánchez, E. Otazu, I. Funcia, M. J. Gil and V. Martínez-Merino, Pretreatment and enzymatic hydrolysis for the efficient production of glucose and furfural from wheat straw, pine and poplar chips, Bioresour. Technol., 2019, 288, 121583, DOI:10.1016/j.biortech.2019.121583.
- A. R. Alimon, Bioconversion and saccharification of some lignocellulosic wastes by Aspergillus oryzae ITCC-4857.01 for fermentable sugar production, Electron. J. Biotechnol., 2011, 14(5), 3, DOI:10.2225/vol14-issue5-fulltext-3.
- H. Zhang, W. Wei, J. Zhang, H. Shihang and X. Jun, Enhancing enzymatic saccharification of sugarcane bagasse by combinatorial pretreatment and Tween 80, Biotechnol. Biofuels, 2018, 11, 1–12, DOI:10.1186/s13068-018-1313-7.
- H. Chen, W. Huo, B. Wang, Y. Wang, H. Wen, D. Cai, C. Zhang, Y. Wu and P. Qin, L-lactic acid production by simultaneous saccharification and fermentation of dilute ethyle diamine pre-treated rice straw, Ind. Crops Prod., 2019, 141, 111749, DOI:10.1016/j.indcrop.2019.111749.
- S. Ethaib, R. Omar, M. K. S. Mazlina, A. B. D. Radiah and M. Y. Harun, Microwave-assisted pretreatment of sago palm bark, J. Wood Chem. Technol., 2017, 3, 26–42, DOI:10.1080/02773813.2016.1224249.
- Anu, B. Singh and A. Kumar, Process development for sodium carbonate pretreatment and enzymatic saccharification of rice straw for bioethanol production, Biomass Bioenergy, 2020, 138, 105574, DOI:10.1016/j.biombioe.2020.105574.
- P. Baral, A. Pundir, V. Kumar, A. K. Kurmi and D. Agrawal, Expeditious production of concentrated glucose-rich hydrolysate from sugarcane bagasse and its fermentation to lactic acid with high productivity, Food Bioprod. Process., 2020, 124, 72–81 CrossRef CAS , http://dspace.lib.cranfield.ac.uk/handle/1826/15724.
- D. Ilanidis, S. Stagge, L. J. Jönsson and C. Martín, Hydrothermal Pretreatment of Wheat Straw: Effects of Temperature and Acidity on Byproduct Formation and Inhibition of Saccharification and Ethanolic Fermentation, Agronomy, 2021, 11(3), 487, DOI:10.3390/agronomy11030487.
|
This journal is © The Royal Society of Chemistry 2023 |
Click here to see how this site uses Cookies. View our privacy policy here.