DOI:
10.1039/D2RA07521K
(Paper)
RSC Adv., 2023,
13, 1877-1882
A new and straightforward route to synthesize novel pyrazolo[3,4-b]pyridine-5-carboxylate scaffolds from 1,4-dihydropyrano[2,3-c]pyrazole-5-carbonitriles†
Received
29th November 2022
, Accepted 25th December 2022
First published on 11th January 2023
Abstract
Among many acidic catalysts, amorphous carbon-supported sulfonic acid (AC-SO3H) has been evaluated as a new-generation solid catalyst with outstanding activity. Because of the –SO3H groups, the surface properties of the amorphous carbon catalyst were improved, which made the catalytic activity of the amorphous carbon-supported sulfonic acid many times greater than that of sulfuric acid. The amorphous carbon-supported sulfonic acid exhibited several advantages such as low cost, non-toxicity, porosity, stability, and easily adjustable chemical surface. In this paper, we introduce a new pathway for the synthesis of pyrazolo[3,4-b]pyridine-5-carboxylate scaffolds from 1,4-dihydropyrano[2,3-c]pyrazole-5-carbonitriles and aniline at room temperature under ethanol in the presence of AC-SO3H as the catalyst. This method provided the desired products with moderate to good yields. The gram-scale synthesis of the major product was carried out with good yields (up to 80%). This strategy involves a sequential opening/closing cascade reaction. This approach presents several advantages, including room temperature conditions, short reaction time, and operational simplicity.
Introduction
Nitrogen-containing heterocycles are among the most important frameworks for the discovery of potent bioactive agents in pharmaceuticals and agrochemicals. The fusion of pyridine systems, pyrazolopyridines, has been evaluated for activity and access to pharmaceutical products, which have been applied with selective antibacterial, antiviral, antifungal, and antitumor activity.1–7 Various synthetic methods have been implemented to synthesize pyrazolopyridines under different conditions (see the previous studies in Scheme 1). To improve the processes and develop new synthesis processes, we report herein a new strategy to obtain pyrazolo[3,4-b]pyridine from the fusion of pyrano[2,3-c]pyrazole containing cyano and amino groups with aniline (this current work, Scheme 1). The previous studies used 1,3,-dialkyl-1H-pyrazole-5-amine as a starting material to synthesize pyrazolo[3,4-b]pyridine.8–12 The highlight of our research is the use of α,β-unsaturated compounds in reactions with N-nucleophiles using amorphous carbon-supported sulfonic acid (AC-SO3H) as an effective catalyst in the presence of ethanol as the reaction medium.
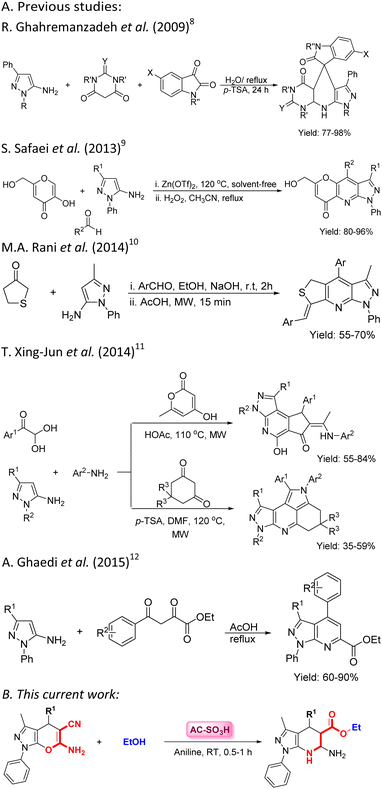 |
| Scheme 1 The pathway for the synthesis of pyrazolo[3,4-b]pyridine frames (A) previous studies and (B) current work. | |
The carbonization of rice husk with sulfuric acid was used to create the sulfonated amorphous carbon material.13 Notably, the SO3H group-containing material had a high density (>4 mg g−1).14 P. Gupta and S. Paul created amorphous carbon-silica composites containing sulfonic acid and used them for N-, O-, and S-acylation reactions as well as the protection of aldehydes as 1,1-diacetates.15 A solid Brønsted acid catalyst made from functional carbon with an SO3H group was utilized in reactions for the formation of C–C bonds as well as for esterification, acetalization, etherification, transesterification, hydration, dehydration, and C–C bond-forming reactions.16
Results and discussion
Characterization of the AC-SO3H catalyst
AC-SO3H was synthesized according to a previously published procedure.17,18 The characterization of the AC-SO3H was confirmed by FTIR, P-XRD, scanning electron microscopy (SEM), TGA, and EDS, and the results are shown in the ESI.† According to the FTIR spectrum, broad FTIR bands can be found between wavelengths of 3500 and 3000 cm−1 (representing the O–H signal of SO3H), 1370 cm−1 (representing the O
S
O stretching in SO3H), 1630 cm−1 (representing the C
C signals of the polyaromatic structure), and 1100 cm−1 (representing the symmetric vibration of the S
O of SO3H) (Fig. 1).19 Thermogravimetric analysis was used to determine the AC-SO3H catalyst's thermal stability (Fig. 2). The first weight loss occurred below 350 °C with 5% loss of water in the structure of AC-SO3H. The following weight loss, which was around 65%, took place between 350 and 500 °C and was observed in conjunction with the loss of organic functionality. The amorphous carbon structures are responsible for the broad C(002) peak that can be seen in the diffraction signal of the P-XRD pattern between 2θ of 15 and 35° (Fig. 3). The peak may be found in this range of values. The a axis of the graphite structure is responsible for the weak and broad C(101) diffraction signal that may be observed between 2θ of 40 and 50°.20 Energy-dispersive X-ray spectroscopy (EDX) was used to determine the elemental composition, and the results showed that the AC-SO3H consisted of carbon (C), silicon (Si), oxygen (O), and sulfur (S) (Fig. 4). SEM was utilized in order to analyze the size and morphology of the AC-SO3H, which revealed the compound to have an agglomerated structure (Fig. 5).
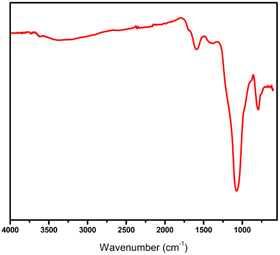 |
| Fig. 1 FT-IR spectrum of AC-SO3H. | |
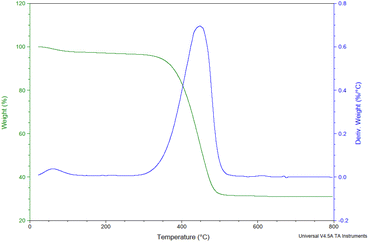 |
| Fig. 2 TGA of AC-SO3H. | |
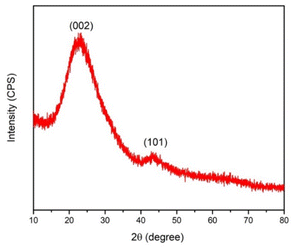 |
| Fig. 3 P-XRD spectrum of AC-SO3H. | |
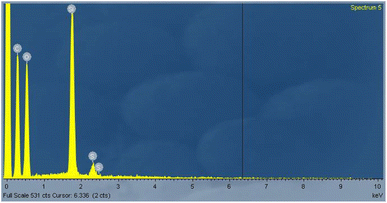 |
| Fig. 4 EDS spectrum of AC-SO3H. | |
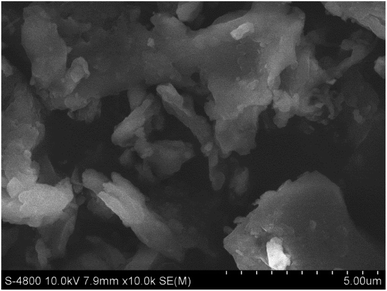 |
| Fig. 5 SEM image of AC-SO3H. | |
Synthesis of pyrazolo[3,4-b]pyridines
Herein, we report a new pathway for the preparation of pyrazolo[3,4-b]pyridines, where AC-SO3H was used as a green catalyst. The catalytic activity of the AC-SO3H was investigated for the synthesis of ethyl 6-amino-3-methyl-1,4-diphenyl-4,5,6,7-tetrahydro-1H-pyrazolo[3,4-b]pyridine-5-carboxylate (1b), which was carried out using 6-amino-3-methyl-1,4-diphenyl-1,4-dihydropyrano[2,3-c]pyrazole-5-carbonitrile (1), and aniline (Scheme 1B). In the first step of the process, 6-amino-3-methyl-1,4-diphenyl-1,4-dihydropyrano[2,3-c]pyrazole-5-carbonitrile (1) was produced through a multicomponent reaction involving phenylhydrazine (1.0 mmol), aldehyde (1.0 mmol), ethyl acetoacetate (1.0 mmol), and malononitrile (1.0 mmol). This was introduced in a previous study.17
The reaction parameters were evaluated for the synthesis of (1b), as shown in Table 1. In the presence of 5 mg of AC-SO3H, which served as the catalyst, the condensation was carried out at a range of temperatures, including room temperature, 60 °C, and 100 °C. The best result was achieved at room temperature for 3 h, with over 83% of (1b) (entries 1–3, Table 1). Then, the yield of the major product (1b) was studied at different reaction times (0.5, 1, 2, and 3 h). Interestingly, the reaction continued even though it was carried out at room temperature, and the time at which it produced the highest yield (almost 97%) was 0.5 hours (entries 4–6, Table 1). The loading of AC-SO3H was examined at different weights from 5 mg to 15 mg, and the best result was achieved with 5 mg of catalyst (entries 5, 7–8, Table 1).
Table 1 Optimization of reaction conditions for maximum yield of (1b)a
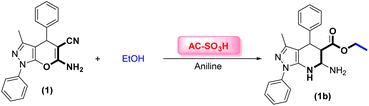
|
Entry |
Temperature (°C) |
Time (h) |
Catalyst (mg) |
Yieldb (%) |
Reaction conditions: (1) (0.25 mmol), aniline (0.25 mmol), AC-SO3H (5 mg), and EtOH (2.0 mL). Isolated yields. NR: no reaction. |
1 |
RT |
3 |
5 |
83 |
2 |
60 |
3 |
5 |
28 |
3 |
100 |
3 |
5 |
NRc |
4 |
RT |
0.5 |
5 |
97 |
5 |
RT |
1 |
5 |
96 |
6 |
RT |
2 |
5 |
69 |
7 |
RT |
1 |
10 |
55 |
8 |
RT |
1 |
15 |
74 |
The catalyst activity was also compared to similar catalysts, and the results are shown in Table 2. The reaction between compound (1) and ethanol/aniline was carried out at room temperature for a period of thirty minutes, during which time the usual Brønsted acid catalysts (such as HCl, H2SO4, H3PO4, and TsOH) used in place of AC-SO3H gave good yields of (1b) (entries 1–4, Table 2). Additionally, SiO2–H2SO4 was synthesized from silica gel and concentrated sulfuric acid,21 and used as the catalyst for the synthesis of (1b) with high yield (entry 5, Table 2). Notably, AC-SO3H exhibited excellent activity in the preparation of the desired product (1b) with 97% yield (entry 6, Table 2). The good catalytic activity of Ac-SO3H toward organic transformation has been reported in previous literature.22–24
Table 2 Effect of different catalystsa
Entry |
Catalysts |
Yieldb (%) |
Reaction conditions: (1) (0.25 mmol), aniline (0.25 mmol), catalyst, and EtOH (2.0 mL). Isolated yields. HCl (37.0 wt% in water): 18.3 μL; H2SO4 (98.0 wt% in water): 13.0 μL; H3PO4 (85.0 wt% in water): 36.3 μL. |
1 |
HClc |
71 |
2 |
H2SO4c |
67 |
3 |
H3PO4c |
80 |
4 |
TsOH |
82 |
5 |
SiO2–H2SO4 |
80 |
6 |
AC-SO3H |
97 |
The yields of the major product (1b) markedly depend on the effect of the substituted pyranopyrazoles. The best results are shown in Scheme 2. With the optimized conditions for the procedure in hand, pyrazolo[3,4-b]pyridine-5-carboxylate scaffolds bearing para-substituents (1–6) and heterocyclic-substituents (7 and 8) were produced from pyranopyrazole and ethanol in the presence of aniline. Pyrazolo[3,4-b]pyridine-5-carboxylate that contained either an electron-donating (–Me) group on the benzene ring at the para-substituent position or an electron-withdrawing (–F, –Br, –Cl, or –NO2) group reacted smoothly to form the desired products in moderate to good yields (3b–6b). The furfural-substituted pyranopyrazole resulted in a good yield at room temperature (7b). Additionally, the furfural-substituted pyranopyrazole containing an NO2 group reacted favorably with ethanol to form the desired product in a good yield (8b). Furthermore, imidazole-substituted pyranopyrazole provided low yields (trace) despite a prolonged reaction time at room or high temperature (60 °C). In most cases, 4,5,6,7-tetrahydro-1H-pyrazolo[3,4-b]pyridine-5-carboxylates quickly fused with pyrano[2,3-c]pyrazoles by employing aniline in the presence of EtOH in the para-substituent position, and this reaction was observed to take place. However, for pyrano[2,3-c]pyrazoles with ortho-substituents (such as –OH, –COOH, –Cl, –F, –Br, etc.), TLC was used to monitor the reaction, and no product traces were formed despite the prolonged reaction time. This can be explained by the stereochemistry of the ortho-substituents and carbonyl groups of the ester.
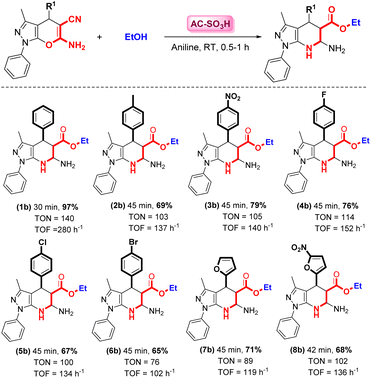 |
| Scheme 2 AC-SO3H-catalyzed synthesis of 4,5,6,7-tetrahydro-1H-pyrazolo[3,4-b]pyridine-5-carboxylate derivatives. Reaction conditions: 1,4-dihydropyrano[2,3-c]pyrazole-5-carbonitriles (1–8) (0.25 mmol), aniline (0.25 mmol), AC-SO3H (5 mg), and EtOH (2.0 mL). Yields shown are isolated yields. | |
The proposed mechanism for the synthesis of (1b) is shown in Scheme 3. First, in the presence of aniline, the reaction between aniline and (1b) took place to form the intermediates (A) and (B), which were identified by HRMS-ESI (m/z 421.1984 [M]+, cacld m/z 421.1903). Then, the precursor (A) was converted to the intermediate (C). AC-SO3H activated the carbonyl group intermediate (C), resulting in the intramolecular reaction with the N-nucleophiles to give intermediate (D) (m/z 402.1694 [M]+, cacld m/z 402.1719). Next, the nitrile group of (E) was converted to an ester in the presence of ethanol through the Pinner reaction (E–H) to form (H), which was recorded by HRMS (m/z 376.1635 [M + H]+, cacld m/z 376.1661). The intermediates (F) and (G) were recorded by HRMS. Then, the ketone group was activated by AC-SO3H through the Leuckart reaction (H-1b) to form the desired product (1b) (m/z 376.1840 [M]+, cacld m/z 376.1899).
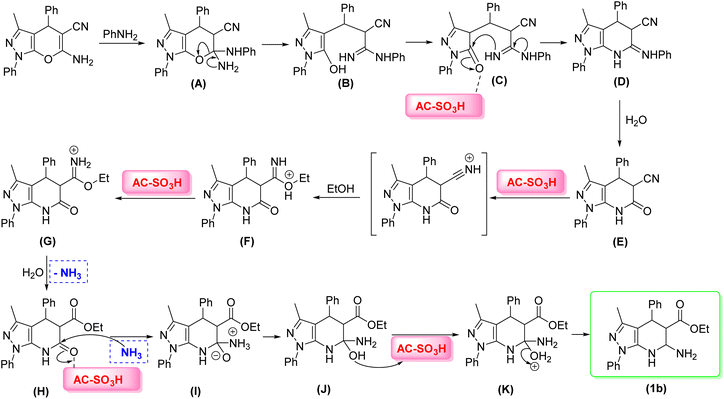 |
| Scheme 3 The mechanism proposed for the synthesis of (1b) using AC-SO3H as the catalyst. | |
Experimental
Chemicals
Phenylhydrazine (97%), ethyl acetoacetate (99%), malononitrile (99%), 4-nitrobenzaldehyde (98%), furfural (99%), 5-nitro-2-furaldehyde (99%), 4-fluorobenzaldehyde (98%), 4-chlorobenzaldehyde (97%), 4-bromobenzaldehyde (99%), and p-toluenesulfonic acid monohydrate (TsOH) (ACS grade reagent, assay ≥ 98.5%) were purchased from Sigma-Aldrich. Benzaldehyde, 4-methylbenzaldehyde, 4-methoxybenzaldehyde, aniline, ethanol, TLC (silica gel 60 F254), sulfuric acid (98%), hydrochloric acid (1 mol L−1), and ortho-phosphoric acid (85%) were purchased from Merck. Ethyl acetate (99.5%) and n-hexane (99.5%) were purchased from Xilong Chemical Co., Ltd (China).
Analytical techniques
A Bruker Avance 500 MHz was used to record the 1H and 13C NMR spectra. CDCl3 was used as the solvent, and either TMS or solvent peaks served as the internal standard. Data for HRMS (ESI) were gathered with the help of Bruker's micrOTOF-QII MS operating at 80 eV. A Buchi melting point B-545 was used to determine boiling points.
Synthesis of amorphous carbon-supported sulfonic acid (AC-SO3H)
The incomplete carbonization of rice husks was carried out at 400 °C under a N2 atmosphere for 10 h. Then, concentrated sulfuric acid was added and heated for 10 h at 150 °C under a N2 atmosphere for sulfonation. Next, the black solid was washed with hot deionized water at 80 °C many times until sulfate anions were not detected in the filtrate. Finally, the sulfonated amorphous carbon catalyst was dried at 100 °C for 2 h. The purity and structure of the catalyst were confirmed using FT-IR, XRD, TGA, EDS, and SEM.
General procedure for the synthesis of ethyl 6-amino-3-methyl-1,4-diphenyl-4,5,6,7-tetrahydro-1H-pyrazolo[3,4-b]pyridine-5-carboxylate
At room temperature, a combination of 6-amino-3-methyl-1,4-diphenyl-1,4-dihydro-pyrano[2,3-c]pyrazole-5-carbonitrile (1) (0.25 mmol), aniline (0.25 mmol, 23.5 mg), and AC-SO3H (5 mg) was agitated for 30–45 minutes in the presence of EtOH (2.0 mL). TLC was utilized in order to track the reactions. Following the termination of the reaction, the mixture was subjected to filtration and washing with EtOH (3 × 5.0 mL). The main product, ethyl 6-amino-3-methyl-1,4-diphenyl-4,5,6,7-tetrahydro-1H-pyrazolo[3,4-b]pyridine-5-carboxylate, was obtained by recrystallization from EtOH. This process produced the compound (1b). 1H NMR, 13C NMR, and HRMS (ESI) were utilized to determine the structure and purity.
Gram-scale synthesis of 1b
A mixture of 6-amino-3-methyl-1,4-diphenyl-1,4-dihydropyrano[2,3-c]pyrazole-5-carbonitrile (1) (5.0 mmol, 1.640 g), aniline (5.0 mmol, 0.470 g), and AC-SO3H (25 mg) in the presence of EtOH (10.0 mL) was agitated for 30 minutes at room temperature in a 100 mL flask. After completion of the reaction, the mixture was filtered and then washed with EtOH (3 × 5.0 mL). The major product was recrystallized from EtOH to provide ethyl 6-amino-3-methyl-1,4-diphenyl-4,5,6,7-tetrahydro-1H-pyrazolo[3,4-b]pyridine-5-carboxylate (1b) with a yield of 1.441 g (80%).
Conclusions
In conclusion, we have developed a novel approach for synthesizing pyrazolo[3,4-b]pyridine by sequential opening/closing cascade under mild conditions. To the best of our knowledge, this is the first example in which novel pyrazolo[3,4-b]pyridine-5-carboxylate scaffolds have been synthesized from 1,4-dihydropyrano[2,3-c]pyrazole-5-carbonitriles. This novel approach provides an effective strategy for building pyrazolo[3,4-b]pyridine-5-carboxylate scaffolds, which have uses in the process of drug development.
Author contributions
Hai Truong Nguyen: investigation, methodology, resources, formal analysis, validation, data curation, writing – original draft. Phu Hoang Dang: formal analysis, writing – review & editing. Phuong Hoang Tran: methodology, resources, formal analysis, validation, data curation, writing – review & editing, supervision.
Conflicts of interest
There are no conflicts to declare.
Acknowledgements
Hai Truong Nguyen acknowledges Vingroup Joint Stock Company supported by the Domestic Master/PhD Scholarship Programme of Vingroup Innovation Foundation (VINIF), Vingroup Big Data Institute (VINBIGDATA), code VINIF.2021.TS.014.
Notes and references
- N. S. El-Gohary and M. I. Shaaban, Eur. J. Med. Chem., 2018, 152, 126–136 CrossRef CAS PubMed.
- H. S. Kim, J. T. Hammill, D. C. Scott, Y. Chen, J. Min, J. Rector, B. Singh, B. A. Schulman and R. K. Guy, J. Med. Chem., 2019, 62, 8429–8442 CrossRef CAS PubMed.
- R. Pagadala, S. Maddila, V. Moodley, W. E. van Zyl and S. B. Jonnalagadda, Tetrahedron Lett., 2014, 55, 4006–4010 CrossRef CAS.
- H.-S. Kim, J. R. Jadhav, S.-J. Jung and J.-H. Kwak, Bioorg. Med. Chem. Lett., 2013, 23, 4315–4318 CrossRef CAS PubMed.
- S. Huang, R. Lin, Y. Yu, Y. Lu, P. J. Connolly, G. Chiu, S. Li, S. L. Emanuel and S. A. Middleton, Bioorg. Med. Chem. Lett., 2007, 17, 1243–1245 CrossRef CAS PubMed.
- L. Bettinetti, K. Schlotter, H. Hübner and P. Gmeiner, J. Med. Chem., 2002, 45, 4594–4597 CrossRef CAS PubMed.
- F. Goli-Garmroodi, M. Omidi, M. Saeedi, F. Sarrafzadeh, A. Rafinejad, M. Mahdavi, G. R. Bardajee, T. Akbarzadeh, L. Firoozpour, A. Shafiee and A. Foroumadi, Tetrahedron Lett., 2015, 56, 743–746 CrossRef CAS.
- R. Ghahremanzadeh, M. Sayyafi, S. Ahadi and A. Bazgir, J. Comb. Chem., 2009, 11, 393–396 CrossRef CAS PubMed.
- S. Safaei, I. Mohammadpoor-Baltork, A. R. Khosropour, M. Moghadam, S. Tangestaninejad, V. Mirkhani and H. R. Khavasi, ACS Comb. Sci., 2013, 15, 141–146 CrossRef CAS PubMed.
- M. A. Rani, V. Jeyachandran, M. Muthu, S. Sivakolunthu and R. R. Kumar, Tetrahedron Lett., 2014, 55, 5805–5807 CrossRef CAS.
- X.-J. Tu, W.-J. Hao, Q. Ye, S.-S. Wang, B. Jiang, G. Li and S.-J. Tu, J. Org. Chem., 2014, 79, 11110–11118 CrossRef CAS PubMed.
- A. Ghaedi, G. R. Bardajee, A. Mirshokrayi, M. Mahdavi, A. Shafiee and T. Akbarzadeh, RSC Adv., 2015, 5, 89652–89658 RSC.
- P. H. Tran and P. V. Tran, Fuel, 2019, 246, 18–23 CrossRef CAS.
- K. Nakajima and M. Hara, ACS Catal., 2012, 2, 1296–1304 CrossRef CAS.
- P. Gupta and S. Paul, Green Chem., 2011, 13, 2365–2372 RSC.
- L. J. Konwar, P. Mäki-Arvela and J.-P. Mikkola, Chem. Rev., 2019, 119, 11576–11630 CrossRef CAS PubMed.
- H. T. Nguyen, T. V. Le and P. H. Tran, J. Environ. Chem. Eng., 2021, 9, 105228 CrossRef CAS.
- H. T. Nguyen, M.-N. H. Truong, T. V. Le, N. T. Vo, H. D. Nguyen and P. H. Tran, ACS Omega, 2022, 7, 17432–17443 CrossRef CAS PubMed.
- S. Suganuma, K. Nakajima, M. Kitano, D. Yamaguchi, H. Kato, S. Hayashi and M. Hara, J. Am. Chem. Soc., 2008, 130, 12787–12793 CrossRef CAS PubMed.
- M. Hara, T. Yoshida, A. Takagaki, T. Takata, J. N. Kondo, S. Hayashi and K. Domen, Angew. Chem., Int. Ed., 2004, 43, 2955–2958 CrossRef CAS PubMed.
- A. Sultan, A. R. Raza, M. Abbas, K. M. Khan, M. N. Tahir and N. Saari, Molecules, 2013, 18, 10081–10094 CrossRef CAS PubMed.
- J. M. Fonseca, L. Spessato, A. L. Cazetta, C. da Silva and V. d. C. Almeida, Chem. Eng. Process., 2022, 170, 108668 CrossRef CAS.
- R. Zhong and B. F. Sels, Appl. Catal., B, 2018, 236, 518–545 CrossRef CAS.
- P. Gupta and S. Paul, Catal. Today, 2014, 236, 153–170 CrossRef CAS.
|
This journal is © The Royal Society of Chemistry 2023 |
Click here to see how this site uses Cookies. View our privacy policy here.