DOI:
10.1039/D2RA08257H
(Paper)
RSC Adv., 2023,
13, 7432-7435
Asymmetric ring-opening reactions of donor–acceptor cyclopropanes with 1,3-cyclodiones†
Received
27th December 2022
, Accepted 24th February 2023
First published on 7th March 2023
Abstract
Asymmetric ring-opening reactions of donor–acceptor cyclopropanes with 1,3-cyclodiones have been established for the synthesis of enantioenriched γ-hydroxybutyric acid derivatives in the presence of Cu(II)/trisoxazoline catalyst. These reactions offered the desired products in 70% to 93% yields with 79% to 99% enantiomeric excesses.
Donor–acceptor (D–A) cyclopropanes are one of the most powerful 1,3-dipolar synthons for the construction of natural products and biologically active compounds.1 The nucleophilic ring-opening reactions of D–A cyclopropanes have been recognized as useful strategies to access 1,3-bifunctionalized scaffolds.1–3 Asymmetric ring-opening reactions of D–A cyclopropanes3 with heteroatom containing nucleophiles have been well established.3a–g For example, Tang and co-workers reported chiral Ni/bisoxazoline (BOX)-catalyzed asymmetric ring-opening reactions of D–A cyclopropanes with secondary aliphatic amines for the synthesis of γ-aminobutyric acid derivatives.3a,b In addition, related asymmetric ring-opening processes using aromatic amines as nucleophiles were also reported by Feng, Wang and Cai.3c–e Using thiols as the nucleophiles, Feng and co-workers disclosed highly enantioselective ring-opening of D–A cyclopropanes in the presence of the chiral Sc/N,N′-dioxide catalyst to afford γ-thiobutyric acid derivatives.3f Furthermore, Tang's group developed a method to access enantioenriched γ-hydroxybutyric acid derivatives by Cu/trisoxazoline (TOX)-catalyzed ring-opening of D–A cyclopropanes with water and alcohols.3g
1,3-Cyclodiones can be used as both O- and C-nucleophiles due to easy formation of enol forms and have been applied in many domino and multi-component reactions.4 Recently, we developed scandium triflate catalyzed O-selective nucleophilic ring-opening of D–A cyclopropanes with 1,3-cyclodiones,5b where the ring-opening products 1,3-cyclodione enol ether derivatives were obtained in good to excellent yields (Scheme 1a). In continuation of our research interests in the reactions between 1,3-dicarbonyl compounds and D–A cyclopropanes,5 herein, we disclose the asymmetric version of the ring opening reactions of D–A cyclopropanes with 1,3-cyclodiones (Scheme 1b).
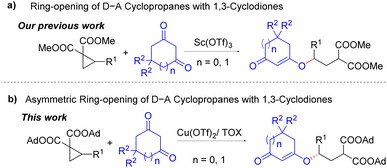 |
| Scheme 1 Our previous work and present work. | |
According to our previous initial attempts,5b reaction of 1a and 2a in the presence of Sc(OTf)3 and ligand L1 (Fig. 1) afforded desired product 3a with 62% ee (Table 1, entry 1). Thus, we started screening the reaction conditions using adamantlyl ester substituted D–A cyclopropane 1a and 1,3-cyclohexanone 2a as the model substrates (Table 1). When chiral ligand L1 was used, Cu(OTf)2 performed much better than Sc(OTf)3 in terms of product enantioselectivity (Table 1, entry 1 vs. entry 2). After screening various bis-/trisoxazoline ligands, cyclohexyl-trisoxazoline (Cy-TOX) L4 was found to give the best results with excellent enantioselectivity (Table 1, entries 3–5). When other solvent, such as toluene and THF, was used, the reaction results became poorer in terms of both yield and enantioselectivity (Table 1, entries 6 and 7). The enantiomeric excess of 3a slightly decreased when the reaction temperature increased to 35 °C (Table 1, entry 8). Reducing the catalyst loading to 10 mol% did not affect the enantioselectivity of 3a, and the yield of 3a was able to be improved to 85% by prolonging the reaction time to 50 h (Table 1, entry 9).
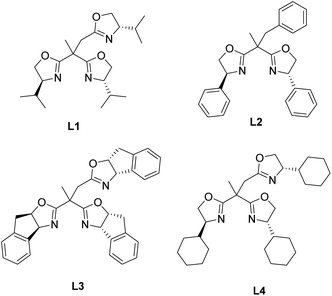 |
| Fig. 1 Ligands used for the asymmetric ring-opening reactions of D–A cyclopropanes with 1,3-cyclodiones. | |
Table 1 Optimization of the reaction conditionsa

|
Entry |
Ln |
Solvent |
Yieldb (%) |
eec (%) |
Unless otherwise noted, reactions were carried out using 1a (0.20 mmol), 2a (0.40 mmol), Cu(OTf)2 (0.04 mmol) with ligand (0.048 mmol) in solvent (1.0 mL) at room temperature (25 °C) for 24 h. Isolated yields. Determined by chiral HPLC. Sc(OTf)3 was used as catalyst. The reaction was carried out at 35 °C. Cu(OTf)2 (0.02 mmol) with ligand (0.024 mmol) was used and the reaction time was 50 h. |
1 |
L1 |
CH2Cl2 |
66 |
62 |
2 d |
L1 |
CH2Cl2 |
80 |
13 |
3 |
L2 |
CH2Cl2 |
58 |
60 |
4 |
L3 |
CH2Cl2 |
46 |
69 |
5 |
L4 |
CH2Cl2 |
70 |
99 |
6 |
L4 |
PhCH3 |
65 |
89 |
7 |
L4 |
THF |
49 |
71 |
8 e |
L4 |
CH2Cl2 |
83 |
97 |
9 f |
L4 |
CH2Cl2 |
85 |
99 |
Next, the substrate scope of the asymmetric ring-opening reactions was investigated under the optimized conditions (Table 1, entry 9). As shown in Scheme 2, the reactions of various D–A cyclopropanes 1 with different 1,3-cyclodiones 2 proceeded smoothly to furnish enantioenriched 1,3-cyclodione enol ether derivatives 3 in good yields and high enantioselectivities (70–93% yield, 79–99% ee). For the reactions of D–A cyclopropanes 1 with methoxyl group substituted on the phenyl ring, the position of the methoxyl group influenced the enantioselectivity of the corresponding products significantly (3a–3c). Reaction of para-methoxyl phenyl substituted D–A cyclopropane afforded product with highest enantioselectivity (3a, 99% ee). When meta- or ortho-methoxyl phenyl substituted D–A cyclopropane was used, the corresponding product enantioselectivity dropped to 90% and 80%, respectively. Similar to our previous studies, reactions of electron-rich D–A cyclopropanes with 1,3-cyclohexanone were faster than electron-deficient ones (3a, 3d vs. 3e, 3f), whereas the electronic nature had no significant effects on enantioselectivities (3d–3f). Heterocyclic substrates, such as 2-thienyl and 3-indolyl substituted D–A cyclopropanes, tolerated well in this asymmetric reaction, and the corresponding products 3g and 3h were accomplished in good yields and high enantioselectivities. Reactions using 1,3-cyclopentanedione (2b) and 5,5-dimethyl-1,3-cyclohexanedione (2c) as nucleophiles in the asymmetric ring-opening reactions were also studied. Reaction of 1a and 2b proceeded well to afford 3i in 81% yield with 95% ee, though longer reaction time was necessary. Reactions of 2c with 1a and 3-indolyl substituted D–A cyclopropane afforded corresponding products in excellent yields and enantioselectivities (3j and 3l), whereas the reaction with 2-thienyl substituted D–A cyclopropane provided 3k with much lower enantiomeric excess, 79%. The absolute configurations of 3a–3l were inferred to be (S) according to Tang's work.3g
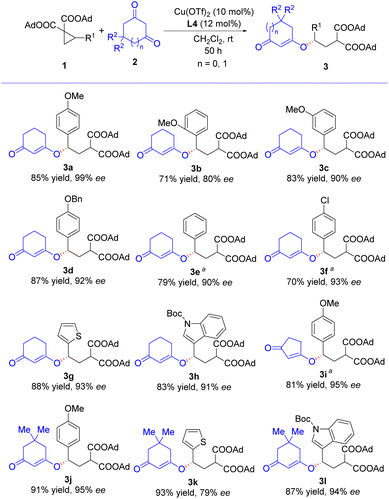 |
| Scheme 2 Reactions of various D–A cyclopropanes 1 with different 1,3-cyclodiones 2. Unless otherwise noted, reactions were carried out using 1 (0.20 mmol), 1,3-cyclodiones 2 (0.40 mmol), Cu(OTf)2 (0.02 mmol) with L4 (0.024 mmol) in 1.0 mL CH2Cl2 at room temperature (25 °C) for 50 h. aThe reaction time was 100 h. | |
The asymmetric ring-opening reaction was demonstrated in a gram-scale reaction (Scheme 3). In this gram-scale reaction of 1a and 2a, the chiral 1,3-cyclodione enol ether 3a was obtained in 82% yield with 99% ee.
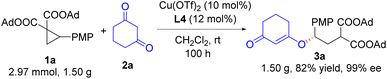 |
| Scheme 3 Gram-scale synthesis of 3a. | |
Conclusions
We have established the asymmetric ring-opening reactions of D–A cyclopropanes with 1,3-cyclodiones for the synthesis of enantioenriched γ-hydroxybutyric acid derivatives in the presence of Cu(II)/TOX catalyst. A range of 1,3-cyclodione enol ether derivatives were obtained in good yields and with high enantioselectivities. This methodology reported here may be of benefit for pharmaceutical research.
Conflicts of interest
There are no conflicts to declare.
Acknowledgements
This study was supported by Anhui Provincial Natural Science Foundation (1908085MB33), and the Anhui University of Technology.
Notes and references
- For selected reviews of donor–acceptor cyclopropanes:
(a) H. U. Reissig and R. Zimmer, Chem. Rev., 2003, 103, 1151–1196 CrossRef CAS PubMed;
(b) D. Agrawal and V. K. Yadav, Chem. Commun., 2008, 6471–6488 RSC;
(c) C. A. Carson and M. A. Kerr, Chem. Soc. Rev., 2009, 38, 3051–3060 RSC;
(d) M. A. Cavitt, L. H. Phun and S. France, Chem. Soc. Rev., 2014, 43, 804–818 RSC;
(e) T. F. Schneider, J. Kaschel and D. B. Werz, Angew. Chem., Int. Ed., 2014, 53, 5504–5523 CrossRef CAS PubMed;
(f) H. K. Grover, M. R. Emmett and M. A. Kerr, Org. Biomol. Chem., 2015, 13, 655–671 RSC;
(g) M. Meazza, H. Guo and R. Rios, Org. Biomol. Chem., 2017, 15, 2479–2490 RSC;
(h) A. U. Augustin and D. B. Werz, Acc. Chem. Res., 2021, 54, 1528–1541 CrossRef CAS PubMed;
(i) K. Ghosh and S. Das, Org. Biomol. Chem., 2021, 19, 965–982 RSC;
(j) V. Pirenne, B. Muriel and J. Waser, Chem. Rev., 2021, 121, 227–263 CrossRef CAS PubMed;
(k) Y. Xia, X. Liu and X.-M. Feng, Angew. Chem., Int. Ed., 2021, 60, 9192–9204 CrossRef CAS PubMed;
(l) H. Huo and Y. Gong, Org. Biomol. Chem., 2022, 20, 3847–3869 RSC.
-
(a) M. R. Emmett, H. K. Grover and M. A. Kerr, J. Org. Chem., 2012, 77, 6634–6637 CrossRef CAS PubMed;
(b) F. de Nanteuil, J. Loup and J. Waser, Org. Lett., 2013, 15, 3738–3741 CrossRef CAS PubMed;
(c) A. Lucht, L. J. Patalag, A. U. Augustin, P. G. Jones and D. B. Werz, Angew. Chem., Int. Ed., 2017, 56, 10587–10591 CrossRef PubMed;
(d) J. Wallbaum, L. K. B. Garve, P. G. Jones and D. B. Werz, Org. Lett., 2017, 19, 98–101 CrossRef CAS PubMed;
(e) S. Das, C. G. Daniliuc and A. Studer, Angew. Chem., Int. Ed., 2017, 56, 11554–11558 CrossRef CAS PubMed;
(f) X.-M. Feng, P. Yang, G. Yang, W. Chen and Z. Chai, J. Org. Chem., 2018, 83, 174–184 CrossRef PubMed;
(g) A. U. Augustin, P. G. Jones and D. B. Werz, Chem.–Eur. J., 2019, 25, 11620–11624 CrossRef CAS PubMed;
(h) A. Lücht, S. Sobottka, L. J. Patalag, P. G. Jones, H. U. Reissig, B. Sarkar and D. B. Werz, Chem.–Eur. J., 2019, 25, 10359–10365 CrossRef PubMed;
(i) A. U. Augustin, J. L. Merz, P. G. Jones, G. Mlostoń and D. B. Werz, Org. Lett., 2019, 21, 9405–9409 CrossRef CAS PubMed;
(j) U. K. Mishra, K. Patel and S. S. V. Ramasastry, Org. Lett., 2020, 22, 3815–3819 CrossRef CAS PubMed;
(k) Z. Zuo and A. Studer, Org. Lett., 2022, 24, 949–954 CrossRef CAS PubMed;
(l) P. Su, H. Li, W. Chen, G. Luo, G. Yang and Z. Chai, Eur. J. Org. Chem., 2020, 33, 5380–5387 CrossRef;
(m) V. Pirenne, E. G. L. Robert and J. Waser, Chem. Sci., 2021, 12, 8706–8712 RSC;
(n) Z. Liu, Y. Wei and M. Shi, Org. Chem. Front., 2022, 9, 1960–1966 RSC.
-
(a) Y. Y. Zhou, L. J. Wang, X. L. Sun and Y. Tang, J. Am. Chem. Soc., 2012, 134, 9066–9069 CrossRef CAS PubMed;
(b) Q. K. Kang, L. J. Wang, Z. B. Zheng, J. F. Li and Y. Tang, Chin. J. Chem., 2014, 32, 669–672 CrossRef CAS;
(c) Y. Xia, L. Lin, F. Chang, Y. Liao, X. Liu and X.-M. Feng, Angew. Chem., Int. Ed., 2016, 55, 12228–12232 CrossRef CAS PubMed;
(d) W. W. Luo, Z.-C. Sun, E. H. N. Fernando, V. N. Nesterov, T. R. Cundari and H. Wang, ACS Catal., 2019, 9, 8285–8293 CrossRef CAS;
(e) L. Xu, Q. Yang, S.-S. Zhong, H.-H. Li, Y.-R. Tang and Y.-F. Cai, Org. Lett., 2020, 22, 9016–9021 CrossRef CAS PubMed;
(f) Y. Xia, L. Lin, F. Chang, X. Fu, X. Liu and X.-M. Feng, Angew. Chem., Int. Ed., 2015, 54, 13748–13752 CrossRef CAS PubMed;
(g) Q.-K. Kang, L.-J. Wang, Q.-J. Liu, J.-F. Li and Y. Tang, J. Am. Chem. Soc., 2015, 137, 14594–14597 CrossRef CAS PubMed;
(h) O. Lifchits, D. Alberico, I. Zakharian and A. B. Charette, J. Org. Chem., 2008, 73, 6838–6840 CrossRef CAS PubMed;
(i) O. Lifchits and A. B. Charette, Org. Lett., 2008, 10, 2809–2812 CrossRef CAS PubMed;
(j) J. P. Qu, Y. Liang, H. Xu, X. L. Sun, Z. X. Yu and Y. Tang, Chem.–Eur. J., 2012, 18, 2196–2201 CrossRef CAS PubMed;
(k) S. M. Wales, M. M. Walker and J. S. Johnson, Org. Lett., 2013, 15, 2558–2561 CrossRef CAS PubMed;
(l) H. Xiong, H. Xu, S. Liao, Z. Xie and Y. Tang, J. Am. Chem. Soc., 2013, 135, 7851–7854 CrossRef CAS PubMed;
(m) H. Xu, J. P. Qu, S. Liao, H. Xiong and Y. Tang, Angew. Chem., Int. Ed., 2013, 52, 4004–4007 CrossRef CAS PubMed;
(n) J. Zhu, Y. Liang, L. Wang, Z. B. Zheng, K. N. Houk and Y. Tang, J. Am. Chem. Soc., 2014, 136, 6900–6903 CrossRef CAS PubMed;
(o) Q. J. Liu, W. G. Yan, L. Wang, X. P. Zhang and Y. Tang, Org. Lett., 2015, 17, 4014–4017 CrossRef CAS PubMed;
(p) H. P. Wang, H. H. Zhang, X. Q. Hu, P. F. Xu and Y. C. Luo, Eur. J. Org. Chem., 2015, 3486–3494 CrossRef CAS;
(q) Y. Xia, F. Z. Chang, L. L. Lin, Y. L. Xu, X. H. Liu and X.-M. Feng, Org. Chem. Front., 2018, 5, 1293–1296 RSC;
(r) S. Nicolai and J. Waser, Angew. Chem., Int. Ed., 2022, 61, e202209006 CrossRef CAS PubMed.
-
(a) Z. B. Zhu, L. P. Liu, L. X. Shao and M. Shi, Synlett., 2007, 1, 115–118 Search PubMed;
(b) S. Liao, X. L. Sun and Y. Tang, Acc. Chem. Res., 2014, 47, 2260–2272 CrossRef CAS PubMed;
(c) X. Bugaut, T. Constantieux, Y. Coquerel, J. Rpdriguez, J. Zhu, Q. Wang and M. X. Wang, Multicomponent Reactions in Organic Synthesis, Wiley-VCH, Weinheim, Germany, 2015, vol. 5, pp. 109–159 Search PubMed;
(d) H. Qian, D. Huang, G. Yan and L. Lu, Curr. Org. Chem., 2018, 22, 2055–2084 CrossRef CAS;
(e) Z. Liu, P. Sivaguru, G. Zanoni, E. A. Anderson and X. Bi, Angew. Chem., Int. Ed., 2018, 57, 1–6 CrossRef;
(f) M. K. Foumani, J. Conrad, W. Frey and U. Beifuss, J. Org. Chem., 2022, 87, 8316–8341 CrossRef PubMed;
(g) Y. Murakami, K. Hisano and H. Nishino, J. Org. Chem., 2022, 87, 8782–8787 CrossRef CAS PubMed;
(h) S. S. Sun, Z. Y. Mo, Y. Y. Chen and Y. L. Xu, J. Org. Chem., 2022, 87, 12447–12454 CrossRef CAS PubMed;
(i) H. Li, H. Gu, Y. Lu, N. Xu, N. Han, J. Li and J. Liu, J. Org. Chem., 2022, 87, 8142–8150 CrossRef CAS PubMed;
(j) Z. Zhong, M. Liang, Z. Zhang, H. Cui, N. Wang, S. Mai and H. Tao, Org. Lett., 2022, 24, 4850–4854 CrossRef CAS PubMed.
-
(a) D. Zhang, L. Yin, J. Zhong, Q. Cheng, H. Cai, Y. Chen and Q.-F. Zhang, Org. Biomol. Chem., 2020, 18, 6492–6496 RSC;
(b) D. Zhang, J. Zhong, L. Yin, Y. Chen, J. Man and Q.-F. Zhang, J. Org. Chem., 2020, 85, 5778–5786 CrossRef CAS PubMed;
(c) D. Zhang, H. Cai, Y. Chen, L. Yin, J. Zhong, Y. Zhang and Q.-F. Zhang, J. Org. Chem., 2020, 85, 14262–14270 CrossRef CAS PubMed;
(d) D. Zhang, Q. Cheng, L. Chen, H. Deng, H. Cai and Q.-F. Zhang, Org. Biomol. Chem., 2021, 19, 9645–9648 RSC;
(e) H. Deng, Q. Cheng, H. Cai, D. Zhang and Q.-F. Zhang, Tetrahedron Lett., 2023, 114, 154257 CrossRef CAS.
Footnote |
† Electronic supplementary information (ESI) available: Experimental details, characterization data of reactants and products, and copies of NMR spectroscopy. See DOI: https://doi.org/10.1039/d2ra08257h |
|
This journal is © The Royal Society of Chemistry 2023 |
Click here to see how this site uses Cookies. View our privacy policy here.