DOI:
10.1039/D3RA02262E
(Paper)
RSC Adv., 2023,
13, 16584-16601
Magnetic polyborate nanoparticles as a green and efficient catalyst for one-pot four-component synthesis of highly substituted imidazole derivatives†
Received
5th April 2023
, Accepted 6th May 2023
First published on 1st June 2023
Abstract
In this study, magnetic polyborate nanoparticles (MPBNPs) were prepared via a simple procedure from boric acid by using ball-milling and then characterized by various spectroscopic, microscopic and analytical methods including FT-IR, EDX, XRD, FESEM, VSM and TGA analysis. The obtained MPBNPs were further explored, as a green and highly efficient catalyst, in the multi-component synthesis of a wide range of tetra-substituted imidazoles from cascade cyclocondensation as well as in situ air oxidation of benzil or benzoin, aromatic aldehydes, primary amine and ammonium acetate in EtOH, as a green solvent, under reflux conditions. Additionally, environmentally friendly conditions for the preparation of the catalyst by the use of non-toxic reactants, facile procedure and high to excellent yields of the desired products as well as the use of a green solvent are some advantages of this new protocol.
1. Introduction
Nowadays, the development of simple, inexpensive, less hazardous and more efficient protocols for the synthesis of organic compounds in higher yields and atom efficiency as well as with shorter reaction times is one of the important goals of green chemistry. Following this goal, many catalytic systems have been investigated to facilitate the progress of organic reactions and be used in both fine and bulk chemicals production.1–11
Among all of these, magnetic nanoparticles (MNPs) have attracted lots of attention by providing promising properties as supports for different catalytic systems due to their appropriate surface area and magnetic properties. The magnetic feature of such catalysts facilitates their separation from the mixture after its completion. These magnetic catalysts have been utilized in Brønsted acid/or base, transitional metal, organo- and enzymatic catalysis reactions. Hence, being specifically robust, chemically stable as well as readily available with a naturally low toxicity and cost has made them efficient alternatives to other well-known catalyst supports especially silica and alumina.12–57 As the case of our study, imidazole derivatives are one of the important nitrogen-containing five-membered heterocyclic compounds. This is due to their essential role as an important scaffold in diverse active pharmaceutical ingredients (APIs) and biologically active molecules such as histidine, histamine, biotin, losartan, olmesartan, eprosartan, miconazole, ketoconazole, clotrimazole and trifenagrel (Fig. 1). Furthermore, they have been proved as efficient anti-cancer and anti-inflammation as well as anti-tuberculosis, antimicrobial and anti-anaphylaxis compounds.58–66 Moreover, highly-substituted 1,3-dialkylimidazoles have demonstrated their high potential in the form of ionic liquids, as green solvents, or precursors of N-heterocyclic carbenes (NHCs) as efficient organocatalysts or ligands in coordination chemistry in the recent decades.67–71 Indeed, the potency and vast application of imidazole derivatives can be attributed to their hydrogen bond donor–acceptor capability as well as high affinity for metals, such as the zinc, iron and magnesium present in many receptor active sites of the biological systems along with anti-corrosion property. Hence, diverse applications of compounds containing imidazole as a moiety highlights the necessity of achieving efficient protocols for the synthesis of corresponding highly substituted derivatives. Following this issue, multi-component reaction (MCR) of benzyl or benzoin with aldehydes, primary amines and ammonium acetate is one of the most convenient protocols for synthesis of multi-substituted imidazole derivatives.72–75
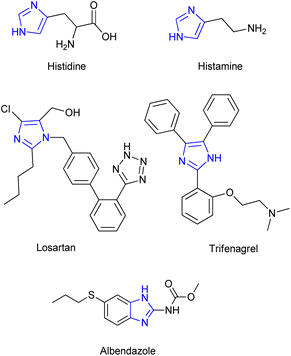 |
| Fig. 1 Structures of some biological molecules and active pharmaceutical ingredients (APIs) containing imidazole scaffold. | |
Different homogeneous or heterogeneous catalytic systems have been deployed for the multi-component synthesis of substituted imidazoles including ZSM-11 or HY zeolite, dimethylpyridinium trinitromethanide, 3-picolinic acid, silica sulfuric acid, ZrO2–Al2O3, ZrO2–β-cyclodextrin, nano-Al-MCM-41, triethylammonium acetate as an ionic liquid, I2, Keggin-type hetero polyacids, chitosan-coated Fe3O4 nanoparticles, Fe3O4–PEG–Cu, Boehmite nanoparticles, silica chloride, 2,6-pyromellitic diamide–diacid bridged mesoporous organosilica nanospheres, (N2H5)2SiF6 and Fe3O4/SiO2 decorated trimesic acid-melamine.6,40,76–82 Despite of their merits, there are also some disadvantages associated with these and similar procedures. These disadvantages include the use of hazardous or expensive reagents, low stability or recyclability of the catalysts as well as yields of desired products, pollution generated during the catalyst preparation, long reaction times and difficult work-up steps. Therefore, development of the productive, green and inexpensive approaches for the synthesis of multi-substituted imidazoles would be very desirable.83
In this work, we have presented magnetic polyborate nanoparticles (MPBNPs), as an environmental-friendly, inexpensive and efficient catalyst, in the one-pot four-component synthesis of 1,2,4,5-tetrasubstituted imidazoles in EtOH, as a green solvent, under reflux conditions (Scheme 1). Key features of this procedure are high to excellent yields of products, environmentally benign conditions, short reaction time and easy work-up that have made this method unique compared to others. Moreover, the catalyst is reusable at least after five runs with negligible loss in its function.
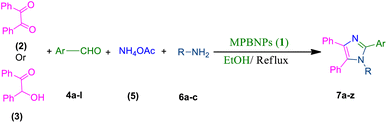 |
| Scheme 1 One-pot four-component synthesis of tetra-substituted imidazoles 7a–z catalyzed by the magnetic polyborate nanoparticles (MPBNPs, 1). | |
2. Experimental
2.1. General information
All chemicals were purchased from Merck and Fluka companies and used as received, except benzaldehyde (4d), thiophene-2-carbaldehyde (4h), furfural (4k) and aniline (6b), which fresh distilled samples were used. The ball mill was a Retsch MM 400 swing mill. 10 ml stainless steel ball mill vessels and two stainless steel balls with 12 mm diameter were used, and the milling frequency was set at 20 Hz at the ambient temperature. Melting points were recorded by using an Electrothermal IA 9000 apparatus. Purity of the chemicals and completion of the reactions was monitored by thin-layer chromatography (TLC) using ethyl acetate and n-hexane as eluting solvents. 1H NMR spectra of products were measured with VARIAN – INOVA 500 MHz in DMSO or CDCl3 as solvent. All products are known and characterized by measuring of their melting points (Tables 2and 3) as well as obtaining of their FT-IR and 1H NMR spectra and comparison with the literature data.
2.2. Preparation method of polyborate
10.0 g of boric acid was heated at 200 °C while stirring for 5 h to form polyborate. Then, the obtained polyborate was converted into nanoscale by using ball milling for 20 min, and the milling frequency was set at 20 Hz. The structure of the obtained polyborate nanoparticles was confirmed by using FT-IR technique.
2.3. Preparation method of magnetic polyborate (MPBNPs, 1)
MPBNPs were prepared from co-precipitation of ferrous and ferric salts with polyborate nanoparticles. First, FeCl3·6H2O (4.0 mmol, 1.08 g) and FeCl2·4H2O (2.0 mmol, 0.40 g) were dissolved in 100 ml of distilled water. Then, pH of the obtained mixture was adjusted into 4.0 from 1.5 by addition of NaOH (1.0 M). Next, another mixture containing 100 mg of polyborate dispersed in 20 ml deionized water under ultrasonic was poured into the obtained mixture by vigorous stirring. After 30 min mixing, pH of the mixture was adjusted into 10.0 using NaOH (1.0 M). Afterward, the obtained mixture was stirred for 1 h and the nanoparticles of magnetic polyborate were washed with double distilled water (2.0 ml) three times and finally separated by using an external magnet and put into an oven at 50 °C to dry for 5 h.
2.4. General procedure for the synthesis of 1,2,4,5-tetrasubstituted imidazoles 7a–z catalyzed by the MPBNPs (1)
10.0 mg of the MPBNPs catalyst (1) was added to a round-bottom flask containing benzyl or benzoin (2 or 3, 1.0 mmol), aldehyde (4a–l, 1.0 mmol), primary amine (6a, 1.0 mmol) and ammonium acetate (5, 1.75 mmol) in EtOH (2.5 ml) and the obtained mixture was heated under reflux conditions. After completion of the reaction monitored by TLC, additional EtOH (2–3 ml) was used to dissolve the products and remain the insoluble MPBNPs (1). The obtained mixture was heated and filtered off to separate the magnetic catalyst 1 using an external magnet. Distilled water was added dropwise to the filtrate at 50 °C to afford pure crystals of the desired products 7a–p. The separated magnetic catalyst 1 was suspended in EtOH (2 ml) and filtered off three times and then dried in an oven at 50 °C for 5 h before using in the next runs.
2.5. Spectral data of the selected derivatives of imidazoles (7e, 7h, 7k, 7l, 7m, 7w, 7r)
2.5.1. 1-Benzyl-2-(4-methoxyphenyl)-4,5-diphenyl-1H-imidazole (7e). Mp: 144–147 °C; white solid; FTIR (KBr; cm−1): 3026, 2929, 2361, 1605, 1530, 1482, 1449; 1H NMR (500 MHz, CDCl3, ppm): δ 7.62–7.56 (m, 3H), 7.42–7.10 (m, 12H), 6.92 (d, J = 8.0 Hz, 2H), 6.82 (dd, J = 7.7, 1.7 Hz, 2H), 5.09 (s, 2H), 3.88–3.76 (m, 3H).
2.5.2. 1-Benzyl-4,5-diphenyl-2-(thiophen-2-yl)-1H-imidazole (7h). Mp: 160–161 °C; yellow solid; FTIR (KBr; cm−1): 3427, 3050, 2376, 1598, 1496, 1444; 1H NMR (500 MHz, DMSO-d6, ppm): δ 7.61 (dt, J = 7.0, 1.5 Hz, 1H), 7.50–7.39 (m, 6H), 7.34–7.12 (m, 8H), 7.06 (m, 1H), 6.92 (d, J = 7.6 Hz, 2H), 5.26 (s, 2H).
2.5.3. 2-(3-Nitrophenyl)-1,4,5-triphenyl-1H-imidazole (7k). Mp: 258–259 °C; yellow solid; FTIR (KBr; cm−1): 3427, 1525, 1344, 766, 697; 1H NMR (500 MHz, DMSO-d6, ppm): δ 8.96 (s, 1H), 8.52 (d, J = 8.0 Hz, 1H), 8.22 (d, J = 8.0 Hz, 1H), 8.18–8.10 (m, 1H), 7.78 (td, J = 8.2, 1.7 Hz, 1H), 7.62–7.19 (m, 14H).
2.5.4. 1,2,4,5-Tetraphenyl-1H-imidazole (7l). Mp: 219–221 °C; white solid; FTIR (KBr; cm−1): 3048, 2360, 1596, 1498, 770, 692; 1H NMR (500 MHz, CDCl3, ppm): δ 7.62 (s, 5H), 7.45 (s, 5H), 7.14 (s, 5H), 7.05 (s, 5H).
2.5.5. N,N-Dimethyl-4-(1,4,5-triphenyl-1H-imidazol-2-yl)aniline (7m). Mp: 206–2027 °C; brown solid; FTIR (KBr; cm−1): 3422, 2926, 2364, 1722, 1612, 1488, 1442, 1370, 816, 694; 1H NMR (500 MHz, DMSO-d6, ppm): δ 7.60–7.50 (m, 1H), 7.47 (d, J = 7.5 Hz, 1H), 7.35–7.30 (m, 6H), 7.21 (m, 10H), 6.57 (d, J = 8.5 Hz, 2H), 2.88 (s, 6H).
2.5.6. 2-(4-Nitrophenyl)-4,5-diphenyl-1-(p-tolyl)-1H-imidazole (7r). Mp: 218–219 °C; yellow solid; FTIR (KBr; cm−1): 3032, 2922, 2852, 2366, 1532, 1352, 750, 694; 1H NMR (500 MHz, DMSO-d6, ppm): δ 7.98 (s, 1H), 7.73–7.56 (m, 3H), 7.50–7.39 (m, 2H), 7.33 (dt, J = 6.0, 3.5 Hz, 3H), 7.27–7.21 (m, 4H), 7.20–7.14 (m, 1H), 7.03 (t, J = 10.5 Hz, 4H), 2.18 (s, 3H).
2.5.7. 2-(4-Methoxyphenyl)-4,5-diphenyl-1-(p-tolyl)-1H-imidazole (7w). Mp: 179–180 °C; white solid; FTIR (KBr; cm−1): 2922, 2376, 1606, 1514, 1438, 1368, 1022, 824, 776, 698, 526; 1H NMR (500 MHz, DMSO-d6, ppm): δ 7.49–7.44 (m, 2H), 7.34–7.26 (m, 5H), 7.26–7.19 (m, 4H), 7.19–7.06 (m, 5H), 6.88–6.82 (m, 2H), 3.73 (s, 3H), 2.26 (s, 3H).
3. Results and discussion
3.1. Characterization of the magnetic polyborate nanoparticles (1)
Prepared MPBNPs were characterized by different spectroscopic, microscopic and analytical methods and techniques such as Fourier transform infrared spectroscopy (FT-IR), X-ray powder diffraction (XRD), field emission scanning electron microscopy (FESEM), energy-dispersive X-ray (EDX) spectroscopy, vibrating sample magnetometer (VSM) and thermogravimetric analysis (TGA).
3.1.1. Fourier transform infrared (FT-IR) analysis. As shown in Fig. 2, Fourier transform infrared spectroscopy for primary identification of the MPBNPs catalyst (1) was employed. The band appeared at 638 cm−1 is related to stretching vibrations of the Fe–O bonds of Fe3O4 magnetic nanoparticles.80 Also, the absorption bands at 1232 and 1430 cm−1 can be attributed to the symmetric and asymmetric stretching vibrations of B–OH and B–O bonds of polyborate nanoparticles, respectively.84 On the other hand, the absorption band in the range of 3208–3410 cm−1 corresponds to the stretching vibrations of the acidic OH groups in structure of MPBNPs, which is a broad band due to strong intermolecular hydrogen bonding between OH groups. In general, according to these observed data it can be suggested that the MPBNPs (1) have been prepared successfully.
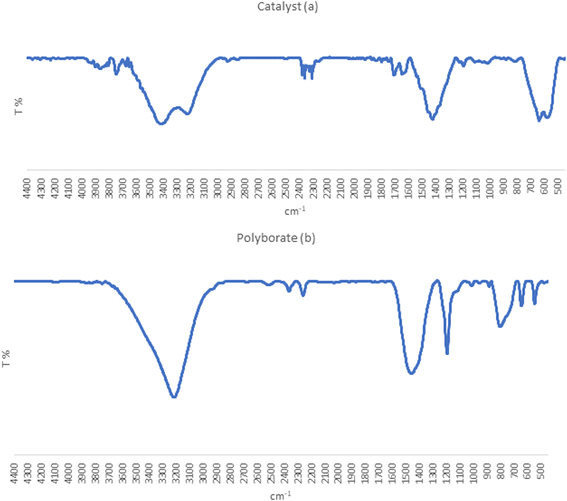 |
| Fig. 2 Fourier transform infrared spectra of (a) MPBNPs (1) and (b) polyborate. | |
3.1.2. X-Ray powder diffraction analysis. The XRD pattern was obtained with copper target (λ = 1.54 Å) at the range of 10–80° for 2θ. Main diffraction peaks at 2θ = 27.06, 32.64, 33.83, 35.43, 36.36, 42.84, 45.38, 50.10, 57.80° are adapted with the reference patterns of corresponding structure of MPBNPs (Fig. 3). Also, the crystallographic structure of Fe3O4 did not change during the process for preparation of the catalyst 1. Moreover, the size of nanoparticles was calculated to be about 35.28 nm based on the Debye–Scherrer equation according to peak of 2θ = 32.64°.
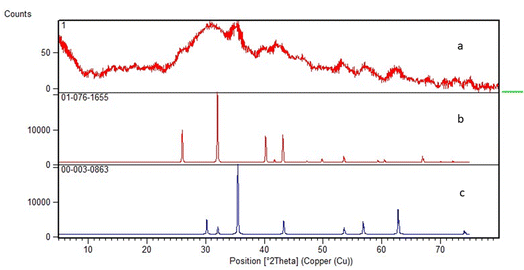 |
| Fig. 3 XRD pattern of (a) MPBNPs catalyst (1); (b) Fe3O4 and (c) B2O3. | |
3.1.3. Field emission scanning electron microscopy (FESEM) analysis. FESEM analysis was performed to investigate the size and morphology of the catalyst 1. The recorded images proved the nano dimension of particles besides spherical nature of them in the structure of catalyst (Fig. 4). Also, according to the FESESM micrograms the average size of nanoparticles is estimated to be about 36 nm that demonstrates good consistency with the XRD results.
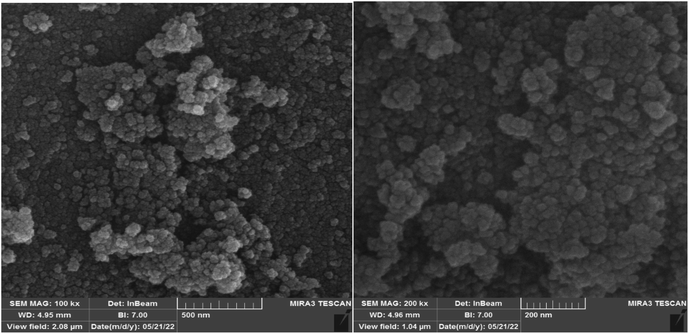 |
| Fig. 4 FESEM images of the MPBNPs catalyst (1). | |
3.1.4. Energy dispersive X-ray (EDX) and mapping analysis. The presence of anticipated elements such as boron, oxygen and iron was justified by EDX technique. Furthermore, uniform distribution of boron atoms in the structure of the catalyst is clear in the elemental mapping analysis (Fig. 5).
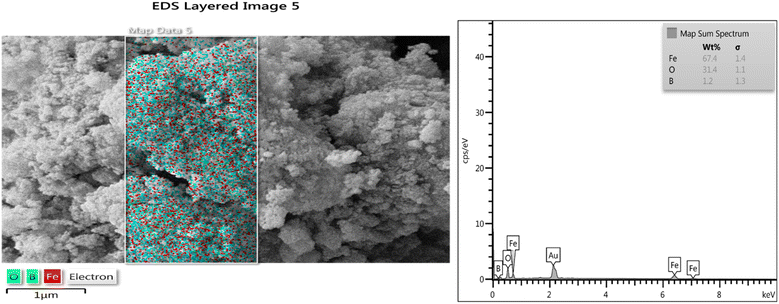 |
| Fig. 5 EDX and mapping analysis of sample nanoparticles (1). | |
3.1.5. Vibrating sample magnetometer (VSM). The magnetic property of MPBNPs (1) was examined by vibrating sample magnetometer. It was measured out at room temperature under magnetic field −8500 to +8500 oersted. According to values obtained from VSM analysis, the magnetization saturation for MPBNPs is 24.03715 emu g−1. The S-shaped curve shown in Fig. 6 proves the magnetic behavior of the MPBNPs catalyst (1).85
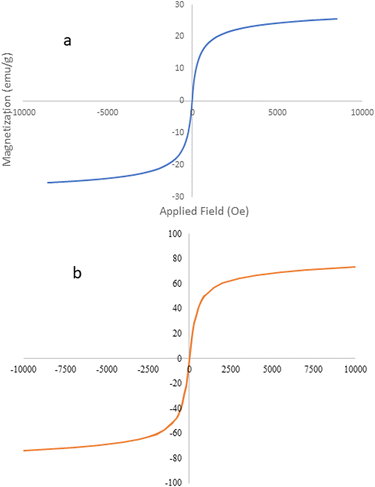 |
| Fig. 6 VSM pattern of the magnetic polyborate nanoparticles (a) vs. the Fe3O4 reference (b).56 | |
3.1.6. Thermogravimetric analysis (TGA). The thermal stability of the MPBNPs nanoparticles (1) was investigated by TGA-DTG analysis at the range of 25–700 °C. As shown in Fig. 7, thermogram illustrate two steps for weight losses. The first step is between 25 to 166 °C, which was associated with physically or chemically adsorbed water in the structure of the catalyst with 3.75% loss in the initial weight of sample. The second step of weight loss occurred between 166 and 700 °C, which counted 5.80% of the total weight and can be attributed to condensation of OH groups on the surface of MPBNPs (1).
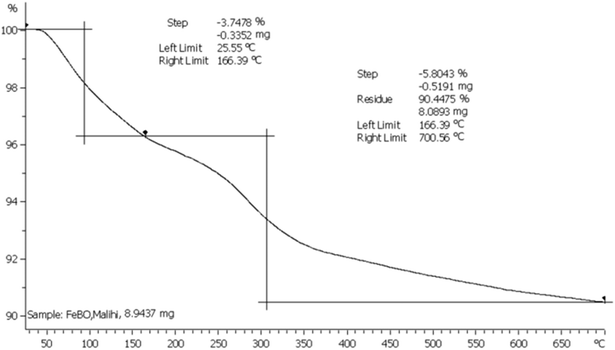 |
| Fig. 7 TGA curve of the MPBNPs catalyst (1). | |
3.2. Optimization of conditions in synthesis of tetra-substituted imidazoles
To show the efficiency of the MPBNPs catalyst (1) for the synthesis of the 1,2,4,5-tetrasubstituted imidazoles, the reaction of benzil (2, 1.00 mmol), 4-chlorobenzaldehyde (4a, 1.00 mmol), ammonium acetate (5, 1.75 mmol) and benzylamine (6a, 1.00 mmol) was carried out as a model reaction (Table 1). The reaction conditions were optimized into the best catalyst loading, solvent and energy input for the synthesis of 1-benzyl-2-(4-chlorophenyl)-4,5-diphenyl-1H-imidazole (7a). The results are shown in Table 1. Indeed, the yeild of the desired product 7a in the absence of catalyst 1 even after 4 h in the EtOH at room temperature was trace (entry 1). Moreover, the effect of the catalyst loading to proceed the model reaction was investigated in the next experiments (entries 10–13). Whereas loading of 5.0 mg of the MPBNPs catalyst (1) increased the yield of desired product 7a in EtOH at room temperature after 60 min to about 45%, the reaction yield increased to about 69% under reflux conditions with same amount of the catalyst 1 loading. Also, the reaction was examined in other solvents such as H2O, MeOH, EtOAc, CH3CN and THF under the same conditions and all of them afforded lower yields than EtOH (entries 2–9). Polarity of EtOH and solubility of the components of reaction in EtOH beside formation of hydrogen bonds with the produced water from cycloaddition are some rational reasons for getting higher yields by using EtOH as a solvent. On the other hand, when the model reaction was carried out in EtOH under reflux conditions by loading 5.0 mg of the catalyst 1 afforded higher yield of desired product 7a. Furthermore, the amount of the obtained product 7a under the same condition was increased significantly after loading 10.0 mg of the MPBNPs catalyst (1) to 96% (entry 13). Additionally, completion of the model reaction in the presence of PB and Fe3O4 individually was examined (entries 14 and 15) and synergic effect between them justifies promising results. Finally, the effect of ultrasound and microwave radiation on the reaction rate was investigated (entries 16 and 17). Consequently, 10.0 mg MPBNPs (1) loading in EtOH under reflux conditions was chosen as the optimized conditions in the next experiments (Tables 2 and 3).
Table 1 Systematic study for optimization of four-component reaction between benzil (2), 4-chlorobenzaldehyde (4a), ammonium acetate (5) and benzylamine (6a) under different conditionsa

|
Entry |
Catalyst loading (mg) |
Solvent |
Temp. (°C) |
Time (min) |
Yieldb (%) |
Reaction conditions: benzil (2, 1.0 mmol), 4-chlorobenzaldehyde (4a, 1.0 mmol), ammonium acetate (5, 1.75 mmol), benzylamine (6a, 1.0 mmol) and magnetic polyborate catalyst (1) at different conditions. Isolated yield. The use of ultrasound as energy source. The use of microwave as energy source. |
1 |
— |
EtOH |
r.t |
240 |
Trace |
2 |
MPBNPs (1, 5.0 mg) |
MeOH |
r.t |
60 |
35 |
3 |
MPBNPs (1, 5.0 mg) |
H2O |
r.t |
60 |
28 |
4 |
MPBNPs (1, 5.0 mg) |
EtOH |
r.t |
60 |
45 |
5 |
MPBNPs (1, 5.0 mg) |
EtOAc |
r.t |
60 |
21 |
6 |
MPBNPs (1, 5.0 mg) |
CH3CN |
r.t |
60 |
30 |
7 |
MPBNPs (1, 5.0 mg) |
THF |
r.t |
60 |
Trace |
8 |
MPBNPs (1, 5.0 mg) |
H2O/MeOH |
r.t |
60 |
46 |
9 |
MPBNPs (1, 5.0 mg) |
H2O/EtOH |
r.t |
60 |
53 |
10 |
MPBNPs (1, 2.5 mg) |
ETOH |
Reflux |
40 |
25 |
11 |
MPBNPs (1, 5.0 mg) |
ETOH |
Reflux |
40 |
69 |
12 |
MPBNPs (1, 7.5 mg) |
ETOH |
Reflux |
40 |
88 |
13 |
MPBNPs (1, 10.0 mg) |
ETOH |
Reflux |
40 |
96 |
14 |
PB (10.0 mg) |
ETOH |
Reflux |
40 |
66 |
15 |
Fe3O4 (10.0 mg) |
ETOH |
Reflux |
40 |
44 |
16c |
MPBNPs (10.0 mg) |
ETOH |
Ultrasound |
40 |
67 |
17d |
MPBNPs (10.0 mg) |
ETOH |
Microwave |
40 |
85 |
Table 2 Synthesis of 1,2,4,5-tetrasubstituted imidazoles via one-pot four-component condensation of benzil (2), aromatic aldehydes 4a–l, ammonium acetate (5) and primary amines (6a–c) in the presence of MPBNPs catalyst (1)a
Table 3 Synthesis of 1,2,4,5-tetrasubstituted imidazoles via one-pot four-component condensation of benzoin (3), aromatic aldehydes 4, ammonium acetate (5) and primary amines (6a–c) in the presence ofMPBNPs catalyst (1)a
3.3. The proposed mechanism for the synthesis of tetra-substituted imidazole derivatives in the presence of MPBNPs catalyst (1)
The most probable mechanism for the formation of tetra-substituted imidazoles has been represented in Scheme 2. In fact, the electrophilicity of the carbonyl groups of aldehydes 4 is increased by involving in the interaction with the Lewis acidic centers of both B and Fe atoms of the MPBNPs catalyst (1). Then, nucleophiles including ammonia (5′) and amines 6 can be added to the activated carbonyl group of aldehydes 4 to afford the corresponding imine (I) and aminal (II) intermediates, respectively. After that, the obtained intermediate II reacts with the activated benzil (2) or benzoin (3) carbonyl groups to produce cyclic intermediate III and IV, respectivly. The later intermediate is formed by losing one molecules of water through simple imine condensation and subsequent air oxidation in the case of benzoin. Desired imidazole derivatives 7 are finally produced after a [1,5-H] shift and the liberated MPBNPs (1) can start a new cycle of its catalytic activity.40,77,82
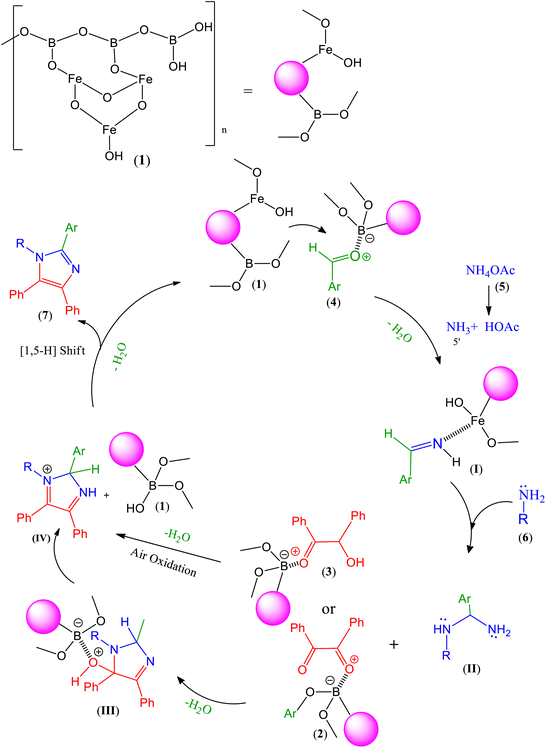 |
| Scheme 2 Feasible mechanism for the synthesis of 1,2,4,5-tetra-substituted imidazoles 7 in the presence of MPBNPs (1). | |
The reusability of the MPBNPs catalyst (1) was also investigated for the model reaction in another part of our study. After completion of the reaction, the catalyst 1 was separated using an external magnet and suspended in EtOH. Afterward, it was filtered off three times and then dried in an oven at 50 °C for 5 h before using in the next runs. The obtained results are summarized in Fig. 8. As it can be observed, the catalyst 1 is reusable for at least five runs with negligible loss in its activity.
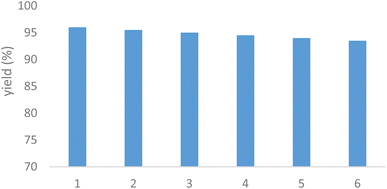 |
| Fig. 8 Reusability of the heterogeneous MPBNPs catalyst (1) in the model reaction to afford 7a. | |
To demonstrate the catalytic efficiency of the MPBNPs catalyst (1) for the synthesis of 1,2,4,5-tetrasubstituted imidazoles, its performance has been compared with some acidic catalytic systems in the model reaction. The results are summarized in Table 4. The provided data clearly illustrate that the MPBNPs catalyst (1) is superior to many introduced catalytic systems for the synthesis of 1,2,4,5-tetrasubstituted imidazole derivatives in terms of the catalyst loading, shorter reaction time, the use of a green solvent, working at lower temperature and reusability of the catalyst for more runs with keeping its activity.
Table 4 Comparative data for the activity of different catalysts for the synthesis of 7a
Entry |
Catalyst |
Catalyst loading |
Reaction conditions |
Time (min) |
Yield (%) |
1 |
3-Picolinic acid6 |
12 mg |
EtOH/80 °C |
120 |
96 |
2 |
PANI–FeCl3 (ref. 86) |
200 mg |
CH3CN/reflux |
1440 |
83 |
3 |
PMAMOSa40 |
15 mg |
EtOH/reflux |
45 |
95 |
4 |
Nano-TiCl4·SiO2 (ref. 87) |
100 mg |
Solvent-free/130 °C |
30 |
95 |
5 |
K5CoW12O40·3H2O88 |
32 |
Solvent-free/140 °C |
180 |
90 |
6 |
Magnetic polyborate nanoparticles |
10 mg |
EtOH/reflux |
40 |
96 (This work) |
7 |
Sulfonic acid functionalized silica89 |
20 mg |
Solvent-free/140 °C |
100 |
94 |
4. Conclusions
In summary, an efficient, environmentally benign, non-hazardous and expeditious protocol for the synthesis of highly-substituted imidazoles has been described in this work. Simple preparation of the magnetic polyborate nanoparticles (MPBNPs) catalyst by using ball-milling technique and its easy separation from the reaction mixture by an external magnetic field as well as accessibility of the reagents are key features of this new protocol and its superiorities to other catalytic systems. The new MPBNPs were applied in the condensation of benzil (or benzoin), aromatic carbocyclic and heterocyclic aldehydes, ammonium acetate and primary amines to afford the corresponnding highly-substituted imidazoles, which are greatly important in numerous of biological and pharmacological compounds. Moreover, it was found that the use of EtOH, as a green solvent, affords the highest yields of the desired products.
Conflicts of interest
There are no conflicts to declare.
Acknowledgements
We are grateful for the financial support from The Research Council of Iran University of Science and Technology (IUST), Tehran, Iran (Grant No.: 160/22061) is highly appreciated. The partial financial support of The Iran Nanotechnology Initiative Council (INIC) is gratefully acknowledged. Mohammad Eslami is also very thankful for the support of the Iran National Science Foundation (INSF).
References
- R. V. Orru and E. Ruijter, Synthesis of heterocycles via multicomponent reactions II, Springer Science & Business Media, 2010 Search PubMed.
- J. Zhu and H. Bienaymé, Multicomponent reactions, John Wiley & Sons, 2006 Search PubMed.
- D. J. Ramón and M. Yus, Angew. Chem., Int. Ed., 2005, 44, 1602–1634 CrossRef PubMed.
- A. Keivanloo, M. Bakherad, E. Imanifar and M. Mirzaee, Appl. Catal., A, 2013, 467, 291–300 CrossRef CAS.
- A. Khazaei, A. R. Moosavi-Zare, F. Gholami and V. Khakyzadeh, Appl. Organomet. Chem., 2016, 30, 691–694 CrossRef CAS.
- A. Z. Al Munsur, H. N. Roy and M. K. Imon, Arabian J. Chem., 2020, 13, 8807–8814 CrossRef CAS.
- J. J. Gabla, S. R. Mistry and K. C. Maheria, Catal. Sci. Technol., 2017, 7, 5154–5167 RSC.
- K. C. Nicolaou, T. Montagnon and S. A. Snyder, Chem. Commun., 2003, 551–564, 10.1039/b209440c.
- B. H. Rotstein, S. Zaretsky, V. Rai and A. K. Yudin, Chem. Rev., 2014, 114, 8323–8359 CrossRef CAS PubMed.
- C. de Graaff, E. Ruijter and R. V. Orru, Chem. Soc. Rev., 2012, 41, 3969–4009 RSC.
- M. G. Dekamin, M. Azimoshan and L. Ramezani, Green Chem., 2013, 15, 811–820 RSC.
- B. Jiang, T. Rajale, W. Wever, S. J. Tu and G. Li, Chem.–Asian J., 2010, 5, 2318–2335 CrossRef CAS PubMed.
- X. B. Wang, L. He, T. Y. Jian and S. Ye, Chin. Chem. Lett., 2012, 23, 13–16 CrossRef CAS.
- M. A. Silver and T. E. Albrecht-Schmitt, Coord. Chem. Rev., 2016, 323, 36–51 CrossRef CAS.
- L. Lv, Y. Chen, A. Shatskiy, J. Q. Liu, X. Liu, M. D. Kärkäs and X. S. Wang, Eur. J. Org. Chem., 2021, 2021, 964–968 CrossRef CAS.
- S. Balalaie and A. Arabanian, Green Chem., 2000, 2, 274–276 RSC.
- R. C. Cioc, E. Ruijter and R. V. Orru, Green Chem., 2014, 16, 2958–2975 RSC.
- M. G. Dekamin, Z. Karimi, Z. Latifidoost, S. Ilkhanizadeh, H. Daemi, M. R. Naimi-Jamal and M. Barikani, Int. J. Biol. Macromol., 2018, 108, 1273–1280 CrossRef CAS PubMed.
- D. M. Perrine, J. T. Ross, S. J. Nervi and R. H. Zimmerman, J. Chem. Educ., 2000, 77, 1479 CrossRef CAS.
- B. Dai, Y. Duan, X. Liu, L. Song, M. Zhang, W. Cao, S. Zhu, H. Deng and M. Shao, J. Fluorine Chem., 2012, 133, 127–133 CrossRef CAS.
- M. Rahman, A. K. Bagdi, D. Kundu, A. Majee and A. Hajra, J. Heterocycl. Chem., 2012, 49, 1224–1228 CrossRef CAS.
- Z. Tavakoli, M. Bagherneghad and K. Niknam, J. Heterocycl. Chem., 2012, 49, 634–639 CrossRef CAS.
- Y. Wan, G. x. Liu, L. l. Zhao, H. y. Wang, S. y. Huang, L. f. Chen and H. Wu, J. Heterocycl. Chem., 2014, 51, 713–718 CrossRef CAS.
- S. A. Laufer, W. Zimmermann and K. J. Ruff, J. Med. Chem., 2004, 47, 6311–6325 CrossRef CAS PubMed.
- M. M. Heravi, F. Derikvand and F. F. Bamoharram, J. Mol. Catal. A: Chem., 2007, 263, 112–114 CrossRef CAS.
- M. M. Heravi, E. Hashemi, Y. S. Beheshtiha, K. Kamjou, M. Toolabi and N. Hosseintash, J. Mol. Catal. A: Chem., 2014, 392, 173–180 CrossRef CAS.
- M. Kidwai, P. Mothsra, V. Bansal, R. K. Somvanshi, A. S. Ethayathulla, S. Dey and T. P. Singh, J. Mol. Catal. A: Chem., 2007, 265, 177–182 CrossRef CAS.
- A. Shaabani and A. Rahmati, J. Mol. Catal. A: Chem., 2006, 249, 246–248 CrossRef CAS.
- A. Teimouri and A. N. Chermahini, J. Mol. Catal. A: Chem., 2011, 346, 39–45 CrossRef CAS.
- H. R. Shaterian and M. Ranjbar, J. Mol. Liq., 2011, 160, 40–49 CrossRef CAS.
- L. Wu, X. Jing, H. Zhu, Y. Liu and C. Yan, J. Chil. Chem. Soc., 2012, 57, 1204–1207 CrossRef CAS.
- M. M. Khodaei, K. Bahrami and I. Kavianinia, J. Chin. Chem. Soc., 2007, 54, 829–833 CrossRef CAS.
- B. Sadeghi, B. Mirjalili, S. Bidaki and M. Ghasemkhani, J. Iran. Chem. Soc., 2011, 8, 648–652 CrossRef CAS.
- L. F. Tietze and A. Modi, Med. Res. Rev., 2000, 20, 304–322 CrossRef CAS PubMed.
- K. Bahrami, M. M. Khodaei and A. Nejati, Monatsh. Chem., 2011, 142, 159–162 CrossRef CAS.
- B. Das, J. Kashanna, R. A. Kumar and P. Jangili, Monatsh. Chem., 2013, 144, 223–226 CrossRef CAS.
- M. M. Heravi, F. Derikvand and M. Haghighi, Monatsh. Chem., 2008, 139, 31–33 CrossRef CAS.
- M. S. Patil, C. K. Khatri and G. U. Chaturbhuj, Monatsh. Chem., 2018, 149, 1453–1457 CrossRef CAS.
- C. Wu, A. Alqahtani, S. Sangtarash, A. Vezzoli, H. Sadeghi, C. M. Robertson, C. Cai, C. J. Lambert, S. J. Higgins and R. J. Nichols, Nanoscale, 2020, 12, 7914–7920 RSC.
- E. Valiey and M. G. Dekamin, Nanoscale Adv., 2022, 4, 294–308 RSC.
- S. Karami, M. G. Dekamin, E. Valiey and P. Shakib, New J. Chem., 2020, 44, 13952–13961 RSC.
- Z. Zarnegar and J. Safari, New J. Chem., 2014, 38, 4555–4565 RSC.
- D. A. Shabalin and J. E. Camp, Org. Biomol. Chem., 2020, 18, 3950–3964 RSC.
- Y. Zhu, C. Li, J. Zhang, M. She, W. Sun, K. Wan, Y. Wang, B. Yin, P. Liu and J. Li, Org. Lett., 2015, 17, 3872–3875 CrossRef CAS PubMed.
- R. B. Sparks and A. P. Combs, Org. Lett., 2004, 6, 2473–2475 CrossRef CAS PubMed.
- S. E. Wolkenberg, D. D. Wisnoski, W. H. Leister, Y. Wang, Z. Zhao and C. W. Lindsley, Org. Lett., 2004, 6, 1453–1456 CrossRef CAS PubMed.
- B. Maleki, G. E. Kahoo and R. Tayebee, Org. Prep. Proced. Int., 2015, 47, 461–472 CrossRef CAS.
- F. Zhang, Q. Gao, B. Chen, Y. Bai, W. Sun, D. Lv and M. Ge, Phosphorus, Sulfur Silicon Relat. Elem., 2016, 191, 786–789 CrossRef CAS.
- L. F. Tietze and N. Rackelmann, Pure Appl. Chem., 2004, 76, 1967–1983 CrossRef CAS.
- M. Esmaeilpour, J. Javidi, F. Dehghani and S. Zahmatkesh, Res. Chem. Intermed., 2017, 43, 163–185 CrossRef CAS.
- H. FaniMoghadam, M. G. Dekamin and N. Rostami, Res. Chem. Intermed., 2022, 48, 3061–3089 CrossRef CAS.
- H. Hanoon, E. Kowsari, M. Abdouss, M. Ghasemi and H. Zandi, Res. Chem. Intermed., 2017, 43, 4023–4041 CrossRef CAS.
- S. J. Saghanezhad, M. H. Sayahi, I. Imanifar, M. Mombeni and S. Deris Hamood, Res. Chem. Intermed., 2017, 43, 6521–6536 CrossRef CAS.
- M. G. Dekamin, E. Arefi and A. Yaghoubi, RSC Adv., 2016, 6, 86982–86988 RSC.
- M. G. Dekamin, S. Ilkhanizadeh, Z. Latifidoost, H. Daemi, Z. Karimi and M. Barikani, RSC Adv., 2014, 4, 56658–56664 RSC.
- T. T. Nguyen, N.-P. T. Le and P. H. Tran, RSC Adv., 2019, 9, 38148–38153 RSC.
- N. Rostami, M. G. Dekamin, E. Valiey and H. FaniMoghadam, RSC Adv., 2022, 12, 21742–21759 RSC.
- M. Salimi, M. A. Nasseri, T. D. Chapesshloo and B. Zakerinasab, RSC Adv., 2015, 5, 33974–33980 RSC.
- N. Thimmaraju and S. M. Shamshuddin, RSC Adv., 2016, 6, 60231–60243 RSC.
- E. Valiey and M. G. Dekamin, RSC Adv., 2022, 12, 437–450 RSC.
- Z. Zarnegar and J. Safari, RSC Adv., 2014, 4, 20932–20939 RSC.
- A. Akbari, M. G. Dekamin, A. Yaghoubi and M. R. Naimi-Jamal, Sci. Rep., 2020, 10, 10646 CrossRef CAS PubMed.
- N. Rostami, M. G. Dekamin, E. Valiey and H. Fanimoghadam, Sci. Rep., 2022, 12, 8642 CrossRef CAS PubMed.
- S. Safapoor, M. G. Dekamin, A. Akbari and M. R. Naimi-Jamal, Sci. Rep., 2022, 12, 10723 CrossRef CAS PubMed.
- M. Sam, M. G. Dekamin and Z. Alirezvani, Sci. Rep., 2021, 11, 2399 CrossRef CAS PubMed.
- S. Rostamnia and E. Doustkhah, Synlett, 2015, 26, 1345–1347 CrossRef CAS.
- T. D. A. Kumar, N. Yamini, C. Subrahmanyam and K. Satyanarayana, Synth. Commun., 2014, 44, 2256–2268 CrossRef CAS.
- A. Davoodnia, M. M. Heravi, Z. Safavi-Rad and N. Tavakoli-Hoseini, Synth. Commun., 2010, 40, 2588–2597 CrossRef CAS.
- M. G. Dekamin, M. Eslami and A. Maleki, Tetrahedron, 2013, 69, 1074–1085 CrossRef CAS.
- A. Padwa and S. K. Bur, Tetrahedron, 2007, 63, 5341 CrossRef CAS PubMed.
- S. Samai, G. C. Nandi, P. Singh and M. Singh, Tetrahedron, 2009, 65, 10155–10161 CrossRef CAS.
- C. Mukhopadhyay, P. K. Tapaswi and M. G. Drew, Tetrahedron Lett., 2010, 51, 3944–3950 CrossRef CAS.
- K. Niknam, A. Deris, F. Naeimi and F. Majleci, Tetrahedron Lett., 2011, 52, 4642–4645 CrossRef CAS.
- M. S. Patil, A. V. Palav, C. K. Khatri and G. U. Chaturbhuj, Tetrahedron Lett., 2017, 58, 2859–2864 CrossRef CAS.
- D. S. Rekunge, C. K. Khatri and G. U. Chaturbhuj, Tetrahedron Lett., 2017, 58, 4304–4307 CrossRef CAS.
- R. Rossi, G. Gaetano, G. Casotti, C. Manzini and M. Lessi, Adv. Synth. Catal., 2019, 361, 2737–2803 CrossRef CAS.
- M. A. Zolfigol, S. Baghery, A. R. Moosavi-Zare and S. M. Vahdat, RSC Adv., 2015, 5, 32933–32940 RSC.
- S. S. Dipake, V. D. Ingale, S. A. Korde, M. K. Lande, A. S. Rajbhoj and S. T. Gaikwad, RSC Adv., 2022, 12, 4358–4369 RSC.
- B. Sadeghi, B. B. F. Mirjalili and M. M. Hashemi, Tetrahedron Lett., 2008, 49, 2575–2577 CrossRef CAS.
- K. Sivakumar, A. Kathirvel and A. Lalitha, Tetrahedron Lett., 2010, 51, 3018–3021 CrossRef CAS.
- F. El hajri, Z. Benzekri, H. Anahmadi, S. Sibous, A. Ouasri, A. Souizi, A. Hassikou, A. Rhandour and S. Boukhris, Inorg. Chim. Acta, 2022, 536, 120915 CrossRef CAS.
- B. Fattahi and M. G. Dekamin, Sci. Rep., 2023, 13, 401 CrossRef CAS PubMed.
- I. V. Machado, J. R. Dos Santos, M. A. Januario and A. G. Corrêa, Ultrason. Sonochem., 2021, 78, 105704 CrossRef CAS PubMed.
- P. Qi, S. Wang, W. Wang, J. Sun, H. Yuan and S. Zhang, Int. J. Biol. Macromol., 2022, 205, 261–273 CrossRef CAS PubMed.
- Z. Alirezvani, M. G. Dekamin and E. Valiey, Sci. Rep., 2019, 9, 17758 CrossRef PubMed.
- M. Abdollahi-Alibeik and M. Moosavifard, Synth. Commun., 2010, 40, 2686–2695 CrossRef CAS.
- B. F. Mirjalili, A. H. Bamoniri and L. Zamani, Sci. Iran., 2012, 19, 565–568 CrossRef CAS.
- L. Nagarapu, S. Apuri and S. Kantevari, J. Mol. Catal. A: Chem., 2007, 266, 104–108 CrossRef CAS.
- G. Mohammadi Ziarani, Z. Dashtianeh, M. Shakiba Nahad and A. Badiei, Arabian J. Chem., 2015, 8, 692–697 CrossRef CAS.
|
This journal is © The Royal Society of Chemistry 2023 |
Click here to see how this site uses Cookies. View our privacy policy here.