DOI:
10.1039/D3RA02524A
(Paper)
RSC Adv., 2023,
13, 25888-25894
Ternary choline chloride/benzene sulfonic acid/ethylene glycol deep eutectic solvents for oxidative desulfurization at room temperature†
Received
16th April 2023
, Accepted 5th June 2023
First published on 30th August 2023
Abstract
Deep eutectic solvents (DESs) have been extensively studied as promising green solvents to attain a better removal efficiency of sulfide. A new DES system formed from choline chloride (ChCl), benzene sulfonic acid (BSA), and ethylene glycol (EG) as a class of ternary DESs was prepared and used in the oxidative desulfurization (ODS) of different sulfides. Ternary DESs have distinct advantages such as volatility and high activity compared with organic acid-based binary DESs. Under the optimum conditions with VDES/VOil = 1
:
5, O/S (molar ratio of oxygen to sulfur) = 5, and T = 25 °C, the desulfurization efficiencies of dibenzothiophene (DBT), 4,6-dimethyldibenzothiophene (4,6-DMDBT), and benzothiophene (BT) were all achieved to 100% in 2 h. Through experimental and density functional theory (DFT) calculation methods, this new system as a class of ternary DESs shows good stability and excellent desulfurization performance at room temperature. The investigation of this study could supply a new idea of ternary DESs for oxidative desulfurization.
1. Introduction
At present, fuel oils like diesel and gasoline remain some of the main sources of energy.1 Accordingly, SOx produced by the combustion of sulfur compounds is one of the main causes of environmental pollution such as haze, PM2.5, acid rain, and so on.2–4 As a result, stringent environmental legislation has been enacted around the world to limit the sulfur content of fuels.5,6 For example, the U.S. government issued environmental regulations in 2006, limiting the sulfur content in diesel to below 15 μg/g.7,8
Therefore, deep desulfurization technologies including hydrodesulfurization (HDS) and non-hydrodesulfurization such as extractive desulfurization (EDS), biodesulfurization (BDS), absorbent desulfurization (ADS), and oxidative desulfurization (ODS) have become hot spots.9–15 Compared with HDS with severe reaction conditions and a huge cost of energy, oxidative desulfurization has great advantages in terms of reaction conditions (atmospheric pressure, low temperature) and low energy consumption for the deep desulfurization technology to achieve 100% desulfurization efficiency.16 Therefore, oxidative desulfurization (ODS) is one of the most promising processes of desulfurization for industrial applications.17 Ye et al. synthesized defect-rich titanium terephthalate with the assistance of acetic acid for oxidative desulfurization.18 Hao et al. studied hierarchically porous Zr-based MOF (HP-Zr-MOF) as a catalyst in oxidative desulfurization (ODS).19,20 But the organic solvents served as polar solvents to remove the corresponding sulfones from oil are flammable and volatile, which would lead to security issues and further environmental pollution.21 As a result, the study of green solvents is expected to achieve environmental protection. Ionic liquids (ILs) oxidative desulfurization system has begun to enter field of vision.22–27 But ILs are expensive, tough to purify, and low biodegradable. It is vital to investigate a novel type of green solvents.
Deep eutectic solvents (DESs) are eutectic mixtures composed of hydrogen-bond acceptor (HBA) and hydrogen-bond donor (HBD) and are formed by hydrogen bond interaction.28–30 DESs with the advantages of low cost and low vapor pressure are regarded as a novel type of green solvents.31–33 DESs also have physicochemical properties similar to tradition of ILs with a series of advantages of low toxicity and good biodegradability.34,35 The process of DESs preparation attains 100% atom utilization under mild conditions by mixing natural materials in the proper ratio without further purification. Some works have investigated the application of different types of DESs in the desulfurization process, and DESs have been used widely in the fields of extraction, catalysis, separation, synthesis and electrochemistry.36 In the published literature, the application of DESs in oxidative desulfurization has attracted wide attention.37,38 Hao et al. synthesized a new series of acidic DESs which were prepared with different molar ratios of caprolactam and oxalic acid and the desulfurization efficiency of such DES reached up to 98%.39 Jiang et al. reported (3-sulfopropyl) triethylammonium chloride/oxalic acid for desulfurization and the ODS efficiency reached 97.7%.40 Nevertheless, the desulfurization conditions of the binary DESs mentioned above are not mild, and the desulfurization efficiencies are generally not high at room temperature. Therefore, it is necessary to explore new DESs, which have the advantages of energy saving and high efficiency at room temperature. Moreover, desulfurization efficiency could be improved at room temperature and the importance of ternary DESs was realized.
In this paper, choline chloride (ClCh)/benzene sulfonate acid (BSA)/ethylene glycol (EG) DES as a class of ternary DESs were used as both extractants and catalysts. The effects of temperature, O/S ratio, and different sulfur-containing compounds were investigated. To have a better understanding of the mechanism about the process of ODS, DESs were characterized by FT-IR and 1H-NMR. In addition, recyclability was also studied to test the system's stability. Furthermore, by introducing free radical scavengers, the active free radicals in the oxidative desulfurization reaction process were explored. Moreover, different molar ratios among ChCl, BSA and EG were investigated.
2. Experiment
2.1 Materials
Benzene sulfonic acid (BSA, 90%), choline chloride (ChCl, 98%) and ethylene glycol (EG, 98%) were obtained from Macklin (Shanghai, China). Benzonthiophene (BT, 99%), dibenzothiophene (DBT, 99%) and 4,6-dimenthyldibenzothiophene (4,6-DMDBT, 97%) were purchased from Sigma-Aldrich (Shanghai, China). Hydrogen peroxide (AR, 30%), AgNO3 (AR, 99.8%), benzoquinone (BQ, 97%), ethylenediaminetetraacetic acid (EDTA, 99.5%) and dimethyl sulfoxide (DMSO, 99%) were bought from Shanghai Sinopharm Chemical Reagent Co. Ltd. All the materials were utilized as provided without further purification.
2.2 Preparation of DESs
DES was prepared by mixing the hydrogen bond acceptor (HBA) and hydrogen bond donor (HBD) under appropriate conditions. Choline chloride (ChCl) was chosen as the HBA. BSA and EG were utilized as the HBDs. Typically, choline chloride was mixed with benzene sulfonic acid and ethylene glycol in a round-bottomed flask with a molar ChCl/BSA/EG ratio of 1
:
2
:
1 under vigorous stirring condition at 25 °C for 2 hours. The homogeneous liquid of as-synthesized DES was obtained.41 The DESs with the ChCl/BSA/EG molar ratio of 1
:
2
:
1, 1
:
1
:
1, 1
:
2
:
2, and 2
:
2
:
1 were denoted as ChCl/2BSA/EG, ChCl/BSA/EG, ChCl/2BSA/2EG, and 2ChCl/2BSA/EG, respectively.
2.3 Characterization
The Fourier transform infrared spectroscopy (FT-IR) of samples was analyzed by a NICOLET 5700 spectrometer which was using KBr pellet technique. The samples were also analyzed by 1H-NMR with an Agilent 400MR spectrometer at room temperature in DMSO-d6 and D2O using tetramethylsilane as an internal reference. The sulfur concentration of the upper clear liquid of the mixture was analyzed by SP6890 gas chromatography with column SE54 and flame ionization detection (GC-FID), and the carrier gas of gas chromatography was nitrogen.
2.4 Oxidative desulfurization process
Organic sulfides such as BT, DBT, and 4,6-DMDBT were dissolved in n-octane with a sulfur concentration of 500 μg/g to act as reference oil. In the meanwhile, the analysis of GC-FID used 2000 μg/g of tetradecane as an internal standard substance. Typically, 10 ml of reference oil, 2 ml of DESs and 0.055 ml of H2O2 were mixed in a round-bottom flask under vigorous stirring condition at 25 °C in a water bath for 2 h. During the process of oxidative desulfurization, the oil in the upper phase was sampled every 30 min, and the samples were analyzed by GC-FID. The sulfur removal efficiency was calculated by the following equation:
where C0 and Ct are the initial and final concentration (μg/g) of sulfides, respectively.11,42
2.5 Experimental process of cyclic reaction
After the first reaction of 2 h, the oil in the upper layer was separated and the DES phase that remained was thoroughly washed with deionized water. The white solid precipitated can be removed by filtration and the filter liquor was transferred to a rotating evaporator in order to obtain the regenerated DES. Afterwards, fresh reference oil and hydrogen peroxide were introduced for the next desulfurization process under the same conditions.11,43
3. Results
3.1 Characterization of DES
3.1.1 FT-IR results. The FT-IR spectra of ChCl, BSA and ChCl/2BSA/EG DES are shown in Fig. 1. BSA shows two characteristic absorption peaks of the sulfonic acid group at 1128 cm−1 and 1016 cm−1, which are also observed at the DES. Two peaks of the monosubstitution of aromatic rings at 730 cm−1 and 694 cm−1 are both found in BSA and DES. In addition, it can be observed that the red shift from 3020 cm−1 of ChCl to 2954 cm−1 of ChCl/BSA/EG on account of the stretching vibration of the methyl group. In a word, there are interactions of H-bounding in DESs.41
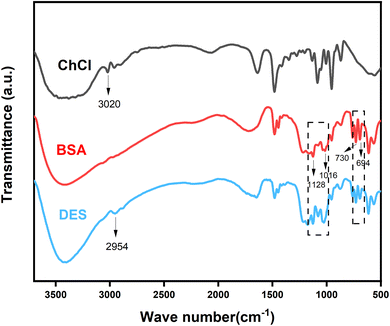 |
| Fig. 1 FT-IR spectra of different samples. | |
3.1.2 1H-NMR results. The 1H-NMR was used and the spectra of the samples are shown in Fig. 2. It was obviously observed that the hydrogen signal of BSA showed at 7.35 ppm and 7.60 ppm while the hydrogen signal of ternary DES and binary DES was upshifted to 7.37 ppm and 7.62 ppm, illustrating that hydrogen bonds between HBAs and HBDs were formed.44 The hydrogen signal of ChCl at 3.07 ppm, 3.38 ppm and 3.93 ppm moved downshift to 2.97 ppm, 3.28 ppm and 3.83 ppm of ChCl/2BSA/EG and ChCl/2BSA, indicating that the hydrogen bonds were formed in DESs as well.41 Compared with BSA, the hydrogen signal of 2BSA/EG at 3.45 ppm may be due to the addition of EG, which can also be clearly observed in the sample of ChCl/2BSA/EG.
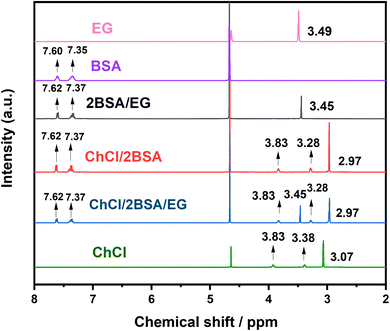 |
| Fig. 2 1H-NMR spectra of different samples. | |
3.1.3 Structure optimization and virtual studying of DES. The density functional theory (DFT) calculation was also performed to better understand the formation of hydrogen bonds. The structure of DES was optimized by using the M06-2X model and the 6-311+G(d, p) basic set level.34 Then, the optimized structure was further analyzed by Multiwfn to draw a sign (λ2)ρ colored δginter isosurface picture with an independent gradient model based on Hirshfeld partition (IGMH) analysis.45,46 As shown in Fig. 3, the bluer the isosurface, the stronger the interaction. It can be observed that the hydroxyl group on two BSA strongly interacts with the chlorine anion of ChCl with a distance of 1.94 Å and 1.96 Å, respectively. In addition, the oxygen atoms in the –SO3H group interact with the hydrogen atoms in the EG and choline cation. Those intermolecular interactions lead to the eutectic effect and forming the DES, which is consistent with the results of FT-IR and 1H-NMR.
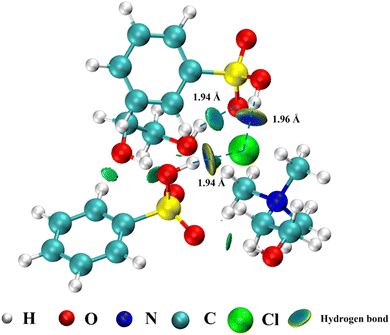 |
| Fig. 3 Optimized structure and visualization of hydrogen bonds. | |
3.2 Catalytic performances
3.2.1 Oxidative desulfurization by DESs with different compositions. The oxidative desulfurization by DESs with different molar rations were carried out. As shown in Table 1, in the absence of hydrogen peroxide, the EDS efficiency of ChCl/2BSA/EG (entry 6) was as much as a double of ChCl/2BSA (entry 4) when EG was introduced into the reaction system. The reason could be the good extractive capacity of EG and the interactions of hydrogen bonds.41 Compared with 2BSA:EG (entry 5), the desulfurization efficiency of ChCl/2BSA/EG (entry 6) reached 100%, indicating the good promotion of ChCl. Compared with ChCl/2BSA/EG (entry 6), none of ChCl/BSA/EG (entry 7), ChCl/2BSA/2EG (entry 8) and 2ChCl/2BSA/EG (entry 9) reached 100%. In particular, entry 7 could not reach 100% desulfurization efficiency because of the small amount of BSA, and entry 8 and 9 were due to the small relative amount of BSA, indicating that BSA played an indispensable role in the desulfurization process. In general, EG plays a critical role in the process of extraction desulfurization, effectively improving the extraction desulfurization efficiency, and may be related to hydrogen bond formation. As the extraction efficiency and oxidation desulfurization efficiency were considered comprehensively, ternary ChCl/2BSA/EG DES was chosen to further study the ODS performances under different reaction conditions.
Table 1 Oxidative desulfurization by DESs with different compositions
Entry |
Catalyst |
Sulfur removal efficiency (%) |
EDSa |
ODSb |
Reaction conditions: EDS: extractive desulfurization, VDES = 2 ml, VOil = 10 ml, T = 25 °C, t = 20 min, without the addition of H2O2. VDES = 2 ml, VOil = 10 ml, T = 25 °C, t = 2 h, O/S = 5. VDES = 2 ml, VOil = 10 ml, T = 25 °C, t = 2 h, without the addition of H2O2. |
1 |
ChCl |
|
13.34 |
2 |
BSA |
|
13.36 |
3 |
EG |
|
2.56 |
4 |
ChCl/2BSA |
7.76 |
98.39 |
5 |
2BSA/EG |
10.40 |
76.53 |
6 |
ChCl/2BSA/EG |
15.73 |
100 |
7 |
ChCl/BSA/EG |
6.30 |
98.01 |
8 |
ChCl/2BSA/2EG |
13.73 |
98.76 |
9 |
2ChCl/2BSA/EG |
8.01 |
94.18 |
10 |
ChCl/2BSA/EG |
9.96c |
|
3.2.2 ODS performances at different temperatures. The effect of different temperatures on desulfurization efficiency was researched in Fig. 4. Obviously, the oxidative desulfurization efficiency was improved with the increase of reaction temperature from 25 °C to 60 °C from 20 min to 30 min. It can be observed that 100% of DBT removal was achieved at 25 °C in 100 min, and 100% DBT removal can be effortlessly achieved within 120 min at 30–60 °C. According to the reaction data from 25 °C to 60 °C, there is no doubt that this system has highly catalytic activity for oxidative desulfurization. The above results indicate that higher temperature can promote the oxidation of DBT.47 Therefore, from the view of both efficiency and economy, 25 °C is chosen as the experimental temperature in the follow-up experiment.
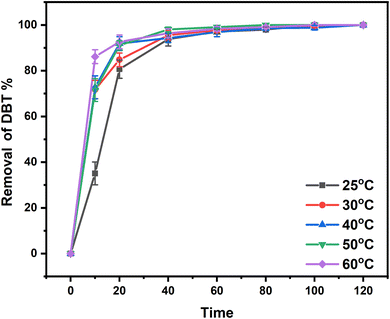 |
| Fig. 4 Sulfur removal at different reaction temperature for the ODS of DBT. Reaction conditions: reference oil: 10 ml (S: 500 μg/g), O/S = 5, DES (2 ml). | |
3.2.3 ODS performances with different O/S ratios. The amount of oxidant plays a key role in the desulfurization process. A series of experiments about the dosage of oxidant were carried out to get a better understanding of the significance of the amount of oxidant and the results are displayed in Fig. 5. On the basis of the theory of the ODS reaction, 2 mol of H2O2 were required to oxidize 1 mol of DBT to its corresponding sulfone.41 As shown in Fig. 5, the reason why the desulfurization efficiencies of O/S = 4 and O/S = 5 were similar is due to the hydrogen peroxide content that is relatively low, so both desulfurization efficiencies did not reach 100%. The desulfurization efficiency reached 96.33% and 97.07% with O/S = 3 and 4 in 2 h, respectively. The explanation could be that hydrogen peroxide was lacking as an oxidant in the desulfurization process. When the O/S molar ratio increases to 5, 6 and 7, 100% desulfurization efficiency was obviously achieved within 100 min. As is expected, sulfur removal efficiency increases with an increase in the O/S ratio. However, with further improvement of the O/S ratio to 7, the efficiency was no longer higher than that with O/S = 6 in reaction time from 40 min to 2 h. The reason could be that the excess water had a negative effect on the desulfurization process as the amount of hydrogen peroxide increased.13 In consideration of efficiency and economy, the ratio of O/S = 5 is chosen as the optimum oxidant dosage.
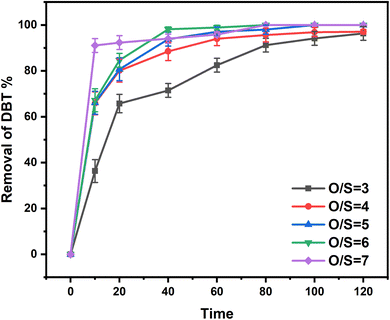 |
| Fig. 5 Sulfur removal with different O/S ratios for the ODS of DBT. Reaction conditions: reference oil: 10 ml (S: 500 μg/g), temperature = 25 °C, DES (2 ml). | |
3.2.4 ODS performances of different sulfur-containing compounds. To further illustrate the catalytic activity of DES on other aromatic sulfur-containing compounds, the removal of BT and 4,6-DMDBT should be tested.41 As shown in Fig. 6, the desulfurization efficiencies for BT, DBT and 4,6-DMDBT could all reached 100%, which is superior than part of the reported literature (Table S1 in ESI†). The removal activity decreases in the sequence of BT > DBT> 4,6-DMDBT from 20 min to 120 min. And because the molecular size of BT, DBT, 4,6-DMDBT gradually increases: BT < DBT < 4,6-DMDBT, the molecular size of BT is the smallest, and the electron density of BT is the lowest, so the spatial steric resistance is the smallest.48 The desulfurization efficiency of BT first reached 100% due to the effect of a small steric hindrance.41 It is worth noting that 4,6-DMDBT shows the lowest efficiency of sulfur removal. It is probably due to the limited mass transfer property and the big steric hindrance effect of 4,6-DMDBT.11
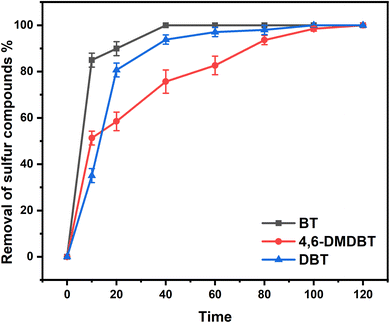 |
| Fig. 6 ODS performances of different sulfur-containing compounds. Reaction conditions: reference oil: 10 ml (S: 500 μg/g), O/S = 5, DES (2 ml), temperature = 25 °C. | |
3.2.5 Recycle performance. The recycling performance of DES plays a crucial role in evaluating its stability. The experiments were carried out and the studies of recycle performance are as shown in Fig. 7. It is worth mentioning that 91.11% of desulfurization efficiency was achieved after the sixth recycle, which indicates the excellent recycle performance and stability of this ternary ChCl/2BSA/EG DES.41
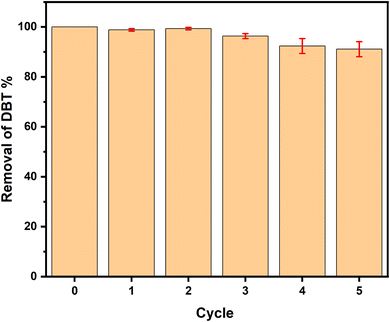 |
| Fig. 7 Reusability of catalytic system in the removal of DBT. Reaction conditions: reference oil 10 ml (S: 500 μg/g), O/S = 5, DES (2 ml), temperature = 25 °C, time = 2 h. | |
3.2.6 The active radical of ODS process. The ODS performance of different radical scavengers was also a significant factor. As is known to us, AgNO3, benzoquinone (BQ), ethylenediaminetetraacetic acid (EDTA) and dimethyl sulfoxide (DMSO) are efficient radical scavengers of e−, ·O2−, H+, and ·OH, respectively. These four scavengers with the concentration of 1500 μg/g were introduced under the same condition, respectively. And the results of DBT removal efficiencies are displayed in Fig. 8. The desulfurization efficiency reached 98.54% and 98.75%, respectively when AgNO3 and EDTA were added into the reaction for 2 h. There were obvious changes in the sulfur removal after adding BQ and DMSO, respectively, while 16.07% and 16.53% of the DBT removal was reached, respectively. It was indicated that ·O2− and ·OH might play an important role in the DES desulfurization system.18
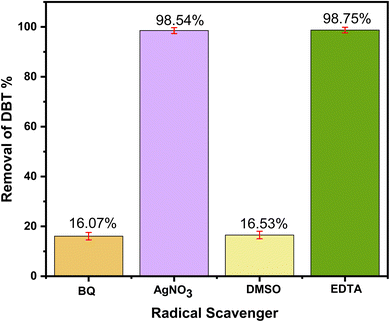 |
| Fig. 8 ODS activities with different radical scavengers. Reaction conditions: reference oil: 10 ml (S: 500 μg/g), O/S = 5, temperature = 25 °C, DES (2 ml), time = 2 h. | |
3.3 The possible mechanism of the ODS process for ChCl/2BSA/EG
Based on the results of experiments, 1H-NMR, FT-IR and some earlier works in our group, a possible ODS mechanism of ternary ChCl/2BSA/EG DESs could be proposed as follows: Firstly, the –SO3H group in the DES was oxidized to peroxysulfonate by H2O2. Then, the DBT was oxidized into dibenzothiophene sulfone by two free radicals of ·O2− and ·OH which were produced by peroxysulfonate.49 At the same time, peroxysulfonate was reduced and both free radicals were turned into water. And the reduced sulfonic acid was oxidized by H2O2 to initiate a new active catalytic cycle.50,51 During the whole process, other components like ChCl and EG in the DES played a vital role in the extraction process, just like extractants, which should not be underestimated as Scheme 1.
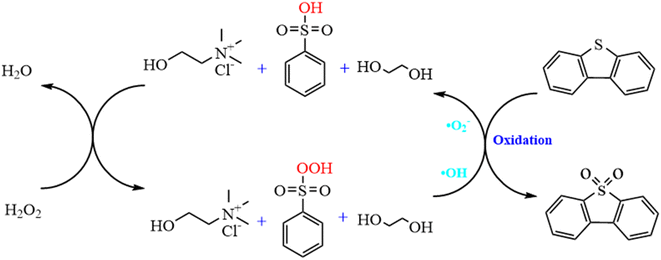 |
| Scheme 1 Proposed catalytic mechanism for the ternary ChCl/2BSA/EG oxidative desulfurization process. | |
4. Conclusion
A novel ternary EG-based ChCl/2BSA/EG DESs was developed for the desulfurization of diesel. The different components of DESs were characterized by 1H-NMR and FT-IR technologies and the structure of DES was optimized by DFT. The effects of DES components on desulfurization efficiencies at different molar ratios were investigated. So that the existence of hydrogen bonds were determined. For the optimal ChCl/2BSA/EG DES, the effects of different temperatures and different O/S on the desulfurization efficiency were studied. And the desulfurization efficiency of 100% was achieved at room temperature with an O/S of 5. After 6 cycles, the desulfurization efficiency was still as high as 91.11%. The good activity of this DES at room temperature is due to the excellent extraction of EG. And the main active species are ·O2− and ·OH in the process of reaction. The ternary DES system, which can achieve 100% of desulfurization efficiency at room temperature, has the advantages of energy saving and high efficiency.
Conflicts of interest
The authors declare no conflicts of interest.
Acknowledgements
The authors acknowledge the financial supports from the National NSFC (No. 22272124 and 21503152), the Open Project of Engineering Research Center of Phosphorus Resources Development and Utilization of Ministry of Education (No. LKF2021010), the Opening Project of the Key Laboratory of Green Chemical Process (Wuhan Institute of Technology) Ministry of Education (GCP20200210; GCX2021122), and the Graduate Innovative Fund of Wuhan Institute of Technology (CX2021017).
References
- S. Guan, Z. Li, B. Xu, J. Wu, N. Wang, J. Zhang, J. Han, T. Guan, J. Wang and K. Li, Chem. Eng. J., 2022, 441, 136022 CrossRef CAS.
- D. V. Wagle, H. Zhao, C. A. Deakyne and G. A. Baker, ACS Sustainable Chem. Eng., 2018, 6, 7525–7531 CrossRef CAS.
- M. M. H. Mondol, B. N. Bhadra and S. H. Jhung, Appl. Catal., B, 2021, 288, 119988 CrossRef CAS.
- I. Shafiq, S. Shafique, P. Akhter, M. Ishaq, W. Yang and M. Hussain, J. Cleaner Prod., 2021, 294, 125731 CrossRef CAS.
- W. Liu, T. Li, G. Yu, J. Wang, Z. Zhou and Z. Ren, Fuel, 2020, 265, 116967 CrossRef CAS.
- L. Lu, B. Zhang, H. Li, Y. Chao, Y. Li, L. Chen, H. Li, H. Ji, P. Wu and W. Zhu, Mol. Catal., 2021, 512, 111757 CrossRef CAS.
- H. Lu, S. Wang, C. Deng, W. Ren and B. Guo, J. Hazard. Mater., 2014, 279, 220–225 CrossRef CAS PubMed.
- H. Lu, P. Li, C. Deng, W. Ren, S. Wang, P. Liu and H. Zhang, Chem. Commun., 2015, 51, 10703–10706 RSC.
- W. Zhu, C. Wang, H. Li, P. Wu, S. Xun, W. Jiang, Z. Chen, Z. Zhao and H. Li, Green Chem., 2015, 17, 2464–2472 RSC.
- J. Yin, J. Wang, Z. Li, D. Li, G. Yang, Y. Cui, A. Wang and C. Li, Green Chem., 2015, 17, 4552–4559 RSC.
- Z. Zhu, H. Lin, M. Chi, X. Gao, Y. Feng, K. Yang and H. Lü, Fuel, 2022, 308, 122070 CrossRef CAS.
- B. N. Bhadra and S. H. Jhung, Chem. Eng. J., 2021, 419, 129485 CrossRef CAS.
- L. Hao, L. Sun, T. Su, D. Hao, W. Liao, C. Deng, W. Ren, Y. Zhang and H. Lü, Chem. Eng. J., 2019, 358, 419–426 CrossRef CAS.
- L. Xu, Y. Luo, H. Liu, J. Yin, H. Li, W. Jiang, W. Zhu, H. Li and H. Ji, J. Mol. Liq., 2021, 338, 116620 CrossRef CAS.
- Y. Guo, X. Liu and B. Hu, New J. Chem., 2022, 46, 22143–22150 RSC.
- E. A. Eseva, A. V. Akopyan, A. V. Anisimov and A. L. Maksimov, Pet. Chem., 2020, 60, 979–990 CrossRef CAS.
- Y. Wei, M. Zhang, P. Wu, J. Luo, D. Tao, C. Peng, L. Dai, L. Wang, H. Li and W. Zhu, Appl. Surf. Sci., 2020, 529, 146980 CrossRef CAS.
- G. Ye, Y. Gu, W. Zhou, W. Xu and Y. Sun, ACS Catal., 2020, 10, 2384–2394 CrossRef CAS.
- L. Hao, S. A. Stoian, L. R. Weddle and Q. Zhang, Green Chem., 2020, 22, 6351–6356 RSC.
- Y. Du, L. Zhou, Z. Liu, Z. Guo, X. Wang and J. Lei, Chem. Eng. J., 2020, 387, 124056 CrossRef CAS.
- M. Jafarinasab and A. Akbari, J. Environ. Chem. Eng., 2021, 9, 106472 CrossRef CAS.
- J. Li, C. Li, L. Yang, Z. Liu, R. Gao and J. Zhao, J. Ind. Eng. Chem., 2022, 109, 338–346 CrossRef CAS.
- Y. Nie, Y. Dong, L. Bai, H. Dong and X. Zhang, Fuel, 2013, 103, 997–1002 CrossRef CAS.
- F. T. Li, B. Wu, R. H. Liu, X. J. Wang, L. J. Chen and D. S. Zhao, Chem. Eng. J., 2015, 274, 192–199 CrossRef CAS.
- R. Abro, N. Kiran, S. Ahmed, A. Muhammad, A. S. Jatoi, S. A. Mazari, U. Salma and N. V. Plechkova, J. Environ. Chem. Eng., 2022, 10, 107369 CrossRef CAS.
- H. A. Elwan, M. T. Zaky, A. S. Farag, F. S. Soliman and M. E. D. Hassan, J. Mol. Liq., 2020, 310, 113146 CrossRef CAS.
- P. Cruz, E.-A. Granados, M. Fajardo, I. del Hierro and Y. Pérez, Appl. Catal., A, 2019, 587, 117241 CrossRef.
- C. Li, D. Li, S. Zou, Z. Li, J. Yin, A. Wang, Y. Cui, Z. Yao and Q. Zhao, Green Chem., 2013, 15, 2793 RSC.
- H. Xu, D. Zhang, F. Wu, X. Wei and J. Zhang, Fuel, 2018, 225, 104–110 CrossRef CAS.
- M. W. Nam, J. Zhao, M. S. Lee, J. H. Jeong and J. Lee, Green Chem., 2015, 17, 1718–1727 RSC.
- S. Tahir, U. Y. Qazi, Z. Naseem, N. Tahir, M. Zahid, R. Javaid and I. Shahid, Fuel, 2021, 305, 121502 CrossRef CAS.
- M. Shaibuna, L. V. Theresa and K. Sreekumar, Soft Matter, 2022, 18, 2695–2721 RSC.
- W. Jiang, L. Dong, W. Liu, T. Guo, H. Li, M. Zhang, W. Zhu and H. Li, RSC Adv., 2017, 7, 55318–55325 RSC.
- L. Sun, Z. Zhu, T. Su, W. Liao, D. Hao, Y. Chen, Y. Zhao, W. Ren, H. Ge and H. Lü, Appl. Catal., B, 2019, 255, 117747 CrossRef CAS.
- C. F. Mao, R. X. Zhao and X. P. Li, Fuel, 2017, 189, 400–407 CrossRef CAS.
- J. Zhang, A. Wang, X. Li and X. Ma, J. Catal., 2011, 279, 269–275 CrossRef CAS.
- W. Jiang, L. Dong, W. Liu, T. Guo, H. Li, S. Yin, W. Zhu and H. Li, Chem. Eng. Process., 2017, 115, 34–38 CrossRef CAS.
- Y. Wei, P. Wu, J. Luo, L. Dai, H. Li, M. Zhang, L. Chen, L. Wang, W. Zhu and H. Li, Microporous Mesoporous Mater., 2020, 293, 109788 CrossRef CAS.
- L. Hao, T. Su, D. Hao, C. Deng, W. Ren and H. Lü, Chin. J. Catal., 2018, 39, 1552–1559 CrossRef CAS.
- W. Jiang, K. Zhu, H. Li, L. Zhu, M. Hua, J. Xiao, C. Wang, Z. Yang, G. Chen, W. Zhu, H. Li and S. Dai, Chem. Eng. J., 2020, 394, 124831 CrossRef CAS.
- W. Jiang, H. Jia, H. Li, L. Zhu, R. Tao, W. Zhu, H. Li and S. Dai, Green Chem., 2019, 21, 3074–3080 RSC.
- M. Zuo, X. Huang, J. Li, Q. Chang, Y. Duan, L. Yan, Z. Xiao, S. Mei, S. Lu and Y. Yao, Catal. Sci. Technol., 2019, 9, 2923–2930 RSC.
- F. Liu, J. Yu, A. B. Qazi, L. Zhang and X. Liu, Environ. Sci. Technol., 2021, 55, 1419–1435 CrossRef CAS PubMed.
- J. Xu, Z. Zhu, T. Su, W. Liao, C. Deng, D. Hao, Y. Zhao, W. Ren and H. Lü, Chin. J. Catal., 2020, 41, 868–876 CrossRef CAS.
- T. Lu and F. Chen, J. Comput. Chem., 2012, 33, 580–592 CrossRef CAS PubMed.
- T. Lu and Q. Chen, J. Comput. Chem., 2022, 43, 539–555 CrossRef CAS PubMed.
- W. Liu, W. Jiang, W. Zhu, W. Zhu, H. Li, T. Guo, W. Zhu and H. Li, J. Mol. Catal. A: Chem., 2016, 424, 261–268 CrossRef CAS.
- B. N. Bhadra and S. H. Jhung, Appl. Catal., B, 2019, 259, 118021 CrossRef CAS.
- L. Hao, M. Wang, W. Shan, C. Deng, W. Ren, Z. Shi and H. Lu, J. Hazard. Mater., 2017, 339, 216–222 CrossRef CAS PubMed.
- M. Chi, T. Su, L. Sun, Z. Zhu, W. Liao, W. Ren, Y. Zhao and H. Lü, Appl. Catal., B, 2020, 275, 119134 CrossRef CAS.
- D. Hao, L. Hao, C. Deng, W. Ren, C. Guo and H. Lü, Chem. Eng. Technol., 2019, 42, 1276–1282 CrossRef CAS.
|
This journal is © The Royal Society of Chemistry 2023 |
Click here to see how this site uses Cookies. View our privacy policy here.