DOI:
10.1039/D3RA02791K
(Paper)
RSC Adv., 2023,
13, 19091-19095
Highly selective fluorescent probe for detecting mercury ions in water†
Received
27th April 2023
, Accepted 7th June 2023
First published on 23rd June 2023
Abstract
Mercury ion (Hg2+) is a well-known toxic heavy metal. It has become one of the most significant environmental pollutants in the world because of its serious physiological toxicity, persistence, easy migration, and high bioconcentration. Thus, the development of methods for monitoring Hg2+ is indispensable. Herein, we have designed and synthesized a new fluorescent probe, TPH, for the detection of Hg2+ in the water environment. The TPH probe could quantitatively detect Hg2+ between 0 and 5 μM (LOD = 16 nM), with a linear range of 0–2.5 μM. In addition, the TPH probe was used to monitor Hg2+ in water samples successfully. Thus, this probe is suitable for monitoring Hg2+ in the actual water environment.
1 Introduction
Mercury has attracted attention from researchers owing to its strong toxicity and bioaccumulation.1–4 With the development of industry, especially gold mining, the burning of fossil and oil refining, mercury ion pollution is widely distributed in the environment.5–7 Through biological enrichment, mercury ions in water can be transformed into organic mercury ions with stronger toxicity and then enter the human body via the food chain.8–10 Even very small amounts of mercury ions have a severe impact on the human body, including the digestive system and kidneys, cognitive disorders, and even central nervous system damage.11–16 Consequently, the development of methods for the detection of mercury ions with simple synthesis and high selectivity and sensitivity is significant.
Recently, many methods have been reported for the detection of mercury ions (Hg2+), including gas chromatography, inductive coupled plasma mass spectroscopy, and atomic absorption spectrometry.17–21 However, most of the above-mentioned methods have some disadvantages, such as complex pretreatment, use of expensive instruments, and difficulty in realizing real-time and on-site monitoring.22,23 In contrast, the use of fluorescent probes has attracted significant attention due to their simple operation and high selectivity and sensitivity.24–30 Thus, an increasing number of fluorescent probes has been used to detect environmental heavy metal pollutants including Hg2+. However, the reported probes for monitoring Hg2+ still have some shortcomings, such as poor selectivity and water solubility and high detection limits (LODs).31–35 Therefore, new fluorescent probes need to be developed for monitoring Hg2+ in the environment with excellent selectivity and sensitivity and good water solubility.
Accordingly, herein, we synthesized the TPH probe, which was based on the TPC-OH dye as the fluorophore36 and phenyl thiochloroformate as the recognition receptor of Hg2+.37,38 Phenyl thiochloroformate possesses high selectivity for the detection of Hg2+, and thus the TPH probe could also achieve the specific and sensitive detection of Hg2+. The TPH probe exhibited the following excellent properties: (1) good water solubility, (2) excellent sensitivity (LOD = 16 nM), (3) high selectivity, and (4) excellent application in the environment. Thus, this probe will have a wide application prospect for monitoring Hg2+ in the environment.
2 Experimental
2.1 Materials and instruments
All chemical reagents were obtained from commercial sources and used without further purification. Absorption and fluorescence spectra were recorded on a UV-3101PC spectrophotometer and Horiba FluoroMax-4 spectrophotometer, respectively.
2.2 Synthesis of TPH probe
TPC-OH dye (236 mg, 1 mmol) was dissolved in dry CH2Cl2 (15 mL), and then phenyl thiochloroformate (259 mg, 1.5 mmol) and N,N-diisopropylethylamine (DIPEA) (194 mg, 1.5 mmol) were added (Scheme 1). The mixed solution was stirred at 25 °C for 2 h.
 |
| Scheme 1 Synthesis of TPH probe. | |
The crude product was purified by column silica chromatography over silica gel using dichloromethane/petroleum ether (14
:
5) as the eluent to provide a faint-yellow pure solid product. 1H NMR (400 MHz, DMSO) δ (ppm): 7.94 (d, J = 5.6 Hz, 1H), 7.81 (d, J = 8.0 Hz, 2H), 7.68 (s, 1H), 7.58 (s, 1H), 7.55–5.52 (m, 4H), 7.48 (t, J = 8.0 Hz, 2H), 7.39 (t, J = 8.0 Hz, 3H), 4.21 (s, 2H). 13C NMR (100 MHz, DMSO) δ (ppm): 193.95, 192.55, 158.01, 153.62, 152.68, 136.23, 135.34, 135.21, 133.66, 131.32, 130.46, 130.42, 129.52, 127.57, 125.82, 122.74, 122.20, 120.54, 32.44.
3 Results and discussion
3.1 Spectral response of TPH probe
All the reactions were carried out in aqueous solution (HEPES 5 mM, pH = 7.4). The fluorescence spectra of the TPH probe for monitoring Hg2+ was investigated. When Hg2+ (20 μM) was added, the fluorescence intensity displayed a significant enhancement at 505 nm (Fig. 1a). The quantum yield of the TPH probe was calculated to be 0.07. Then, its absorption spectrum was also studied. According to the results, the absorption peak changed from 325 nm to 350 nm (Fig. 1b), implying that Hg2+ could promote the splitting of the carbonothioate moiety (Scheme 2). Furthermore, we conducted HRMS and NMR to explore the reaction mechanism of the TPH probe and Hg2+ (Fig. S1–S4 in the ESI†).
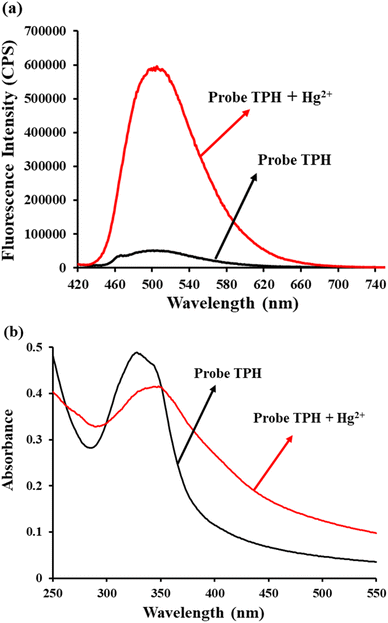 |
| Fig. 1 (a) Fluorescence and (b) absorption spectrum changes of TPH probe (5 μM and 20 μM, respectively) before and after the addition of Hg2+ (20 μM). λex = 400 nm and λem = 505 nm. Conditions: HEPES (5 mM, pH 7.4). | |
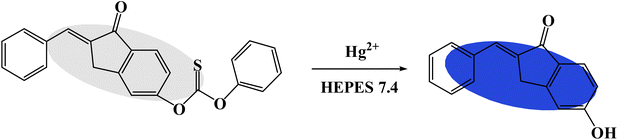 |
| Scheme 2 Recognition mechanism of TPH probe for Hg2+. | |
3.2 Quantification of Hg2+
The TPH probe showed good water solubility, and thus the influence of the concentration of Hg2+ on its fluorescence intensity in pure water was investigated. With an increase in Hg2+ concentration (0–5 μM), the fluorescence intensity of the TPH probe at 505 nm increased accordingly (Fig. 2a). In addition, when the concentration of Hg2+ was 0–2.5 μM, it was linearly correlated with the fluorescence intensity (y = 201
921 [Hg2+] (μM) + 85
603, R2 = 0.9828) (Fig. 2b), and the LOD was 16 nM (3σ/k). Thus, all the above-mentioned results indicate that the TPH probe can provide a sensitive detection tool for Hg2+ in the actual water environment.
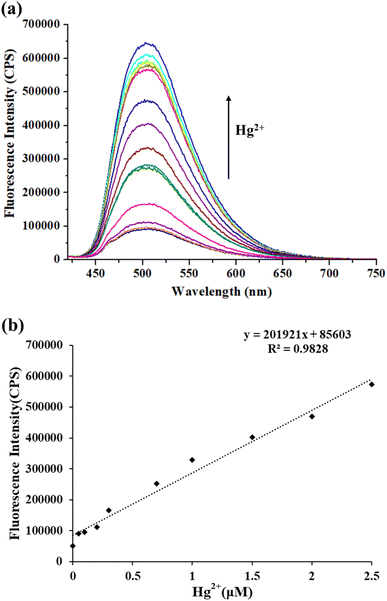 |
| Fig. 2 (a) Fluorescence spectra of TPH probe (5 μM) for Hg2+ (0–5 μM). (b) Linear plot of fluorescence intensity (505 nm) to Hg2+ (0–2.5 μM). λex = 400 nm and λem = 505 nm. Conditions: in HEPES (5 mM, pH 7.4). | |
3.3 Specificity for Hg2+
The specificity of the TPH probe toward Hg2+ and other various relevant analytes including Cd2+, Cu2+, Fe2+, Ca2+, K+, Mg2+, Na+, Ni2+, Pb2+, Sn2+, Zn2+, Co2+, Fe3+, NO3−, SO42− and Cl− was analyzed. The concentration of Hg2+ and the other analytes was 5 μM and 50 μM. Only Hg2+ caused a fluorescence response at 505 nm, while the other relevant analytes did not cause obvious fluorescence changes (Fig. 3a). Besides, interference experiments were also conducted. The fluorescence intensity response values exhibited a slight change at 505 nm (Fig. 3b). Thus, all these results strongly suggest that the TPH probe can specifically recognize Hg2+.
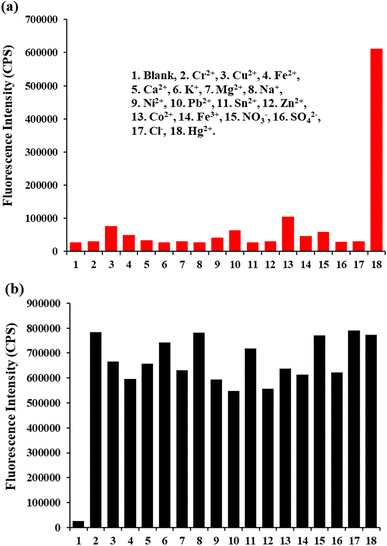 |
| Fig. 3 (a) Fluorescence response of TPH probe (5 μM) toward Hg2+ (5 μM) and other ions (50 μM). (b) Fluorescence response of TPH probe (5 μM) toward Hg2+ (5 μM) in the presence of other ions (50 μM). λex = 400 nm and λem = 505 nm. Conditions: in HEPES (5 mM, pH 7.4). | |
3.4 Analytical applications in real water samples
Then, the analytical application of the TPH probe in three water samples (lake water, underground water and river water) for the detection of Hg2+ was investigated. Firstly, no Hg2+ was found in the samples. Then, after 5 μM TPH probe was added to the test water samples, 1 and 2 μM Hg2+ were also respectively added. Each sample was tested three times. As can be seen in Table 1, the recoveries of the three water samples were 84.07–109.20%, further confirming that this newly synthesized probe could effectively detect Hg2+ in the real water environment.
Table 1 Application of TPH probe in three water samplesa
Real water samples |
Found Hg2+ |
Addition Hg2+ (μM) |
Found (μM) |
Recovery (%) |
RSD (n = 3) (%) |
ND: not detected. Sample A from JiaZi Lake, University of Jinan and samples B and C from Jinyun River and Jinyang River in Jinan, China. |
Sample A |
ND |
1 |
0.97 ± 0.03 |
96.93 |
3.08 |
2 |
2.01 ± 0.13 |
100.38 |
6.40 |
Sample B |
ND |
1 |
1.09 ± 0.06 |
109.20 |
6.42 |
2 |
1.68 ± 0.13 |
84.07 |
6.64 |
Sample C |
ND |
1 |
0.96 ± 0.08 |
95.97 |
8.41 |
2 |
1.72 ± 0.11 |
85.81 |
5.40 |
4 Conclusions
The fluorescent TPH probe with phenyl thiochloroformate as the Hg2+ recognition site was synthesized in this study. This probe could specifically recognize Hg2+ and quantitatively detect Hg2+ in aqueous solution. According to the experimental results, we calculated that its detection limit is 16 nM. Meanwhile, the TPH probe has excellent water solubility, which is conducive for its application in the actual environment.
Data availability
The datasets generated and analyzed during the current study are available from the corresponding author on reasonable request.
Conflicts of interest
The authors have no conflicts of interest to declare.
Acknowledgements
We gratefully acknowledge financial support from the NSFC (42007176, 52070122), and Natural Science Foundation of Shandong Province (ZR2020QD125, ZR2020ME222), Key Scientific and Technological Innovation Foundation of Shandong Province (No. 2020CXGC011404).
References
- S. Y. Chen, Z. Li, K. Li and X. Q. Yu, Coord. Chem. Rev., 2021, 429, 213691 CrossRef CAS.
- M. A. Peckham, M. S. Gustin and P. J. Weisberg, Environ. Sci. Technol., 2019, 53, 3663 CrossRef CAS PubMed.
- L. Wang, Y. Zhao and J. Zhang, Ind. Eng. Chem. Res., 2017, 56, 3804 CrossRef CAS.
- S. X. Wang, L. Zhang, L. Wang, Q. R. Wu, F. Y. Wang and J. M. Hao, Front. Environ. Sci. Eng., 2014, 8, 631 CrossRef CAS.
- Y. M. Yu, C. Y. Liu, B. Tian, X. Y. Cai, H. C. Zhu, P. Jia, Z. L. Li, X. Zhang, W. L. Sheng and B. C. Zhu, Dyes Pigm., 2020, 177, 108290 CrossRef CAS.
- X. Yuan, T. H. Leng, Z. Q. Guo, C. Y. Wang, J. Z. Li, W. W. Yang and W. H. Zhu, Dyes Pigm., 2019, 161, 403 CrossRef CAS.
- Q. X. Duan, M. Zhang, C. X. Sheng, C. Y. Liu, L. Wu, Z. M. Ma, Q. Zhao, Z. P. Wang and B. C. Zhu, Anal. Sci., 2017, 33, 1169 CrossRef CAS PubMed.
- F. Song, C. Yang, X. T. Shao, L. Du, J. Zhu and C. Kan, Dyes Pigm., 2019, 165, 444 CrossRef CAS.
- C. Y. Qin, B. Y. Du, R. S. Yin, B. Meng, X. W. Fu, P. Li, L. M. Zhang and X. B. Feng, Environ. Sci. Technol., 2020, 54, 14334 CrossRef CAS PubMed.
- Y. Zhang, C. Y. Liu, M. J. Su, X. D. Rong, X. Wang, K. Wang, X. W. Li, H. C. Zhu, M. H. Yu, W. L. Sheng and B. C. Zhu, J. Photochem. Photobiol., A, 2022, 425, 113706 CrossRef CAS.
- X. Wang and W. X. Wang, Environ. Sci. Technol., 2015, 49, 10173 CrossRef CAS PubMed.
- J. Xu, Z. K. Wang, C. Y. Liu, Z. H. Xu, N. Wang, X. Cong and B. C. Zhu, J. Lumin., 2018, 6, 1122 CrossRef PubMed.
- R. Roy, S. Rakshit, S. Bhar and S. Bhattacharya, RSC Adv., 2015, 5, 67833 RSC.
- M. Jing, D. Lin, P. Wu, M. J. Kainz, K. Bishop, H. Yan, Q. Li and X. Feng, Sci. Total Environ., 2021, 782, 146410 CrossRef CAS PubMed.
- P. Srivastava, S. Razi, R. Ali, R. Gupta, S. Yadav, G. Narayan and A. Misra, Anal. Chem., 2014, 86, 8693 CrossRef CAS PubMed.
- B. Yuan, D. X. Wang, L. N. Zhu, Y. L. Lan, M. Cheng, L. M. Zhang, J. Q. Chu and X. Z. Li, Chem. Sci., 2019, 10, 4220 RSC.
- Z. Mermer, O. Yavuz, S. K. Atasen, Y. Alcay and I. Yilmaz, J. Hazard. Mater., 2021, 410, 124597 CrossRef CAS PubMed.
- A. Thongsaw, R. Sananmuang, Y. Udnan, G. M. Ross and W. C. Chaiyasith, Spectrochim. Acta, Part B, 2019, 160, 105685 CrossRef CAS.
- Q. X. Zhou, Y. L. Liu, Y. L. Wu, Z. Li, Y. H. Li, M. H. Liu, T. X. Qu and C. M. Chen, Chemosphere, 2021, 274, 129959 CrossRef CAS PubMed.
- S. E. Long, J. E. Norris, J. Carney and J. V. Ryan, Pollut. Res., 2020, 11, 909 CrossRef CAS.
- I. Narin, M. Soylak, L. Elci and M. Dogan, Talanta, 2000, 52, 1041 CrossRef CAS PubMed.
- S. Huang, X. Cheng, Q. Lei, B. Feng, X. H. Liu, J. P. Ding, C. Zhong, J. H. Liang and W. B. Zeng, Dyes Pigm., 2021, 187, 109125 CrossRef.
- Y. N. Zhang, S. Guo, Z. R. Jiang, G. B. Mao, X. H. Ji and Z. K. He, Anal. Chem., 2018, 90, 9796 CrossRef CAS PubMed.
- T. H. Chen, S. Zhang, M. Jaishi, R. Adhikari, J. Bi, M. Fang, S. Xia, Y. Zhang, R. L. Luck, R. Pati, H. M. Lee, F. T. Luo, A. Tiwari and H. Liu, ACS Appl. Bio Mater., 2018, 1, 549 CrossRef CAS PubMed.
- S. Y. Ma, L. Y. Li, M. Y. She, Y. Mo, S. Y. Zhang, P. Liu and J. L. Li, Chin. Chem. Lett., 2017, 28, 2014 CrossRef CAS.
- Q. X. Duan, H. C. Zhu, C. Y. Liu, R. F. Yuan, Z. T. Fang, Z. K. Wang, P. Jia, Z. L. Li, W. L. Sheng and B. C. Zhu, Analyst, 2019, 144, 1426 RSC.
- W. Shu, L. G. Yan, J. Liu, Z. K. Wang, S. Zhang, C. C. Tang, C. Y. Liu, B. C. Zhu and B. Du, Ind. Eng. Chem. Res., 2015, 54, 8056 CrossRef CAS.
- Y. Z. Liu, H. Z. Zhao, N. Gao, C. L. Yang, R. B. Zhang and X. L. Zhang, Sens. Actuators, B, 2021, 344, 130201 CrossRef CAS.
- B. Yuan, D. X. Wang, L. N. Zhu, Y. L. Lan, M. Cheng, L. M. Zhang, J. Q. Chu, X. Z. Li and D. M. Kong, Chem. Sci., 2019, 10, 4220 RSC.
- Q. X. Duan, X. Y. Lv, C. Y. Liu, Z. F. Geng, F. F. Zhang, W. L. Sheng, Z. K. Wang, P. Jia, Z. L. Li, H. C. Zhu and B. C. Zhu, Ind. Eng. Chem. Res., 2019, 58, 11 CrossRef CAS.
- M. Zhao, G. K. Shao, Y. S. Guo, Y. L. Tang, J. B. Liu and D. S. Guo, New J. Chem., 2020, 44, 12538 RSC.
- S. Maity, S. Banerjee, K. Sunwoo, J. Kim and P. Bharadwaj, Inorg. Chem., 2015, 54, 3929 CrossRef CAS PubMed.
- H. Un, C. Huang, C. Huang, T. Jia, X. Zhao, C. Wang, L. Xu and H. Yang, Org. Chem. Front., 2014, 1, 1083 RSC.
- T. Zhang, B. Wu, Z. Zou, Y. Wu, J. Zheng, W. Wong and K. Wong, J. Lumin., 2016, 170, 187 CrossRef CAS.
- S. Y. Qin, B. Chen, J. Huang and Y. F. Han, New J. Chem., 2018, 42, 12766 RSC.
- C. Y. Liu, P. Jia, Z. H. Zhuang, Z. K. Wang, Q. X. Duan, Z. R. Li, H. C Zhu, X. Zhang, B. C. Zhu and W. L. Sheng, Sens. Actuators, B, 2019, 291, 243 CrossRef CAS.
- J. Xu, Z. H. Xu, Z. K. Wang, C. Y. Liu, B. C. Zhu, X. R. Wang, K. Wang, J. T. Wang and G. Q. Sang, J. Lumin., 2018, 33, 219 CrossRef CAS PubMed.
- W. Shu, Y. W. Wang, L. Wu, Z. K. Wang, Q. X. Duan, Y. B. Gao, C. Y. Liu, B. C. Zhu and L. G. Yan, Ind. Eng. Chem. Res., 2016, 55, 8713 CrossRef CAS.
|
This journal is © The Royal Society of Chemistry 2023 |
Click here to see how this site uses Cookies. View our privacy policy here.