DOI:
10.1039/D3RA02829A
(Paper)
RSC Adv., 2023,
13, 25959-25967
Ultrasound-assisted-one-pot synthesis and antiplasmodium evaluation of 3-substituted-isoindolin-1-ones†
Received
29th April 2023
, Accepted 21st August 2023
First published on 1st September 2023
Abstract
As the attempts to control malaria through chemotherapy strategies are restricted, we have prepared a small library of 3-substituted-isoindolinones from (Z)-3-benzylideneisobenzofuran-1(3H)-ones in one-pot fashion under ultrasound irradiation. The one-pot reaction was scalable and efficiently produced the desired products (1a–m) in high yields in a short reaction time. Evaluation of their in vitro antiplasmodium assay against the 3D7 (chloroquine-sensitive) and FCR3 (chloroquine-resistant) strains of Plasmodium falciparum demonstrated that they displayed moderate to strong antiplasmodium activities (the IC50 values ranging from 4.21–34.80 μM) and low resistance indices. The in silico prediction of ADME and physicochemical properties showed that the synthesized compounds met the drug-likeliness requirements and featured low toxicity effects. Based on the evaluation of the antiplasmodium profiles, 3-substituted-isoindolinone derivatives of 1a, 1d, 1h, and 1l may become potential antiplasmodium candidates.
Introduction
Malaria is one of severe infectious diseases which threaten the global population both in tropical and sub-tropical countries. This disease is transmitted by the female Anopheles mosquito where the protozoan parasite of Plasmodium falciparum is the most predominant species which is responsible for the mortality.1 The efforts to control malaria through chemotherapy are restricted since Plasmodium falciparum has developed resistance to artemisinin combination therapies (ACT) and other antimalaria drugs such as chloroquine, quinine, mefloquine and halofantrine.2,3 Therefore, the development of more effective antimalaria agents with good resistance profile is urgently required.
Isoindolin-1-ones, particularly 3-substituted-isoindolin-1-ones 1, are one of the most privileged scaffolds found in natural products,4 such as entonalactam A5 and (−)-goniolanceolactam.6 The synthetic γ-lactams have been reported to exhibit various pharmaceutical applications including N-p-methoxy-1,α-dihydroaristoyagonine7 as anticancer and N-benzylisoindolin-1-one8 as antibacterial. Moreover, isoindolin-1-one derivatives as exemplified by pazinaclone and pagoclone are commercial anxiolytic drugs.9 Due to their interesting applications in medicinal chemistry, a considerable attention has been devoted to produce 3-substituted-isoindolin-1-ones through simple and facile synthetic strategies (Fig. 1).
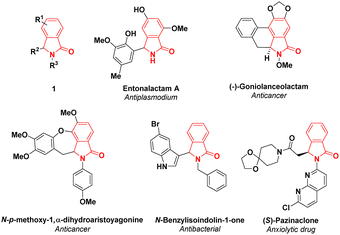 |
| Fig. 1 3-Substituted isoindolin-1-ones and their pharmaceutical applications. | |
Isoindolin-1-ones 1 have been synthesized through intramolecular hydroamidation of ortho-vinylbenzamides.10 The construction of isoindolinone moiety can be carried out through the treatment of isobenzofuran-1(3H)-ones with primary amines.11,12 Other groups have carried out the ortho-alkenylation of substituted benzamides to generate ortho-vinybenzamides in the presence of transition metal catalysts such as Co,13,14 Ru15 and Rh.16 Then, the in situ generated synthetic intermediates may undergo subsequent intramolecular amidation to furnish lactam 1. Another application of transition metal catalyst in the synthesis of isoindolin-1-ones is Rh-catalyzed-three component reaction using benzamides, ketones and hydrazines.17 The formation of isoindolin-1-ones 1 has been achieved through the addition of Grignard reagent to 2-(N-sulfinylimines)benzoate esters followed by the intramolecular lactamization.18–20 Other strategies involved the modification of other isoindolin-1-one moieties such as alkylation of isoindolin-1-ones at C3 position,21,22 hydrogenation of 3-alkylideneisoindolin-1-ones,23,24 or reduction of 3-hydroxyisoindolin-1-ones.25,26 In spite of these robust methodologies, a facile, rapid and straightforward approach to efficiently generate biologically active isoindolin-1-ones 1 should be still developed.
Our recent study reported an efficient synthesis of 3-hydroxyisoindolin-1-ones 7 through nucleophilic addition reaction of primary amines 3 into (Z)-3-benzylideneisobenzofuran-1(3H)-ones 2. We demonstrated that the reactivity of 3-hydroxyisoindolin-1-ones 7 can be further extended to give various motifs of isoindolin-1-ones. On reaction of 3-benzyl-3-hydroxyisoindolin-1-one with hydride source (NaBH3CN) under acidic condition, 3-benzylisoindolin-1-one was generated through the formation of N-acyliminium intermediate (NAI). We also found that ultrasound irradiation may accelerate the rate of those reactions.27
Our strategy was driven to meet the green chemistry principles as an efficient approach for 3-isoindolin-1-ones 1. Based on the promising preliminary results, we envisioned that performing both reactions based on ultrasound-assisted-one-pot reaction would efficiently generate 3-isoindolin-1-ones 1. One-pot reaction is well known as an efficient and straightforward strategy in chemical synthesis and has been widely applied in synthesis of drugs.28 Application of ultrasound in chemical process has been well documented for the synthesis of various heterocyclic scaffolds. Performing organic reaction under ultrasound irradiation has been found to be more superior than conventional heating or stirring. This green protocol is featured with higher reaction yields, shorter reaction time, energy efficiency and mild conditions.29–33
We present, herein, one-pot synthesis of 3-substituted-isoindolin-1-ones 1 under ultrasound irradiation, evaluation of their cytotoxicity against Plasmodium falciparum FCR3 (chloroquine-resistant) and 3D7 (chloroquine-sensitive) strains as well as prediction of their physicochemical and pharmacokinetic properties.
Results and discussion
Chemistry
The one-pot synthesis of 3-substituted-isoindolin-1-ones 1 was initially investigated by performing the nucleophilic addition reaction between (Z)-3-benzylideneisobenzofuran-1(3H)-one 2a (prepared from 2-iodobenzoic acid and terminal alkynes via ultrasound-assisted-Sonogashira coupling27) and butylamine 3a in iso-propanol at 50 °C under ultrasound irradiation. This reaction in situ produced the synthetic intermediate of 3-hydroxyisoindolin-1-ones 7. After completion of the reaction, p-TSA as the acid catalyst (10 equiv.) and NaBH3CN (3 equiv.) as the hydride source were introduced to the subsequent NAI reaction in the same flask without isolating the intermediates 7.
The use of high amount of p-TSA was required to completely convert 3-hydroxyisoindolin-1-ones 7 into the corresponding NAI intermediates 8. The reaction was further irradiated at 50 °C for more 1 h (Table 1, entry 1). To our delight, we observed the formation of the desired 3-benzyl-2-butylisoindolin-1-one 1a as a racemic mixture in 63% yield. Attempt to increase the number of hydride source may improve the reaction yields (entries 2–4). The product 1a was furnished in 86% yield when using 10 equiv. of NaBH3CN (entry 3). Further survey of solvents (entries 5–7) and catalysts (entries 8–10) demonstrated that the excellent yield of 1a was obtained when the reaction proceeded in acetonitrile in the presence of trifluoroacetic acid (entry 9). The control experiment demonstrated that the lactam 1a was obtained in lower yields when the one-pot reaction was carried out using conventional heating for 10 h (entries 9 vs. 11). The increase of both reaction yields and rates of the ultrasound-assisted-one-pot reaction was because the reaction medium received additional energy which was supplied by the mechanical effect of cavitation phenomenon generated from ultrasound waves.34–36
Table 1 Optimization of ultrasound-assisted-one-pot synthesis of 3-substituted-isoindolin-1-onesa
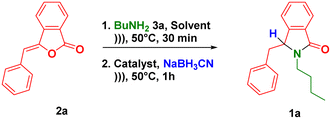
|
Entry |
[NaBH3CN] (equiv.) |
Solvent |
Catalyst |
Yieldb (%) |
The experiments were performed using 0.5 mmol of 2a and 1.0 mmol of 3a under ultrasonic irradiation (40 kHz, 350 W) for 30 min (1st step) + 60 min (2nd step). Isolated yields. The reaction was performed at 50 °C for 5 h + 5 h using conventional heating. |
1 |
3 |
i-PrOH |
p-TSA |
63 |
2 |
5 |
i-PrOH |
p-TSA |
70 |
3 |
10 |
i-PrOH |
p-TSA |
86 |
4 |
15 |
i-PrOH |
p-TSA |
75 |
5 |
10 |
DMSO |
p-TSA |
79 |
6 |
10 |
MeCN |
p-TSA |
90 |
7 |
10 |
THF |
p-TSA |
77 |
8 |
10 |
MeCN |
HCl |
72 |
9 |
10 |
MeCN |
TFA |
93 |
10 |
10 |
MeCN |
BF3·OEt2 |
80 |
11c |
10 |
MeCN |
TFA |
77 |
The scopes of one-pot synthesis of 3-substituted-isoindolin-1-ones 1 have been examined under the optimized reaction conditions (Scheme 1). The reaction with phenethylamine afforded the desired product 1b in 79% yield. It should be noted that we did not observe the side product coming from the intramolecular Friedel–Crafts reaction towards the NAI intermediate 8 as previously reported.37 The ultrasound-assisted-one-pot reaction also worked well (1c) when benzylamine was used as substrate.
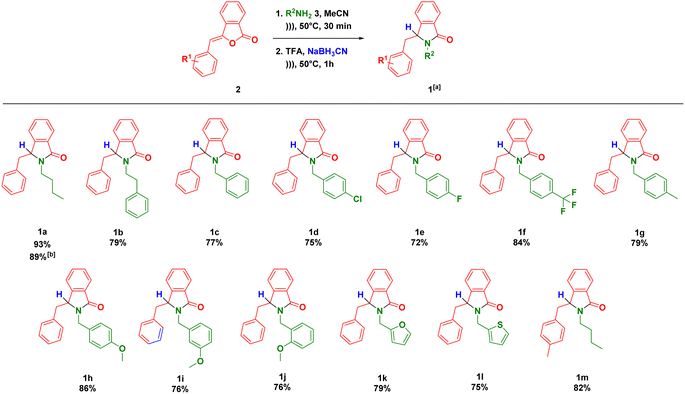 |
| Scheme 1 Scopes of reaction, areaction conditions: 2 (0.5 mmol), 3 (1 mmol, 2 equiv.), MeCN (1 mL), under ultrasonic irradiation (40 kHz, 350 W) at 50 °C for 30 min (1st step) + 60 min (2nd step); bthe reaction was conducted using 10 mmol of 2. | |
The nature of substituent at benzylamine was then evaluated under the optimal reaction conditions. One of strategies to enhance antiplasmodium activity is by incorporating halogen, trifluoromethyl and methoxy groups to the lead compounds.38,39 Therefore, we decided to install both electron withdrawing (Cl, F and CF3) and donating (Me and OMe) groups at para position of benzylamines. These groups were tolerated to the reaction conditions, delivering the products (1d–h) in high yields. Furthermore, benzylamines with methoxy group at the ortho and meta positions smoothly reacted to give the corresponding isoindolin-1-ones 1i–j. Heterocyclic amines such as furfurylamine and 2-thiophenemethylamine could be subjected to our methodology leading to the direct formation of the products 1k–l in good yields. As exemplified by 1m, we also managed to employ the precursor of (Z)-3-(4-methylbenzylidene)isobenzofuran-1(3H)-one in the developed one-pot reaction.
Encouraged by the scopes of the reaction, we then evaluated the scalability of the one-pot reaction. Performing the gram scale-reaction using 10 mmol of (Z)-3-benzylideneisobenzofuran-1(3H)-one 2a allowed us to obtain the product 1a in 89% yield (Scheme 1).
The plausible reaction mechanism is depicted in Scheme 2. Initial nucleophilic addition of primary amines 3 into (Z)-3-benzylideneisobenzofuran-1(3H)-ones 2 results in enols 5, which subsequently transformed into the stable keto form 6. Then, the intramolecular nucleophilic addition of ketoamides 6 produces 3-hydroxyisoindolin-1-ones 7. The in situ generated lactams 7 reacts with acid catalyst of trifluoroacetic acid leading to the formation the reactive N-acyliminium ions (NAI) 8.40–42 Then, two possible reaction mechanism can be considered. The first one is the trapping of the reactive ketimines 8 with hydride source (NaBH3CN) to directly furnish the isoindolin-1-ones 1 (Scheme 2, route A). In the second pathway, the NAI intermediates 8 may undergo subsequent β-elimination and hydrogenation reactions (Scheme 2, route B). To examine the mechanistic pathway, a control experiment was conducted by introducing 3-benzylideneisoindolin-1-one 4a to the second reaction under the standard condition (Scheme 2, eqn (1)). However, there was no lactam 1 generated even after 8 h of ultrasound irradiation, which let us omit the second scenario. Therefore, the ultrasound-assisted-one-pot reaction of isoindolin-1-ones 1 may proceed through nucleophilic addition or trapping of the NAI intermediates 8 with hydride sources.
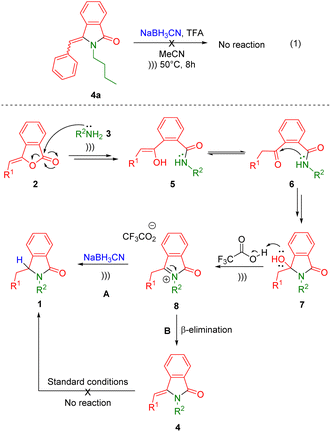 |
| Scheme 2 Proposed reaction mechanism. | |
In vitro antiplasmodium assay
Having prepared a small library of racemic 3-substituted-isoindolin-1-ones, we then turned our attention to the evaluation of their antiplasmodium activities. These products 1a–m were tested in vitro against chloroquine-sensitive (3D7) and -resistant (FCR3) strains of Plasmodium falciparum.43 The antiplasmodium activities were presented as mean of IC50 values.
The results of the antiplasmodium assay are presented in Table 2. We were pleased to observe that the N-butylisoindolin-1-one 1a displayed great activities of 8.18 and 5.98 μM against chloroquine-sensitive (3D7) and -resistant (FCR3) strains, respectively. The antiplasmodium activity was reduced when phenylethyl group was installed in the isoindolin-1-one skeleton 1b. Introducing halogen substituents, such as Cl, F and CF3, on the N-benzyl moiety at 4-position gave positive influence on antiplasmodium activity if compared with hydrogen substituent (1c versus 1d–f). However, a slight reduction of activity against 3D7 strain was detected for 1d derivative. The presence of halogen substituents on the bioactive compounds may improve their pharmacological properties (for example lipophilicity, metabolic stability, and membrane permeation) and may block metabolic pathway such as cytochrome P450 oxidation.44,45 The effect of electron donating groups, such as Me and OMe, was evaluated and enhanced activities against both strains was observed (1c versus 1g–h). Change in the position of methoxy groups to 3- and 2- positions reduced their antiplasmodium activity, signifying the importance of substituent at 4-position (1g versus 1h–i). The position of substituent may direct the orientation of molecules and affect the binding ability and energy towards the target proteins of Plasmodium parasites.46 Interestingly, heterocycle-substituted-isoindolin-1-ones 1k–l exhibited high activities against both strains. However, the decreased activities on 3D7 and FCR3 strains were observed when methyl group was installed at C-benzyl moiety (1a versus 1m).
Table 2 In vitro data and calculated physical properties of isoindolin-1-ones 1
Compound |
IC50 (μM) |
Resistance indices |
Molecular weight (g mol−1) |
Log P |
HBD |
HBA |
3D7 |
FCR3 |
1a |
8.18 |
5.98 |
0.73 |
279.38 |
3.81 |
0 |
1 |
1b |
32.60 |
26.90 |
0.83 |
327.42 |
4.34 |
0 |
1 |
1c |
15.90 |
27.53 |
1.73 |
313.39 |
4.03 |
0 |
1 |
1d |
15.89 |
7.48 |
0.47 |
347.84 |
4.59 |
0 |
1 |
1e |
8.73 |
10.45 |
1.20 |
331.38 |
4.36 |
0 |
2 |
1f |
13.85 |
4.21 |
0.30 |
381.39 |
5.07 |
0 |
4 |
1g |
8.25 |
6.77 |
0.82 |
327.42 |
4.40 |
0 |
1 |
1h |
12.28 |
14.38 |
1.17 |
343.42 |
4.03 |
0 |
2 |
1i |
27.58 |
28.23 |
1.02 |
343.42 |
4.07 |
0 |
2 |
1j |
34.80 |
30.37 |
0.87 |
343.42 |
4.01 |
0 |
2 |
1k |
13.08 |
5.22 |
0.40 |
303.35 |
3.35 |
0 |
2 |
1l |
9.50 |
6.19 |
0.65 |
319.42 |
4.04 |
0 |
1 |
1m |
33.96 |
9.31 |
0.27 |
293.40 |
4.17 |
0 |
1 |
CQ |
0.01 |
0.11 |
11 |
319.87 |
4.15 |
1 |
2 |
The antiplasmodium activity can be classified into 5 categories, including very active (IC50 < 1 μM), active (IC50 1–20 μM), moderate (IC50 20–100 μM), low (IC50 100–200 μM), and not active (IC50 > 200 μM). By considering the IC50 values (Table 2), most of the isoindolin-1-one derivatives can be classified as active antiplasmodium agents against both parasite strains. Some of tested compounds showed moderate activities (for instance 1b, 1i, 1j, 1m against 3D7 strain and 1b, 1c, 1i, 1j against FCR3 strain).
For all tested compounds, the corresponding resistance indice (RI) was also calculated (ratio IC50(FCR3)/IC50(3D7)). The high value of RI correlates to the high level of resistance, while low RI value indicates that the compounds have potential as antiplasmodium agents.47,48 It should be noted that the RI values of all products were relatively low and in the range of 0.27–1.73. Despite the antiplasmodium activity of chloroquine against both strains were higher than the tested compounds, the RI values of the isoindolin-1-ones 1a–m were 6–40 times lower than that of chloroquine.
Physicochemical and pharmacokinetic properties
The physicochemical properties were calculated through http://www.swissadme.ch/ to predict the drug likeliness of the products based on Lipinski “rule of five”.49,50 The calculation of the tested compounds (Table 1) showed that (a) the molecular weight was lower than 500; (b) log
P value was lower than 5; (c) number of hydrogen bond donor (HBD) was lower than 5 and (d) number of hydrogen acceptor was lower than 10. All the tested compounds were compliant to the Lipinski rule, indicating that isoindolin-1-ones 1a–m may be easily absorbed, have good permeability and promising potential as antiplasmodium agents.
The in silico pharmacokinetic properties of synthesized compounds were also predicted using the online web tools (Tables 3 and 4). The screening of pharmacokinetic properties like absorption, distribution, metabolism, and toxicity parameters were evaluated using the PreADMET web (http:/preadmet.bmdrc.org), while the excretion parameters were calculated using the pkCSM web (https://biosig.lab.uq.edu.au/pkcsm/). The absorption of drugs depends on the human intestinal absorption (HIA), CaCo-2 cell permeability and Madin–Darby canine kidney (MDCK) cell permeability. The results showed that all lactams 1a–m were predicted to have more than 70% HIA values, suggesting that they had good human intestinal absorption. For CaCo-2 cell permeability, all isoindolin-1-ones 1a–m exhibited moderate permeability. On the MDCK cell permeability, five derivatives (1a, 1b, 1c, 1i and 1k) were anticipated to show moderate permeability and the other compounds were expected to display low permeability.
Table 3 Predicted absorption and distribution paramaters of isoindolin-1-ones 1
Compound |
Absorption |
Distribution |
HIA (%) |
Caco-2 cell permeability (nm s−1) |
MDCK (nm s−1) |
PPB (%) |
Blood–brain barrier penetration (Cbrain/Cblood) |
1a |
100 |
52.05 |
34.49 |
97.18 |
1.31 |
1b |
100 |
52.23 |
47.21 |
94.75 |
1.89 |
1c |
100 |
51.04 |
32.47 |
94.85 |
2.20 |
1d |
100 |
54.23 |
3.23 |
100 |
1.05 |
1e |
100 |
54.27 |
2.97 |
99.80 |
0.64 |
1f |
100 |
41.86 |
0.04 |
99.08 |
4.65 |
1g |
100 |
29.52 |
23.99 |
85.81 |
10.36 |
1h |
100 |
53.31 |
3.49 |
93.15 |
0.21 |
1i |
100 |
53.31 |
53.31 |
93.10 |
0.21 |
1j |
100 |
53.25 |
13.25 |
92.98 |
0.21 |
1k |
99.24 |
56.11 |
223.93 |
94.82 |
3.18 |
1l |
97.48 |
53.23 |
23.77 |
99.51 |
2.29 |
1m |
100 |
52.15 |
16.49 |
97.21 |
2.21 |
Required parameters |
>20 |
>4 |
>25 |
>90 |
>0.1 |
Table 4 Predicted metabolism and excretion paramaters of isoindolin-1-ones 1
Compound |
Metabolism |
Excretion |
CYP2C19 inhibition |
CYP2C9 inhibition |
CYP2D6 inhibition |
CYP2D6 substrate |
CYP3A4 inhibition |
CYP3A4 substrate |
Total clearance (log ml min−1 kg−1) |
1a |
None |
None |
Inhibitor |
None |
None |
Substrate |
0.35 |
1b |
None |
None |
Inhibitor |
None |
None |
Substrate |
0.30 |
1c |
None |
None |
Inhibitor |
None |
None |
Substrate |
0.27 |
1d |
None |
None |
Inhibitor |
None |
None |
Substrate |
−0.05 |
1e |
None |
None |
Inhibitor |
None |
None |
Substrate |
1.63 |
1f |
None |
None |
Inhibitor |
None |
None |
Substrate |
0.06 |
1g |
Inhibitor |
Inhibitor |
Inhibitor |
Substrate |
None |
Substrate |
0.27 |
1h |
None |
None |
None |
None |
None |
Substrate |
0.29 |
1i |
None |
None |
None |
None |
None |
Substrate |
0.29 |
1j |
None |
None |
None |
None |
None |
Substrate |
0.28 |
1k |
None |
None |
None |
None |
None |
Substrate |
0.29 |
1l |
None |
None |
Inhibitor |
None |
None |
Substrate |
0.11 |
1m |
None |
None |
Inhibitor |
None |
None |
Substrate |
0.35 |
The blood–brain barrier (BBB) penetration and plasma protein binding (PPB) are used to characterize the distribution of compounds. All compounds 1a–m exhibited higher BBB penetration values in the range 0.21–10.36, indicating that they can cross the BBB. For the plasma protein binding, values of % bound of all isoindolin-1-ones 1a–m were estimated more than 90%, indicating decreased excretion and increased half-life.51
Cytochrome P450 isoforms (CYP2C19, CYP2C9, CYP2D6 and CYP3A4) is an important enzyme for drug metabolism in liver. Metabolism is predicted based on the CYP models for substrate or inhibition. This investigation revealed that most of 3-substituted-isoindolin-1-ones were non-inhibitors for CYP2C19 and CYP2C9 and were not substrates for CYP2D6, except 1g. Only a few compounds (1i–k) were not inhibitor for CYP2D6. The compound 1a–m were non-inhibitors and substrate of the CYP3A4 enzyme.
Excretion is predicted based on the total clearance. The higher the total clearance value of the compound, the faster the excretion process.52 The prediction showed that the total clearance of 1e was the highest and followed by 1a, 1m, 1b, 1h, 1i, 1k, 1j, 1c, 1g, 1l, 1f and 1d.
The toxicity of the compounds 1a–m was predicted by the Ames toxicity (mutagenicity) and carcinogenicity mouse and rat. The toxicity prediction showed that all compounds act as mutagen. Only compound 1e and 1f had positive carcinogenicity in mouse while only compound 1k had positive for rat.
Conclusion
Numbers of substituted 3-substitutedisoindolin-1-ones 1 has been conveniently and directly accessed from various (Z)-3-benzylideneisobenzofuran-1(3H)-ones 2 and primary amines 3 thanks to ultrasound-assisted-one-pot reaction strategy. The developed methodology provided the desired compounds 1a–m as a racemic mixture in good to excellent yields and can be scaled up using 10 mmol scale. The results showed that introducing aliphatic, halogen, methyl, methoxy and heterocyclic groups on isoindolin-1-one scaffold could enhance their antiplasmodium activities against chloroquine-sensitive (3D7) and -resistant (FCR3) strains of Plasmodium falciparum. In addition, the presence of substituent at 4-position of N-benzyl moiety played important role in antiplasmodium activity. Based on the in vitro antiplasmodium activity, the resistance indice value, the prediction of physicochemical and pharmacokinetic properties, 4 out of 13 tested compounds, namely 1a, 1d, 1h, and 1l, may become promising antiplasmodium agents.
Materials and methods
Chemistry
All the experiments were carried out under argon atmosphere in an ultrasonic bath (Powersonic 505, 40 kHz, 350 W). The solvents for the synthesis were initially distilled from calcium hydride. The chemicals used in this study were purchased from Merck and used without any further purification. The reaction was monitored using thin layer chromatography on Merck Kieselgel 60 F254 plates and was observed under UV light (254 nm). The purification of product was carried out using column chromatography on Merck Kiesgel 60 (0.040–0.063 nm).
The melting point was determined using the electrothermal apparatus (Electrothermal 9100). The NMR spectra were recorded on JEOL JNM-ECZ500R (at 500 MHz for 1H and 125 MHz for 13C). The chemical shifts were reported in parts per million (ppm) relative to the internal solvent signal of CDCl3 (δH 7.26 ppm and δC 77.16 pm). Multiplicity is indicated as follows: s (singlet), d (doublet), t (triplet), q (quartet), m (multiplet), ddd (doublet of doublet of doublet), dd (doublet of doublet), dt (doublet of triplet), and td (triplet of doublet). The additional NMR analysis was conducted using DEPT, COSY and HMQC. The high-resolution mass spectrometry experiments were conducted using a QSTAR Elite mass spectrometer (Applied Biosystems SCIEX) or a SYNAPT G2 HDMS mass spectrometer (Waters) equipped with an electrospray ionization source operated in the positive ion mode. The IR spectra were recorded from Shimadzu Prestige-21, where the samples were prepared as KBr pellets.
General synthesis of 3-alkylisoindolin-1-ones (1)
(Z)-3-benzylideneisobenzofuran-1(3H)-one derivatives 2 (0.5 mmol, 1 equiv.) and primary amines 3 (1 mmol, 2 equiv.) was dissolved in 1 mL of acetonitrile. The flask was placed in the pre-heated ultrasonic bath (50 °C) and the reaction was carried out for 30 min. The reaction mixture was placed in the ice bath, followed with the addition of NaBH3CN (314 mg, 5 mmol, 10 equiv.) and trifluoroacetic acid (0.39 mL, 5 mmol, 10 equiv.). The reaction was continued under ultrasonic irradiation at 50 °C for 60 min. The reaction was quench with the addition of the saturated aqueous solution of NaHCO3, followed with the extraction with dichloromethane (3 × 5 mL). The combined organic layer was washed with brine, dried over Na2SO4, filtered and removed under vacuum. The crude product was purified by column chromatography using the eluent of n-hexane/ethyl acetate (9
:
1).
3-Benzyl-2-butylisoindolin-1-one (1a). Small scale: white solid; yield: 130 mg (93%); mp: 88–91 °C. Scaled up synthesis: white solid; 2.48 g (89%); mp: 89–92 °C. IR (KBr): 3024, 2970, 2924, 2862, 1681, 1604, 1458, 1419, 1273, 1095, 702 cm−1; 1H-NMR (CDCl3, 500 MHz): δ = 7.78–7.76 (m, 1H, CHAr), 7.40–7.36 (m, 2H, CHAr), 7.28–7.22 (m, 3H, CHAr), 7.09–7.07 (m, 2H, CHAr), 6.92–6.90 (m, 1H, CHAr), 4.80 (dd, J = 8 and 4.5 Hz, 1H, CH), 4.13–4.07 (m, 1H, CH2), 3.40 (dd, J = 14 and 4.5 Hz, 1H, CH2), 3.22 (ddd, J = 14, 8.5, 5.5 Hz, 1H, CH2), 2.84 (dd, J = 14 and 8 Hz, 1H, CH2), 1.71–1.59 (m, 2H, CH2), 1.40–1.32 (m, 2H, CH2), 0.96 (t, J = 7.5 Hz, 3H, CH3); 13C-NMR (CDCl3, 125 MHz): δ = 168.3 (C
O), 144.9 (CAr), 136.1 (CAr), 132.5 (CAr), 130.8 (CHAr), 129.6 (2CHAr), 128.6 (2CHAr), 128.2 (CHAr), 127.1 (CHAr), 123.6 (CHAr), 123.0 (CHAr), 60.0 (CH), 40.0 (CH2), 38.5 (CH2), 30.5 (CH2), 20.2 (CH2), 13.9 (CH3); HR-MS (ESI): m/z [M + Na]+ cald for C19H21NONa+: 302.1515, found: 302.1517.
3-Benzyl-2-phenethylisoindolin-1-one (1b). Yellow oil; yield: 130 mg (79%); IR (nujol): 3023, 2970, 2924, 2860, 1681, 1604, 1412, 1080, 756, 702 cm−1; 1H-NMR (CDCl3, 500 MHz): δ = 7.79–7.77 (m, 1H, CHAr), 7.41–7.35 (m, 2H, CHAr), 7.30–7.21 (m, 6H, CHAr), 7.19–7.17 (m, 2H, CHAr), 6.98–6.96 (m, 2H, CHAr), 6.86–6.85 (m, 1H, CHAr), 4.52 (dd, J = 7.5 and 5.0 Hz, 1H, CH), 4.33–4.27 (m, 1H, CH2), 3.44–3.34 (m, 1H, CH2), 3.25 (dd, J = 14.0 and 5.0 Hz, 1H, CH2), 3.01–2.90 3.44–3.34 (m, 2H, CH2), 2.76 (dd, J = 14.0 and 8.0 Hz, 1H, CH2); 13C-NMR (CDCl3, 125 MHz): δ = 168.4 (C
O), 145.1 (CAr), 139.1 (CAr), 136.2 (CAr), 132.3 (CAr), 131.0 (CHAr), 129.5 (2CHAr), 128.9 (2CHAr), 128.8 (2CHAr), 128.6 (2CHAr), 128.2 (CHAr), 127.1 (CHAr), 126.7 (CHAr), 123.6 (CHAr), 122.9 (CHAr), 60.8 (CH), 42.2 (CH2), 38.6 (CH2), 35.0 (CH2); HR-MS (ESI): m/z [M + H]+ cald for C23H22NO+: 328.1696, found: 328.1693.
2,3-Dibenzylisoindolin-1-one (1c). Light yellow solid; mp: 98–101 °C; yield: 121 mg (77%); IR (KBr): 3024, 2970, 2924, 2860, 1681, 1604, 1435, 1072, 972, 694 cm−1; 1H-NMR (CDCl3, 500 MHz): δ = 7.85–7.83 (m, 1H, CHAr), 7.43–7.37 (m, 2H, CHAr), 7.34–7.28 (m, 3H, CHAr), 7.25–7.21 (m, 5H, CHAr), 7.00–6.98 (m, 2H, CHAr), 6.87 (d, J = 7.5 Hz, 1H, CHAr), 5.46 (d, J = 15.0 Hz, 1H, CH2), 4.57 (dd, J = 8.0 and 5.0 Hz, 1H, CH), 4.22 (d, J = 15.0 Hz, 1H, CH2), 3.37 (dd, J = 14.0 and 5.5 Hz, 1H, CH2), 2.83 (dd, J = 14.0 and 8.0 Hz, 1H, CH2); 13C-NMR (CDCl3, 125 MHz): δ = 168.6 (C
O), 145.2 (CAr), 137.2 (CAr), 136.2 (CAr), 132.1 (CAr), 131.2 (CHAr), 129.6 (2CHAr), 128.9 (2CHAr), 128.7 (2CHAr), 128.3 (CHAr), 128.2 (2CHAr), 127.8 (CHAr), 127.1 (CHAr), 123.9 (CHAr), 123.1 (CHAr), 59.7 (CH), 44.4 (CH2), 38.5 (CH2); HR-MS (ESI): m/z [M + H]+ cald for C22H20NO+: 314.1539, found: 314.1539.
3-Benzyl-2-(4-chlorobenzyl)isoindolin-1-one (1d). Yellow solid; mp: 108–110 °C; yield: 131 mg (75%); IR (KBr): 3020, 2975, 2925, 2860, 1680, 1603, 1404, 1072, 879, 702 cm−1; 1H-NMR (CDCl3, 500 MHz): δ = 7.85–7.83 (m, 1H, CHAr), 7.45–7.39 (m, 2H, CHAr), 7.31–7.24 (m, 5H, CHAr), 7.12–7.11 (m, 2H, CHAr), 7.02–7.00 (m, 2H, CHAr), 6.91 (d, J = 8.0 Hz, 1H, CHAr), 5.36 (d, J = 15.0 Hz, 1H, CH2), 4.57 (dd, J = 7.5 and 5.0 Hz, 1H, CH), 4.18 (d, J = 15.0 Hz, 1H, CH2), 3.30 (dd, J = 14.0 and 5.5 Hz, 1H, CH2), 2.86 (dd, J = 14.0 and 7.5 Hz, 1H, CH2); 13C-NMR (CDCl3, 125 MHz): δ = 168.6 (C
O), 145.2 (CAr), 136.2 (CAr), 135.8 (CAr), 131.9 (CAr), 131.4 (CHAr), 129.7 (CAr), 129.59 (2CHAr), 129.56 (2CHAr), 129.1 (2CHAr), 128.7 (2CHAr), 128.4 (CHAr), 127.2 (CHAr), 124.0 (CHAr), 123.1 (CHAr), 59.8 (CH), 43.8 (CH2), 38.9 (CH2); HR-MS (ESI): m/z [M + H]+ cald for C22H19ClNO+: 348.1150, found: 348.1146.
3-Benzyl-2-(4-fluorobenzyl)isoindolin-1-one (1e). Yellow solid; mp: 108–112 °C; yield: 119 mg (72%); IR (KBr): 3024, 2975, 2924, 2865, 1682, 1603, 1404, 1219, 1072, 764, 702 cm−1; 1H-NMR (CDCl3, 500 MHz): δ = 7.85–7.83 (m, 1H, CHAr), 7.44–7.39 (m, 2H, CHAr), 7.28–7.23 (m, 3H, CHAr), 7.18–7.15 (m, 2H, CHAr), 7.02–6.98 (m, 4H, CHAr), 6.90 (d, J = 8.0 Hz, 1H, CHAr), 5.37 (d, J = 15.0 Hz, 1H, CH2), 4.56 (dd, J = 8.0 and 5.0 Hz, 1H, CH), 4.19 (d, J = 15.0 Hz, 1H, CH2), 3.32 (dd, J = 14.0 and 5.5 Hz, 1H, CH2), 2.86 (dd, J = 14.0 and 7.5 Hz, 1H, CH2); 13C-NMR (CDCl3, 125 MHz): δ = 168.6 (C
O), 162.4 (d, J = 245 Hz, CAr), 145.2 (CAr), 136.2 (CAr), 133.0 (d, J = 3.0 Hz, CAr), 132.0 (CAr), 131.4 (CHAr), 129.9 (d, J = 8.0 Hz, 2CHAr), 129.6 (2CHAr), 128.7 (2CHAr), 128.4 (CHAr), 127.2 (CHAr), 124.0 (CHAr), 123.1 (CHAr), 115.7 (d, J = 21.5 Hz, 2CHAr), 59.7 (CH), 43.7 (CH2), 38.8 (CH2); 13F-NMR (CDCl3, 470 MHz): δ = −114.7; HR-MS (ESI): m/z [M + H]+ cald for C22H19FNO+: 332.1445, found: 332.1443.
3-Benzyl-2-(4-(trifluoromethyl)benzyl)isoindolin-1-one (1f). Yellow oil; yield: 160 mg (84%); IR (nujol): 3022, 2974, 2924, 2860, 1683, 1404, 1217, 1072, 764, 702 cm−1; 1H-NMR (CDCl3, 500 MHz): δ = 7.87–7.85 (m, 1H, CHAr), 7.55 (d, J = 8.0 Hz, 2H, CHAr), 7.46–7.42 (m, 2H, CHAr), 7.28–7.25 (m, 5H, CHAr), 7.02–7.00 (m, 2H, CHAr), 6.95–6.96 (m, 1H, CHAr), 5.41 (d, J = 15.0 Hz, 1H, CH2), 4.59 (dd, J = 7.5 and 5.0 Hz, 1H, CH), 4.28 (d, J = 15.0 Hz, 1H, CH2), 3.28 (dd, J = 14.0 and 5.5 Hz, 1H, CH2), 2.91 (dd, J = 14.0 and 7.5 Hz, 1H, CH2); 13C-NMR (CDCl3, 125 MHz): δ = 168.8 (C
O), 145.2 (CAr), 141.5 (CAr), 136.1 (CAr), 131.8 (CAr), 131.6 (CHAr), 130.1 (q, J = 32.5 Hz, CAr), 129.5 (2CHAr), 128.8 (2CHAr), 128.5 (CHAr), 128.4 (2CHAr), 127.4 (CHAr), 125.9 (CHAr), 125.8 (CHAr), 124.1 (CHAr), 123.1 (CHAr), 122.1 (q, J = 270.0 Hz, CF3), 60.0 (CH), 44.1 (CH2), 39.0 (CH2); 13F-NMR (CDCl3, 470 MHz): δ = −62.5; HR-MS (ESI): m/z [M + H]+ cald for C23H19F3NO+: 382.1413, found: 382.1415.
3-Benzyl-2-(4-methylbenzyl)isoindolin-1-one (1g). White solid; mp: 118–120 °C; yield: 130 mg (79%); IR (KBr): 3025, 2973, 2924, 2865, 1681, 1404, 1072, 702 cm−1; 1H-NMR (CDCl3, 500 MHz): δ = 7.81 (d, J = 8.0 Hz, 1H, CHAr), 7.41–7.34 (m, 2H, CHAr), 7.24–7.21 (m, 3H, CHAr), 7.14–7.10 (m, 4H, CHAr), 7.00–6.97 (m, 2H, CHAr), 6.83 (d, J = 8.0 Hz, 1H, CHAr), 5.42 (d, J = 15.0 Hz, 1H, CH2), 4.54 (dd, J = 8.0 and 5.0 Hz, 1H, CH), 4.16 (d, J = 15.0 Hz, 1H, CH2), 3.36 (dd, J = 14.0 and 5.0 Hz, 1H, CH2), 2.80 (dd, J = 14.0 and 8.0 Hz, 1H, CH2), 2.32 (s, 3H, CH3); 13C-NMR (CDCl3, 125 MHz): δ = 168.5 (C
O), 145.2 (CAr), 137.5 (CAr), 136.2 (CAr), 134.3 (CAr), 132.2 (CAr), 131.2 (CHAr), 129.6 (2CHAr), 129.5 (2CHAr), 128.6 (2CHAr), 128.33 (2CHAr), 128.32 (CHAr), 127.1 (CHAr), 123.9 (CHAr), 123.1 (CHAr), 59.6 (CH), 44.1 (CH2), 38.5 (CH2), 21.3 (CH3); HR-MS (ESI): m/z [M + H]+ cald for C23H22NO+: 328.1696, found: 328.1693.
3-Benzyl-2-(4-methoxybenzyl)isoindolin-1-one (1h). White solid; mp: 92–98 °C; yield: 148 mg (86%); IR (KBr): 3025, 2973, 2924, 2860, 1682, 1512, 1404, 1242, 1180, 1072, 756, 702 cm−1; 1H-NMR (CDCl3, 500 MHz): δ = 7.82 (d, J = 7.0 Hz, 1H, CHAr), 7.41 (td, J = 7.0 and 1 Hz, 1H, CHAr), 7.37 (td, J = 7.0 and 1 Hz, 1H, CHAr), 7.25–7.23 (m, 3H, CHAr), 7.16 (d, J = 8.0 Hz, 2H, CHAr), 7.01 (d, J = 8.0 Hz, 1H, CHAr), 7.00 (d, J = 7.0 Hz, 1H, CHAr), 6.86–6.84 (m, 1H, CHAr), 6.84 (d, J = 8.0 Hz, 2H, CHAr), 5.41 (d, J = 15.0 Hz, 1H, CH2), 4.55 (dd, J = 8.0 and 5.0 Hz, 1H, CH), 4.15 (d, J = 15.0 Hz, 1H, CH2), 3.79 (s, 3H, CH3) 3.37 (dd, J = 13.5 and 5.0 Hz, 1H, CH2), 2.82 (dd, J = 13.5 and 8.0 Hz, 1H, CH2); 13C-NMR (CDCl3, 125 MHz): δ = 168.5 (C
O), 159.2 (CAr), 145.2 (CAr), 136.3 (CAr), 132.3 (CAr), 131.2 (CHAr), 129.6 (4CHAr), 129.3 (CAr), 128.7 (2CHAr), 128.3 (CHAr), 127.1 (CHAr), 123.9 (CHAr), 123.1 (CHAr), 114.3 (2CHAr), 59.6 (CH), 55.4 (CH3), 43.7 (CH2), 38.5 (CH2); HR-MS (ESI): m/z [M + H]+ cald for C23H22NO2+: 344.1645, found: 344.1645.
3-Benzyl-2-(3-methoxybenzyl)isoindolin-1-one (1i). Yellow oil; yield: 131 mg (76%); IR (nujol): 3025, 2973, 2924, 2860, 1682, 1512, 1411, 1257, 1049, 702 cm−1; 1H-NMR (CDCl3, 500 MHz): δ = 7.83 (d, J = 6.5 Hz, 1H, CHAr), 7.42 (td, J = 6.5 and 1 Hz, 1H, CHAr), 7.38 (td, J = 6.5 and 1 Hz, 1H, CHAr), 7.25–7.22 (m, 4H, CHAr), 7.00 (dd, J = 7.5 and 2 Hz, 2H, CHAr), 6.87 (d, J = 6.5 Hz, 1H, CHAr), 6.83 (d, J = 2 Hz, 1H, CHAr), 6.81 (d, J = 2 Hz, 1H, CHAr), 6.76 (t, J = 2 Hz, 1H, CHAr), 5.43 (d, J = 15.0 Hz, 1H, CH2), 4.60 (dd, J = 8.0 and 5.0 Hz, 1H, CH), 4.19 (d, J = 15.0 Hz, 1H, CH2), 3.77 (s, 3H, CH3), 3.37 (dd, J = 14.0 and 5.0 Hz, 1H, CH2), 2.83 (dd, J = 14.0 and 8.0 Hz, 1H, CH2); 13C-NMR (CDCl3, 125 MHz): δ = 168.5 (C
O), 160.1 (CAr), 145.2 (CAr), 138.8 (CAr), 136.2 (CAr), 132.1 (CAr), 131.2 (CHAr), 129.9 (CHAr), 129.6 (2CHAr), 128.7 (2CHAr), 128.3 (CHAr), 127.1 (CHAr), 124.0 (CHAr), 123.1 (CHAr), 120.6 (CHAr), 113.8 (CHAr), 113.3 (CHAr), 59.7 (CH), 55.4 (CH3), 44.3 (CH2), 38.6 (CH2); HR-MS (ESI): m/z [M + H]+ cald for C23H22NO2+: 344.1645, found: 344.1646.
3-Benzyl-2-(2-methoxybenzyl)isoindolin-1-one (1j). Yellow oil; yield: 130 mg (76%); IR (nujol): 3032, 2973, 2924, 2860, 1682, 1412, 1103, 756, 702 cm−1; 1H-NMR (CDCl3, 500 MHz): δ = 7.77 (d, J = 7.0 Hz, 1H, CHAr), 7.37–7.32 (m, 2H, CHAr), 7.28–7.20 (m, 5H, CHAr), 6.99–6.98 (m, 2H, CHAr), 6.91–6.90 (m, 2H, CHAr), 6.78 (d, J = 7 Hz, 1H, CHAr), 5.23 (d, J = 15.0 Hz, 1H, CH2), 4.59 (m, 1H and 1H, CH and CH2), 3.87 (s, 3H, CH3) 3.56–3.53 (m, 1H, CH2), 2.80–2.75 (m, 1H, CH2); 13C-NMR (CDCl3, 125 MHz): δ = 168.6 (C
O), 157.5 (CAr), 145.3 (CAr), 136.4 (CAr), 132.4 (CAr), 130.9 (CHAr), 130.5 (CHAr), 129.7 (2CHAr), 129.1 (CHAr), 128.5 (2CHAr), 128.1 (CHAr), 127.0 (CHAr), 125.5 (CAr), 123.7 (CHAr), 123.1 (CHAr), 121.1 (CHAr), 110.6 (CHAr), 60.2 (CH), 55.6 (CH3), 38.6 (CH2), 38.1 (CH2); HR-MS (ESI): m/z [M + H]+ cald for C23H22NO2+: 344.1645, found: 344.1642.
3-Benzyl-2-(furan-2-ylmethyl)isoindolin-1-one (1k). Orange solid; mp: 98–101 °C; yield: 120 mg (79%); IR (KBr): 3032, 2973, 2932, 2860, 1682, 1466, 1420, 1211, 1103, 756, 710 cm−1; 1H-NMR (CDCl3, 500 MHz): δ = 7.81–7.79 (m, 1H, CHAr), 7.41–7.35 (m, 3H, CHAr), 7.28–7.22 (m, 3H, CHAr), 7.06–7.04 (m, 2H, CHAr), 6.87–6.85 (m, 1H, CHAr), 6.33 (dd, J = 3.5 and 1.0 Hz, 1H, CHAr), 6.27 (d, J = 3.5 Hz, 1H, CHAr), 5.33 (d, J = 16.0 Hz, 1H, CH2), 4.67 (dd, J = 8.0 and 5.0 Hz, 1H, CH), 4.30 (d, J = 15.0 Hz, 1H, CH2), 3.47 (dd, J = 14.0 and 5.0 Hz, 1H, CH2), 2.82 (dd, J = 14.0 and 8.5 Hz, 1H, CH2); 13C-NMR (CDCl3, 125 MHz): δ = 168.2 (C
O), 150.6 (CAr), 145.2 (CAr), 142.6 (CHAr), 136.2 (CAr), 132.0 (CAr), 131.3 (CHAr), 129.7 (2CHAr), 128.7 (2CHAr), 128.3 (CHAr), 127.2 (CHAr), 123.9 (CHAr), 123.1 (CHAr), 110.6 (CHAr), 108.8 (CHAr), 60.3 (CH), 38.4 (CH2), 37.2 (CH2); HR-MS (ESI): m/z [M + H]+ cald for C20H18NO2+: 304.1332, found: 304.1330.
3-Benzyl-2-(thiophen-2-ylmethyl)isoindolin-1-one (1l). White solid; mp: 106–108 °C; yield: 120 mg (75%); IR (KBr): 3032, 2973, 2928, 2860, 1682, 1420, 1080, 694, 570 cm−1; 1H-NMR (CDCl3, 500 MHz): δ = 7.82 (d, J = 8.5 Hz, 1H, CHAr), 7.43–7.38 (m, 2H, CHAr), 7.29–7.23 (m, 4H, CHAr), 7.07 (d, J = 7.0 Hz, 2H, CHAr), 6.97–6.95 (m, 2H, CHAr), 6.89 (dd, J = 3.5 Hz, 1H, CHAr), 6.27 (d, J = 3.5 Hz, 1H, CHAr), 5.53 (d, J = 16.0 Hz, 1H, CH2), 4.71 (dd, J = 7.5 and 5.5 Hz, 1H, CH), 4.42 (d, J = 15.0 Hz, 1H, CH2), 3.41 (dd, J = 13.5 and 5.0 Hz, 1H, CH2), 2.88 (dd, J = 13.5 and 8.0 Hz, 1H, CH2); 13C-NMR (CDCl3, 125 MHz): δ = 168.3 (C
O), 145.3 (CAr), 139.6 (CAr), 136.2 (CAr), 131.9 (CAr), 131.4 (CHAr), 129.6 (2CHAr), 128.7 (2CHAr), 128.4 (CHAr), 127.2 (CHAr), 127.1 (CHAr), 126.9 (CHAr), 125.8 (CHAr), 124.0 (CHAr), 123.1 (CHAr), 59.6 (CH), 38.9 (CH2), 38.7 (CH2); HR-MS (ESI): m/z [M + H]+ cald for C20H18NOS+: 320.1104, found: 320.1102.
2-Butyl-3-(4-methylbenzyl)isoindolin-1-one (1m). White solid; mp: 96–101 °C; yield: 121 mg (82%); IR (KBr): 3031, 2973, 2925, 2865, 1682, 1404, 1072, 702 cm−1; 1H-NMR (CDCl3, 500 MHz): δ = 7.77–7.76 (m, 1H, CHAr), 7.39–7.37 (m, 2H, CHAr), 7.05 (d, J = 8 Hz, 2H, CHAr), 6.95 (d, J = 8 Hz, 2H, CHAr), 6.94–6.93 (m, 1H, CHAr), 4.75 (dd, J = 8 and 5.0 Hz, 1H, CH), 4.10–4.04 (m, 1H, CH2), 3.34 (dd, J = 14 and 4.5 Hz, 1H, CH2), 3.20 (ddd, J = 14, 8.5, 5.5 Hz, 1H, CH2), 2.77 (dd, J = 14 and 8 Hz, 1H, CH2), 2.31 (s, 3H, CH3), 1.69–1.58 (m, 2H, CH2), 1.39–1.31 (m, 2H, CH2), 0.94 (t, J = 7.0 Hz, 3H, CH3); 13C-NMR (CDCl3, 125 MHz): δ = 168.4 (C
O), 145.1 (CAr), 136.7 (CAr), 133.0 (CAr), 132.6 (CAr), 130.8 (CHAr), 129.4 (2CHAr), 129.3 (2CHAr), 128.2 (CHAr), 123.6 (CHAr), 123.0 (CHAr), 60.1 (CH), 40.0 (CH2), 38.0 (CH2), 30.6 (CH2), 21.2 (CH3), 20.3 (CH2), 13.9 (CH3); HR-MS (ESI): m/z [M + H]+ cald for C20H24NO+: 294.1852, found: 294.1854.
In vitro antiplasmodium assay
The cytotoxicity of products was evaluated using Plasmodium falciparum strains of 3D7 (chloroquine-sensitive) and FCR3 (chloroquine-resistant). The antiplasmodium assay was conducted using candle jar method.
For the in vitro assay, each synthesized compound was dissolved in DMSO and was prepared in a series of concentration, i.e. 10, 5, 2.5, 1.25 and 0.625 μg mL−1 in RPMI medium. A total of 100 μL of each series of concentrations was put into the 96-well microplate with three repetition and then 100 μL Plasmodium suspension was added. The culture was incubated at 37 °C for 72 h, a thin blood smear was made and treated with 20% Giemsa dyes. The percentage of parasitemia was determined by calculating the number of the infected erythrocytes for minimum 1000 erythrocytes and then used to calculate the inhibition percentage of P. falciparum growth. The antiplasmodium activities were presented as mean of IC50 values. The IC50 value was calculated by probit analysis using SPSS software.
Physicochemical and pharmacokinetic properties
The pharmacokinetic properties including absorption, distribution, metabolism, and toxicity parameters were screened through the PreADMET web (http:/preadmet.bmdrc.org). Moreover, the excretion parameters were determined using the pkCSM web (https://biosig.lab.uq.edu.au/pkcsm/).
Author contributions
Conceptualization, M. I. D. M. and L. C.; synthesis and analysis, M. F. H., H. S. S. and M. I. D. M.; antiplasmodium assay, C. R. M.; supervision, M. I. D. M., E. S. K. and B. P.; writing-draft preparation, M. I. D. M. and L. C.; writing-review and editing, M. I. D. M. and L. C. All authors have read the manuscript.
Conflicts of interest
There are no conflicts to declare.
Acknowledgements
Technical support from Laboratory of Organic Chemistry, Universitas Gadjah Mada is highly acknowledged.
Notes and references
- H. A. H. Elshemy, M. A. Zaki, E. I. Mohamed, S. I. Khan and P. F. Lamie, Bioorg. Chem., 2020, 97, 103673 CrossRef CAS PubMed.
- A. Jaromin, A. Czopek, S. Parapini, N. Basilico, E. Misiak, J. Gubernator and A. Zagórska, Biomolecules, 2020, 11, 33 CrossRef PubMed.
- J. N. Burrows, S. Duparc, W. E. Gutteridge, R. Hooft van Huijsduijnen, W. Kaszubska, F. Macintyre, S. Mazzuri, J. J. Möhrle and T. N. C. Wells, Malar. J., 2017, 16, 26 CrossRef PubMed.
- S. P. Upadhyay, P. Thapa, R. Sharma and M. Sharma, Fitoterapia, 2020, 146, 104722 CrossRef CAS PubMed.
- V. Choomuenwai, K. D. Beattie, P. C. Healy, K. T. Andrews, N. Fechner and R. A. Davis, Phytochem, 2015, 117, 10–16 CrossRef CAS.
- N. E. Rasol, F. B. Ahmad, X.-Y. Lim, F. F.-L. Chung, C.-O. Leong, C.-W. Mai, N. V. Bihud, H. M. Zaki and N. H. Ismail, Phytochem. Lett., 2018, 24, 51–55 CrossRef CAS.
- S. Caccamese, G. Scivoli, S. Bianca, J. M. López-Romero and F. J. Ortiz-López, J. Chromatogr. A, 2006, 1129, 140–144 CrossRef CAS PubMed.
- M. Dutta, S. M. Mandal, R. Pegu and S. Pratihar, J. Org. Chem., 2017, 82, 2193–2198 CrossRef CAS PubMed.
- A. K. Maity and S. Roy, Adv. Synth. Catal., 2014, 356, 2627–2642 CrossRef CAS.
- Z. Chen, L. Wu, H. Fang, T. Zhang, Z. Mao, Y. Zou, X. Zhang and M. Yan, Adv. Synth. Catal., 2017, 359, 3894–3899 CrossRef CAS.
- F.-Z. Han, B.-B. Su, L.-N. Jia, P.-W. Wang and X.-P. Hu, Adv. Synth. Catal., 2017, 359, 146–152 CrossRef CAS.
- Y. Tian, J. Sun, K. Zhang, G. Li and F. Xu, Synthesis, 2018, 50, 2255–2265 CrossRef CAS.
- N. Muniraj and K. R. Prabhu, Adv. Synth. Catal., 2018, 360, 1370–1375 CrossRef CAS.
- N. Muniraj and K. R. Prabhu, Adv. Synth. Catal., 2018, 360, 3579–3584 CrossRef CAS.
- H. Miura, S. Terajima, K. Tsutsui and T. Shishido, J. Org. Chem., 2017, 82, 1231–1239 CrossRef CAS PubMed.
- S. Shaaban, C. Davies, C. Merten, J. Flegel, F. Otte, C. Strohmann and H. Waldmann, Chem. –Eur. J., 2020, 26, 10729–10734 CrossRef CAS PubMed.
- Y. Zhang, D. Wang and S. Cui, Org. Lett., 2015, 17, 2494–2497 CrossRef CAS PubMed.
- R. Kawęcki, W. Stańczyk and A. Jaglińska, Tetrahedron, 2018, 74, 578–584 CrossRef.
- J. E. Rode, K. Lyczko, M. Jawiczuk, R. Kawęcki, W. Stańczyk, A. Jaglińska and J. Cz. Dobrowolski, ChemPhysChem, 2018, 19, 2411–2422 CrossRef CAS PubMed.
- W. Zhou, Y.-X. Zhang, X.-D. Nie, C.-M. Si, X. Sun and B.-G. Wei, J. Org. Chem., 2018, 83, 9879–9889 CrossRef CAS PubMed.
- X.-Y. Liu, X.-M. Luo and L.-F. Tang, Tetrahedron, 2020, 76, 131341 CrossRef CAS.
- L.-Y. Fu, J. Ying, X. Qi, J.-B. Peng and X.-F. Wu, J. Org. Chem., 2019, 84, 1421–1429 CrossRef CAS PubMed.
- T. Yao, X. Liang, Z. Guo and D. Yang, Tetrahedron, 2019, 75, 3088–3100 CrossRef CAS.
- A. G. Griesbeck, J.-M. Neudörfl, B. Goldfuss and S. Molitor, ChemPhotoChem, 2017, 1, 355–362 CrossRef CAS.
- N. Kise, S. Isemoto and T. Sakurai, Tetrahedron, 2012, 68, 8805–8816 CrossRef CAS.
- M.-W. Chen, Q.-A. Chen, Y. Duan, Z.-S. Ye and Y.-G. Zhou, Chem. Commun., 2012, 48, 1698–1700 RSC.
- M. I. D. Mardjan, M. F. Hariadi, I. M. Putri, N. A. Musyarrofah, M. Salimah, P. Priatmoko, B. Purwono and L. Commeiras, RSC Adv., 2022, 12, 19016–19021 RSC.
- Y. Hayashi, Chem. Sci., 2016, 7, 866–880 RSC.
- B. Banerjee, Ultrason. Sonochem., 2017, 35, 1–14 CrossRef CAS PubMed.
- B. Banerjee, Ultrason. Sonochem., 2017, 35, 15–35 CrossRef CAS PubMed.
- G. Kaur, A. Sharma and B. Banerjee, ChemistrySelect, 2018, 3, 5283–5295 CrossRef CAS.
- M. C. Bubalo, I. Sabotin, I. Radoš, J. Valentinčič, T. Bosiljkov, M. Brnčić and P. Žnidaršič-Plazl, Green Process. Synth., 2013, 2, 579–590 CrossRef CAS.
- L. da Silveira Pinto and M. de Souza, Synthesis, 2017, 49, 2677–2682 CrossRef CAS.
- B. Savun-Hekimoğlu, Acoustics, 2020, 2, 766–775 CrossRef.
- S. V. Sancheti and P. R. Gogate, Ultrason. Sonochem., 2017, 36, 527–543 CrossRef CAS PubMed.
- G. Chatel and R. S. Varma, Green Chem., 2019, 21, 6043–6050 RSC.
- V. Castro-Castillo, C. Suárez-Rozas, A. Pabón, E. G. Pérez, B. K. Cassels and S. Blair, Bioorg. Med. Chem. Lett., 2013, 23, 327–329 CrossRef CAS PubMed.
- C. de Souza Barbosa, D. S. M. Guimarães, J. da Costa Alves, C. F. A. de Brito, R. M. Ribeiro-Viana, F. de Pilla Varotti and G. H. Ribeiro Viana, Int. J. Travel Med. Glob. Health, 2019, 7, 129–134 CrossRef.
- L. C. S. Pinheiro, L. M. Feitosa, M. O. Gandi, F. F. Silveira and N. Boechat, Molecules, 2019, 24, 4095 CrossRef PubMed.
- M. I. D. Mardjan, J.-L. Parrain and L. Commeiras, Adv. Synth. Catal., 2016, 358, 543–548 CrossRef CAS.
- M. I. D. Mardjan, S. Perie, J.-L. Parrain and L. Commeiras, Org. Biomol. Chem., 2017, 15, 3304–3309 RSC.
- M. I. D. Mardjan, A. Mayooufi, J.-L. Parrain, J. Thibonnet and L. Commeiras, Org. Process Res. Dev., 2020, 24, 606–614 CrossRef CAS.
- M. Zakiah, R. A. Syarif, M. Mustofa, J. Jumina, N. Fatmasari and E. N. Sholikhah, J. Trop. Med., 2021, 2021, 1–11 CrossRef PubMed.
- D. Cornut, H. Lemoine, O. Kanishchev, E. Okada, F. Albrieux, A. H. Beavogui, A.-L. Bienvenu, S. Picot, J.-P. Bouillon and M. Médebielle, J. Med. Chem., 2013, 56, 73–83 CrossRef CAS PubMed.
- K. C. Nicolaou, Angew. Chem., Int. Ed., 2014, 53, 9128–9140 CrossRef CAS PubMed.
- N. Sharma, D. Mohanakrishnan, U. K. Sharma, R. Kumar, R. Richa, A. K. Sinha and D. Sahal, Eur. J. Med. Chem., 2014, 79, 350–368 CrossRef CAS PubMed.
- N. S. Dambuza, P. Smith, A. Evans, J. Norman, D. Taylor, A. Andayi, T. Egan, K. Chibale and L. Wiesner, Malar. J., 2015, 14, 505 CrossRef PubMed.
- P. F. Salas, C. Herrmann, J. F. Cawthray, C. Nimphius, A. Kenkel, J. Chen, C. de Kock, P. J. Smith, B. O. Patrick, M. J. Adam and C. Orvig, J. Med. Chem., 2013, 56, 1596–1613 CrossRef CAS PubMed.
- C. A. Lipinski, F. Lombardo, B. W. Dominy and P. J. Feeney, Adv. Drug Delivery Rev., 2001, 46, 3–26 CrossRef CAS PubMed.
- L. A. Vélez, Y. Delgado, Y. Ferrer-Acosta, I. J. Suárez-Arroyo, P. Rodríguez and D. Pérez, Int. J. Plant Biol., 2022, 13, 163–179 CrossRef.
- A. M. Naglah, A. A. Askar, A. S. Hassan, T. K. Khatab, M. A. Al-Omar and M. A. Bhat, Molecules, 2020, 25, 1431 CrossRef CAS PubMed.
- D. E. V. Pires, T. L. Blundell and D. B. Ascher, J. Med. Chem., 2015, 58, 4066–4072 CrossRef CAS PubMed.
|
This journal is © The Royal Society of Chemistry 2023 |
Click here to see how this site uses Cookies. View our privacy policy here.