DOI:
10.1039/D3RA03193D
(Paper)
RSC Adv., 2023,
13, 26581-26586
Regioselective synthesis of 3-nitroindoles under non-acidic and non-metallic conditions†
Received
13th May 2023
, Accepted 26th July 2023
First published on 5th September 2023
Abstract
An electrophilic substitution reaction, without acid and metal, of indole with ammonium tetramethylnitrate for accessing 3-nitroindole has been developed. In this protocol, trifluoroacetyl nitrate (CF3COONO2) was produced by metathesis of ammonium tetramethyl nitrate and trifluoroacetic anhydride at sub-room temperature. Trifluoroacetyl nitrate (CF3COONO2) is an electrophilic nitrating agent for a variety of indoles, aromatic and heterocyclic aromaticity. Meanwhile, this strategy could be applied to construct the skeleton structure of many kinds of bioactive molecules. Interestingly, 3-nitroindole can be further derivatived as a pyrrolo[2,3-b]indole.
Introduction
Indole and its derivatives are important structural motifs in organic chemistry.1 In particular, 3-nitroindole is an important intermediate that has been widely used by scientists for the synthesis of organic molecules2 with biological activity (Scheme 1).3 However, the synthesis of 3-nitroindole still faces many challenges, including the lack of classical methods and low yield, and the use of nitric acid in the reaction process is not friendly to the environment.
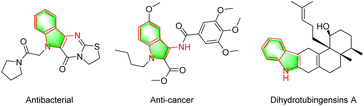 |
| Scheme 1 Biological compounds derived from 3-nitroindoles. | |
Therefore, it is of great significance to develop efficient and green methods for the synthesis of 3-nitroindole. At present, the preparation methods of 3-nitroindole and its derivatives mainly include strong acid,4 radical,5 electrochemistry6 and other methods (Scheme 2).7 Although there are some methods to prepare 3-nitroindole and its analogues, there are some defects. For example, the use of concentrated nitric acid has potential safety hazards and great harm to the environment, low yield of prepared products, poor functional group compatibility. Therefore, the convenient and efficient synthesis of 3-nitroindole and its analogues from simple starting materials still faces great challenges.
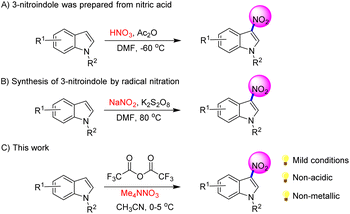 |
| Scheme 2 3-Nitroindoles was prepared from indoles. | |
Nitration is one of the most common and earliest organic reactions. The 1834 discovered the direct nitration of benzene to nitrobenzene. With the development of printing and dyeing industry,8 pharmaceutical industry9 and materials science industry,10 nitrification has been widely used in organic synthesis industry.11 Nitro compound by mixing acids is still the mainstream method.12 But the reaction's regioselectivity, by-products, and functional group tolerance have long puzzled scientists. In recent years, many nitration methods have been developed, including free radical,13 transition metal catalysis,14 electrochemistry,15 microwave16 and other methods.17 Although there are many nitrification reactions at present, the development of efficient, rapid and environment-friendly nitrification methods is still facing great challenges.
According to literature, ammonium nitrate can react with anhydride to form nitrates.18 We suspect that nitrates can further react with indole to form 3-nitroindole. Therefore, we tried the reaction with N-Boc indole and obtained the target product in medium yield. Herein, we have developed a method for the preparation of 3-nitroindole without acid.
Results and discussion
We initially studied the protocol with tert-butyl 1H-indole-1-carboxylate (1a) under various conditions (Table 1, see ESI for detailed data†). Gratifyingly, when trifluoroacetic anhydride and tetramethylammonium nitrate were used as reaction reagents, tert-butyl 3-nitro-1H-indole-1-carboxylate (2a) was obtained in medium yield (entry 1). Then we investigated other ammonium salts, which only got trace product except tetrabutylammonium nitrate which could get the product in medium yield (entries 2 and 3). We tried to improve the yield of the reaction by changing the type of anhydride. It was regrettable that acetic anhydride was not capable of performing this reaction (entry 4). The triflic anhydride could only get trace amounts of the product (entry 5). The exciting thing was that the yield was unexpectedly increased to 85% when the amount of trifluoroacetic anhydride was two equivalent (entry 6). We also investigated the effects of different solvents on the reaction, most of which could not get ideal results (entries 7 and 8). Interestingly, when acetonitrile was used as a solvent, the yield of the reaction could be dramatically increased to 97% (entry 9). What's more, the reaction was almost impossible when the temperature was reduced to −20 °C (entry 10). Finally, only trace amounts of the product were obtained when the temperature increased to 25 °C (entry 11).
Table 1 Optimization of the reaction conditionsa,b
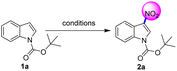
|
Entry |
Ammonium salts |
Anhydride |
Solvent |
T (°C) |
Yield (%), 2a |
Reaction conditions: 1a (0.5 mmol), ammonium salts (0.55 mmol), anhydride (1 mL), solvent (1 mL), 4 h. Yield refers to isolated product. 1 mmol of anhydride was used. |
1 |
NMe4NO3 |
(CF3CO)2O |
DCM |
0–5 |
78 |
2 |
KNO3 |
(CF3CO)2O |
DCM |
0–5 |
Trace |
3 |
NBu4NO3 |
(CF3CO)2O |
DCM |
0–5 |
56 |
4 |
NMe4NO3 |
(Ac)2O |
DCM |
0–5 |
NR |
5 |
NMe4NO3 |
(CF3SO2)2O |
DCM |
0–5 |
Trace |
6c |
NMe4NO3 |
(CF3CO)2O |
DCM |
0–5 |
85 |
7c |
NMe4NO3 |
(CF3CO)2O |
THF |
0–5 |
41 |
8c |
NMe4NO3 |
(CF3CO)2O |
DMSO |
0–5 |
Trace |
9c |
NMe4NO3 |
(CF3CO)2O |
CH3CN |
0–5 |
97 |
10c |
NMe4NO3 |
(CF3CO)2O |
DCM |
−20 |
NR |
11c |
NMe4NO3 |
(CF3CO)2O |
DCM |
25 |
Trace |
We explored the substrate universality of this protocol according to our preferred optimal conditions (Scheme 3). Therefore, a series of indole derivatives used to prepare derivatives of 3-nitroindole. In addition, X-ray diffraction patterns of 2g (CCDC: 2251492†) further proved that the nitration took place at position 3 of indole. It should be noted that in the next experiment we expanded the scale of the reaction to 1 mmol. When the indole 1 position was attached to the alkyl or benzyl group of the electron donor, the protocol could prepare the target product in a medium yield (2b–2d). What's more, the yield of 3-nitro-indole was slightly increased when the 1-position of indole was inserted into the phenyl group of drawing electron (2e). Interestingly, when the 2-position of indoles were connected to other substituents, regardless of the electron-donating or electron-withdrawing substitution, could proceed smoothly in this process to deliver corresponding 3-nitro-indoles in good to excellent yields (2f–2h). It was concluded that the effect of 2-position of indole electric property on the reaction was small. In addition, 4-substituted indoles could also deliver the products smoothly. But the yield of N-boc-4-bromoindole was lower than that of N-boc-4-chloroindole (2i–2j). Using 4-methyl-Boc-indole as starting material, the nitration product (2y see ESI for detailed data†) containing impurities was obtained in a yield of only 33%. This phenomenon showed that the 4-site steric hindrance had an effect on the reaction. Moreover, no matter the 5-position of indole was replaced by the nitro group or halogen, the reaction could proceed smoothly and the derivatives of 3-nitroindole could be obtained in good to excellent yield (2k–2m). In addition, when indole 6-linked substituents, the protocol can be successfully implemented and the target product can be obtained with a medium yield (2n). Gratifyingly, 7-substituted indole was also compatible with the protocol and carried out smoothly with good yield (2o). Furthermore, under this protocol, the corresponding 3-nitroindole can also be prepared from disubstituted indole in good yield (2p). The above cases showed that 5-7-substituted indoles had little influence on the protocol and could be used to prepare 3-nitroindoles in good to excellent yields (2k–2p).
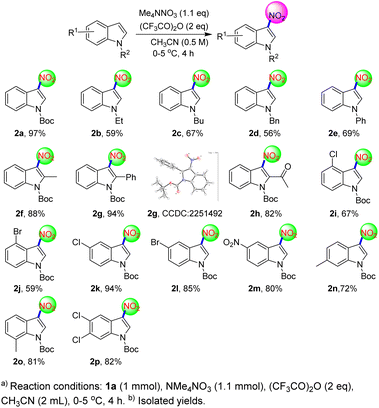 |
| Scheme 3 Substrate scope of indoles.a,b | |
Next we turned our attention to common aromatic and heteroaromatic hydrocarbons (Scheme 4). Gratifyingly, various aromatic and heteroaromatic hydrocarbons were well applicable in this transformation. Interestingly, benzene was compatible with this protocol and nitrobenzene was prepared in good yield (2q). Furthermore, the nitration of naphthalene and phenanthrene could be carried out smoothly under this protocol and the product could be obtained in good to excellent yield (2r–2s). Additionally, oxygen-containing aromatic hydrocarbons can be delivered successfully in good yields regardless of whether the oxygen atom is conjugated or not (2t–2u). Finally, sulfur-containing aromatic hydrocarbons are also compatible with the protocol to successfully prepare nitro compound in good yields (2v–2w). These examples showed that the protocol could be used to efficiently prepare other aromatic and heteroaromatic nitro compounds.
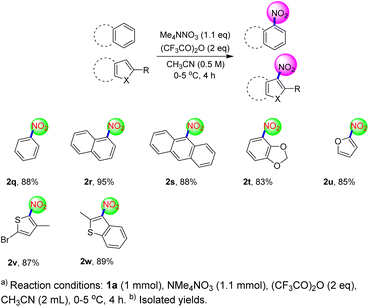 |
| Scheme 4 Substrate scope of aromatic and heteroaromatic hydrocarbons.a,b | |
In order to explore the potential application of this protocol, a 100 g scale reaction was carried out with N-Boc indole 1a, and the N-boc-3-nitroindole 2a was isolated in 91% yield (Scheme 5A). 3-Nitroindole is an important intermediate that has been widely used by scientists for the synthesis of organic molecules2 with biological activity.3 Although there were several methods for the synthesis of 3-nitroindole.4–7 However, the synthesis of 3-nitroindole without nitric acid still faces some challenges. Therefore, the deprotection of N-boc-3-nitroindole indicated the practical significance of this method (Scheme 5B). It is very challenging to assemble polyindoles in synthetic chemistry and medicinal chemistry.19 4-(tert-Butyl) 3-methyl pyrrolo[3,4-b]indole-3,4(2H)-dicarboxylate (4a) could be prepared by Barton–Zard reaction of tert-butyl 3-nitro-1H-indole-1-carboxylate (2a) in excellent yield.4c This result also reveals another value of the product (Scheme 5C).
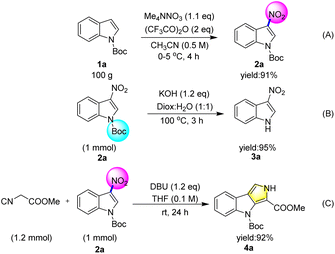 |
| Scheme 5 Scale-up reaction and synthetic application. | |
The calculated reaction transition states and processes were shown in Scheme 6. N-Boc indole (1a) and trifluoroacetyl nitrate (5) undergo a highly regioselective via TS to form product.
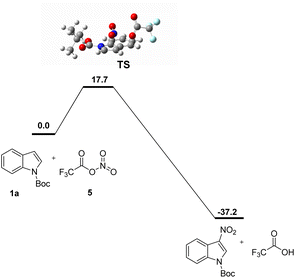 |
| Scheme 6 Complete reaction pathway. Free energies are computed at the PCM(dichloromethane)/B3LYP-D3BJ/6-31G(d)level and are in kcal mol−1. | |
To reveal the mechanism and Regioselectivity of this reaction, we conducted a series of controlled experiments (Scheme 7). The fact that the reaction could not be carried out smoothly when there was no anhydride in this protocol proved that anhydride was an indispensable condition of the protocol. If the indole 3-position is occupied by the methyl group, the reaction can not be carried out smoothly, which shows that the protocol has a strong regioselectivity. Laali had described that nitrate could decompose with trifluoroacetic anhydride to produce trifluoroacetyl nitrate (CF3COONO2), and it had strong electrophilic nitrification for many aromatic hydrocarbons.18 Based on the previous literature and the results of computational chemistry (Scheme 6), we proposed a possible reaction mechanism (Scheme 7). Initially, ammonium tetramethylnitrate and trifluoroacetic anhydride worked together to form trifluoroacetyl nitrate (A). Species A reacted with N-Boc indole to form four-member ring transition state B. The trifluoroacetic acid was shed from the transition state to form a product.
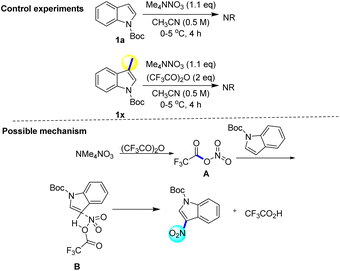 |
| Scheme 7 Control experiments and possible mechanism. | |
Conclusions
In summary, we have developed a practical method for the regioselective nitration of indoles using ammonium tetramethylnitrate under non-acidic and non-metallic conditions. This protocol is not only mild and environmentally friendly, but also reveals the aromatic electrophilic nitration of trifluoroacetyl nitrate, which provides a new method for the further construction of 3-nitroindole and its analogues. Meanwhile, the 3-nitroindole can be further derivatived as a pyrrolo[3,4-b]indole. In the future, we will explore the value of 3-nitroindole in pharmaceutical chemistry and synthetic chemistry.
Experimental
General procedure for the synthesis of 2
Add aromatic or heteroaromatic hydrocarbons (1 mmol) and NMe4NO3 (150 mg, 1.1 mmol) to the reaction tube and immediately dissolve it with acetonitrile (1 mL). The reaction system was then cooled to 0–5 °C and the trifluoroacetic anhydride solution (420 mg dissolved in 1 mL CH3CN) was added. The reaction system was incubated at 0–5 °C for 4 hours. At the same time, the reaction was monitored by TLC. When the reaction was completed, the reaction was quenched by saturated sodium carbonate. Extract with EA and transfer to a round bottom flask. Silica gel was added to the flask, and the solvent was evaporated under vacuum. Purified by silica gel column chromatography using ethyl acetate/petroleum ether as eluent to obtain compound 2.
Conflicts of interest
There are no conflicts to declare.
Acknowledgements
We are grateful for the financial support from The North Sichuan Medical College Talent Fund (No. CBY21-QD11 and CBY21-QD19) and Special Fund for Strategic Cooperation in Science and Technology in Nanchong (No. 22SXQT0396).
References
-
(a) I. G. Sonsona, Indole, a Privileged Structural Core Motif, Synlett, 2015, 26, 2325–2326 CrossRef;
(b) P. A. Patel, N. Kvaratskhelia, Y. Mansour, J. Antwi, L. Feng, P. Koneru, M. J. Kobe, N. Jena, G. Shi, M. S. Mohamed, C. Li, J. J. Kessl and J. R. Fuchs, Indole-based allosteric inhibitors of HIV-1 integrase, Bioorg. Med. Chem. Lett., 2016, 26, 4748–4752 CrossRef CAS PubMed;
(c) M.-Z. Zhang, Q. Chen and G.-F. Yang, A review on recent developments of indole-containing antiviral agents, Eur. J. Med. Chem., 2015, 89, 421–441 CrossRef CAS PubMed.
-
(a) M. Laugeois, J. Ling, C. Férard, V. Michelet, V. Ratovelomanana-Vidal and M. R. Vitale, Palladium(0)-Catalyzed Dearomative [3+2] Cycloaddition of 3-Nitroindoles with Vinylcyclopropanes: An Entry to Stereodefined 2,3-Fused Cyclopentannulated Indoline Derivatives, Org. Lett., 2017, 19, 2266–2269 CrossRef CAS PubMed;
(b) Y. Li, F. Tur, R. P. Nielsen, H. Jiang, F. Jensen and K. A. Jørgensen, Enantioselective Formal [4+2] Cycloadditions to 3-Nitroindoles by Trienamine Catalysis: Synthesis of Chiral Dihydrocarbazoles, Angew. Chem., Int. Ed., 2016, 55, 1020–1024 CrossRef CAS PubMed;
(c) S. Pandit, V. K. Pandey, A. S. Adhikari, S. Kumar, A. K. Maurya, R. Kant and N. Majumda, Palladium-Catalyzed Dearomative [4+2]-Cycloaddition toward Hydrocarbazoles, J. Org. Chem., 2023, 88, 97–105 CrossRef CAS PubMed;
(d) L. Birbaum, L. Gillard, H. Gérard, H. Oulyadi, G. Vincent, X. Moreau, M. D. Paolis and I. Chataigner, Dearomatization of 3-Nitroindoles with Highly γ-Functionalized Allenoates in Formal (3+2) Cycloadditions, Chem.–Eur. J., 2019, 25, 1–7 CrossRef PubMed;
(e) B. Biolatto, M. Kneeteman and P. Mancini, Diels-Alder reactions of N-tosyl-3-nitroindole and dienamides: synthesis of intermediates of Aspidospermine alkaloids, Tetrahedron Lett., 1999, 40, 3343–3346 CrossRef CAS;
(f) Q. Wan, J.-H. Xie, C. Zheng, Y.-F. Yuan and S.-L. You, Silver-Catalyzed Asymmetric Dearomatization of Electron-Deficient Heteroarenes via Interrupted Barton-Zard Reaction, Angew. Chem., Int. Ed., 2021, 60, 19730–19734 CrossRef CAS PubMed.
-
(a) W. Al-Zereini, I. Schuhmann, H. Laatsch, E. Helmke and H. Anke, New Aromatic Nitro Compounds from Salegentibacter sp. T436, an Arctic Sea Ice Bacterium: Taxonomy, Fermentation, Isolation and Biological Activities, J. Antibiot., 2007, 60, 301–308 CrossRef CAS PubMed;
(b) J. Tang and H. Wang, Indole derivatives as efflux pump inhibitors for TolC protein in a clinical drug-resistant Escherichia coli isolated from a pig farm, Int. J. Antimicrob. Agents, 2008, 31, 497–498 CrossRef CAS PubMed;
(c) N. K. Kaushik, N. Kaushik, P. Attri, N. Kumar, C. H. Kim, A. K. Verma and E. H. Choi, Biomedical Importance of Indoles, Molecules, 2013, 18, 6620–6662 CrossRef CAS PubMed;
(d) R. Romagnoli, F. Prencipe, P. Oliva, M. K. Salvador, A. Brancale, S. Ferla, E. Hamel, G. Violad, R. Bortolozzi, L. Persoons, J. Balzarini, S. Liekens and D. Schols, Design, Synthesis and Biological Evaluation of 2-Alkoxycarbonyl-3- Anilinoindoles as a New Class of Potent Inhibitors of Tubulin Polymerization, Bioorg. Chem., 2020, 97, 103665 CrossRef CAS PubMed.
-
(a) S. Roy and G. W. Gribble, Convenient Synthesis of Masked Aminoindoles by Indium Mediated Ont-Pot Reductive Acylation of 3- and 2-Nitroindoles, Heterocycles, 2006, 70, 51–56 CrossRef CAS PubMed;
(b) A. A.-H. Abdel-Rahman, W. A. El-Sayed, H. M. Abdel-Bary, A. E.-S. Abdel-Megied and E. M. I. Morcy, Amino acid derivatives, VIII [1]: synthesis and antimicrobial evaluation of α-amino acid esters bearing an indole side chain, Monatsh. Chem., 2008, 139, 1095–1101 CrossRef CAS;
(c) E. T. Pelkey and G. W. Gribble, Synthesis and Reactions of N-Protected 3-Nitroindoles, Synthesis, 1999, 7, 1117–1122 CrossRef.
-
(a) D. Tu, J. Luo and C. Jiang, Copper-mediated domino C-H iodination and nitration of indoles, Chem. Commun., 2018, 54, 2514–2517 RSC;
(b) J.-P. Zou, A. Shoberu, C.-K. Li, Z.-K. Tao and G.-Y. Zhang, NaNO2/K2S2O8-mediated Selective Radical Nitration/Nitrosation of Indoles: Efficient Approach to 3-Nitro- and 3-Nitrosoindoles, Adv. Synth. Catal., 2019, 361, 2255–2261 CrossRef.
- A. C. Lindsay, P. A. Kilmartin and J. Sperry, Synthesis of 3-nitroindoles by sequential paired electrolysis, Org. Biomol. Chem., 2021, 19, 7903–7913 RSC.
-
(a) G. A. Chesnokov, A. A. Ageshina, A. V. Maryanova, S. A. Rzhevskiy, P. S. Gribanov, M. A. Topchiy, M. S. Nechaev and A. F. Asachenko, Nitromethane as a reagent for the synthesis of 3-nitroindoles from 2-haloarylamine derivatives, Russ. Chem. Bull., 2020, 69, 2370–2377 CrossRef CAS;
(b) A. Shoberu, C.-K. Li, H.-F. Qian and J.-P. Zou, Copper-catalyzed, N-auxiliary group-controlled switchable transannulation/nitration initiated by nitro radicals: selective synthesis of pyridoquinazolones and 3-nitroindoles, Org. Chem. Front., 2021, 8, 5821–5830 RSC.
-
(a) H. Zollinger, Color Chemistry, Wiley-VCH, New York, 1987 Search PubMed;
(b) G. Booth, Dyes and Pigments, in Ullmann's Encyclopedia of Industrial Chemistry, Wiley-VCH, Weinheim, Germany, 2000 Search PubMed.
-
(a) S. Patterson and S. Wyllie, Nitro Drugs for the Treatment of Trypanosomatid Diseases: Past, Present, and Future Prospects, Trends Parasitol., 2014, 30, 289–298 CrossRef CAS PubMed;
(b) M. Belciug and V. S. Ananthanarayanan, Interaction of Calcium Channel Antagonists with Calcium: Structural Studies on Nicardipine and its Ca2+ Complex, J. Med. Chem., 1994, 37, 4392–4399 CrossRef CAS PubMed;
(c) J. A. Squella, S. Bollo and L. J. Nunez-Vergara, Recent Developments in the Electrochemistry of Some Nitro Compounds of Biological Significance, Curr. Org. Chem., 2005, 9, 565–581 CrossRef CAS;
(d) K.-S. Ju and R. E. Parales, Nitroaromatic Compounds, from Synthesis to Biodegradation, Microbiol. Mol. Biol. Rev., 2010, 74, 250–272 CrossRef CAS PubMed;
(e) K. Nepali, H.-Y. Lee and J.-P. Liou, Nitro-group-containing Drugs, J. Med. Chem., 2019, 62, 2851–2893 CrossRef CAS PubMed;
(f) W. E. Müller, The Benzodiazepine Receptor, Cambridge University Press, New York, 1988 Search PubMed.
-
(a) A. A. Kulkarni, Continuous Flow Nitration in Miniaturized Devices, Beilstein J. Org. Chem., 2014, 10, 405–424 CrossRef PubMed;
(b) F.-R. Fan, Y. Yao, L. Cai, L. Cheng, J. M. Tour and A. J. Bard, Structure-dependent Charge Transport and Storage in Self-assembled Monolayers of Compounds of Interest in Molecular Electronics: Effects of Tip Material, Headgroup, and Surface Concentration, J. Am. Chem. Soc., 2004, 126, 4035–4042 CrossRef CAS PubMed.
- N. Ono, The Nitro Group in Organic Synthesis, John Wiley & Sons, Weinheim, Germany, 2001 Search PubMed.
-
(a) Y. Nieves-Quinones and D. A. Singleton, Dynamics and the Regiochemistry of Nitration of Toluene, J. Am. Chem. Soc., 2016, 138, 15167–15176 CrossRef CAS PubMed;
(b) E. S. Halberstadt, E. D. Hughes and C. K. Ingold, Kinetics of Aromatic Nitration: The Nitracidium Ion, Nature, 1946, 158, 514–515 CrossRef CAS;
(c) A. Topchiev, Nitration of Hydrocarbons and Other Organic Compounds, Elsevier, 1959 Search PubMed;
(d) K. Schofield, Aromatic Nitrations, Cambridge University Press, Cambridge, U.K., 1980 Search PubMed;
(e) G. A. Olah, R. Malhorta and S. C. Narang, Nitration: Methods and Mechanisms, Wiley-VCH, New York, 1989 Search PubMed;
(f) G. A. Olah, S. C. Narang, J. A. Olah and K. Lammertsma, Recent Aspects of Nitration: New Preparative Methods and Mechanistic Studies, Proc. Natl. Acad. Sci. U. S. A., 1982, 79, 4487–4494 CrossRef CAS;
(g) B. Galabov, G. Koleva, S. Simova, B. Hadjieva, H. F. Schaefer III and P. v. R. Schleyer, Arenium Ions are not Obligatory Intermediates in Electrophilic Aromatic Substitution, Proc. Natl. Acad. Sci. U. S. A., 2014, 111, 10067–10072 CrossRef CAS PubMed;
(h) G. Koleva, B. Galabov, B. Hadjieva, H. F. Schaefer and P. v. R. Schleyer, An Experimentally Established Key Intermediate in Benzene Nitration with Mixed Acid, Angew. Chem., Int. Ed., 2015, 54, 14123–14127 CrossRef CAS PubMed;
(i) B. Galabov, D. Nalbantova, P. v. R. Schleyer and H. F. Schaefer, Electrophilic Aromatic Substitution: New Insights Into an Old Class of Reactions, Acc. Chem. Res., 2016, 49, 1191–1199 CrossRef CAS PubMed;
(j) E. D. Hughes, C. K. Ingold and R. I. Reed, Kinetics of Aromatic Nitration : The Nitronium Ion, Nature, 1946, 158, 448–449 CrossRef CAS PubMed.
-
(a) D. Koley, O. C. Colón and S. N. Savinov, Chemoselective Nitration of Phenols with tert-Butyl Nitrite in Solution and on Solid Support, Org. Lett., 2009, 11, 4172–4175 CrossRef CAS PubMed;
(b) P. Chaudhary, S. Gupta, N. Muniyappan, S. Sabiah and J. Kandasamy, Regioselective nitration of N-alkyl anilines using tert-butyl nitrite under mild condition, J. Org. Chem., 2019, 84, 104–119 CrossRef CAS PubMed;
(c) K. Tabatabaian, M. Mamagkhani and T. Navai-Dyva, Synthesis of a New a α-Methylene-γ-butyrolactone Skeleton with the Use of Cobaloxime as Catalyst, Russ. J. Org. Chem., 2002, 38, 210–212 CrossRef CAS;
(d) G. V. M. Sharma and T. Gopinath, A radical mediated first total synthesis from diacetone glucose and determination of the absolute stereochemistry of xylobovide, Tetrahedron Lett., 2005, 46, 1307–1309 CrossRef CAS;
(e) M. Paira, B. Banerjee, S. Jana, S. K. Mandal and S. C. Roy, Titanocene(III) chloride mediated radical-induced one-pot synthesis of α-methylene-γ-butyrolactones, Tetrahedron Lett., 2007, 48, 3205–3207 CrossRef CAS;
(f) T.-S. Zhang, L. Yang, P.-J. Cai, S.-J. Tu and B. Jiang, Silver-Catalyzed Nitration/Annulation of α-Alkynyl Arylols toward 3-Nitrated Benzofurans, Chem.–Asian J., 2019, 14, 4383–4388 CrossRef CAS PubMed;
(g) D. Xia, L.-Y. Shen, Y. Zhang and W.-C. Yang, Radical spirocyclization of biaryl ynones for the construction of NO2-containing spiro[5.5]trienones, New J. Chem., 2022, 46, 20061–20064 RSC;
(h) W.-C. Yang, L.-Y. Shen, J.-N. Li, J.-G. Feng and P. Li, Oxidative Cyclization of Aryl Ynones with NaNO2 for the Divergent Synthesis of NO2-Containing Spiro[5.5]trienones, Indenones and Thioflavones, Adv. Synth. Catal., 2022, 364, 3651–3656 CrossRef CAS.
-
(a) B. P. Fors and S. L. Buchwald, Pd-Catalyzed Conversion of Aryl Chlorides, Triflates, and Nonaflates to Nitroaromatics, J. Am. Chem. Soc., 2009, 131, 12898–12899 CrossRef CAS PubMed;
(b) G. K. S. Prakash and T. Mathew, Ipso-Nitration of Arenes, Angew. Chem., Int. Ed., 2010, 49, 1726–1728 CrossRef CAS PubMed;
(c) S. Saito and Y. Koizumi, Copper-Catalyzed Coupling of Aryl Halides and Nitrite Salts: A Mild Ulmann-type Synthesis of Aromatic Nitro Compounds, Tetrahedron Lett., 2005, 46, 4715–4717 CrossRef CAS;
(d) G. Yan, L. Zhang and J. Yu, Copper-Catalyzed Nitration of Arylboronic Acids with Nitrate Salts Under Mild Conditions: An Efficient Synthesis of Nitroaromatics, Lett. Org. Chem., 2012, 9, 133–137 CrossRef CAS;
(e) L.-R. Song, Z. Fan and A. Zhang, Recent Advances in Transition Metal-Catalyzed C(sp2)-H Nitration, Org. Biomol. Chem., 2019, 17, 1351–1361 RSC;
(f) T. Yang, X. Li, S. Deng, X. Qi, H. Cong, H.-G. Cheng, L. Shi, Q. Zhou and L. Zhuang, From N–H Nitration
to Controllable Aromatic Mononitration and Dinitration-The Discovery of a Versatile and Powerful N-Nitropyrazole Nitrating Reagent, JACS Au, 2022, 2, 2152–2161 CrossRef CAS PubMed.
-
(a) J.-P. Zhao, L.-j. Ding, P.-C. Wang, Y. Liu, M.-J. Huang, X.-L. Zhou and M. Lu, Electrochemical Nonacidic N-Nitrosation/N-Nitration of Secondary Amines through a Biradical Coupling Reaction, Adv. Synth. Catal., 2020, 362, 5036–5043 CrossRef CAS;
(b) Y. Wang, S. You, M. Ruan, F. Wang, C. Ma, C. Lu, G. Yang, Z. Chen and M. Gao, The Use of Potassium/Sodium Nitrite as a Nitrosating Agent in the Electrooxidative N-Nitrosation of Secondary Amines, Eur. J. Org Chem., 2021, 22, 3289–3293 CrossRef;
(c) S. P. Blum, C. Nickel, L. Schäffer, T. Karakaya and S. R. Waldvogel, Electrochemical Nitration with Nitrite, ChemSusChem, 2021, 14, 4936–4940 CrossRef CAS PubMed.
- S. M. S. Chauhan, A. Kumar and K. A. Srinivas, β-Polynitration of 5,10,15,20-tetrakis(20,60-Dichlorophenyl)porphyrins with HNO3 and Cu(NO3)2 on Clay Using Microwave Irradiation, Synth. Commun., 2004, 34, 2673–2680 CrossRef CAS.
-
(a) K. Zhang, A. Budinská, A. Passera and D. Katayev, N-Nitroheterocycles: Bench-Stable Organic Reagents for Catalytic Ipso-Nitration of Aryl- and Heteroarylboronic Acids, Org. Lett., 2020, 22, 2714–2719 CrossRef CAS PubMed;
(b) S. Salzbrunn, J. Simon, G. K. S. Prakash, N. A. Petasis and G. A. Olah, Regioselective Nitration of Arylboronic Acids, Synlett, 2000, 10, 1485–1487 Search PubMed;
(c) L. Lu, H. Liu and R. Hua, HNO3/HFIP: A Nitrating System for Arenes with Direct Observation of π-Complex Intermediates, Org. Lett., 2018, 20, 3197–3201 CrossRef CAS PubMed.
- G. Aridoss and K. K. Laali, EthylammoniumNitrate(EAN)/Tf2 O and EAN/TFAA:Ionic Liquid Based Systems for Aromatic Nitration, J. Org. Chem., 2011, 76, 8088–8094 CrossRef CAS PubMed.
-
(a) W. Zi, Z. Zuo and D. Ma, Intramolecular Dearomative Oxidative Coupling of Indoles: A Unified Strategy for the Total Synthesis of Indoline Alkaloids, Acc. Chem. Res., 2015, 48, 702–711 CrossRef CAS PubMed;
(b) K. Higuchi and T. Kawasaki, Simple indole alkaloids and those with a nonrearranged monoterpenoid unit, Nat. Prod. Rep., 2007, 24, 843–868 RSC;
(c) H. J. Knölker and K. R. Reddy, Isolation and Synthesis of Biologically Active Carbazole Alkaloids, Chem. Rev., 2002, 102, 4303–4427 CrossRef PubMed.
Footnote |
† Electronic supplementary information (ESI) available: Experimental procedures, spectroscopic data for all compounds. CCDC 2251492. For ESI and crystallographic data in CIF or other electronic format see DOI: https://doi.org/10.1039/d3ra03193d |
|
This journal is © The Royal Society of Chemistry 2023 |
Click here to see how this site uses Cookies. View our privacy policy here.