DOI:
10.1039/D3RA03369D
(Review Article)
RSC Adv., 2023,
13, 23087-23121
Recent developments in natural biopolymer based drug delivery systems
Received
20th May 2023
, Accepted 24th July 2023
First published on 31st July 2023
Abstract
Targeted delivery of drug molecules to diseased sites is a great challenge in pharmaceutical and biomedical sciences. Fabrication of drug delivery systems (DDS) to target and/or diagnose sick cells is an effective means to achieve good therapeutic results along with a minimal toxicological impact on healthy cells. Biopolymers are becoming an important class of materials owing to their biodegradability, good compatibility, non-toxicity, non-immunogenicity, and long blood circulation time and high drug loading ratio for both macros as well as micro-sized drug molecules. This review summarizes the recent trends in biopolymer-based DDS, forecasting their broad future clinical applications. Cellulose chitosan, starch, silk fibroins, collagen, albumin, gelatin, alginate, agar, proteins and peptides have shown potential applications in DDS. A range of synthetic techniques have been reported to design the DDS and are discussed in the current study which is being successfully employed in ocular, dental, transdermal and intranasal delivery systems. Different formulations of DDS are also overviewed in this review article along with synthesis techniques employed for designing the DDS. The possibility of these biopolymer applications points to a new route for creating unique DDS with enhanced therapeutic qualities for scaling up creative formulations up to the clinical level.
1 Introduction
Biopolymers are diverse and remarkably versatile class compounds derived from biological systems or synthesized from biological sources. Like other polymers, biopolymers are composed of similar repeating units (monomers) which are linked together.1 Owing to the peculiar properties of biopolymers e.g. biodegradability, availability, and possibility of engineering the physicochemical characteristics, they are being engaged in innovative formulations. Particularly, while moving towards a green sustainable life, biopolymers offer a platform that fits into the paradigm of achieving an eco-friendly environment. Recently biopolymers have received special attention for designing and fabricating DDS (DDS).2 DDS is a tool to incorporate therapeutic agents to ensure the availability of a highly specific drug to target the diseased site with minimum side effects in the body.3 An ideal DDS can target as well as control release of the loaded drug. Drug delivery carriers act as a vehicle to protect the drugs from decomposition during transportation in the body before targeting the diseased site. DDS is intended to reduce side effects by virtue of being biocompatible and biodegradable. In order to provide the intended pharmacological response, it also modifies drug release at the target site. Both natural as well as synthetic polymers are recognized as potential candidate materials suitable for exploitation in designing the DDS.
Although natural polymers have shown remarkable contributions in developing the DDS but blending and functionalization of polymers through different physical and chemical means transfers into a state-of-the-art class of materials Natural polymers having though good biocompatibility and biodegradability but their low mechanical and thermal properties as well as low solubilities restrict their applications.4 Blending as well as functionalization of polymer to fabricate innovative materials with resultant properties reflecting the parent compounds, that are not exhibited by individual ones. Functionalization of biopolymers through blends/composites by forming hybrid structures is an approach, widely used in DDS to launch the combined roles in the resultant hybrid system.3,4 By reducing the negative effects, the beneficial properties of each biopolymer are enhanced, which improves the effectiveness of the created DDS.5
Polymer–polymer or filler–polymer combinations may be used to create polymeric nano-biocomposites. Metal nanoparticles (NPs), hydroxyapatite, organic or inorganic clays, and other materials may be used as fillers. The highest amount of drugs can be loaded into a nano-composite system while using the smallest possible amounts of carrier. Drug loading is typically done via an impregnation or inclusion approach. While the integration approach includes drug trapping by nano-composites at the time of manufacture, the impregnation involves drug entrapment by typically incubating the nano-composites from a solution.6
In DDS, polymer–drug complexes are formed usually via hydrophobic interactions, van der Waals forces, hydrogen bonding and electrostatic attractions between opposite charges of the biopolymers. To get mechanical strength, aggregates are sometimes cross-linked with suitable linker(s) to enhance stability and integrity. A cavity-bearing supra-molecular aggregation is often necessary for the inclusion complexation procedure in order to serve as a host for an entering guest molecule (s). Nanoprecipitation, another worthwhile method is generally adopted for hydrophobic polymers. In the supercritical fluid method, another precipitation technique, involves the liquid or gas, and polymer(s)/drug(s) are solubilized together above their supercritical points. The selection of the preparative method is determined by a number of factors, such as the thermal and chemical stability of the bioactive components, the toxicity of the leftover chemicals after processing, particle sizes, release kinetic profiles, and finally the kind of delivery system. Two separate drying techniques are often used: freeze-drying and spray-drying. For freeze-drying, heat-sensitive materials have been selected, while for spray-drying, the nanoparticle solution is introduced into a stream of hot air, causing the solvent to quickly evaporate and the dried particles to aggregate.7
This review is also aimed to summarize the contribution of different natural biopolymers, particularly sugar-based polymers, amino-sugars and polynucleotide-based polymers. Fig. 1 gives the classification of different natural polymers which have been employed to design the DDS. Although almost all polymers have a prestigious role in DDS still their derivatives, functionalized composites are also in clinical trials in different formulations for designing the DDS. These encouraging biopolymer applications provide us a new route for creating unique DDS with enhanced therapeutic qualities for scaling up creative formulations to the clinical level.
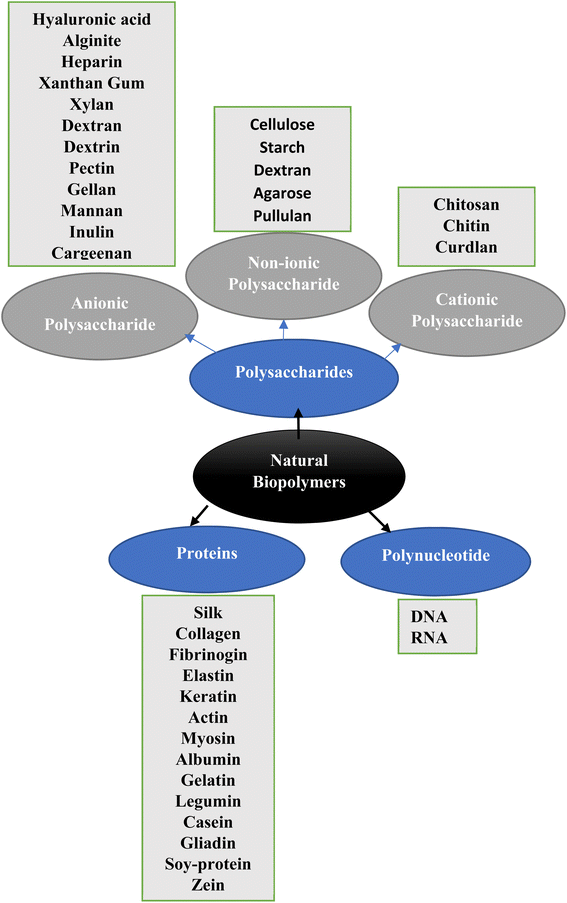 |
| Fig. 1 Classification of different polysaccharide, protein and nucleotide-based biopolymers. | |
2 Types of DDS
To improve the solubility of the pharmaceuticals for stable complex formation and their safety during delivery at the target location, many formulations of individual biopolymers and their composites have been described, including powder, tablets, beads, films, fibres, meshes, membranes, and hydrogels.4,8
2.1 Microspheres based DDS
The microsphere-based delivery method is often selected because of its long lifespan, control over drug release, and ability to distribute just certain types of medications. The interaction with counter ions, solvent evaporation, crosslinking, spray drying, ionic gelation, precipitation/coacervation, emulsion polymerization, and other processes may all be used to create microspheres17–20 Glutaraldehyde cross-linked microspheres by using mitoxantrone are also reported.9,10
2.2 Tablets/capsules based DDS
DDS based on tablets or capsules is often created using the wet granulation method or just direct compression. Diltiazem's release behaviour from oral mucosal adhesive tablets manufactured with the direct compression method and a matrix of chitosan and alginate was evaluated, and it showed a noteworthy response. The chitosan–sodium alginate matrix system exhibited comparable characteristics.11 Another study examined how different combinations of anionic polymers affected the release rate of chitosan.12
2.3 NPs based DDS
NPs are very effective in transferring macromolecules across the nasal, oral, tracheal, and ocular epithelium and improving pharmaceutical absorption via the nasal mucosa.13 For the production of biopolymer-based NPs, a number of techniques, including emulsion, nanoprecipitation coacervation, ionic gelation, reverse micellar approach, and sieving method, have been described.14,15 As the tumor-targeted carriers for the dextran–doxorubicin combination, chitosan nanoparticles (NPs).16 Similarly, it has been shown that chitosan nanoparticles can encapsulate DOX and N-trifluoroacetyl DOX.17 Significant anticancer activity of a photosensitizer meso-tetra (N-methyl-4-pyridyl) porphine tetra tosylated and encapsulated in antibody-targeted chitosan–alginate nanoparticles.17 Chitosan NPs are also reported as suitable stable delivery devices for siRNA and protein.18 Entrapment of DOX in chitosan NPs is also reported.19 Chitosan NPs loaded with paclitaxel illustrated superb tumor-homing.20 The antiviral behavior of interferon-alpha via orally administered chitosan NPs is also evaluated.21
2.4 Nanofibers based DDS
To improve the properties like hydrophobicity, solubility, biological activity, biocompatibility etc. for widening their applications, chemical modification of biopolymers is an effectual tool. Another technique to increase the potential of biopolymer nanofibers for drug delivery applications is surface functionalization.22 For the announcement of controlled drugs, nanofibrous chitosan-polyethylene oxide was developed.23 Electrospun membranes with ibuprofen-loaded poly(lactide-co-glycolide)/poly(ethylene glycol)-g-chitosan have been used in controlled drug delivery applications.24 Quaternary electrospun polymers containing DOX showed enhanced cytotoxicity against the graffiti tumor cells.25 Chitosan and phospholipids hybrid nanofiber has been employed for transdermal drug delivery.26
2.5 Beads-based DDS
Crosslinked beads are an important form of biopolymers exploited broadly in delivery systems.27 The controlled release of diclofenac sodium from glutaraldehyde crosslinked polymeric beads were also evaluated.28 Multi-layered alginate and chitosan beads directed controlled gastrointestinal passage of ampicillin, which is a low molecular weight compound.29
2.6 Films-based DDS
Biopolymeric thin films find numerous applications in DDS. Mostly, casting methods are preferred to deposit thin films. In comparison to their parent material, hybrid materials have notably better characteristics. Drug delivery systems using biopolymeric crosslinked films have been researched in a number of applications, such as oral mucosal delivery,30 buccal delivery,31 transdermal delivery,32 sublingual delivery,33 and periodontal delivery.34 For oral mucosal administration, super critical solution impregnation technique films filled with ibuprofen have been studied.30
2.7 Hydrogels-based DDS
Three-dimensional crosslinked polymeric networks called hydrogels may absorb a lot of water without dissolving.35 Through implantation, the biocompatibility of crosslinked biopolymeric hydrogels is assessed.36 Swell behaviour and delivery in a pH-dependent manner The development of sensitive alginate–chitosan hydrogel beads loaded with nifedipine is also being studied.37 For the purpose of promoting wound healing, photosensitive cationic NPs based hydrogels of hyaluronic acid and chitosan, with chlorin e6 and quaternary ammonium salt, were described.38 Gallic acid conjugated with chitosan hydrogel beads are reported to be employed for the loading of rhodamine B.39 The mechanical characteristics of the treated cotton gauze were evaluated on drug-loaded silica during an ex vivo drug penetration research via isolated rat skin, and bio polysaccharides-based hydrogels were studied using the culture count technique.40 The injectable administration of the anticancer medication doxycycline hydrochloride has been reported to use Schiff base alginate–chitosan hydrogels with nanosilver incorporated in them.41 Epigallocatechin gallate has been found to be transported using lanthanum-modified chitosan hydrogel.42 5-Fluorouracil is delivered using chitosan/agarose/graphene oxide nanohydrogel in the treatment of breast cancer.43 Melaninin incorporated polysaccharide hydrogels of chitosan and oxidized β-glucanis has been reported for treating the bacterially infected diabetic wounds.44 Carboxymethyl cellulose based hydrogels have been reported for colon-specific delivery of gentamicin. For the administration of ciprofloxacin, composite hydrogels with ZnO embedded in polyethylene glycol diacrylate and cross-linked carboxymethyl tamarind kernel gum have been reported.45 Gelatin/lignin hydrogels have been utilized drug carriers for ribavirin.46 Three dimensional chitosan and carboxymethyl cellulose-based hydrogels, loaded with nano-curcumin for synergistic diabetic wounds have been very well reported.47 Polyacrylic acid-carboxymethyl cellulose hydrogel incorporating halloysite nanotubes have been reported for curcumin delivery release.48 Natural gums and their derivatives based hydrogels have also drug delivery potential.49
2.8 Conjugates-based DDS
It has been claimed that the development of nano-conjugates allows for both passive and active delivery of medicinal substances to the desired region. Using DOX hydrochloride, foliate-chitosan conjugated NPs improved tumour target selectivity without causing any harm.50 Polymers by functionalization with graphene oxide were employed as nanocarriers for camptothecin.51 Multi-walled carbon nano tubes have also been employed for the functionalization of biopolymers and showed good biocompatibility against HeLa cells and protein immobilization.52 Biopolymeric–biopolymeric functionalization i.e. alginate with chitosan is a combination reported with enhanced behavior.8
3 Formulations of biopolymers and their composites
Currently, natural polymers are revealing remarkable contributions in developing the DDS through physical and chemical means by blending and functionalization of polymers in different forms. In this review article, designs of different DDS made from natural biopolymer building blocks at nano and micro scale levels are tried explored and discussed individually. Fig. 1 classifies the natural biopolymers into different classes.
3.1 Polysaccharides based DDS
Polysaccharides are monosaccharides units attached by glycosidic linkages. They have properties i.e. bioactivity, biodegradability and processability, which make them promising biomaterials for developing the DDS under complex biological environments. Especially recent developments by using polysaccharides-derived functional biomaterials.53 The role of different cationic, anionic and neutral polysaccharides, their composites and derivatives for DDS is discussed.
3.1.1 Cellulose. The most common non-ionic polysaccharide that is found naturally is cellulose. It has also been used to release repaglinide orally using cellulose and chitosan nanoparticles (NPs).54 Specifically developed to target colon diseased sites, calcium alginate beads with carboxymethylcellulose loaded with 5-fluoroalkyl.55 These four cellulose derivatives—methylcellulose, sodium carboxymethylcellulose, hydroxypropyl methylcellulose, and cationic hydroxyethyl cellulose—have been used as DDS in the nasal mucosa.56 The impact of hydroxy propyl methylcellulose-based nanocomposites with cellulose nanofibrils for drug release in the form of thin films was explored.57 As magnetic-responsive drug carriers for in vitro anti-colon cancer treatment, Fe3O4 loaded at cellulose containing curcumin has been used.58 For use in in vitro ciprofloxacin drug release, cellulose–polyacrylamide hydrogel nanocomposite with gold nanoparticles has been developed.59 Table 1 lists some other instances of the use of cellulose and its mixtures for medication delivery.
Table 1 Cellulose and its composites in drug delivery
Polymer |
Bioactive agent |
References |
Carboxymethyl cellulose |
DOX |
60 |
Cellulose |
Betulinic acid |
61 |
Microcrystalline cellulose |
Luteolin and luteoloside |
62 |
Carboxymethyl cellulose |
5-Fluorouracil |
63 |
Carboxymethyl cellulose |
DOX |
64 |
Passion fruit peel cellulose |
Tetracycline |
65 |
Hydroxy ethyl cellulose |
DOX |
66 |
Cellulose |
Felodipine |
67 |
Ethylcellulose |
Multilayer layer coatings that allow for instant or customised release |
68 |
Hydroxypropyl methylcellulose acetate succinate |
For creating capsules and coating layers for instantaneous or regulated release |
68 |
Hydroxypropyl methylcellulose |
The release of nitrofurantoin might be impacted by the HPMC fraction of 0–40% |
69 |
Hydroxypropyl methylcellulose |
For printing capsules and coating layers for immediate or modified release, barrier material |
68 |
Hydroxypropylcellulose |
Design of the capsule and pulsatile drug release |
70 |
Hydroxypropylcellulose |
Serving as a polymer carrier for theophylline release |
71 |
As a carrier polymer for intragastric domperidone release |
72 |
Poly(1-O-methacryloyl-β-dfructopyranose)-block-poly(methyl methacylate) |
Paclitexal |
73 |
Graphene oxide in bacterial cellulose |
Nanocarrier of ibuprofen |
74 |
3.1.2 Chitosan. The most prevalent naturally occurring cationic amino polysaccharide after cellulose is chitosan. Chitosan was discovered and discussed by Rouget in 1859 for the first time and entered the pharmaceuticals field in 1990 owing to the versatility of its active amino groups.8 Chitosan, a cationic polysaccharide derivative of chitin has been employed in different routes of administration including nasal, ocular, intravenous, oral, mucosal, etc. The nasal absorption of peptide medicines as an enhancer is one of its many biological uses that merits highlighting. Peptide pharmaceuticals are often employed as an adjuvant in immunotherapy, a carrier for siRNA/DNA and gene therapy, as anticancer drugs, and as a scaffold in the healing of wounds. Functionalization is a viable method to accomplish unattainable therapeutic objectives in order to fully realise the promise of nanomedicines.75 Chitosan along with amaranth red and microencapsulation with alginate released the intestinal and gastric fluids for the protection of molecules, after oral administration for intestinal release.76,77 It has also been claimed that chitosan–alginate nanocomposites improve the delivery of daptomycin to the ocular epithelium for antibacterial activities.78 Chitosan that has been functionalized to release catechol has been tried as a buccal medication delivery method for lidocaine.79Chitosan nanoparticles have been discovered to significantly increase medication absorption through nasal mucosa and to transfer macromolecules across the nasal, ocular epithelium oral and tracheal.13 Chitosan NPs that were paclitaxel-loaded demonstrated excellent tumor-homing.20 Interferon-alpha administered by chitosan nanoparticles' antiviral efficacy is also evaluated.21 In the quest to search the thermosensitive and mucoadhesive biopolymers, moxifloxacin-loaded sustained release periodontal showed that poloxamer-and chitosan-based formulations sustained the drug release for 8 h with low initial burst release.80 Chitosan microspheres embedded with selenium NPs are reported to express gastroprotective potential.81 Amoxicillin is degraded by the acidic pH of the stomach, and was encapsulated in a biopolymer functionalized with lipids.82 Fe3O4/chitosan nanocomposite has been employed for the intravenous supply of gemcitabine (an anticancer nucleoside analog).83 Chitosan-encapsulated mesoporous Fe3O4/SiO2 nanocomposite is tested and shown to be adequate for the controlled release of DOX.84
A composite made of polyethylene glycol, chitosan, and iron oxide that also contains cyanin dye, a near-infrared fluorescent, and has paramagnetic, targeting, fluorescent, and anticancer properties is described for self-targeted curative drug delivery.85 It is also claimed that chitosan plus zinc oxide make an excellent medication delivery system.86 Iron oxide and cadmium telluride functionalized on zinc sulfide quantum dots with carboxymethyl chitosan have been employed for cell labeling and drug release.64,87,88 5-Fluorouracil encapsulated carboxymethyl chitosan for colon cancer therapy.89 Chitosan, cyclodextrin, and carboxymethyl chitosan were combined to create pH-sensitive magnetic hydrogels for the controlled release of the medication.90 The effect of the incorporation of Fe3O4 NPs was also explored on carboxymethyl chitosan, cyclodextrin, and chitosan hydrogel to deliver methotrexate.91 pH-sensitive chitosan and carboxymethyl chitosan biopolymers have also been used for colon-targeting medication delivery.92 Using carboxymethyl chitosan, cyclodextrin, and chitosan, Fe2O3 hydrogels sensitive to pH were created, and they have been employed for the controlled release of medications.90 The effect of the incorporation of iron oxide NPs was also explored on carboxymethyl chitosan, cyclodextrin, and chitosan hydrogel to deliver methotrexate.91 pH-sensitive chitosan and carboxymethyl chitosan biopolymers for colon-targeting drug delivery have also been reported.92
Chitosan–chondroitin sulfate is being used for transporting the lornoxicam as a gastroretentive delivery system.123 Hollow and bio-adhesive microspheres composed of ethyl cellulose and glyceryl monooleate have been fabricated and had proved to extend the drug retention time in the stomach.125 For an 8 hour continuous release of loratadine, oil-entrapped floating microbeads were developed as a gastro retentive controlled release device. Chitosan derivative with polyaniline side chain for effective suppression of tumor growth is also reported.93 An injectable succinate chitosan and oxidized alginate for in vitro release of DOX for inhibition of tumor growth breast cancer have been formulated.94 In order to transport the antibiotic ceftazidime to the eye, the effectiveness of hydroxypropyl methylcellulose containing chitosan, sodium tripolyphosphate, and hyaluronic acid NPs is assessed.95 For the oral delivery of medications, chitosan, alginate, and pectin NPs have shown promise.96 It is widely known that carboxymethyl chitosan may release intra-nasal carbamazepine by evading the blood–brain barrier membrane.97 Through γ-ray irradiation polymerization, poly(butyl acrylate) modified chitosan-organophilic nanocomposite has been devised for DDS.98 Through folate, conjugation of doped carboxymethyl chitosan-ferro-ferric oxide with cadmium telluride quantum dots a DDS has been synthesized.99 Hybrid polysaccharides composites with crosslinked chitosan with carboxymethyl-β-cyclodextrin grafted on Fe3O4 have been reported for the transportation of 5-fluorouracil.100 Using the drug's controlled release in N-(2-hydroxyl) propyl-3-trimethyl ammonium chitosan chloride was similarly successful.101 Alginate nanocomposites with quaternized carboxymethyl chitosan clay are tested for their drug release characteristics.102 When combined with cloisite 30B, chitosan-polyvinyl alcohol effectively administers curcumin release.103 DOX release was observed using electrospun nanofibrous scaffolds made of polyethylene, chitosan, and graphene oxide.104
Chitosan and dextran were tested as carriers for the anticancer medication DOX after being modified with graphene oxide.105 Chitosan–alginate nanoblends with cloisite 30B have been evaluated for the controlled release of curcumin.106 Graphene/gold nanocomposite films for glucose biosensing are also reported. Commercially accessible, water-soluble derivatives of glycol chitosan have been utilised to deliver drugs like paclitaxel and DOX.107 Protonated chitosan with ionized alginate shows prolonged retention of the structures in the intestinal mucosa.108,109 In vitro, study revealed the ability to deliver DNA by folic acid–chitosan conjugates.110 Hyaluronan–cisplatin fabricated the nanoconjugates to target colon cancer.111 A chitosan-based hydrogel containing latanoprost eye drops was discovered in the aqueous humour seven days after the system had been applied topically only once112 and A polymer made of poly (N-isopropylacrylamide) and chitosan was used to administer timolol topically over a 12 hour period.113 Additionally, sustained drug release patterns were shown using carboxymethyl chitosan and a poloxamer made of polyethylene oxide, polypropylene oxide, and polyethylene oxide.114
Several conjugates with mitomycin C, exhibited good in vitro antitumor activities against sarcoma, melanoma, murine leukemias, hepatic cell carcinoma, and metastatic liver cancer.115 DOX–chitosan conjugates showed suppress tumor growth against breast cancer,116 melanoma,117 and mesothelioma cells.118 Paclitaxel-chitosan nanoconjugates, showed appreciable inhibition of murine melanoma when applied for oral administration,.119 For the purpose of developing the DDS, docetaxel–chitosan conjugates also shown desirable features, such as bioavailability, decreased acute toxicity, and in vivo effective anticancer activity.120 Targeted anticancer drug delivery and photothermal treatment have both been achieved using chitosan/sodium alginate functionalized magnetised graphene oxide nanocomposites.121 Silver NPs were physically crosslinked in chitosan to form hydrogel beads for application as DDSs.122 Chitosan supported ciprofloxacin Tween-80/tripolyphosphate along with bovine serum albumin are reported to target the site.123 Hydrogel nanocomposite of Fe3O4 NPs with acrylic acid/N-isopropyl acrylamide and chitosan for controlled release of DOX.124 Chitosan composite with mesoporous aluminosilicate thin films was employed for the delivery of metformin.125 Chitosan NP with Fe2O3 modification was created to regulate the distribution of DOX and cell imaging,88 for simultaneous cancer imaging and therapy using methotrexate and gemcitabine administration.83,126 Chitosan–alginate constructs have also been employed for delivering anticancer,127,128 ocular,129 pulmonary and asthma,130 and anti-inflammatory drugs.131 Chitosan-hyaluronic acid systems were also employed for ocular applications132,133 and for treating asthma and osteoarthritis.134,135 For sustained drug release in the intestine chitosan and xanthan gum-based tablets showed excellent results.136,137
The optimum encapsulation characteristics of benzalkonium chloride inside mesoporous silica/polysaccharide hybrid materials increase the amount of the drug release by improving the dispersion of the MSN and permitting enhanced drug diffusion.138 The prolonged administration of medications to the eye is improved by chitosan and gelatin hydrogels.139 Crosslinked chitosan with embedded Fe3O4 NPs showed good rational drug administration.140 Chitosan microspheres loaded with 5-fluorouracil to DDS were developed in order to understand in vitro cytotoxicity and in vivo efficacy for the treatment of colon cancer.141
Fe3O4 NPs functionalized with 3-amino propyl, triethoxy silane were covered in tragacanth gum and chitosan to create capsules for the medicine curcumin.142 Zinc oxide composites with chitosan have also been used as drug delivery platforms.143 Effective drug carriers for cancer treatment with improved absorption are pH-sensitive fluorinated carboxymethyl chitosan nanoparticles.144 In colorectal cancer treatment efficiency of 5-fluorouracil was seen to be enhanced via nanoencapsulation.145 Table 1 provides some other instances of the use of chitosan and its mixtures in medication administration.
3.1.3 Cellulose. Cellulose is a non-ionic polysaccharide. Cellulose and chitosan NPs are also employed for the oral release of repaglinide is also practiced.54 Targeting colon diseased cites, calcium alginate beads with carboxymethylcellulose loaded with 5-fluoroalkyl.55 These four cellulose derivatives—methylcellulose, sodium carboxymethylcellulose, hydroxypropyl methylcellulose, and cationic hydroxyethyl cellulose—have been used as DDS in the nasal mucosa.56 The impact of hydroxy propyl methylcellulose-based nanocomposites with cellulose nanofibrils for drug release in the form of thin films was explored.57 For in vitro anti-colon cancer treatment, magnetic-responsive drug carriers loaded at cellulose containing curcumin were utilised instead of Fe3O4.58 In vitro drug release of ciprofloxacin with a 96.6 percent success rate over a 5 hour period using gold nanoparticles with cellulose grafted polyacrylamide hydrogel.59 Colon cancer treatment with 5-fluorouracil-encapsulated carboxymethyl chitosan is widely documented.89 Chitosan, cyclodextrin, and carboxymethyl chitosan were combined to create pH-sensitive magnetic hydrogels for the controlled release of the medication.90 In order to distribute methotrexate, the impact of including Fe3O4 NPs on carboxymethyl chitosan, cyclodextrin, and chitosan hydrogel was also investigated.91 pH-sensitive chitosan and carboxymethyl chitosan biopolymers have also been used for colon-targeting medication delivery.92 Table 2 lists some other instances of the use of cellulose and its mixtures for medication delivery.
Table 2 Chitosan and its composites in drug deliver
Polymer |
Bioactive agent |
References |
Polyurethane–alginate/chitosan |
A model antigen |
146 |
Chitosan–alginate |
Silver |
147 |
Crocin |
148 |
Naringenin |
149 |
Quercetin |
150 |
Insulin |
151 |
DOX |
152 |
N-Octyl-N arginine chitosan |
Insulin |
153 |
Alginate–chitosan |
Diclofenac sodium |
154 |
Alginate–chitosan |
Verapamil |
155 |
Alginate–chitosan microparticles |
Prednisolone |
156 |
Chitosan-coated composite-sodium alginate microbeads |
Amoxicillin |
157 |
Blend of chitosan–sodium alginate and cloisite 30b |
Curcumin |
129 |
Chitosan |
5-Fluorouracil, indomethacin |
158 |
Chitosan/N-trimethyl azone |
Testosterone |
159 |
Chitosan eucalyptol, transcutol® P |
Ondansetron hydrochloride |
160 |
Chitosan, rhodamine, polydimethylsiloxane |
Bovine serum albumin |
161 |
Chitosan, SL (SoftCAT™), sodium deoxycholate |
Lidocaine hydrochloride |
162 |
Chitosan, hyaluronic acid, glyceryl monostearate, cetyltrimethylammonium bromide |
Lidocaine |
163 |
Chitosan, hyaluronic acid, glycidyl methacrylate |
Lidocaine |
164 |
Chitosan, b-CD, cineole, menthol, limonene, streptozotocin |
Glimepiride |
165 |
Hydrochloride chitosan, PLGA |
Donepezil |
166 |
Chitosan, oleic acid |
Propranolol hydrochloride |
167 |
Chitosan, N-vinyl caprolactam, azobisisobutyronitrile |
Etoricoxib, paracetamol |
168 |
Chitosan, cholesterol, deoxycorticosterone acetate |
Carvedilol |
169 |
Chitosan, triphenylphosphine |
Aciclovir |
170 |
Chitosan, lipoid S45, lipoid S100 |
Melatonin |
171 |
Chitosan, hydroxypropyl methylcellulose, polyvinyl alcohol, polyamidoamine dendrimer, dibutyl phthalate |
Meloxicam |
172 |
L-a-Phosphatidylcholine, chitosan, cholestrol, triton X 100, dihexadecyl phosphate |
Resveratrol |
173 |
Chitosan oligosaccharide, cellulose nanocrystal, sodium tetraphenylborate |
Procaine hydrochloride |
174 |
Chitosan, azolectin |
Curcumin, diclofenac and vitamin B12 chitosan |
175 |
Chitosan, hydroxypropyl methylcellulose, dibutyl phthalate |
Metformin hydrochloride |
176 |
Chitosan, lactic acid |
Lisinopril |
177 |
Chitosan |
Bovine serum albumin |
178 |
Chitosan, bovine serum albumin, poly(sodium-4-styrene sulfonate) |
Tetanus toxoid |
179 |
Chitosan, mannitol, hydroxypropyl methylcellulose |
Ketoprofen, chondroitin sulfate |
180 |
Chondroitin sulfate |
Methotrexate |
181 |
Chorine6 |
182 |
Glycyl-prednisolone |
183 |
Quercetin |
184 |
Histamine |
185 |
Diacerein |
186 |
Chitosan, p-nitrophenyl chloroformate and amino1-propanol, Pluronic F127 |
Curcumin |
187 |
Chitosan, DMPC, lipiodol, D-glucose (dextrose) |
Indocyanine green |
188 |
Xanthan-graft-C16 alkyl chain |
Glibenclamide |
189 |
Chitin-graft-hexadecyl |
DOX |
190 |
Fucoidan-graft-octenyl succinic anhydride |
Cur |
191 |
3.1.4 Hyaluronic acid. Different forms of cancer, such as breast cancer, lung cancer, and colon cancer, have been treated using a variety of hyaluronic acid (anionic polysaccharide) and its derivatives combined with paclitaxel.109 They are proved prospective carriers for butyric acid to treat Lewis lung, melanoma, and leukaemia and have undergone significant research for the transport of analgesics, siRNA, proteins, antibiotics and anticancer medicines.192 In human ovarian carcinoma xenografts, hyaluronic acid (HA) alone and in conjugation with DOX demonstrated targeted toxicity in vitro and potent anticancer action in vivo193 and bladder carcinoma respectively.194 HA functionalized by adipic dihydrazide or methacrylic anhydride showed an appreciable DD profile.195 Hyaluronic acid coupled with g-poly (N isoorpylacrylamide) has high drug-loading capabilities. Another system for loading cyclosporine A showed results comparable to commercially available DDS.196 Zhong et al. did extensive study on reversible crosslinked hyaluronic acid nanoparticles to address medicine resistance (NPs).197 Crosslinked hyaluronic acid composite hydrogels and nano-carriers have been used to deliver drugs to the eyes.195 The modified hyaluronic macromers were crosslinked with matrix metalloprotease peptides to allow for a sustained release of the growth hormones.198The creation and subsequent loading of an injectable hydrogel based on the chemical bonds between hyaluronic acid and adipic acid dihydrazide and hyaluronic acid aldehyde.Paclitexal comes in micelle and microparticulate forms.199 Results revealed a significant tumor reduction. Cho et al. developed platinum-incorporated HA NPs to inhibit tumor growth.200 Ueda et al. developed an injectable interferon-alpha containing hyaluronic acid-tyramine that was subsequently combined with sorafenib in a kidney cancer xenograft mice model (a tyrosine kinase inhibitor).201 Ferrocenium tetradecyl coated with HA was used for the delivery of the DOX drug.94,202 An enhanced therapeutic effect was shown by a nanocomposite comprised of fluorochrome indocyanine green, carboxyl terminated dendrimer, HA and DOX.203 Iron oxide NPs with dopamine-modified Hyaluronic acid have been reported.204 For lung cancer treatment, an injectable alginate-calcium hydrogel containing dendrimer-encapsulated platinum NPs was used.205 Table 3 lists some further instances of hyaluronic acid and its compounds being used for medication delivery.
Table 3 Drug delivery using hyaluronic acid and its composites
Polymer |
Bioactive agent |
References |
Adipic dihydrazole hyaluronic acid |
Quercetin |
206 |
Hyaluronic acid |
Curcumin |
207 |
Hyaluronic acid |
Curcumin |
208 |
Dithiodipropionic acid hyaluronic acid |
Quercetin curcumin |
209 |
Hyaluronic acid |
Paclitaxel |
210 |
Butyric acid |
211 |
Cisplatin |
212 |
Cisplatin |
213 |
Paclitaxel |
214 |
Paclitaxel |
215 |
Hyaluronic acid ceramide |
DOX |
216 |
Hyaluronic acid-functionalized SLN |
Paclitaxel |
217 |
Hyaluronic acid-nanographene oxide |
HA-conjugate NPs |
218 |
Functionalized hyaluronic acid |
Noisome |
219 |
Hyaluronic acid-functionalized transferases |
DOX |
220 |
Hyaluronic acid-chitosan functionalized |
PCL nanofiber scaffold |
221 |
Hyaluronic acid polymeric micelle |
Coenzyme Q10 |
222 |
Hyaluronic acid conjugate |
Human growth hormone |
223 |
3.1.5 Xylan. Another prevalent cationic polysaccharide biopolymer, xylan is mostly found in plants and grains and is hemicellulose. Due of colonic microflora's ability to generate enzymes that may lead to biodegradation, it is a crucial factor in the development of colonic DDS. Thus, xylan was approved as a biopolymer that is only used in the colon.224 A sort of illness connected to the colon area is colorectal cancer. The activation of the carboxylic acid was employed by Sauraj and his collaborators to create xylan-5-fluorouracil-1-acetic acid conjugates, which they found to be more effective than the medicine when administered alone.225 Additionally, xylan–curcumin conjugates for the therapy of cancer have been described.226 These systems are described for the delivery of peptides and proteins, as well as for the treatment of Chron's disease and ulcerative colitis, in addition to having shown promise for colon medication delivery.227
3.1.6 Alginate/alginic acid. Alginate composites, conjugates, and derivatives with a variety of therapeutically active components have been described and are offered in the market commercially.228 Active compounds, ranging from microscopic drug molecules to macromolecular proteins, may be released from alginate gels in a controlled manner depending on the kind and cross-linking procedure. Their applications in the pharmaceutical sector are expanded by the fact that they may also be administered orally or through injection.228 HT-29 cells were used as the test subject for the in vitro cytotoxicity of capecitabine-loaded interpenetrating polymeric network created by the ionotropic gelation process employing the polymers locust bean gum and sodium alginate. Results showed that cell proliferation has been significantly reduced.229 For the treatment of depression, alginate NPs have been used to release venlafaxine through intranasal delivery.230 Moxifloxacin hydrochloride is effectively delivered over time using sodium alginate.231 Anti-inflammatory medications have also been administered to the eye using sodium alginate hydrogels. Another such very effective formulation is created.232 Román et al. developed alginate microcapsules containing epidermal growth factor linked to its exterior section to precisely target the non-small cell lung cancer cells.233 Additionally put in the NPs was cisplatin. To increase the penetration of daptomycin into the ocular epithelium in an effort to have an antibacterial impact, chitosan-coated alginate NPs were used.78To create drug delivery systems, alginate nanoaggregates of 250 to 850 nm may be coupled with chitosan and glycol chitosan.234 Alginate nano aggregates have been used to deliver DNA, antisense oligonucleotides, insulin, cisplatin, and DOX.107 Using galactosylated chitosan graft dextran, DNA complexes have been delivered to the liver.235 Chitosan/hyaluronic acid microparticles were used to load the DOX hydrochloride drug.236 To deliver 5-fluorouracil for colon cancer therapy and blood drug concentration, a mesoporous silica-alginate/folic acid-conjugated O-carboxymethyl chitosan-gelatin nanocomposite was developed.237 By avoiding the burst release, sodium alginate-ZnO hydrogel beads improved the release of curcumin.237 Films made of calcium alginate loaded with diclofenac sodium and other hydrophilic polymers performed well.238 Ca-alginate composite films are used in clinical therapeutic applications.239 Table 4 lists some other instances of the use of alginate and its mixtures for medication administration.
Table 4 Drug delivery using alginate and its composites
Polymer |
Bioactive agent |
References |
Alginate |
Zidovudine |
240 |
Alginate |
DOX loaded liposomes |
241 |
Sodium-alginate |
Indomethacin |
242 |
Retinoic acid |
243 |
DOX |
244 |
5-Fluorouracil |
245 |
Alginate |
Ibuprofen |
246 |
Sodium-alginate |
Ketoprofen |
247 |
Alginic acid |
DOX |
248 |
Alginate–calcium phosphate NPs |
Glucocerebrosidase |
249 |
Alginate–montmorillonite NPs |
Vitamin B1 and B6 |
250 |
Alginate–magnesium stearate NPs entrapped in oil |
Ibuprofen |
251 |
Alginate gel entrapped in oil- |
Risperidone |
252 |
Alginate tamarind gum magnesium stearate buoyant NPs |
Metronidazole |
253 |
Alginate-calcium silicate effervescent beads |
Alfuzocin HCl |
254 |
Alginate-calcium silicate muco adhesive NPs |
5-Fluorouracil |
255 |
Glass-alginate-samarium composite |
DOX and paclitaxel |
256 |
Alginate-calcium carbonate hybrid NPs |
DOX |
257 |
Alginate-strontium-substituted hydroxyapatite nanocomposites |
Vancomycin |
258 |
Montmorillonitealginate composites |
Diclofenac sodium |
259 |
Composite of calcium alginate and methyl cellulose |
Gliclazide |
260 |
Microbeads made of polyvinylpyrrolidone and calcium alginate |
Diclofenac sodium |
261 |
Alginate-polysaccharide beads made of linseed |
Diclofenac sodium |
262 |
Okra gum with zinc alginate beads |
Diclofenac sodium |
263 |
Mucilage-alginate mucoadhesive beads made from paghula husk |
Gliclazide |
264 |
NPs made of ispaghula husk and alginate |
Glibenclamide |
265 |
Microspheres made of tamarind seed polysaccharide that are mucoadhesive |
Gliclazide |
266 |
Polysaccharide-alginate mucoadhesive beads made with tamarind seeds |
Metformin HCl |
267 |
Microspheres of esterified gellan gum and alginate |
Aceclofenac |
268 |
Lipid/alginate |
Dexamethasone |
269 |
Calcium/alginate |
Hector (Aah), attenuated androctonus australis venom |
270 |
Graphene conjugated sodium alginate |
Carrier of DOX hydrochlorid |
271 |
Carboxymethyl cellulose and sodium alginate protected silver NPs |
Nanomedicine |
272 |
Polyurethane–alginate |
Insulin |
229 |
Poly vinyl alcohol–alginate |
Metformin |
273 |
Lipid/alginate |
Dexamethasone |
269 |
Calcium/alginate |
Attenuated Androctonus australis hector (Aah) venom |
270 |
Alginate |
Curcumin |
274 and 275 |
Galactosylated alginate |
Curcumin |
276 |
Sterculia gum–alginate floating beads with oil entrapment |
Aceclofeanc |
277 |
Mucilage-alginate mucoadhesive beads made from fenugreek seeds |
Metformin HCl |
278 |
Tamarind seed polysaccharide–alginate floating beads that have been emulsion-gelled |
Diclofenac sodium |
279 |
Microbeads of zinc alginate-carboxymethyl cashew gum |
Isoxsuprine HCl |
280 |
Sodium alginate |
Theophylline |
247 |
Diltiazem HCl |
281 |
Metronidazole |
282 |
Gliclazide |
283 |
Sulindac |
284 |
Ampicillin |
285 |
Diclofenac sodium |
286 |
Furosemide |
287 |
Alginate–locust bean gum |
Diclofenac sodium |
288 |
Alginate–pectinate |
Aceclofenac |
289 |
Alginate–gellan gum |
Glipizide |
290 |
Alginate–gellan gum |
Aceclofenac |
268 |
Alginate–xanthan gum |
Diclofenac sodium |
291 |
Alginate, guar gum, locust bean gum, and xanthan gum |
Diclofenac sodium |
292 |
Alginate–gum Arabic |
Glibenclamide |
293 |
Sterculia gum–alginate |
Pantoprazole |
294 |
Gum Arabic–alginate |
Glibenclamide |
293 |
Tamarind gum–alginate |
Gliclazide |
266 |
Tamarind gum–alginate |
Metformin HCl |
267 |
Tamarind gum–pectinate |
Metformin HCl |
295 |
Tamarind gum–alginate |
Diclofenac sodium |
296 |
Tamarind gum–gellan gum |
Metformin HCl |
297 |
Gum–alginate of okra |
Diclofenac sodium |
263 |
Alginate from okra |
Glibenclamide |
263 |
Linseed polysaccharide–alginate |
Diclofenac sodium |
262 |
Seed mucilage-alginate of fenugreek |
Metformin HCl |
265 |
Mucilage-alginate from the ispaghula husk |
Glibenclamide |
298 |
Gliclazide |
298 |
Isoniazid |
299 |
Metformin HCl |
300 |
Fruit gum–alginate from dellinia |
Timolol maleate |
301 |
Kondagogu-alginate gum |
Glipizide |
302 |
Mucilage-alginate from fenugreek seeds |
Metformin HCl |
265 |
Alginate |
Zidovudine |
240 |
Alginate |
DOX loaded liposomes |
241 |
Sodium-alginate |
Indomethacin |
242 |
Retinoic acid |
243 |
DOX |
244 |
5-Fluorouracil |
245 |
Alginate |
Ibuprofen |
246 |
Sodium-alginate |
Ketoprofen |
247 |
Alginic acid |
DOX |
248 |
Chitosan, sodium alginate |
Rabeprazole sodium |
303 |
Alginate–locust bean gum |
Diclofenac sodium |
288 |
Alginate–pectinate |
Aceclofenac |
289 |
Alginate–gellan gum |
Glipizide |
290 |
Alginate–gellan gum |
Aceclofenac |
268 |
Alginate–xanthan gum |
Diclofenac sodium |
291 |
Alginate, guar gum, locust bean gum, and xanthan gum |
Diclofenac sodium |
292 |
Alginate–gum Arabic |
Glibenclamide |
293 |
Sterculia gum–alginate |
Pantoprazole |
294 |
Gum Arabic–alginate |
Glibenclamide |
293 |
Tamarind gum–alginate |
Gliclazide |
266 |
Tamarind gum–alginate |
Metformin HCl |
267 |
Tamarind gum–pectinate |
Metformin HCl |
295 |
Tamarind gum–alginate |
Diclofenac sodium |
296 |
Gellan gum with tamarind gum |
Metformin HCl |
297 |
Gum-alginate of okra |
Diclofenac sodium |
263 |
Gum-alginate of okra |
Glibenclamide |
263 |
Linseed polysaccharide–alginate |
Diclofenac sodium |
262 |
Seed mucilage-alginate of fenugreek |
Metformin HCl |
265 |
Mucilage-alginate from the ispaghula husk |
Glibenclamide |
298 |
Mucilage-alginate made from spigola husk |
Gliclazide |
298 |
Mucilage-pectinate from the ispaghula husk |
Aceclofenac |
304 |
Spaghula husk mucilage-pectinate |
Metformin HCl |
305 |
Mucilage-gellan gum made from spaghetti husk |
Metformin HCl |
306 |
3.1.7 Dextran. The microbially produced dextran is a complex branching poly-d-glucoside with variable-length glycosidic linkages. On a group of human tumour xenografts and colon cancer, a dextran–camptothecin combination demonstrated strong anticancer efficacy in vivo.192 A combination of dextran and exatecan demonstrated substantial therapeutic effectiveness against a panel of murine solid tumours and human xenografts.307 Compared to the free medication, methotrexate to dextran combination demonstrated enhanced activity against human tumour xenograft models.308 Multiple colon cancer cell lines exposed to paclitexal–carboxymethyl–dextran ester conjugates shown strong anticancer activity in vivo.309 In a Lewis lung cancer rat model, an imine conjugation of DOX to Ox-dextran shown greater therapeutic effectiveness.192 Additionally, a clinical investigation for a CM-dextran combination with delimotecan has begun.310 Dextran nanogels that have been PEGylated have been used for gene therapy using short interfering RNA,311 which, in human hepatoma and glioblastoma, have effectively accomplished gene knockdown. Drug-resistant cancer cell lines may be treated using Dextran nanogels that carry siRNA to silence the genes that cause multidrug resistance.192 Fast-dissolving oral medication administration was achieved by electrospinning metronidazole/hydroxypropyl-β-cyclodextrin inclusion complex nanofibrous webs.312For complexation, cyclodextrins are most often used. Examples include budesonide (for pulmonary drug delivery), acetazolamide (for ocular drug administration), and buserelin (nasal drug delivery).313 To overcome drug resistance, a gold-paclitaxel nanoconjugate was developed using -cyclodextrin.314 Additionally, diisocyanate-modified Fe3O4 and cyclodextrin were used to create magnetic nanoconjugates of dacarbazine.315 Methotrexate was conjugated with dextran to lower the dosage and lessen unwanted effects.316 Dextran and 5-aminosalicylic acid conjugates have been utilised to carry drugs to the small intestine and stomach. The prodrug of the azo-coupled dextran-salicylic acid combination regulated the drug's release into the colon.317 Since nalidixic acid alone is pH sensitive, dextran-nalidixic acid ester conjugate is used as a colon-specific prodrug.318 When used to treat human ovarian cancer cells, dextran nanocomposites with paclitaxel and DOX shown encouraging results for more tumour mass penetration and less side effects than the pure medication.210 Curcumin- and curcumin-γ-hydroxypropyl cyclodextrin-loaded nanoconjugates were shown to be more efficient than the pure medication for delivering genetic material in cancer cells.319 Table 5 lists some further instances of dextran and its compounds being used for medication delivery.
Table 5 Drug delivery using dextran and its compounds
Polymer |
Bioactive agent |
References |
Dextran |
Curcumin |
320 |
Indomethacin |
321 |
Indomethacin |
322 |
Fructose |
Curcumin |
323 |
Cyclodextrin |
Paclitaxel |
324 |
Cyclodextrin |
Dacarbazine |
314 |
Dextran 5 |
Aminosalicylic acid |
315 |
Nalidixic acid |
317 |
Methotrexate |
325 |
Ibuprofen |
316 |
DOX and paclitaxel |
326 |
Cyclodextrin |
Camptothecin |
327 |
β-cyclodextrin |
Camptothecin |
328 |
Carboxymethyl dextran |
Delimotecan |
329 |
Carboxymethyl dextran |
Exatecan |
330 |
Dextran |
DOX |
331 |
3.1.8 Heparin. A member of the glycosaminoglycan family, heparin is a sulfated polysaccharide containing a linear anionic unit composition that is mostly composed of 2-O-sulfo-iduonic acid and 2-deoxy-2-sulfamino-6-O-sulfo-d-glucose, with trace quantities of 2-acetamido-2-deoxy-d-glucose. DDS also came into contact with heparin anionic polysaccharides. All-trans-retinoic acid and DOX were combined to form a system that Zhang and colleagues created. It was coupled to low molecular weight heparin, and DOX was physically loaded.109 A heparin/DOX complex-containing composite system was described. To create self-assembled NPs encapsulating docetaxel, a polymer in the coupling of stearyl amine with low molecular weight heparin was employed. The research examined the NPs' effects on human breast cancer cell lines.332 Table 6 lists some further instances of the use of heparin and its mixtures in medication administration.
Table 6 Drug delivery using heparin and its blends
Polymer |
Bioactive agent |
References |
Heparin |
DOX |
333 |
DOX and all trans retinoic acid |
334 |
A peptide-modified DOX |
335 |
Retinoic acid |
336 |
Heparin-graft-α-tocopherol |
Docetaxel |
337 |
Heparin-graft-deocycholate |
DOX |
338 |
Pegylated heparin a |
Pyropheophorbide-a |
339 |
Heparin |
Paclitaxel |
340 |
3.1.9 Xanthan gum. A polysaccharide, xanthan gum is often used as a food ingredient in industry. It has been reported that the drug release mechanism from binary composition tablets made of quetiapine fumarate, xanthan, and tragacanth gum (anionic polysaccharide) obtained a drug control release similar to that of the commercial product.341,342 A successful report of ibuprofen-loaded xanthan gum microsphere.343 When combined with xanthan gum, Terminalia chebula, Glycyrrhiza glabra, Emblica Officinalis, Terminalia belerica, and Turbinella rapa herbal extracts shown increased efficacy.344 Lamivudine microsphere made from xanthan and guar gum revealed a slower release rate and continuous release for up to 24 hours.345 Metformin hydrochloride mucoadhesive microspheres produced with various XG and guar gum concentrations have been reported.346 A carbamazepine mucoadhesive nanoemulgel for targeting the brain via the olfactory mucosa is also disclosed.347 For effective transport of curcumin to the brain through the nose, xanthan gum-coated mucoadhesive liposomes were studied. The ability to effectively carry medication into the brain through the nasal route using xanthan gum-coated liposomes or other nanocarriers has been shown.348 Drug release from formulations including xanthan gum and carbopol 934 was maintained for 8 hours.349
3.1.10 Pectin. The major goal of the work was to alter specific pectin characteristics by adding thiol moieties to the polymer via the construction of pectin (anionic polysaccharide)–cysteine conjugates.109 Pectin-cysteine beads with zinc added showed increased stability in simulated gastrointestinal settings, but their insulin release profile was identical to that of unaltered zinc pectinate beads.109 To administer paclitaxel, a pectin-conjugated magnetic graphene oxide composite was created.109 Methotrexate-conjugated pectin nanoparticles (NPs) were developed for the delivery of a cytotoxic drug to hepatic cancer cells.350 Pectin–adriamycin conjugates' potential for lymphatic targeting was investigated.351 Using pectin matrices coated with eudragit 100, the release of 5-fluorouracil in the colon was examined. Pectin formulation reduced cytotoxicity concentration in cells by 50% in human colon cancer cells.352 Some other examples of pectin in drug delivery are reported in Table 7.
Table 7 Role of Pectin in drug delivery
Polymer |
Bioactive agent |
References |
Pectin |
Indomethacin |
353 |
Rutin |
354 and 355 |
Theophylline |
356 |
Ketoprofen |
357 |
Cisplatin |
358 |
Insulin |
359 |
Paclitaxel |
360 |
Methotrexate |
350 |
Adriamycin |
351 |
5-Flourouracil |
352 |
3.1.11 Polyarginine. This sugar containing molecules also made its space for designing DDS. Because of its capacity to permeate membranes, polyarginine is often employed in DDS as a cell-penetrating peptide.361 For the delivery of quantum dots, arginine-rich peptides have been widely used.362 Role of arginine peptides in drug delivery is reported in Table 8.
Table 8 Role of Polyarginine in drug delivery
Polymer |
Bioactive agent |
References |
Polyarginine |
Cyclosporin A |
363 |
NLC encapsulated spantide II and ketoprofen |
364 |
Liposomes encapsulated curcumin |
365 |
Liposomes encapsulated polygonium |
366 |
3.1.12 Pullulan. A polysaccharide made of maltotriose trimers is pullulan. A neutral polysaccharide called pullulan has been researched for non-viral gene delivery techniques.107 In order to explore their potential for usage in gene delivery applications, Rekha and colleagues produced polyethyleneimine-conjugated pullulans.367 Utilizing pullulan-deoxycholic acid conjugated for medication administration in cancer patients, Na and colleagues created a self-organized nano gel of DOX. The effective transfer of plasmid DNA to the liver was made possible by serine pullulan samples.368 DNA could be quantitatively loaded into pullulan microspheres using 1,2-chloro-2,3-epoxypropane without DNA degradation.369 Pullulan nanoparticles disulfide-cross-linked with folic acid for antitumoral hepatic drug delivery.370 In order to functionalize nanocarriers for targeted medication delivery in the treatment of cancer, heparin has been widely researched for its anticancer action.109 Table 9 lists some further instances of pullulan and its mixtures being used for medication delivery.
Table 9 Drug delivery using pullulan and its composites
Polymer |
Bioactive agent |
References |
Pullulan-graft-polycaprolactone |
Ciprofloxacin |
371 |
Pullulan-graft-α-tocopherol |
ε-Caprolactone |
372 |
Pullulan–graft-SA |
DOX |
373 |
Pullulan-graft, biotin, and retinoic acid |
DOX |
374 |
Desaxycholic acid from pullulan grafts -graft-polyethyleneimine |
DOX DNA |
375 |
Pulullan-graft-retinoic acid |
DOX |
376 |
Dibutyl amino propyl carbamate-pululan-graft |
DNA |
377 |
Pullulan-graft-cholesterol |
Methotrexate |
378 |
Cysteine from heparin-graft-β-sitosterol |
DOX |
90 |
Pullulan |
Polyethyleneimine, DOX, adriamycin |
367 and 379 |
Schizophyllan-graft-styrene acrylonitrile |
Paclitexal |
380 |
3.1.13 Starch. Starch is a non-ionic carbohydrate. Occurring in situ during the formation of CuO NPs, oxidised starch-CuO nanocomposite hydrogels allowed for the measurement of extended drug release for the CuO NPs containing oxidised starch that was elevated by increasing the CuO amount. In a separate study, two controlled-release drug carriers for the medicine methylprednisolone were developed as silver-starch nanocomposite beads.121 Table 10 lists some other instances of the use of starch and its composites for medication delivery.
Table 10 Starch and its Composites in drug delivery
Polymer |
Bioactive agent |
References |
Hydroxyethyl starch |
Curcumin |
381 |
Starch coated onto the Fe3O4 |
Magnetic carrier |
272 |
Pectinate–high amylase starch |
Diclofenac sodium |
382 |
Tapioca starch–alginate |
Metoprolol tartrate |
383 |
Potato starch–alginate |
Tolbutamide |
384 |
Potato starch–alginate |
Ibuprofen |
385 |
Assam bora rice starch–alginate |
Metformin HCl |
386 |
Jackfruit seed starch–alginate |
Pioglitazone |
279 |
Jackfruit seed starch–pectinate |
Metformin HCl |
387 |
Jackfruit seed starch–alginate |
Metformin HCl |
388 |
Jackfruit seed starch–gellan gum |
Metformin HCl |
389 |
Starch |
DOX, hydroxycamptothecin, chlorpheniramine maleate |
390 |
Aminated starch |
Curcumin |
391 |
Starch |
Tungstophosphoric acid |
392 |
Carboxymethyl starch |
Mesalamine |
393 |
PEGylated starch |
DOX |
394 |
Starch acetate |
Cisplatin |
395 |
Commercial glycerin latex, glutinous starch, rice, and potatoes |
Lidocaine |
396 |
Alginate-starch beads |
Aceclofenac |
397 |
Alginate beads made from jackfruit seed |
Pioglitazone |
398 |
Alginate-starch beads made of jackfruit seeds |
Metformin HCl |
292 |
Particles made of soluble starch composites with Ca21–Zn21-alginate |
Aceclofenac |
399 |
Rice-starch-alginate beads from Assam |
Metformin HCl |
386 |
Particles made of soluble starch composites with Ca21–Zn21-alginate |
Aceclofenac |
399 |
3.1.14 Guar gum. To assess their potential for drug delivery, neutral and cationic guar gum nanocomposites containing montmorillonite-loaded ibuprofen have been studied.400 Guar gum-graft-acrylic acid was synthesized by an L-alanine crosslinker for hydrophilic drug delivery.401 Guar-gum-polyacrylamide incorporated with diltiazem hydrochloride has been reported.402 Acrylamide-grafted-guar-gum blended with chitosan as DDS has been evaluated.403 For the transdermal distribution of the medication diclofenac sodium, carboxymethyl guar gum containing nano silica was created.404 Guar-gum nanocomposite hydrogels and multiwalled carbon nanotubes have been utilised to administer the drug diclofenac sodium.405Target-specific crosslinked hydrogels based biopolymers for the controlled release of cephradine.406 For the in vitro release of cephradine, chitosan/guar gum hydrogels were created by mixing with PEG. Results indicated that 85 percent of the cephradine was released in 130 minutes. Gelatin is a linear polypeptide made up of 18 distinct kinds of amino acids and a hydrophilic biopolymer. To treat resected primary/metastatic bone locations, zoledronic acid-containing nanocomposites of gelatin and beta-tricalcium phosphate were created.150 The biocompatible gelatin was filled with methotrexate.151 Zinc oxide was synthesised in situ to create antibacterial chitosan/zinc oxide nanocomposite hydrogels that were used as naproxen drug delivery systems.152 Table 11 lists further instances of gelatin being used for medication delivery.
Table 11 Examples of Gealtin in drug delivery
Polymer |
Bioactive agent |
References |
Gelatin |
Tizanidine hydrochloride |
407 |
408 |
Gatifloxacin |
409 |
Fluconazole |
410 |
The local administration of anticancer medications using injectable chitosan-based gels has shown significant potential. For instance, liposomal DOX was loaded into a thermosensitive injectable hydrogel with chitosan and -glycerophosphate that released DOX in vitro in a pH-dependent manner.217 Some other examples of gum and its composites in drug delivery are reported in Table 12.
Table 12 Some examples of Gum and its composites in drug delivery
Polymer |
Bioactive agent |
References |
Guar gum galactomannan-graft-acetic anhydride |
Cur |
411 |
Bletilla striata-graft-SA |
DOX |
412 |
Phthalated cashew gum |
Benznidazole |
413 |
Gellan gum |
Amoxicillin, amoxicillin trihydrate, cephalexin |
414 and 415 |
Fenugreek seed mucilage–pectinate |
Metformin HCl |
416 |
Fenugreek seed mucilage–gellan gum |
Metformin HCl |
417 |
3.2 Protein-based DDS
Natural poly(amino acids), a kind of biodegradable ionic polymers, has only one type of amino acid. The biomaterials that are examined the most often are poly(γ-glutamic acid) and poly(L-lysine). The glutamic acid polymer Because of the polymer's reactive side carboxylate centres, other functional groups and medications may be attached covalently.4 Antibiotics, vaccines, DNA, and proteins have all been explored to be transported using poly(γ-glutamic acid)-based particles. A poly(glutamic acid)-based carrier that contains paclitaxel is a well-known cancer product. In biomedical applications, it has been employed as a carrier to increase the effectiveness of several interferon inducers, antiviral medications, and anticancer medications. Biomedical uses for polynucleotides like DNA and RNA exist.418 The role of individual proteinaceous polymers is discussed below:
3.2.1 Collagen. Collagen, the primary element of connective tissues, is the most abundant protein in the human body. Due to their diverse qualities, which include mechanical strength and biocompatibility-degradability, 29 distinct kinds of collagen have been identified and are being intensively explored for use in the fabrication of the DDS.79 Low-molecular-weight pharmaceuticals may be transported well by collagen; gentamicin-loaded collagen-based delivery systems are one example of this. Numerous different collagen and synthetic polymer composite systems are being described as DDS.79,419 Polylactic-co-glycolic acid and alginate microparticles containing collagen have been produced for continuous administration of recombinant human bone morphogenetic protein 2, making them an effective controlled delivery vehicle of the pro osteogenic factor.420 Additionally, collagen/polyvinyl alcohol is being tested for the continuous administration of salicylic acid.421 To combat bacterial infections, collagen scaffolds crosslinked with hexamethylene diisocyanate and containing cefaclor have been created.422 Collagen bandage contact lenses to lubricate the eye along with active ingredients are reported.423 It has been reported to use collagen-alginate microspheres to deliver medications to the eyes.424 In order to lubricate the eye, collagen bandage contact lenses containing active substances and a coating of collagen have been used.423 Alginate hydrogels with composite collagen content have been described for medication administration to the eye.425 Table 13 discusses the few applications of collagen and its composite.
Table 13 Collagen and its composites in drug delivery
Polymer |
Bioactive agent |
References |
Collagen |
DOX |
426 |
Hydrolyzed collagen |
Hydrocortisone |
8 |
Collagen |
DOX |
427 |
Chloramphenicol |
428 |
Ibuprofen |
429 |
Fludarabine/epirubicin |
430 |
Cardamom extract |
431 |
5-Fluorouracil |
432 |
Econazole nitrate |
433 |
Ciprofloxacin |
434 |
Hydroxyapatite-collagen alginate composites |
Bone morphogenetic protein |
435 |
3.2.2 Natural rubber. Natural rubber is a very elastic polymer with a strongly crosslinked structure that is an intriguing biomaterial for DDS for proteins, antitumoral drugs, and antimicrobial chitosan.4 Pilocarpine was released from gelatin hydrogels created by Natu et al. during an 8 hour period at a rate ranging from 29 to 99 percent.436 Human elastin-like polypeptides were included into the creation of bioactive molecules, which showed how therapeutic compounds may be delivered in response to proteolytic stimuli.437 In order to combine the best qualities of two materials that may be employed for therapeutic molecule administration, a composite matrix made of human elastin-like polypeptide hydrogel and electrospun poly-L-lactic acid was created.438,439 Some examples of starch and its composites in drug delivery are reported in Table 14.
Table 14 Natural rubber and its composites in drug delivery
Polymer |
Bioactive agent |
References |
Polyethylene glycol, natural rubber, carbazole, ammonium persulfate, 2-methyl-4-(methylthio)-2-morpholino propiophenone |
Indomethacin |
440 |
Hevea brasiliensis' isolated natural rubber latex |
Diclofenac potassium |
441 |
Natural rubber latex |
Ketoprofen natural |
442 |
3-Mercaptopropionate, 2-methyl-4-(methylthio)-2-morpholinopropiophenone, and natural rubber |
Ibuprofen |
443 |
3.2.3 Keratin. In clinical medicine, the administration of anticancer drugs may be possible using keratin, which is a highly practical and affordable protein for biomedical purposes.418,444 Han et al. used keratin for drug release of rhBMP-2, rhIGF-1, and ciprofloxacin, as well as simple alkylation on keratin for regulated release of gel G, which demonstrated no toxicity.445 As a model drug, rhodamine B dye release from films made of keratin and polyvinyl alcohol that were crosslinked with starch was studied.446 DOX-loaded keratin NPs are also well reported.447
3.2.4 Albumin. Proteins are water-soluble, three-dimensional folded structures, made up of amino acids joined together by amide bonds.35 Different drug binding sites are found in albumin, allowing a range of medicines to be loaded.448 The most potent drug ever developed, albumin paclitaxel nanoparticle, is the first DDS approved by the FDA for the treatment of metastatic breast cancer. Levemir, created by Novo Nordisk, is another medication with albumin that is authorised for use in the treatment of type 1 and type 2 diabetes. A human insulin derivative that binds to albumin is present. Additionally, Herceptin and Avastin, which are NPs for the antibodies trastuzumab and bevacizumab, respectively, have been studied to learn more about their potential.449 An N-lysinal-N′-succinyl chitosan and poly (N-isopropylacrylamide) hydrogel was enclosed within a crosslinked bovine serum albumin shell in order to function as an effective carrier of chemotherapeutic drugs.450 Calcium corbonate hybrid particles with bovine serum albumin along with polydopamine showed good DDS applications.451 Table 15 lists some further instances of albumin and its mixtures being used for medication delivery.
Table 15 Albumin and its composites in drug delivery
Polymer |
Bioactive agent |
References |
Albumin |
Paclitaxel, warfarin, silibinin, diazepam |
452 |
Bovine serum albumin |
Curcumin |
453 |
Albumin |
Vancomycin |
454 |
HAS |
5-Aminosalicylic acid |
455 |
Bovine serum albumin |
Dimethylcurcumin |
456 |
Bovine serum albumin |
Curcumin |
457 |
Albumin |
Ibuprofen |
458 |
Irinotecan |
459 |
Panobinostat |
460 |
Cabazitaxel, noscapine, mitoxantrone |
407 |
461 |
462 |
Bovine serum albumin is a polymer made of oligo (ethylene glycol) methyl ether methacrylate |
Sprouty 1 (C-12) (Spry1) protein |
463 |
Human serum albumin |
Adriamycin |
464 |
3.2.5 Fibrin. The protein substance known as fibrin is often rigid and organised into long fibrous threads. Numerous fibrin matrices come in different forms that enable the controlled release and/or targeted administration of chemotherapeutics, growth factors, and cells.438 The organic protein biopolymer called silk sericin is derived from silkworms. Because of its abilities to promote cell growth, distribute drugs, heal wounds, and have certain therapeutic benefits, it is referred to as a biomaterial.418 Additionally, the kinetics of physically crosslinked silk films carrying the medicines crizotinib and DOX are evaluated.465 Core-shell silk fibroin hydrogels incorporated with albumin for drug delivery are also designed.466 Silk NPs that are DOX-loaded exhibit better NP cell uptake and promote cytotoxicity against cancer cells.467 Insulin was employed as a growth factor in silk NPs by Wenk et al., who demonstrated that the release was constant for 7 weeks.468 Some other examples of silk fibroin and its composites in drug delivery are reported in Table 16.
Table 16 Silk fibroin and its composites in drug delivery
Polymer |
Bioactive agent |
References |
Silk fibroin |
5-Fluorouracil |
469 |
Methacrylated silk fibroin |
Mouse articular chondrocytes |
470 |
Silk fibroin or gelatin |
Ibuprofen |
471 |
Ibuprofen |
472 |
Curcumin |
473 |
DOX |
474 |
Ciprofloxacin |
475 |
DOX |
476 |
DOX |
477 |
Silk NPs |
FITC |
478 |
Silk microcapsules |
FITC labeled dextrans |
479 |
Silk NPs |
FITC |
480 |
Silk |
DOX |
481 |
5-Fluorouracil |
469 |
DOX, indocyanine green |
482 |
3-Mercapto propionic acid coated CdTe quantum dots |
483 |
Rhodamine B |
484 |
Methylene blue |
485 |
Recombinant human insulin |
486 |
FITC dextran |
487 |
Insulin |
488 |
Tetracycline |
489 |
Ibuprofen |
490 |
Antibiotics |
491 |
3.2.6 Soy protein and pea legumin. Soy and pea proteins are very protein-dense with around 90% protein content while soy protein is widely-known for its abundance of essential amino acids, pea protein is gaining ground for its vegan-friendly profile. Combinations of proteins (soy protein and pea legumin) substances with other biopolymers are also reported to be engaged in DDS to deliver active ingredients like nutraceuticals.418 Legumin was employed to deliver methylene blue as a model drug.492 Soy proteins was used to deliver the timolol maleate.493 Soy protein-based films by glycerol and gelatin are also reported.494 Various formulations e.g. micro and nano hydrogel, tablet and electrospun fibers were reported.419 Soy protein is a well-characterized resource for the production of nanogel-based drug delivery and nutraceutical delivery applications.495 The enzymes pepsin and pancreatin are capable of degrading soy protein compositions. Additional reports of soy protein hydrogel and tablets containing riboflavin are available.496
3.2.7 Zein. Zein, a hydrophobic protein abundant in prolamine and present in the endosperm of the maize kernel, is often employed in films and coatings. Zein is typically extracted by aqueous alcohol at 60 °C from corn gluten meal. Zein NPs coated with sodium deoxycholate are stable, biocompatible colloidal carriers that may be utilised as effective DDS.3,438 The bioavailability of carvedilol was improved by up to 7 times by NPs made from casein-silk fibroin.497 In vivo tests on rats using NPs created by crosslinking silk fibroin and casein revealed enhanced bioavailability.498 Rutin was enclosed by pectin–casein NPs in simulated gastric and intestinal settings, extending the period of release and sustaining release.499 Casein NPs with alfuzosin hydrochloride loaded on them showed persistent alfuzosin hydrochloride release for 24 hours.500 Whey proteins, synthetic and biopolymers, and electrospun fibres and microspheres were used to create a number of different compositions.419 Due to matrix degradation, riboflavin-loaded whey proteins and alginate NPs showed pH-sensitive drug release.501 Some other examples of protein and its composites in drug delivery are reported in Table 17.
Table 17 Protein and its composites in drug delivery
Polymer |
Bioactive agent |
References |
Milk protein casein |
DOX indocyanine green |
502 |
Lactoferrin and glycomacropeptide |
Nanocarrier for curcumin |
503 |
Gliadin |
Carbazole |
504 and 505 |
Whey protein |
Ndomethacin |
506 |
Investigational new drug |
507 |
Carvedilol |
508 |
Folic acid |
509 |
Fenofibric acid |
510 |
Curcumin |
511 |
Saffron |
512 |
Vitamin E |
513 |
Resveratrol and naringenin |
514 |
Curcumin |
515 |
Theophylline |
516 |
Riboflavin |
517 |
Insulin |
518 |
Curcumin |
519 |
Proguanil hydrochloride, chloroquine diphosphate |
520 |
Daidzein |
521 |
Lycopene |
522 |
Zinc citrate |
523 |
Zinc |
524 |
Theophylline |
525 |
Puerarin 5 |
526 |
Indomethacin, carvedilol, and furosemide |
527 |
Whey protein isolate |
Curcumin |
528 |
Curcumin with amylase |
Encapsulated curcumin |
272 |
ABD035 peptide |
Paclitaxel |
529 |
Conjugates with insulin released the payload for several hours in the intestine hence, controls the blood glucose levels effectively by increasing its bioavailability.530 DOX-loaded nanospheres functionalized showed great promise against breast and ovarian cancers with targeting antibodies.313 Deoxycholic acid NPs that have been crosslinked with chitosan and are filled with plasmid DNA successfully transfect COS-1 cells. Chitosan NPs delivered tumor-suppressing interleukin receptor B, siRNA and DOX to inhibit the migration of breast cancer cells. Gal-1 expression in tumor-bearing mice was significantly reduced by chitosan NPs, which were created to carry siRNA against a brain tumour.192 It was shown that chitosan conjugates with CdS might function as adaptable nanoplatforms for the creation of in vivo and in vitro cancer treatments.531 Using chitosan-DOX nanoconjugates for targeted administration, ovarian and breast cancer outcomes were favourable.313
A variety of therapeutic molecules e.g. proteins and vaccines are delivered by zein microsphere, particularly for drugs with low solubility in water in oral delivery.192 Zein films showed proven biocompatibility by successfully culturing in the mice fibroblasts and liver cells of human.202 According to reports, it is also used as a covering material for tablets because of its exceptional resistance to abrasion, heat, and humidity. Ivermectin, coumarin, and 5-fluorouracil will be delivered via Zein.407,532 Zein (core) and pectin (shell) are also reported to deliver encapsulated curcumin.533 Electrospun woven fibres made of zein and hydroxyapatite showed improved calcium phosphate mineralization in simulated bodily fluid and were shown to be biocompatible with adipose-derived stem cells.196 Hyaluronic acid has been combined with mesoporous based-silica nanoconjugates for cell-specific regulated and targeted drug release for the enzymes at target location.534
3.2.8 Gellan gum. Gellan gum is an anionic, water-soluble polymer that is produced by the bacterium Sphingomonas elodea. Gellan gum-based nano-hydrogel systems have been widely documented for use in ophthalmic, gastric, and nasal drug administration applications. These systems take the shape of micro/macrobeads, films, hydrogels, fibres, granules, particles, pellets, spheres, and spheroids. It has been reported that paclitaxel and prednisolone are put onto gellan gum.535 To explore gold nanoparticles stabilised by gellan gum, human glioma cell lines and mouse embryonic fibroblast cells were employed.536 Gellan gum were also loaded with DOX hydrochloride.537 Along with gold, silver NPs swere also tabilized with the help of gellan gum for testing the cytotoxic activity and for intercellular drug delivery and imaging in mouse embryonic fibroblast cells,538. Hydrocolloid bead based gellan gum was also evaluated for slow drug release applications.539 For applications in mucoadhesive and gastroretentive drug administration, ofloxacin-loaded gellan gum and polyvinyl alcohol nanofibers.540 Resveratrol loaded chitosan/gellan gum nanofibers have also been reported for gastrointestinal delivery system.541 Sericin (natural protein)-chitosan doped maleate gellan gum composites have also benn reported to cure Mycobacterium tuberculosis.542 It has been reported that gellan gum in the form of hydrogels combined with LAPONITE® clay makes an effective medication delivery mechanism.543 Gellan gum composites with sericin and rice bran albumin has also been employed as a drug carrier DOX.542 For the buccal delivery of aceclofenac, composites of gellan gum-amino methacrylate has been employed.544 There have also been reports of gellan gum derivatives for the controlled release of ciprofloxacin.545 Metformin HCl was delivered intragastrially under regulated conditions using gellan gum hydrogel composites with olive oil-incorporated pectin that had been modified by diethanolamine.546
3.2.9 Gum acacia/gum Arabic. Gum acacia is an environmentally benevolent and biodegradable natural polymer. Gum arabic has reportedly been used to construct multiple-unit DDS, such as beads, microparticles, NPs, etc. for sustained drug release for a variety of medicines.547 It has been utilized for the release of bisphosphonate drugs.548 Gum acacia–hydroxyapatite nanocomposite was utilized to deliver the naringenin drug.549 Additionally, it has been used as a controlled release method for antiprotozoal medications including curcumin and derivatives of the 4-aminoquinoline ring.550 When prednisone was delivered using polyvinyl alcohol/gum acacia/titania nanocomposites, it was revealed that the release of the medication was pH-responsive.136 Acacia-carbopol-polyvinyl imidazole hydrogel loaded with gentamicin and lidocaine has also been reported.551
4 Conclusions and future perspectives
Natural biopolymers and their derivatives play a remarkable role in improving the biosafety of medication cargo and targeted delivery while reducing adverse effects. The above-discussed promising uses for biopolymers point the way to a new technique for creating unique DDS with enhanced therapeutic benefits for scaling up novel formulations to the clinical level. Above discussion also revealed that since properties and hence applications of the resultant biopolymer composites are dictated by the composition of the constituents, reaction parameters and synthetic techniques, therefore it is very crucial to optimize the biopolymeric formulations for any specific application. Another important consideration is the polymeric carrier's bioacceptability, which is influenced by the shape, size and physicochemical characteristics of both the polymer and the medication. The above discussion reviewed here motivates to devise the of engineered biopolymer-based materials with optimized properties by composites and functionalization fabrication. But there are still some things to think about before using these nanocarrier systems for therapeutic purposes. One of these entails improving biosafety even further, as well as the effects of repeated doses at the intended spot. Important issues that need additional research are chemical and structural stability during application and storage, as well as the interpretation of drug delivery design from an experimental to a commercial development perspective.
Data availability
The datasets generated during and/or analyzed during the current study are available from the corresponding author upon reasonable request.
Author contributions
The manuscript was written with the contributions of all authors. All authors have approved the final version of the manuscript.
Conflicts of interest
The authors declare no conflict of interest.
Acknowledgements
The authors extend their appreciation to the Deanship of Scientific Research at King Khalid University for supporting this work through research groups program under grant number RGP.2/273/44.
References
- J. Wróblewska-Krepsztul, et al., Biopolymers for Biomedical and Pharmaceutical Applications: Recent Advances and Overview of Alginate Electrospinning, Nanomaterials, 2019, 9, 404 CrossRef.
- S. Talebian, et al., Biopolymers for antitumor implantable DDS: recent advances and future outlook, Adv. Mater., 2018, 30(31), 1706665 CrossRef.
- A. Gagliardi, et al., Sodium deoxycholate-decorated zein nanoparticles for a stable colloidal drug delivery system, Int. J. Nanomed., 2018, 13, 601 CrossRef CAS.
- M. C. García, DDS based on nonimmunogenic biopolymers, in Engineering of Biomaterials for DDS, Elsevier, 2018, pp. 317–344 Search PubMed.
- X. Chen, et al., Ultra-selective molecular-sieving gas separation membranes enabled by multi-covalent-crosslinking of microporous polymer blends, Nat. Commun., 2021, 12(1), 6140 CrossRef CAS PubMed.
- C. R. Lynch, et al., Hydrogel biomaterials for application in ocular drug delivery, Front. bioeng. biotechnol., 2020, 8, 228 CrossRef.
- E. Calzoni, et al., Biocompatible polymer nanoparticles for drug delivery applications in cancer and neurodegenerative disorder therapies, J. Funct. Biomater., 2019, 10(1), 4 CrossRef CAS PubMed.
- A. Ali and S. Ahmed, A review on chitosan and its nanocomposites in drug delivery, Int. J. Biol. Macromol., 2018, 109, 273–286 CrossRef CAS.
- S. R. Jameela and A. Jayakrishnan, Glutaraldehyde cross-linked chitosan microspheres as a long acting biodegradable drug delivery vehicle: studies on the in vitro release of mitoxantrone and in vivo degradation of microspheres in rat muscle, Biomaterials, 1995, 16(10), 769–775 CrossRef CAS.
- V. R. Sinha, et al., Chitosan microspheres as a potential carrier for drugs, Int. J. Pharm., 2004, 274(1–2), 1–33 CAS.
- L. Li, et al., Effect of formulation variables on in vitro release of a water-soluble drug from chitosan–sodium alginate matrix tablets, Asian J. Pharm. Sci., 2015, 10(4), 314–321 CrossRef.
- Y. Shao, et al., Evaluation of chitosan−anionic polymers based tablets for extended-release of highly water soluble drugs, Asian J. Pharm. Sci., 2015, 10, 24–30 CrossRef.
- A. M. Dyer, et al., Nasal delivery of insulin using novel chitosan based formulations: a comparative study in two animal models between simple chitosan formulations and chitosan nanoparticles, Pharm. Res., 2002, 19(7), 998–1008 CrossRef CAS PubMed.
- A. G. Luque-Alcaraz, et al., Preparation of chitosan nanoparticles by nanoprecipitation and their ability as a drug nanocarrier, RSC Adv., 2016, 6(64), 59250–59256 RSC.
- J. Jacob, et al., Biopolymer based nanomaterials in DDS: A review, Mater. Today Chem., 2018, 9, 43–55 CrossRef CAS.
- S. Mitra, et al., Tumour targeted delivery of encapsulated dextran-DOX conjugate using chitosan nanoparticles as carrier, J. Controlled Release, 2001, 74(1–3), 317–323 CrossRef CAS PubMed.
- S. Sanyakamdhorn and D. Agudelo, Encapsulation of Antitumor Drug DOX and Its Analogue by Chitosan Nanoparticles, Biomacromolecules, 2013, 14, 557–563 CrossRef CAS PubMed.
- H. Katas, M. A. Raja and K. L. Lam, Development of Chitosan Nanoparticles as a Stable Drug Delivery System for Protein/siRNA, Int. J. Biomater., 2013, 2013, 146320 Search PubMed.
- K. A. Janes, et al., Chitosan nanoparticles as delivery systems for DOX, J. Controlled Release, 2001, 73(2–3), 255–267 CrossRef CAS.
- I. C. Kwon, Chitosan-based nanoparticles for cancer therapy; tumor specificity and enhanced therapeutic efficacy in tumor-bearing mice, J. Controlled Release, 2008, 132(3), e69–e70 CrossRef CAS.
- C. Cánepa, et al., Development of a Drug Delivery System Based on Chitosan Nanoparticles for Oral Administration of Interferon-α, Biomacromolecules, 2017, 18(10), 3302–3309 CrossRef.
- H. S. Yoo, T. G. Kim and T. G. Park, Surface-functionalized electrospun nanofibers for tissue engineering and drug delivery, Adv. Drug Delivery Rev., 2009, 61(12), 1033–1042 CrossRef CAS PubMed.
- R. Jayakumar, et al., Novel chitin and chitosan nanofibers in biomedical applications, Biotechnol. Adv., 2010, 28(1), 142–150 CrossRef CAS PubMed.
- S.-H. Chen, et al., Preparation and characterization of antiadhesion barrier film from hyaluronic acid-grafted electrospun poly(caprolactone) nanofibrous membranes for prevention of flexor tendon postoperative peritendinous adhesion, Int. J. Nanomed., 2014, 9, 4079–4092, DOI:10.2147/ijn.s67931.
- R. Toshkova, et al., Antitumor activity of quaternized chitosan-based electrospun implants against Graffi myeloid tumor, Int. J. Pharm., 2010, 400(1–2), 221–233 CrossRef CAS PubMed.
- A. C. Mendes, et al., Hybrid electrospun chitosan-phospholipids nanofibers for transdermal drug delivery, Int. J. Pharm., 2016, 510(1), 48–56 CrossRef CAS PubMed.
- R. Barreiro-Iglesias, et al., Preparation of chitosan beads by simultaneous cross-linking/insolubilisation in basic pH. Rheological optimisation and drug loading/release behaviour, Eur. J. Pharm. Sci., 2005, 24(1), 77–84 CrossRef CAS PubMed.
- V. H. Kulkarni, P. V. Kulkarni and J. Keshavayya, Glutaraldehyde-crosslinked chitosan beads for controlled release of diclofenac sodium, J. Appl. Polym. Sci., 2007, 103(1), 211–217 CrossRef CAS.
- A. K. Anal and W. F. Stevens, Chitosan-alginate multilayer beads for controlled release of ampicillin, Int. J. Pharm., 2005, 290(1–2), 45–54 CrossRef CAS PubMed.
- C. Tang, et al., Preparation of ibuprofen-loaded chitosan films for oral mucosal drug delivery using supercritical solution impregnation, Int. J. Pharm., 2014, 473(1–2), 434–441 CrossRef CAS PubMed.
- C. Giovino, et al., Development and characterisation of chitosan films impregnated with insulin loaded PEG-b-PLA nanoparticles (NPs): a potential approach for buccal delivery of macromolecules, Int. J. Pharm., 2012, 428(1–2), 143–151 CrossRef CAS.
- A. S. Can, et al., Optimization and characterization of chitosan films for transdermal delivery of ondansetron, Molecules, 2013, 18(5), 5455–5471 CrossRef CAS PubMed.
- V. F. Patel, F. Liu and M. B. Brown, Advances in oral transmucosal drug delivery, J. Controlled Release, 2011, 153(2), 106–116 CrossRef CAS.
- A. S. Pedro, et al., Chitosan: An option for development of essential oil delivery systems for oral cavity care?, Carbohydr. Polym., 2009, 76(4), 501–508 CrossRef CAS.
- J. Duan, et al., High Strength Chitosan Hydrogels with Biocompatibility via New Avenue Based on Constructing Nanofibrous Architecture, Macromolecules, 2015, 48 CrossRef CAS.
- A. K. Azab, et al., Biocompatibility evaluation of crosslinked chitosan hydrogels after subcutaneous and intraperitoneal implantation in the rat, J. Biomed. Mater. Res., Part A, 2007, 83(2), 414–422 CrossRef PubMed.
- Y. N. Dai, et al., Swelling characteristics and drug delivery properties of nifedipine-loaded pH sensitive alginate-chitosan hydrogel beads, J. Biomed. Mater. Res., Part B, 2008, 86(2), 493–500 CrossRef PubMed.
- C. Hu, et al., Synergistic chemical and photodynamic antimicrobial therapy for enhanced wound healing mediated by multifunctional light-responsive nanoparticles, Biomacromolecules, 2019, 20(12), 4581–4592 CrossRef CAS.
- G. R. Park, et al., pH-sensitive gallol-rich chitosan hydrogel beads for on-off controlled drug delivery, Int. J. Biol. Macromol., 2023, 240, 124346 CrossRef CAS.
- H. Elmotasem, S. A. El-Marasy and A. L. Mohamed, Benzocaine mesoporous silica nanoparticles/bio polysaccharides-based hydrogels loaded cotton bandage as a platform for topical anesthesia, Results Chem., 2023, 5, 100830 CrossRef CAS.
- R. Lali Raveendran, M. Valsala and T. Sreenivasan Anirudhan, Development of nanosilver embedded injectable liquid crystalline hydrogel from alginate and chitosan for potent antibacterial and anticancer applications, J. Ind. Eng. Chem., 2023, 119, 261–273 CrossRef CAS.
- M. Jakubowski, et al., Chitosan hydrogel modified with lanthanum as a drug delivery system for epigallocatechin gallate: Investigation of hydrogel – drug interaction by FT-IR and Raman spectroscopy, Spectrochim. Acta, Part A, 2023, 297, 122748 CrossRef CAS.
- M. Rajaei, et al., Chitosan/agarose/graphene oxide nanohydrogel as drug delivery system of 5-fluorouracil in breast cancer therapy, J. Drug Deliv. Sci. Technol., 2023, 82, 104307 CrossRef CAS.
- Y. Xiang, et al., Highly efficient bacteria-infected diabetic wound healing employing a melanin-reinforced biopolymer hydrogel, Chem. Eng. J., 2023, 460, 141852 CrossRef CAS.
- I. Rani, S. G. Warkar and A. Kumar, Nano ZnO embedded poly (ethylene glycol) diacrylate cross-linked carboxymethyl tamarind kernel gum (CMTKG)/poly (sodium acrylate) composite hydrogels for oral delivery of ciprofloxacin drug and their antibacterial properties, Mater. Today Commun., 2023, 35, 105635 CrossRef CAS.
- E. Chiani, et al., Synthesis and characterization of gelatin/lignin hydrogels as quick release drug carriers for Ribavirin, Int. J. Biol. Macromol., 2023, 224, 1196–1205 CrossRef CAS PubMed.
- S. A. Shah, et al., Chitosan and carboxymethyl cellulose-based 3D multifunctional bioactive hydrogels loaded with nano-curcumin for synergistic diabetic wound repair, Int. J. Biol. Macromol., 2023, 227, 1203–1220 CrossRef CAS PubMed.
- M. Pourmadadi, et al., pH-responsive polyacrylic acid (PAA)-carboxymethyl cellulose (CMC) hydrogel
incorporating halloysite nanotubes (HNT) for controlled curcumin delivery, Ind. Crops Prod., 2023, 197, 116654 CrossRef CAS.
- M. Tanwar, R. K. Gupta and A. Rani, Natural gums and their derivatives based hydrogels: in biomedical, environment, agriculture, and food industry, Crit. Rev. Biotechnol., 2023, 1–27 CrossRef PubMed.
- H. Song, et al., Folic acid-chitosan conjugated nanoparticles for improving tumor-targeted drug delivery, BioMed Res. Int., 2013, 2013, 723158 Search PubMed.
- H. Bao, et al., Chitosan-functionalized graphene oxide as a nanocarrier for drug and gene delivery, Small, 2011, 7(11), 1569–1578 CrossRef CAS PubMed.
- C. Li, et al., Highly biocompatible multi-walled carbon nanotube–chitosan nanoparticle hybrids as protein carriers, Acta Biomater., 2011, 7(8), 3070–3077 CrossRef CAS.
- E. S. Lee, et al., A feasibility study of a pH sensitive nanomedicine using DOX loaded poly(aspartic acid-graft-imidazole)-block-poly(ethylene glycol) micelles, J. Mater. Chem. B, 2014, 2(9), 1152–1159 RSC.
- W. S. Abo-Elseoud, et al., Chitosan nanoparticles/cellulose nanocrystals nanocomposites as a carrier system for the controlled release of repaglinide, Int. J. Biol. Macromol., 2018, 111, 604–613 CrossRef CAS PubMed.
- T. Agarwal, et al., Calcium alginate-carboxymethyl cellulose beads for colon-targeted drug delivery, Int. J. Biol. Macromol., 2015, 75, 409–417 CrossRef CAS PubMed.
- K. Hansen, et al., Feasibility Investigation of Cellulose Polymers for Mucoadhesive Nasal Drug Delivery Applications, Mol. Pharmaceutics, 2015, 12, 2732–2741 CrossRef CAS PubMed.
- J. T. Orasugh, et al., Jute cellulose nano-fibrils/hydroxypropylmethylcellulose nanocomposite: a novel material with potential for application in packaging and transdermal drug delivery system, Ind. Crops Prod., 2018, 112, 633–643 CrossRef CAS.
- L. E. Low, et al., Magnetic cellulose nanocrystal stabilized Pickering emulsions for enhanced bioactive release and human colon cancer therapy, Int. J. Biol. Macromol., 2019, 127, 76–84 CrossRef CAS.
- K. Prusty and S. K. Swain, Release of ciprofloxacin drugs by nano gold embedded cellulose grafted polyacrylamide hybrid nanocomposite hydrogels, Int. J. Biol. Macromol., 2019, 126, 765–775 CrossRef CAS PubMed.
- Z. Rao, et al., Carboxymethyl cellulose modified graphene oxide as pH-sensitive drug delivery system, Int. J. Biol. Macromol., 2018, 107, 1184–1192 CrossRef CAS PubMed.
- L. Dai and C.-L. Si, Cellulose-graft-poly(methyl methacrylate) nanoparticles with high biocompatibility for hydrophobic anti-cancer drug delivery, Mater. Lett., 2017, 207, 213–216 CrossRef CAS.
- W. Qing, et al., The modified nanocrystalline cellulose for hydrophobic drug delivery, Appl. Surf. Sci., 2016, 366, 404–409 CrossRef CAS.
- R. Wang, et al., pH-Controlled drug delivery with hybrid aerogel of chitosan, carboxymethyl cellulose and graphene oxide as the carrier, Int. J. Biol. Macromol., 2017, 103, 248–253 CrossRef CAS PubMed.
- S. Javanbakht and H. Namazi, DOX loaded carboxymethyl cellulose/graphene quantum dot nanocomposite hydrogel films as a potential anticancer drug delivery system, Mater. Sci. Eng. C, 2018, 87, 50–59 CrossRef CAS PubMed.
- C. J. Wijaya, et al., Cellulose nanocrystals from passion fruit peels waste as antibiotic drug carrier, Carbohydr. Polym., 2017, 175, 370–376 CrossRef CAS PubMed.
- M. G. Bekaroğlu, Y. İşçi and S. İşçi, Colloidal properties and in vitro evaluation of Hydroxy ethyl cellulose coated iron oxide particles for targeted drug delivery, Mater. Sci. Eng. C, 2017, 78, 847–853 CrossRef PubMed.
- A. Solanki and S. Thakore, Cellulose crosslinked pH-responsive polyurethanes for drug delivery: α-hydroxy acids as drug release modifiers, Int. J. Biol. Macromol., 2015, 80, 683–691 CrossRef CAS PubMed.
- A. Melocchi, et al., Hot-melt extruded filaments based on pharmaceutical grade polymers for 3D printing by fused deposition modeling, Int. J. Pharm., 2016, 509(1–2), 255–263 CrossRef CAS PubMed.
- J. Boetker, et al., Modifying release characteristics from 3D printed drug-eluting products, Eur. J. Pharm. Sci., 2016, 90, 47–52 CrossRef CAS PubMed.
- A. Melocchi, et al., 3D printing by fused deposition modeling (FDM) of a swellable/erodible capsular device for oral pulsatile release of drugs, J. Drug Delivery Sci. Technol., 2015, 30, 360–367 CrossRef CAS.
- K. Pietrzak, A. Isreb and M. A. Alhnan, A flexible-dose dispenser for immediate and extended release 3D printed tablets, Eur. J. Pharm. Biopharm., 2015, 96, 380–387 CrossRef CAS PubMed.
- X. Chai, et al., Fused deposition modeling (FDM) 3D printed tablets for intragastric floating delivery of domperidone, Sci. Rep., 2017, 7(1), 1–9 CrossRef PubMed.
- D. Bhandari, et al., A Review on Bioactive Peptides: Physiological Functions, Bioavailability and Safety, Int. J. Pept. Res. Ther., 2020, 26(1), 139–150 CrossRef CAS.
- H. Xiao, et al., Acetylated starch nanocrystals: Preparation and antitumor drug delivery study, Int. J. Biol. Macromol., 2016, 89, 456–464 CrossRef CAS PubMed.
- R. Gul, et al., Functionalised nanostructures for transdermal delivery of drug cargos, J. Drug Targeting, 2018, 26(2), 110–122 CrossRef CAS PubMed.
- G. Garrait, E. Beyssac and M. Subirade, Development of a novel drug delivery system: chitosan nanoparticles entrapped in alginate microparticles, J. Microencapsulation, 2014, 31(4), 363–372 CrossRef CAS PubMed.
- J. K. Patra, et al., Nano based DDS: recent developments and future prospects, J. Nanobiotechnol., 2018, 16(1), 1–33 CrossRef.
- J. R. Costa, et al., Potential chitosan-coated alginate nanoparticles for ocular delivery of daptomycin, Eur. J. Clin. Microbiol. Infect. Dis., 2015, 34(6), 1255–1262 CrossRef CAS PubMed.
- G. Thakur, F. C. Rodrigues, and K. Singh, Crosslinking biopolymers for advanced drug delivery and tissue engineering applications, Cutting-Edge Enabling Technologies for Regenerative Medicine, 2018, pp. 213–231 Search PubMed.
- R. Sheshala, et al., Investigation on solution-to-gel characteristic of thermosensitive and mucoadhesive biopolymers for the development of moxifloxacin-loaded sustained release periodontal in situ gels, Drug Delivery Transl. Res., 2019, 9(2), 434–443 CrossRef CAS PubMed.
- K. Bai, et al., Selenium Nanoparticles-Embedded Chitosan Microspheres and Their Effects Upon Alcohol-Induced Gastric Mucosal Injury in Rats: Rapid Preparation, Oral Delivery, and Gastroprotective Potential of Selenium Nanoparticles, Int. J. Nanomed., 2020, 15, 1187–1203 CrossRef CAS.
- Y. Cong, et al., Ureido-modified carboxymethyl chitosan-graft-stearic acid polymeric nano-micelles as a targeted delivering carrier of clarithromycin for Helicobacter pylori: Preparation and in vitro evaluation, Int. J. Biol. Macromol., 2019, 129, 686–692 CrossRef CAS PubMed.
- J. L. Arias, L. H. Reddy and P. Couvreur, Fe3O4/chitosan nanocomposite for magnetic drug targeting to cancer, J. Mater. Chem., 2012, 22(15), 7622–7632 RSC.
- J. Wu, et al., Synthesis and characterization of mesoporous magnetic nanocomposites wrapped with chitosan gatekeepers for pH-sensitive controlled release of DOX, Mater. Sci. Eng. C, 2017, 70, 132–140 CrossRef CAS PubMed.
- J. Lin, et al., Drug/Dye-Loaded, Multifunctional PEG-Chitosan-Iron Oxide Nanocomposites for Methotraxate Synergistically Self-Targeted Cancer Therapy and Dual Model Imaging, ACS Appl. Mater. Interfaces, 2015, 7(22), 11908–11920 CrossRef CAS PubMed.
- Q. Yuan, S. Hein and R. D. K. Misra, New generation of chitosan-encapsulated ZnO quantum dots loaded with drug: Synthesis, characterization and in vitro drug delivery response, Acta Biomater., 2010, 6, 2732–2739 CrossRef CAS PubMed.
- M. Pooresmaeil, S. B. Nia and H. Namazi, Green encapsulation of LDH (Zn/Al)-5-Fu with carboxymethyl cellulose biopolymer; new nanovehicle for oral colorectal cancer treatment, Int. J. Biol. Macromol., 2019, 139, 994–1001 CrossRef CAS PubMed.
- Y. Ding, et al., Chitosan-based magnetic/fluorescent nanocomposites for cell labelling and controlled drug release, New J. Chem., 2017, 41(4), 1736–1743 RSC.
- S. Javanbakht, M. Pooresmaeil and H. Namazi, Green one-pot synthesis of carboxymethylcellulose/Zn-based metal-organic framework/graphene oxide bio-nanocomposite as a nanocarrier for drug delivery system, Carbohydr. Polym., 2019, 208, 294–301 CrossRef CAS PubMed.
- T. A. Debele, S. L. Mekuria and H.-C. Tsai, Synthesis and characterization of redox-sensitive heparin-β-sitosterol micelles: Their application as carriers for the pharmaceutical agent, DOX, and investigation of their antimetastatic activities in vitro, Mater. Sci. Eng. C, 2017, 75, 1326–1338 CrossRef CAS.
- V. A. Kuznetsov, et al., Graft copolymers of carboxymethyl cellulose with N-vinylimidazole: synthesis and application for drug delivery, Polym. Bull., 2019, 76(10), 4929–4949 CrossRef CAS.
- X. Sun, et al., pH-sensitive ZnO/carboxymethyl cellulose/chitosan bio-nanocomposite beads for colon-specific release of 5-fluorouracil, Int. J. Biol. Macromol., 2019, 128, 468–479 CrossRef CAS PubMed.
- C. W. Hsiao, et al., Photothermal tumor ablation in mice with repeated therapy sessions using NIR-absorbing micellar hydrogels formed in situ, Biomaterials, 2015, 56, 26–35 CrossRef CAS.
- J. Shi, et al., Schiff based injectable hydrogel for in situ pH-triggered delivery of DOX for breast tumor treatment, Polym. Chem., 2014, 5(21), 6180–6189 RSC.
- M. M. Silva, et al., Chitosan Nanoparticles as a Mucoadhesive Drug Delivery System for Ocular Administration, Mar. Drugs, 2017, 15(12) CrossRef CAS PubMed.
- S. Pistone, et al., Formulation of polysaccharide-based nanoparticles for local administration into the oral cavity, Eur. J. Pharm. Sci., 2017, 96, 381–389 CrossRef CAS.
- S. Liu, S. Yang and P. Ho, Intranasal Administration of Carbamazepine-loaded Carboxymethyl Chitosan Nanoparticles for Drug Delivery to the Brain, Asian J. Pharm. Sci., 2018, 13, 72–81 CrossRef.
- L. Yu, et al., A new hybrid nanocomposite prepared by graft copolymerization of butyl acrylate onto chitosan in the presence of organophilic montmorillonite, Radiat. Phys. Chem., 2004, 69, 467–471 CrossRef CAS.
- J.-M. Shen, et al., A novel carboxymethyl chitosan-based folate/Fe3O4/CdTe nanoparticle for targeted drug delivery and cell imaging, Carbohydr. Polym., 2012, 88(1), 239–249 CrossRef CAS.
- Y. Ding, et al., Design and construction of polymerized-chitosan coated Fe3O4 magnetic nanoparticles and its application for hydrophobic drug delivery, Mater. Sci. Eng., C, 2015, 48, 487–498 CrossRef CAS PubMed.
- X. Wang, Y. Du and J. Luo, Biopolymer/montmorillonite nanocomposite: preparation, drug-controlled release property and cytotoxicity, Nanotechnology, 2008, 19(6), 065707 CrossRef.
- B. Liu, et al., Alginate/quaternized carboxymethyl chitosan/clay nanocomposite microspheres: preparation and drug-controlled release behavior, J. Biomater. Sci., Polym. Ed., 2013, 24(5), 589–605 CrossRef CAS PubMed.
- U. Parida, et al., Synthesis and Characterization of Chitosan-Polyvinyl Alcohol Blended with Cloisite 30B for Controlled Release of the Anticancer Drug Curcumin, J. Biomater. Nanobiotechnol., 2011, 02, 414–425 CrossRef CAS.
- S. A. Agnihotri, S. S. Jawalkar and T. M. Aminabhavi, Controlled release of cephalexin through gellan gum beads: effect of formulation parameters on entrapment efficiency, size, and drug release, Eur. J. Pharm. Biopharm., 2006, 63(3), 249–261 CrossRef CAS PubMed.
- M. Xie, et al., Non-covalent modification of graphene oxide nanocomposites with chitosan/dextran and its application in drug delivery, RSC Adv., 2016, 6(11), 9328–9337 RSC.
- C.-L. Huang, et al., Application of paramagnetic graphene quantum dots as a platform for simultaneous dual-modality bioimaging and tumor-targeted drug delivery, J. Mater. Chem. B, 2015, 3(4), 651–664 RSC.
- F. Abedini, et al., Overview on natural hydrophilic polysaccharide polymers in drug delivery, Polym. Adv. Technol., 2018, 29(10), 2564–2573 CrossRef CAS.
- A. C. F. Patta, et al., Polyionic complexes of chitosan-N-arginine with alginate as pH responsive and mucoadhesive particles for oral drug delivery applications, Int. J. Biol. Macromol., 2020, 148, 550–564 CrossRef.
- S. Kumar, J. Ali, and S. Baboota, Polysaccharide nanoconjugates for drug solubilization and targeted delivery, Polysaccharide Carriers for Drug Delivery, 2019, pp. 443–475 Search PubMed.
- P. Chanphai and H. A. Tajmir-Riahi, DNA binding to folic acid-chitosan nanoconjugates, J. Biomol. Struct. Dyn., 2018, 36(10), 2746–2751 CrossRef CAS.
- S. W. Tsai, et al., Hyaluronan-cisplatin conjugate nanoparticles embedded in Eudragit S100-coated pectin/alginate microbeads for colon drug delivery, Int. J. Nanomed., 2013, 8, 2399–2407 CrossRef PubMed.
- Y.-H. Cheng, et al., Thermosensitive chitosan-based hydrogel as a topical ocular drug delivery system of latanoprost for glaucoma treatment, Carbohydr. Polym., 2016, 144, 390–399 CrossRef CAS.
- Y. Cao, et al., Poly(N-isopropylacrylamide)-chitosan as thermosensitive in situ gel-forming system for ocular drug delivery, J. Controlled Release, 2007, 120(3), 186–194 CrossRef CAS PubMed.
- S. Yu, et al., A novel pH-induced thermosensitive hydrogel composed of carboxymethyl chitosan and poloxamer cross-linked by glutaraldehyde for ophthalmic drug delivery, Carbohydr. Polym., 2017, 155, 208–217 CrossRef CAS PubMed.
- H. S. Adhikari and P. N. Yadav, Anticancer Activity of Chitosan, Chitosan Derivatives, and Their Mechanism of Action, Int. J. Biomater., 2018, 2018, 2952085 Search PubMed.
- F. Q. Hu, et al., Synthesis and antitumor activity of DOX conjugated stearic acid-g-chitosan oligosaccharide polymeric micelles, Biomaterials, 2009, 30(36), 6955–6963 CrossRef CAS PubMed.
- J. Hyung Park, et al., Self-assembled nanoparticles based on glycol chitosan bearing hydrophobic moieties as carriers for DOX: in vivo biodistribution and anti-tumor activity, Biomaterials, 2006, 27(1), 119–126 CrossRef PubMed.
- Y. J. Son, et al., Biodistribution
and anti-tumor efficacy of DOX loaded glycol-chitosan nanoaggregates by EPR effect, J. Controlled Release, 2003, 91(1), 135–145 CrossRef CAS PubMed.
- E. Lee, et al., Conjugated chitosan as a novel platform for oral delivery of paclitaxel, J. Med. Chem., 2008, 51(20), 6442–6449 CrossRef CAS PubMed.
- E. Lee, et al., In vivo antitumor effects of chitosan-conjugated docetaxel after oral administration, J. Controlled Release, 2009, 140(2), 79–85 CrossRef CAS PubMed.
- M. Xie, et al., Layer-by-layer modification of magnetic graphene oxide by chitosan and sodium alginate with enhanced dispersibility for targeted drug delivery and photothermal therapy, Colloids Surf., B, 2019, 176, 462–470 CrossRef CAS PubMed.
- M. Yadollahi, S. Farhoudian and H. Namazi, One-pot synthesis of antibacterial chitosan/silver bio-nanocomposite hydrogel beads as DDS, Int. J. Biol. Macromol., 2015, 79, 37–43 CrossRef CAS PubMed.
- Y. K. Manea, et al., Ciprofloxacin-supported chitosan/polyphosphate nanocomposite to bind bovine serum albumin: Its application in drug delivery, J. Mol. Liq., 2019, 292, 111337 CrossRef.
- S. Hosseinzadeh, et al., Synthesis of stimuli-responsive chitosan nanocomposites via RAFT copolymerization for DOX delivery, Int. J. Biol. Macromol., 2019, 121, 677–685 CrossRef PubMed.
- Z. Shariatinia and Z. Zahraee, Controlled release of metformin from chitosan-based nanocomposite films containing mesoporous MCM-41 nanoparticles as novel DDS, J. Colloid Interface Sci., 2017, 501, 60–76 CrossRef CAS PubMed.
- M. Jia, et al., Development of both methotrexate and mitomycin C loaded PEGylated chitosan nanoparticles for targeted drug codelivery and synergistic anticancer effect, ACS Appl. Mater. Interfaces, 2014, 6(14), 11413–11423 CrossRef CAS.
- Q. S. Zhao, et al., Preparation of alginate coated chitosan hydrogel beads by thermosensitive internal gelation technique, J. Sol-Gel Sci. Technol., 2010, 54(2), 232–237 CrossRef CAS.
- M. Tavakol, E. Vasheghani-Farahani and S. Hashemi-Najafabadi, The effect of polymer and CaCl(2) concentrations on the sulfasalazine release from alginate-N,O-carboxymethyl chitosan beads, Prog. Biomater., 2013, 2(1), 10 CrossRef.
- V. K. Malesu, D. Sahoo and P. Nayak, Chitosan–sodium alginate nanocomposites blended with cloisite 30B as a novel drug delivery system for anticancer drug curcumin, Int. J. Appl. Biol. Pharm. Technol., 2011, 162176 Search PubMed.
- A. A. Elzatahry, et al., Evaluation of alginate–chitosan bioadhesive beads as a drug delivery system for the controlled release of theophylline, J. Appl. Polym. Sci., 2009, 111(5), 2452–2459 CrossRef CAS.
- T. W. Wong, et al., Design of controlled-release solid dosage forms of alginate and chitosan using microwave, J. Controlled Release, 2002, 84(3), 99–114 CrossRef CAS.
- M. de la Fuente, B. Seijo and M. J. Alonso, Novel hyaluronic acid-chitosan nanoparticles for ocular gene therapy, Invest. Ophthalmol. Visual Sci., 2008, 49(5), 2016–2024 CrossRef.
- L. Contreras-Ruiz, et al., Ocular tolerance to a topical formulation of hyaluronic acid and chitosan-based nanoparticles, Cornea, 2010, 29(5), 550–558 CrossRef PubMed.
- F. A. Oyarzun-Ampuero, et al., Chitosan-hyaluronic acid nanoparticles loaded with heparin for the treatment of asthma, Int. J. Pharm., 2009, 381(2), 122–129 CrossRef CAS.
- H. D. Lu, et al., Novel hyaluronic acid-chitosan nanoparticles as non-viral gene delivery vectors targeting osteoarthritis, Int. J. Pharm., 2011, 420(2), 358–365 CrossRef CAS PubMed.
- M. Fukuda, N. A. Peppas and J. W. McGinity, Properties of sustained release hot-melt extruded tablets containing chitosan and xanthan gum, Int. J. Pharm., 2006, 310(1), 90–100 CrossRef CAS.
- T. Phaechamud and G. C. Ritthidej, Sustained-release from Layered Matrix System Comprising Chitosan and Xanthan Gum, Drug Dev. Ind. Pharm., 2007, 33(6), 595–605 CrossRef CAS PubMed.
- A. M. Pandele, et al., New biocompatible mesoporous silica/polysaccharide hybrid materials as possible DDS, Materials, 2019, 12(1), 15 CrossRef CAS.
- Y. Song, et al., In situ formation of injectable chitosan-gelatin hydrogels through double crosslinking for sustained intraocular drug delivery, Mater. Sci. Eng. C, 2018, 88, 1–12 CrossRef CAS PubMed.
- J. Wei, et al., Multi-Stimuli-Responsive Microcapsules for Adjustable Controlled-Release, Adv. Funct. Mater., 2014, 24(22), 3312–3323 CrossRef CAS.
- K. Ganguly, A. R. Kulkarni and T. M. Aminabhavi, In vitro cytotoxicity and in vivo efficacy of 5-fluorouracil-loaded enteric-coated
PEG-crosslinked chitosan microspheres in colorectal cancer therapy in rats, Drug Delivery, 2015, 1–14 CrossRef.
- S. Shafiee, H. A. Ahangar and A. Saffar, Taguchi method optimization for synthesis of Fe3O4 @chitosan/Tragacanth Gum nanocomposite as a drug delivery system, Carbohydr. Polym., 2019, 222, 114982 CrossRef CAS PubMed.
- M. Yadollahi, et al., Facile synthesis of chitosan/ZnO bio-nanocomposite hydrogel beads as DDS, Int. J. Biol. Macromol., 2016, 82, 273–278 CrossRef CAS PubMed.
- X. Cheng, et al., Surface-fluorinated and pH-sensitive carboxymethyl chitosan nanoparticles to overcome biological barriers for improved drug delivery in vivo, Carbohydr. Polym., 2019, 208, 59–69 CrossRef CAS PubMed.
- F. Luo, et al., pH-responsive stearic acid-O-carboxymethyl chitosan assemblies as carriers delivering small molecular drug for chemotherapy, Mater. Sci. Eng. C, 2019, 105, 110107 CrossRef CAS PubMed.
- C. Zhang, et al., Targeted antigen delivery to dendritic cell via functionalized alginate nanoparticles for cancer immunotherapy, J. Controlled Release, 2017, 256, 170–181 CrossRef CAS PubMed.
- J. Venkatesan, et al., Antimicrobial and anticancer activities of porous chitosan-alginate biosynthesized silver nanoparticles, Int. J. Biol. Macromol., 2017, 98, 515–525 CrossRef CAS.
- S. Rahaiee, et al., Nanoparticles based on crocin loaded chitosan-alginate biopolymers: Antioxidant activities, bioavailability and anticancer properties, Int. J. Biol. Macromol., 2017, 99, 401–408 CrossRef CAS PubMed.
- S. Maity, et al., Alginate coated chitosan core-shell nanoparticles for efficient oral delivery of naringenin in diabetic animals—An in vitro and in vivo approach, Carbohydr. Polym., 2017, 170, 124–132 CrossRef CAS PubMed.
- D. Aluani, et al., Evaluation of biocompatibility and antioxidant efficiency of chitosan-alginate nanoparticles loaded with quercetin, Int. J. Biol. Macromol., 2017, 103, 771–782 CrossRef CAS.
- P. Mukhopadhyay, et al., pH-sensitive chitosan/alginate core-shell nanoparticles for efficient and safe oral insulin delivery, Int. J. Biol. Macromol., 2015, 72, 640–648 CrossRef CAS PubMed.
- N. P. Katuwavila, et al., Chitosan-alginate nanoparticle system efficiently delivers DOX to MCF-7 cells, J. Nanomater., 2016, 2016, 3178904 Search PubMed.
- Z.-H. Zhang, et al., N-Octyl-N-Arginine Chitosan Micelles as an Oral Delivery System of Insulin, J. Biomed. Nanotechnol., 2013, 9(4), 601–609 CrossRef CAS PubMed.
- M. L. González-Rodriguez, et al., Alginate/chitosan particulate systems for sodium diclofenac release, Int. J. Pharm., 2002, 232(1), 225–234 CrossRef.
- G. Pasparakis and N. Bouropoulos, Swelling studies and in vitro release of verapamil from calcium alginate and calcium alginate-chitosan beads, Int. J. Pharm., 2006, 323(1–2), 34–42 CrossRef CAS.
- S. Wittaya-areekul, J. Kruenate and C. Prahsarn, Preparation and in vitro evaluation of mucoadhesive properties of alginate/chitosan microparticles containing prednisolone, Int. J. Pharm., 2006, 312(1), 113–118 CrossRef CAS PubMed.
- S. C. Angadi, L. S. Manjeshwar and T. M. Aminabhavi, Novel composite blend microbeads of sodium alginate coated with chitosan for controlled release of amoxicillin, Int. J. Biol. Macromol., 2012, 51(1), 45–55 CrossRef CAS PubMed.
- L. Gao, et al., Evaluation of genipin-crosslinked chitosan hydrogels as a potential carrier for silver sulfadiazine nanocrystals, Colloids Surf., B, 2016, 148, 343–353 CrossRef CAS PubMed.
- K. Sharma, V. Mahalwal and A. Arora, Natural biodegradable polymers as matrices in transdermal drug delivery, Int. J. Drug Dev. Res., 2011, 3, 85–103 CAS.
- M. Sano, et al., Chitosan Gel for Transdermal Delivery of Morphine Hydrochloride, Iryo Yakugaku, 2007, 33(6), 515–519 CAS.
- H. Dureja, A. K. Tiwary and S. Gupta, Simulation of skin permeability in chitosan membranes, Int. J. Pharm., 2001, 213(1), 193–198 CrossRef CAS.
- V. H. Kulkarni, et al., Transdermal delivery of antiasthmatic drug through modified chitosan membrane, Indian J. Pharm. Sci., 2005, 67, 544–547 CAS.
- J. R. Fried, Polymer science and technology, Pearson Education, 2014 Search PubMed.
- S. Chen, et al., Intestinal glucuronidation protects against chemotherapy-induced toxicity by irinotecan (CPT-11), Proc. Natl. Acad. Sci. U. S. A., 2013, 110(47), 19143–19148 CrossRef CAS PubMed.
- T. S. Anirudhan, S. S. Nair and A. S. Nair, Fabrication of a bioadhesive transdermal device from chitosan and hyaluronic acid for the controlled release of lidocaine, Carbohydr. Polym., 2016, 152, 687–698 CrossRef CAS.
- A. J. Shinde, A. L. Shinde and H. N. More, Design and evaluation of transdermal drug delivery system of gliclazide, Asian J. Pharm., 2014, 4(2), 201–211 Search PubMed.
- S. F. Taveira, A. Nomizo and R. F. V. Lopez, Effect of the iontophoresis of a chitosan gel on DOX skin penetration and cytotoxicity, J. Controlled Release, 2009, 134(1), 35–40 CrossRef CAS PubMed.
- J. J. Escobar-Chávez, et al., Transdermal nortriptyline hydrocloride patch formulated within a chitosan matrix intended to be used for smoking cessation, Pharm. Dev. Technol., 2011, 16(2), 162–169 CrossRef.
- G. Banche, et al., Antimicrobial chitosan nanodroplets: new insights for ultrasound-mediated adjuvant treatment of skin infection, Future Microbiol., 2015, 10(6), 929–939 CrossRef CAS PubMed.
- S. Indulekha, et al., Thermoresponsive polymeric gel as an on-demand transdermal drug delivery system for pain management, Mater. Sci. Eng. C, 2016, 62, 113–122 CrossRef CAS.
- H.-N. Huang, et al., Transdermal immunization with low-pressure-gene-gun mediated chitosan-based DNA vaccines against Japanese encephalitis virus, Biomaterials, 2009, 30(30), 6017–6025 CrossRef CAS PubMed.
- L. Sadhasivam, et al., Transdermal patches of chitosan nanoparticles for insulin delivery, Int. J. Pharm. Pharm. Sci., 2015, 7, 84–88 CAS.
- J.-g. Gao, et al., Preparation of chitosan microspheres loading of 3,5-dihydroxy-4-i-propylstilbene and in vitro release, J. Polym. Res., 2011, 18(6), 1501–1508 CrossRef CAS.
- J. Kim, A. Lund and C. Dombrowski, Telling the story in big data, Interactions, 2013, 20(3), 48–51 CrossRef.
- N. Charernsriwilaiwat, et al., Preparation of Chitosan-Thiamine Pyrophosphate/Polyvinyl Alcohol Blend Electrospun Nanofibers, Adv. Mater. Res., 2012, 506, 118–121 CAS.
- R. V. N. Gundloori, A. Singam, and N. Killi, Chapter 19 – Nanobased Intravenous and Transdermal DDS, in Applications of Targeted Nano Drugs and Delivery Systems, ed. S.S. Mohapatra, et al., Elsevier, 2019, pp. 551–594 Search PubMed.
- R. T. Allena, et al., Preparation and evaluation of transdermal patches of metformin hydrochloride using natural polymer for sustained release, Int. J. Pharm. Pharm. Sci., 2012, 4(3), 297–302 CAS.
- V. Bhat, et al., Influence of blending of chitosan and pullulan on their drug release behavior: an in-vitro study research article, 2012 Search PubMed.
- Y. Xie, B. Xu and Y. Gao, Controlled transdermal delivery of model drug compounds by MEMS microneedle array, Nanomed.: Nanotechnol. Biol. Med., 2005, 1(2), 184–190 CrossRef CAS PubMed.
- K. Siddhapura, H. Harde and S. Jain, Immunostimulatory effect of tetanus toxoid loaded chitosan nanoparticles following microneedles assisted immunization, Nanomed.: Nanotechnol. Biol. Med., 2016, 12(1), 213–222 CrossRef CAS PubMed.
- X. Ling, et al., Synthesis and characterization of hyaluronic acid–platinum(iv) nanoconjugate with enhanced antitumor response and reduced adverse effects, RSC Adv., 2015, 5(99), 81668–81681 RSC.
- F. Li and K. Na, Self-Assembled Chlorin e6 Conjugated Chondroitin Sulfate Nanodrug for Photodynamic Therapy, Biomacromolecules, 2011, 12(5), 1724–1730 CrossRef CAS PubMed.
- H. Onishi, Y. Isoda and M. Matsuyama, In vivo evaluation of chondroitin sulfate-glycyl-prednisolone for anti-arthritic effectiveness and pharmacokinetic characteristics, Int. J. Pharm., 2013, 456(1), 113–120 CrossRef CAS PubMed.
- C. Yu, et al., Redox-responsive shell-sheddable micelles self-assembled from amphiphilic chondroitin sulfate-cholesterol conjugates for triggered intracellular drug release, Chem. Eng. J., 2013, 228, 290–299 CrossRef CAS.
- C. Yu, et al., Facile preparation of pH-sensitive micelles self-assembled from amphiphilic chondroitin sulfate-histamine conjugate for triggered intracellular drug release, Colloids Surf., B, 2014, 115, 331–339 CrossRef CAS.
- A. Jain, et al., Targeting of diacerein loaded lipid nanoparticles to intra-articular cartilage using chondroitin sulfate as homing carrier for treatment of osteoarthritis in rats, Nanomed.: Nanotechnol. Biol. Med., 2014, 10(5), e1031–e1040 CrossRef PubMed.
- A. Nawaz and T. W. Wong, Quantitative characterization of chitosan in the skin by Fourier-transform infrared spectroscopic imaging and ninhydrin assay: application in transdermal sciences, J. Microsc, 2016, 263(1), 34–42 CrossRef CAS PubMed.
- Y. Ramesh and V. Sireesha, Transdermal patch of ramipril loaded chitosan nanoparticles dispersed in carbopol gel, J. Drug Delivery Ther., 2017, 7, 56–65 CAS.
- S. Maiti and S. Mukherjee, Controlled drug delivery attributes of co-polymer micelles and xanthan-O-carboxymethyl hydrogel particles, Int. J. Biol. Macromol., 2014, 70, 37–43 CrossRef CAS PubMed.
- N. Peng, et al., Amphiphilic hexadecyl-quaternized chitin micelles for DOX delivery, Int. J. Biol. Macromol., 2019, 130, 615–621 CrossRef CAS.
- C. Cao, et al., Correlation between Drug Loading Content and Biological Activity: The Complexity Demonstrated in Paclitaxel-Loaded Glycopolymer Micelle System, Biomacromolecules, 2019, 20(4), 1545–1554 CrossRef CAS PubMed.
- J. D. Schneible, M. A. Daniele, and S. Menegatti, Natural and Synthetic Biopolymers in Drug Delivery and Tissue Engineering, Biopolymers for Biomedical and Biotechnological Applications, 2021, pp. 265–356 Search PubMed.
- S. Cai, et al., Localized DOX chemotherapy with a biopolymeric nanocarrier improves survival and reduces toxicity in xenografts of human breast cancer, J. Controlled Release, 2010, 146(2), 212–218 CrossRef CAS PubMed.
- I. M. Montagner, et al., Paclitaxel-hyaluronan hydrosoluble bioconjugate: Mechanism of action in human bladder cancer cell lines, Urol. Oncol.: Semin. Orig. Invest., 2013, 31(7), 1261–1269 CrossRef CAS PubMed.
- L. K. Widjaja, et al., Hyaluronic acid-based nanocomposite hydrogels for ocular drug delivery applications, J. Biomed. Mater. Res., Part A, 2014, 102(9), 3056–3065 CrossRef PubMed.
- Y. Wu, et al., Enhanced and sustained topical ocular delivery of cyclosporine A in thermosensitive hyaluronic acid-based in situ forming microgels, Int. J. Nanomed., 2013, 8, 3587–3601 Search PubMed.
- Y. Zhong, et al., Reversibly crosslinked hyaluronic acid nanoparticles for active targeting and intelligent delivery of DOX to drug resistant CD44+ human breast tumor xenografts, J. Controlled Release, 2015, 205, 144–154 CrossRef CAS.
- J. L. Holloway, et al., Modulating hydrogel crosslink density and degradation to control bone morphogenetic protein delivery and in vivo bone formation, J. Controlled Release, 2014, 191, 63–70 CrossRef CAS PubMed.
- G. Bajaj, et al., Hyaluronic acid-based hydrogel for regional delivery of paclitaxel to intraperitoneal tumors, J. Controlled Release, 2012, 158(3), 386–392 CrossRef CAS PubMed.
- E. J. Cho, et al., Intraperitoneal delivery of platinum with in-situ crosslinkable hyaluronic acid gel for local therapy of ovarian cancer, Biomaterials, 2015, 37, 312–319 CrossRef CAS PubMed.
- K. Ueda, et al., Growth inhibitory effect of an injectable hyaluronic acid–tyramine hydrogels incorporating human natural interferon-α and sorafenib on renal cell carcinoma cells, Acta Biomater., 2016, 29, 103–111 CrossRef CAS PubMed.
- H. L. Mao, et al., Delivery of DOX from Hyaluronic Acid-Modified Glutathione-Responsive Ferrocene Micelles for Combination Cancer Therapy, Mol. Pharm., 2019, 16(3), 987–994 CrossRef CAS.
- P. Wu, et al., Enhanced anti-tumor efficacy of hyaluronic acid modified nanocomposites combined with sonochemotherapy against subcutaneous and metastatic breast tumors, Nanoscale, 2019, 11(24), 11470–11483 RSC.
- G. Wang, et al., Theranostic Hyaluronic Acid–Iron Micellar Nanoparticles for Magnetic-Field-Enhanced in vivo Cancer Chemotherapy, ChemMedChem, 2018, 13(1), 78–86 CrossRef CAS.
- Z. Pan, H. Ye and D. Wu, Recent advances on polymeric hydrogels as wound dressings, APL Bioeng., 2021, 5(1), 011504 CrossRef CAS PubMed.
- X. Pang, et al., Hyaluronic acid-quercetin conjugate micelles: Synthesis, characterization, in vitro and in vivo evaluation, Colloids Surf., B, 2014, 123, 778–786 CrossRef CAS PubMed.
- J. Li, et al., Modified curcumin with hyaluronic acid: Combination of pro-drug and nano-micelle strategy to address the curcumin challenge, Food Res. Int., 2015, 69, 202–208 CrossRef CAS.
- C. Tian, et al., The effect of the molecular weight of hyaluronic acid on the physicochemical characterization of hyaluronic acid-curcumin conjugates and in vitro evaluation in glioma cells, Colloids Surf., B, 2018, 165, 45–55 CrossRef CAS PubMed.
- Z. Cheng, et al., Preparation and characterization of dissolving hyaluronic acid composite microneedles loaded micelles for delivery of curcumin, Drug Delivery Transl. Res., 2020, 10(5), 1520–1530 CrossRef CAS PubMed.
- S. C. Abeylath and M. M. Amiji, ‘Click’ synthesis of dextran macrostructures for combinatorial-designed self-assembled nanoparticles encapsulating diverse anticancer therapeutics, Bioorg. Med. Chem., 2011, 19(21), 6167–6173 CrossRef CAS PubMed.
- E. M. Kemper, et al., Increased penetration of paclitaxel into the brain by inhibition of P-Glycoprotein, Clin. Cancer Res., 2003, 9(7), 2849–2855 CAS.
- D. Coradini, et al., Hyaluronic acid as drug delivery for sodium butyrate: Improvement of the anti-proliferative activity on a breast-cancer cell line, Int. J. Cancer, 1999, 81(3), 411–416 CrossRef CAS PubMed.
- S. Cai, et al., Phase I-II clinical trial of hyaluronan-cisplatin nanoconjugate in dogs with naturally occurring malignant tumors, Am. J. Vet. Res., 2016, 77(9), 1005–1016 CAS.
- P. F. Bassi, et al., Paclitaxel-Hyaluronic Acid for Intravesical Therapy of Bacillus Calmette-Guérin Refractory Carcinoma In Situ of the Bladder: Results of a Phase I Study, J. Urol., 2011, 185(2), 445–449 CrossRef CAS PubMed.
- A. Rosato, et al., HYTAD1-p20: A new paclitaxel-hyaluronic acid hydrosoluble bioconjugate for treatment of superficial bladder cancer, Urol. Oncol.: Semin. Orig. Invest., 2006, 24(3), 207–215 CrossRef CAS.
- Y. J. Jin, et al., Hyaluronic acid derivative-based self-assembled nanoparticles for the treatment of melanoma, Pharm. Res., 2012, 29(12), 3443–3454 CrossRef CAS PubMed.
- H. Shen, et al., Coating Solid Lipid Nanoparticles with Hyaluronic Acid Enhances Antitumor Activity against Melanoma Stem-like Cells, Theranostics, 2015, 5(7), 755–771 CrossRef CAS.
- H. S. Jung, et al., Nanographene Oxide–Hyaluronic Acid Conjugate for Photothermal Ablation Therapy of Skin Cancer, ACS Nano, 2014, 8(1), 260–268 CrossRef CAS PubMed.
- M. Kong, et al., Construction of hyaluronic acid noisome as functional transdermal nanocarrier for tumor therapy, Carbohydr. Polym., 2013, 94(1), 634–641 CrossRef CAS PubMed.
- L. Hou and M. Kong, Enhanced transdermal lymphatic drug delivery of hyaluronic acid modified transfersome for tumor metastasis therapy, J. Controlled Release, 2015, 213, e77 CrossRef PubMed.
- P. Lee, et al., Influence of chondroitin sulfate and hyaluronic acid presence in nanofibers and its alignment on the bone marrow stromal cells: cartilage regeneration, J. Biomed. Nanotechnol., 2014, 10(8), 1469–1479 CrossRef CAS PubMed.
- D. Šmejkalová, et al., Hyaluronan polymeric micelles for topical drug delivery, Carbohydr. Polym., 2017, 156, 86–96 CrossRef PubMed.
- J.-A. Yang, et al., Transdermal delivery of hyaluronic acid – Human growth hormone conjugate, Biomaterials, 2012, 33(25), 5947–5954 CrossRef CAS.
- A. Ebringerová and Z. Hromádková, Xylans of Industrial and Biomedical Importance, Biotechnol. Genet. Eng. Rev., 1999, 16(1), 325–346 CrossRef PubMed.
- S. U. Kumar, P. Gopinath and Y. S. Negi, Synthesis and bio-evaluation of xylan-5-fluorouracil-1-acetic acid conjugates as prodrugs for colon cancer treatment, Carbohydr. Polym., 2017, 157, 1442–1450 CrossRef.
- S. U. Kumar, et al., pH-responsive prodrug nanoparticles based on xylan-curcumin conjugate for the efficient delivery of curcumin in cancer therapy, Carbohydr. Polym., 2018, 188, 252–259 CrossRef.
- S. C. da Costa Urtiga, et al., Xylan in drug delivery: a review of its engineered structures and biomedical applications, Eur. J. Pharm. Biopharm., 2020, 151, 199–208 CrossRef.
- P. Severino, et al., Alginate nanoparticles for drug delivery and targeting, Curr. Pharm. Des., 2019, 25(11), 1312–1334 CrossRef CAS PubMed.
- M. Upadhyay, et al., Development of biopolymers based interpenetrating polymeric network of capecitabine: a drug delivery vehicle to extend the release of the model drug, Int. J. Biol. Macromol., 2018, 115, 907–919 CrossRef CAS PubMed.
- S. Haque, et al., Development and evaluation of brain targeted intranasal alginate nanoparticles for treatment of depression, J. Psychiatr. Res., 2014, 48(1), 1–12 CrossRef.
- S. Mandal, et al., Formulation and evaluation of an in situ gel-forming ophthalmic formulation of moxifloxacin hydrochloride, Int. J. Pharm. Invest., 2012, 2(2), 78–82 CrossRef CAS.
- D. Bharathi, et al., Long acting ophthalmic formulation of indomethacin: Evaluation of alginate gel systems, Indian J. Pharm. Sci., 2007, 69(1), 37 CrossRef.
- J. V. Román, M. A. Galán and E. M. M. del Valle, Preparation and preliminary evaluation of alginate crosslinked microcapsules as potential drug delivery system (DDS) for human lung cancer therapy, Biomed. Phys. Eng. Express, 2016, 2(3), 035015 CrossRef.
- M. Rajaonarivony, et al., Development of a new drug carrier made from alginate, J. Pharm. Sci., 1993, 82(9), 912–917 CrossRef CAS PubMed.
- I. K. Park, et al., Galactosylated chitosan-graft-dextran as hepatocyte-targeting DNA carrier, J. Controlled Release, 2000, 69(1), 97–108 CrossRef CAS PubMed.
- J. Wei, et al., Hollow hydroxyapatite/polyelectrolyte hybrid microparticles with controllable size, wall thickness and drug delivery properties, J. Mater. Chem. B, 2015, 3(41), 8162–8169 RSC.
- T. S. Anirudhan, C. Sekhar V and S. S. Nair, Polyelectrolyte complexes of carboxymethyl chitosan/alginate based drug carrier for targeted and controlled release of dual drug, J. Drug Delivery Sci. Technol., 2019, 51, 569–582 CrossRef CAS.
- K. Ghosal, et al., Synthesis and characterization of interpenetrating polymeric networks based bio-composite alginate film: A well-designed drug delivery platform, Int. J. Biol. Macromol., 2019, 130, 645–654 CrossRef CAS PubMed.
- H. Yan, et al., Entrapment of bacterial cellulose nanocrystals stabilized Pickering emulsions droplets in alginate beads for hydrophobic drug delivery, Colloids Surf., B, 2019, 177, 112–120 CrossRef CAS PubMed.
- K. S. Joshy, et al., Encapsulation of zidovudine in PF-68 coated alginate conjugate nanoparticles for anti-HIV drug delivery, Int. J. Biol. Macromol., 2018, 107, 929–937 CrossRef CAS PubMed.
- Y. Shtenberg, et al., Mucoadhesive alginate pastes with embedded liposomes for local oral drug delivery, Int. J. Biol. Macromol., 2018, 111, 62–69 CrossRef CAS PubMed.
- Z.-C. Yin, Y.-L. Wang and K. Wang, A pH-responsive composite hydrogel beads based on agar and alginate for oral drug delivery, J. Drug Delivery Sci. Technol., 2018, 43, 12–18 CrossRef CAS.
- F. Wang, S. He and B. Chen, Retinoic acid-loaded alginate microspheres as a slow release drug delivery carrier for intravitreal treatment, Biomed. Pharmacother., 2018, 97, 722–728 CrossRef CAS.
- M. Xie, et al., Surface modification of graphene oxide nanosheets by protamine sulfate/sodium alginate for anti-cancer drug delivery application, Appl. Surf. Sci., 2018, 440, 853–860 CrossRef CAS.
- B. Zhang, et al., A colon targeted drug delivery system based on alginate modificated graphene oxide for colorectal liver metastasis, Mater. Sci. Eng. C, 2017, 79, 185–190 CrossRef CAS PubMed.
- S. M. H. Dabiri, et al., New in-situ synthetized hydrogel composite based on alginate and brushite as a potential pH sensitive drug delivery system, Carbohydr. Polym., 2017, 177, 324–333 CrossRef CAS.
- P. Bonilla, et al., Influence of crosslinked alginate on drug release from highly concentrated emulsions, Colloids Surf., A, 2018, 536, 148–155 CrossRef CAS.
- S. M. Tawfik, et al., Naturally modified nonionic alginate functionalized upconversion nanoparticles for the highly efficient targeted pH-responsive drug delivery and enhancement of NIR-imaging, J. Ind. Eng. Chem., 2018, 57, 424–435 CrossRef CAS.
- C. C. Ribeiro, C. C. Barrias and M. A. Barbosa, Calcium phosphate-alginate microspheres as enzyme delivery matrices, Biomaterials, 2004, 25(18), 4363–4373 CrossRef CAS PubMed.
- B. D. Kevadiya, et al., Montmorillonite-Alginate Nanocomposites as a Drug Delivery System: Intercalation and In Vitro Release of Vitamin B1 and Vitamin B6, J. Biomater. Appl., 2010, 25(2), 161–177 CrossRef CAS PubMed.
- J. Malakar and A. K. Nayak, Formulation and statistical optimization of multiple-unit ibuprofen-loaded buoyant system using 23-factorial design, Chem. Eng. Res. Des., 2012, 90(11), 1834–1846 CrossRef CAS.
- H. Bera, et al., Alginate gel-coated oil-entrapped alginate–tamarind gum–magnesium stearate buoyant beads of risperidone, Int. J. Biol. Macromol., 2015, 78, 102–111 CrossRef CAS.
- Y. Javadzadeh, et al., Evaluation of drug release kinetics and physico-chemical characteristics of metronidazole floating beads based on calcium silicate and gas-forming agents, Pharm. Dev. Technol., 2010, 15(4), 329–338 CrossRef CAS PubMed.
- R. H. Fahmy, Statistical Approach for Assessing the Influence of Calcium Silicate and HPMC on the Formulation of Novel Alfuzosin Hydrochloride Mucoadhesive-Floating Beads as Gastroretentive DDS, AAPS PharmSciTech, 2012, 13(3), 990–1004 CrossRef CAS.
- F. F. Azhar and A. Olad, A study on sustained release formulations for oral delivery of 5-fluorouracil based on alginate–chitosan/montmorillonite nanocomposite systems, Appl. Clay Sci., 2014, 101, 288–296 CrossRef.
- Y. Zhang, et al., A DOX delivery system: Samarium/mesoporous bioactive glass/alginate composite microspheres, Mater. Sci. Eng. C, 2016, 67, 205–213 CrossRef CAS PubMed.
- J.-L. Wu, et al., Multi-drug delivery system based on alginate/calcium carbonate hybrid nanoparticles for combination chemotherapy, Colloids Surf., B, 2014, 123, 498–505 CrossRef CAS PubMed.
- H. Li, et al., Bioactive apatite incorporated alginate microspheres with sustained drug-delivery for bone regeneration application, Mater. Sci. Eng. C, 2016, 62, 779–786 CrossRef CAS PubMed.
- B. D. Kevadiya, et al., Montmorillonite-Alginate Composites as a Drug delivery System: Intercalation and In vitro Release of Diclofenac sodium, Indian J. Pharm. Sci., 2010, 72(6), 732–737 CrossRef CAS PubMed.
- D. Pal and A. K. Nayak, Development, Optimization, and Anti-diabetic Activity of Gliclazide-Loaded Alginate–Methyl Cellulose Mucoadhesive Microcapsules, AAPS PharmSciTech, 2011, 12(4), 1431–1441 CrossRef CAS PubMed.
- A. Nayak, et al., Development of alginate-PVP K 30 microbeads for controlled diclofenac sodium delivery using central composite design, Daru, J. Fac. Pharm., 2011, 19, 356–366 CAS.
- M. S. Hasnain, et al., Isolation and characterization of Linum usitatisimum polysaccharide to prepare mucoadhesive beads of diclofenac sodium, Int. J. Biol. Macromol., 2018, 116, 162–172 CrossRef PubMed.
- P. Sinha, et al., Alginate-okra gum blend beads of diclofenac sodium from aqueous template using ZnSO4 as a cross-linker, Int. J. Biol. Macromol., 2015, 79, 555–563 CrossRef CAS PubMed.
- A. K. Nayak, et al., Mucoadhesive beads of gliclazide: Design, development, and evaluation, ScienceAsia, 2010, 36(1), 319–325 CrossRef CAS.
- A. K. Nayak, D. Pal and K. Santra, Plantago ovata F. Mucilage-alginate mucoadhesive beads for controlled release of glibenclamide: development, optimization, and in vitro-in vivo evaluation, J. Pharm, 2013, 2013, 151035 Search PubMed.
- D. Pal and A. K. Nayak, Novel tamarind seed polysaccharide-alginate mucoadhesive microspheres for oral gliclazide delivery: in vitro–in vivo evaluation, Drug Delivery, 2012, 19(3), 123–131 CrossRef CAS PubMed.
- A. K. Nayak, D. Pal and K. Santra, Swelling and drug release behavior of metformin HCl-loaded tamarind seed polysaccharide-alginate beads, Int. J. Biol. Macromol., 2016, 82, 1023–1027 CrossRef CAS PubMed.
- S. Jana, et al., Aceclofenac-loaded unsaturated esterified alginate/gellan gum microspheres: In vitro and in vivo assessment, Int. J. Biol. Macromol., 2013, 57, 129–137 CrossRef CAS.
- B. J. Dukovski, et al., Lipid/alginate nanoparticle-loaded in situ gelling system tailored for dexamethasone nasal delivery, Int. J. Pharm., 2017, 533(2), 480–487 CrossRef CAS PubMed.
- F. A. N. Mohamed and F. Laraba-Djebari, Development and characterization of a new carrier for vaccine delivery based on calcium-alginate nanoparticles: Safe immunoprotective approach against scorpion envenoming, Vaccine, 2016, 34(24), 2692–2699 CrossRef PubMed.
- S. Gopi, et al., Biopolymers and their composites for drug delivery: a brief review, in Macromolecular Symposia, Wiley Online Library, 2018 Search PubMed.
- Z. Shariatinia and Z. Zahraee, Controlled release of metformin from chitosan–based nanocomposite films containing mesoporous MCM-41 nanoparticles as novel DDS, J. Colloid Interface Sci., 2017, 501, 60–76 CrossRef CAS PubMed.
- S. Kumar, et al., Metformin-loaded alginate nanoparticles as an effective antidiabetic agent for controlled drug release, J. Pharm. Pharmacol., 2017, 69(2), 143–150 CrossRef CAS.
- S. Dey and K. Sreenivasan, Conjugation of curcumin onto alginate enhances aqueous solubility and stability of curcumin, Carbohydr. Polym., 2014, 99, 499–507 CrossRef CAS PubMed.
- P. R. Sarika, N. R. James, P. R. Anil Kumar and D. K. Raj, et al., Galactosylated alginate-curcumin micelles for enhanced delivery of curcumin to hepatocytes, Int. J. Biol. Macromol., 2016, 86, 1–9 CrossRef CAS PubMed.
- D. Lachowicz, et al., Blood-compatible, stable micelles of sodium alginate – Curcumin bioconjugate for anti-cancer applications, Eur. Polym. J., 2019, 113, 208–219 CrossRef CAS.
- P. R. Guru, A. K. Nayak and R. K. Sahu, Oil-entrapped sterculia gum–alginate buoyant systems of aceclofenac: development and in vitro evaluation, Colloids Surf., B, 2013, 104, 268–275 CrossRef CAS PubMed.
- A. K. Nayak, et al., Fenugreek seed mucilage-alginate mucoadhesive beads of metformin HCl: Design, optimization and evaluation, Int. J. Biol. Macromol., 2013, 54, 144–154 CrossRef CAS PubMed.
- A. K. Nayak, D. Pal and J. Malakar, Development, optimization, and evaluation of emulsion-gelled floating beads using natural polysaccharide-blend for controlled drug release, Polym. Eng. Sci., 2013, 53(2), 238–250 CrossRef CAS.
- B. Das, et al., Zinc alginate-carboxymethyl cashew gum microbeads for prolonged drug release: development and optimization, Int. J. Biol. Macromol., 2014, 70, 506–515 CrossRef CAS PubMed.
- A. El-kamel, O. Al-Gohary and E. Hosny, Alginate-diltiazem hydrocloride beads: Optimization of formulation factors, in vitro and in vivo availability, J. Microencapsulation, 2003, 20, 211–225 CrossRef CAS.
- Y. L. Patel, P. Sher and A. P. Pawar, The effect of drug concentration and curing time on processing and properties of calcium alginate beads containing metronidazole by response surface methodology, AAPS PharmSciTech, 2006, 7, 86, DOI:10.1208/pt070486.
- R. S. Al-Kassas, O. M. Al-Gohary and M. M. Al-Faadhel, Controlling of systemic absorption of gliclazide through incorporation into alginate beads, Int. J. Pharm., 2007, 341(1–2), 230–237 CrossRef CAS PubMed.
- B. A. Yegin, et al., Sulindac loaded alginate beads for a mucoprotective and controlled drug release, J. Microencapsulation, 2007, 24(4), 371–382 CrossRef CAS.
- M. L. Torre, et al., Formulation and Characterization of Calcium Alginate Beads Containing Ampicillin, Pharm. Dev. Technol., 1998, 3(2), 193–198 CrossRef CAS.
- M. Morshed, et al., Effect of Barium Chloride as A Cross Linking Agent on the Sodium Alginate Based Diclofenac Sodium Beads, Bangladesh Pharm. J., 2012, 15, 53–57 Search PubMed.
- M. K. Das and P. C. Senapati, Furosemide-loaded Alginate Microspheres Prepared by Ionic Cross-linking Technique: Morphology and Release Characteristics, Indian J. Pharm. Sci., 2008, 70(1), 77–84 CrossRef CAS PubMed.
- V. Deshmukh, et al., Formulation, Optimization and Evaluation of Controlled Release Alginate Microspheres Using Synergy Gum Blends, Res. J. Pharm. Technol., 2008, 2 Search PubMed.
- D. S. Chakraborty, et al., Preparation, in vitro and in vivo evaluation of algino-pectinate bioadhesive microspheres: An investigation of the effects of polymers using multiple comparison analysis, Acta Pharm., 2010, 60, 255–266 Search PubMed.
- J. Raja Kumar and S. Muralidharan, Formulation and In vitro Evalution of Gellan Gum/Carbopol and Sodium Alginate based Solution to Gel Depot of Ketotifen Fumarate System, J. Pet. Sci. Res., 2012, 4, 1973–1977 Search PubMed.
- T. Pongjanyakul and S. Puttipipatkhachorn, Xanthan-alginate composite gel beads: molecular interaction and in vitro characterization, Int. J. Pharm., 2007, 331(1), 61–71 CrossRef CAS PubMed.
- A. K. Nayak and D. Pal, Formulation optimization and evaluation of jackfruit seed starch–alginate mucoadhesive beads of metformin HCl, Int. J. Biol. Macromol., 2013, 59, 264–272 CrossRef CAS PubMed.
- A. K. Nayak, B. Das and R. Maji, Calcium alginate/gum Arabic beads containing glibenclamide: Development and in vitro characterization, Int. J. Biol. Macromol., 2012, 51(5), 1070–1078 CrossRef CAS PubMed.
- B. Singh, V. Sharma and D. Chauhan, Gastroretentive floating sterculia–alginate beads for use in antiulcer drug delivery, Chem. Eng. Res. Des., 2010, 88(8), 997–1012 CrossRef CAS.
- A. K. Nayak, D. Pal and K. Santra, Development of calcium pectinate-tamarind seed polysaccharide mucoadhesive beads containing metformin HCl, Carbohydr. Polym., 2014, 101, 220–230 CrossRef CAS PubMed.
- A. K. Nayak and D. Pal, Development of pH-sensitive tamarind seed polysaccharide-alginate composite beads for controlled diclofenac sodium delivery using response surface methodology, Int. J. Biol. Macromol., 2011, 49(4), 784–793 CrossRef CAS.
- A. Nayak, D. Pal and K. Santra, Tamarind seed polysaccharide–gellan mucoadhesive beads for controlled release of metformin HCl, Carbohydr. Polym., 2014, 103, 154–163 CrossRef CAS.
- A. Nayak, B. Mohanty and K. Sen, Comparative evaluation of in vitro diclofenac sodium permeability across excised mouse skin from different common pharmaceutical vehicles, Int. J. PharmTech Res., 2010, 2, 920–930 CAS.
- D. P. Maurya, et al., Formulation and optimization of alkaline extracted ispaghula husk microparticles of isoniazid – in vitro and in vivo assessment, J. Microencapsulation, 2011, 28(6), 472–482 CrossRef CAS.
- V. Kumar and A. Bhattacharya, Release of Metformin Hydrochloride from Ispaghula-Sodium Alginate Beads Adhered on Cock Intestinal Mucosa, Indian J. Pharm. Educ. Res., 2008, 42(4) Search PubMed.
- H. Sharma, B. Sarangi and S. P. Pradhan, Preparation and in-vitro evaluation of mucoadhesive microbeads containing timolol maleate using mucoadhesive substances of Dillenia indica L, Arch. Pharm. Sci. Res., 2009, 1, 181–188 CAS.
- R. Raparla and T. E. G. K. Murthy, Preparation and evaluation of mucoadhesive microcapsules of glipizide formulated with gum kondagogu: In vitro and in vivo, Acta Pharm. Sci., 2010, 52, 335–344 Search PubMed.
- R. Justin, et al., Biodegradable and conductive
chitosan–graphene quantum dot nanocomposite microneedles for delivery of both small and large molecular weight therapeutics, RSC Adv., 2015, 5(64), 51934–51946 RSC.
- P. R. Guru, et al., Aceclofenac-Loaded Plantago ovata F. Husk Mucilage-Zn+2-Pectinate Controlled-Release Matrices, Starch – Stärke, 2018, 70(3–4), 1700136 CrossRef.
- A. K. Nayak, D. Pal and K. Santra, Ispaghula mucilage-gellan mucoadhesive beads of metformin HCl: development by response surface methodology, Carbohydr. Polym., 2014, 107, 41–50 CrossRef CAS.
- A. K. Nayak, D. Pal and K. Santra, Artocarpus heterophyllus L. seed starch-blended gellan gum mucoadhesive beads of metformin HCl, Int. J. Biol. Macromol., 2014, 65, 329–339 CrossRef CAS PubMed.
- J. A. Posey, et al., Phase 1 study of weekly polyethylene glycol-camptothecin in patients with advanced solid tumors and lymphomas, Clin. Cancer Res., 2005, 11(21), 7866–7871 CrossRef CAS PubMed.
- Y. Chau, et al., Antitumor efficacy of a novel polymer-peptide-drug conjugate in human tumor xenograft models, Int. J. Cancer, 2006, 118(6), 1519–1526 CrossRef CAS PubMed.
- S.-i. Sugahara, et al., Complete regression of xenografted human carcinomas by a paclitaxel–carboxymethyl dextran conjugate (AZ10992), J. Controlled Release, 2007, 117(1), 40–50 CrossRef CAS PubMed.
- S. A. Veltkamp, et al., Clinical and pharmacologic study of the novel prodrug delimotecan (MEN 4901/T-0128) in patients with solid tumors, Clin. Cancer Res., 2008, 14(22), 7535–7544 CrossRef CAS PubMed.
- B. Naeye, et al., PEGylation of biodegradable dextran nanogels for siRNA delivery, Eur. J. Pharm. Sci., 2010, 40(4), 342–351 CrossRef CAS.
- A. Celebioglu and T. Uyar, Metronidazole/Hydroxypropyl-β-Cyclodextrin inclusion complex nanofibrous webs as fast-dissolving oral drug delivery system, Int. J. Pharm., 2019, 572, 118828 CrossRef CAS.
- P. Yousefpour, et al., Targeted delivery of DOX-utilizing chitosan nanoparticles surface-functionalized with anti-Her2 trastuzumab, Int. J. Nanomed., 2011, 6, 1977–1990 CAS.
- F. Li, et al., Reducing Both Pgp Overexpression and Drug Efflux with Anti-Cancer Gold-Paclitaxel Nanoconjugates, PLoS One, 2016, 11(7), e0160042 CrossRef PubMed.
- A. Solanki, et al., β-Cyclodextrin based magnetic nanoconjugates for targeted drug delivery in cancer therapy, RSC Adv., 2016, 6(101), 98693–98707 RSC.
- W. Dang, et al., Covalent coupling of methotrexate to dextran enhances the penetration of cytotoxicity into a tissue-like matrix, Cancer Res., 1994, 54(7), 1729–1735 CAS.
- T. F. Vandamme, et al., The use of polysaccharides to target drugs to the colon, Carbohydr. Polym., 2002, 48(3), 219–231 CrossRef CAS.
- J. S. Lee, et al., Synthesis and properties of dextran–nalidixic acid ester as a colon-specific prodrug of nalidixic acid, Drug Dev. Ind. Pharm., 2001, 27(4), 331–336 CrossRef CAS PubMed.
- A. Popat, et al., Curcumin-cyclodextrin encapsulated chitosan nanoconjugates with enhanced solubility and cell cytotoxicity, Colloids Surf., B, 2014, 117, 520–527 CrossRef CAS PubMed.
- A. Hinz, et al., Analysis of toxicity and anticancer activity of micelles of sodium alginate-curcumin, Int. J. Nanomed., 2019, 14, 7249–7262 CrossRef PubMed.
- W. Ji, et al., Chemosensitizing indomethacin-conjugated dextran-based micelles for effective delivery of paclitaxel in resistant breast cancer therapy, PLoS One, 2017, 12(7), e0180037 CrossRef PubMed.
- X. Zeng, et al., Indomethacin-grafted and pH-sensitive dextran micelles for overcoming inflammation-mediated multidrug resistance in breast cancer, Carbohydr. Polym., 2020, 237, 116139 CrossRef CAS PubMed.
- S. Wong, et al., Just add sugar for carbohydrate induced self-assembly of curcumin, Nat. Commun., 2019, 10(1), 582 CrossRef CAS.
- D. Agudelo, et al., Transporting antitumor drug tamoxifen and its metabolites, 4-hydroxytamoxifen and endoxifen by chitosan nanoparticles, PLoS One, 2013, 8(3), e60250 CrossRef CAS PubMed.
- J. S. Lee, et al., Synthesis and properties of dextran-nalidixic acid ester as a colon-specific prodrug of nalidixic acid, Drug Dev. Ind. Pharm., 2001, 27(4), 331–336 CrossRef CAS PubMed.
- A. O. Abioye and A. Kola-Mustapha, Controlled Electrostatic Self-Assembly of Ibuprofen-Cationic Dextran Nanoconjugates Prepared by low Energy Green Process – a Novel Delivery Tool for Poorly Soluble Drugs, Pharm. Res., 2015, 32(6), 2110–2131 CrossRef CAS.
- G. J. Weiss, et al., First-in-human phase 1/2a trial of CRLX101, a cyclodextrin-containing polymer-camptothecin nanopharmaceutical in patients with advanced solid tumor malignancies, Invest. New Drugs, 2013, 31(4), 986–1000 CrossRef CAS PubMed.
- M. E. Davis, Design and development of IT-101, a cyclodextrin-containing polymer conjugate of camptothecin, Adv. Drug Delivery Rev., 2009, 61(13), 1189–1192 CrossRef CAS PubMed.
- S. A. Veltkamp, et al., Clinical and Pharmacologic Study of the Novel Prodrug Delimotecan (MEN 4901/T-0128) in Patients with Solid Tumors, Clin. Cancer Res., 2008, 14, 7535–7544 CrossRef CAS.
- O. Soepenberg, et al., Phase I and pharmacokinetic study of DE-310 in patients with advanced solid tumors, Clin. Cancer Res., 2005, 11(2), 703–711 CrossRef CAS.
- S. Danhauser-Riedl, et al., Phase I clinical and pharmacokinetic trial of dextran conjugated DOX (AD-70, DOX-OXD), Invest. New Drugs, 1993, 11(2–3), 187–195 CrossRef CAS PubMed.
- K. Park, et al., Preparation and Characterization of Self-Assembled Nanoparticles of Heparin-Deoxycholic Acid Conjugates, Langmuir, 2004, 20(26), 11726–11731 CrossRef CAS PubMed.
- K. Park, et al., Heparin–deoxycholic acid chemical conjugate as an anticancer drug carrier and its antitumor activity, J. Controlled Release, 2006, 114(3), 300–306 CrossRef CAS.
- T. Zhang, et al., Combination chemotherapy of DOX, all-trans retinoic acid and low molecular weight heparin based on self-assembled multi-functional polymeric nanoparticles, Nanotechnology, 2015, 26(14), 145101 CrossRef PubMed.
- N. U. Khaliq, et al., DOX/heparin composite nanoparticles for caspase-activated prodrug chemotherapy, Biomaterials, 2016, 101, 131–142 CrossRef CAS PubMed.
- T. H. Tran, et al., Heparin-folate-retinoic acid bioconjugates for targeted delivery of hydrophobic photosensitizers, Carbohydr. Polym., 2013, 92(2), 1615–1624 CrossRef CAS PubMed.
- J. Emami, et al., Novel pH-triggered biocompatible polymeric micelles based on heparin–α-tocopherol conjugate for intracellular delivery of docetaxel in breast cancer, Pharm. Dev. Technol., 2020, 25(4), 492–509 CrossRef CAS.
- Y. Wu, et al., Tumor microenvironment-responsive PEGylated heparin-pyropheophorbide-a nanoconjugates for photodynamic therapy, Carbohydr. Polym., 2021, 255, 117490 CrossRef CAS.
- L. Mei, et al., Antitumor and Antimetastasis Activities of Heparin-based Micelle Served As Both Carrier and Drug, ACS Appl. Mater. Interfaces, 2016, 8(15), 9577–9589 CrossRef CAS.
- X. Wang, et al., HFT-T, a Targeting Nanoparticle, Enhances Specific Delivery of Paclitaxel to Folate Receptor-Positive Tumors, ACS Nano, 2009, 3(10), 3165–3174 CrossRef CAS.
- C. H. Salamanca, et al., Natural gum-type biopolymers as potential modified nonpolar drug release systems, Carbohydr. Polym., 2018, 189, 31–38 CrossRef CAS PubMed.
- L. I. Atanase, Micellar DDS based on natural biopolymers, Polymers, 2021, 13(3), 477 CrossRef CAS PubMed.
- B. Devrim, A. Bozkir and K. Canefe, Formulation and evaluation of reconstitutable suspensions containing ibuprofen-loaded Eudragit microspheres, Acta Pol. Pharm., 2011, 68(4), 593–599 CAS.
- G. Roopa, R. S. Bhat and M. S. Dakshina, Formulation and evaluation of an antacid and anti-ulcer suspension containing herbal drugs, Biomed. Pharmacol. J., 2015, 3(1), 01–06 Search PubMed.
- M. Chaturvedi, et al., Recent development in novel DDS of herbal drugs, Int. J. Green Pharm., 2011, 5(2), 363–371 Search PubMed.
- R. Nethaji, et al., Formulation and evaluation of metformin hydrochloride loaded mucoadhesive microspheres, Int. J. Pharm., Chem. Biol. Sci., 2016, 6(2) CAS.
- O. Samia, R. Hanan and E. T. Kamal, Carbamazepine mucoadhesive nanoemulgel (MNEG) as brain targeting delivery system via the olfactory mucosa, Drug Delivery, 2012, 19(1), 58–67 CrossRef CAS PubMed.
- S. Samudre, et al., Xanthan gum coated mucoadhesive liposomes for efficient nose to brain delivery of curcumin, Drug Delivery Lett., 2015, 5(3), 201–207 CrossRef CAS.
- R. Saudagar and K. Badhe, Development and evaluation of ph dependent in situ nasal gel of loratadine, Eur. J. Biomed. Pharm. Sci., 2016, 1(3), 233–238 Search PubMed.
- C. Chittasupho, M. Jaturanpinyo and S. Mangmool, Pectin nanoparticle enhances cytotoxicity of methotrexate against hepG2 cells, Drug Delivery, 2013, 20(1), 1–9 CrossRef CAS PubMed.
- M. Cheng, et al., [Preparation and lymphatic targeting research of targeting antitumor drug: pectin-adriamycin conjugates], Shengwu Yixue Gongchengxue Zazhi, 2009, 26(3), 569–574 CAS.
- R. K. Dev, V. Bali and K. Pathak, Novel microbially triggered colon specific delivery system of 5-Fluorouracil: Statistical optimization, in vitro, in vivo, cytotoxic and stability assessment, Int. J. Pharm., 2011, 411(1), 142–151 CrossRef CAS PubMed.
- J. T. Chung and Z. Zhang, Mechanical characterization of calcium pectinate hydrogel for controlled drug delivery, Hem. Ind., 2003, 57, 611–616 CrossRef CAS.
- A. Assifaoui, O. Chambin and P. Cayot, Drug release from calcium and zinc pectinate beads: Impact of dissolution medium composition, Carbohydr. Polym., 2011, 85(2), 388–393 CrossRef CAS.
- C. Lara-Espinoza, et al., Pectin and pectin-based composite materials: Beyond food texture, Molecules, 2018, 23(4), 942 CrossRef PubMed.
- P. Sriamornsak, et al., Effect of drug loading method on drug content and drug release from calcium pectinate gel beads, AAPS PharmSciTech, 2010, 11(3), 1315–1319 CrossRef CAS PubMed.
- I. El-Gibaly, Oral delayed-release system based on Zn-pectinate gel (ZPG) microparticles as an alternative carrier to calcium pectinate beads for colonic drug delivery, Int. J. Pharm., 2002, 232(1), 199–211 CrossRef CAS.
- K. A. Verma and K. Sachin, Novel Hydrophilic Drug Polymer Nano-Conjugates of Cisplatin Showing Long Blood Retention Profile - Its Release Kinetics, Cellular Uptake and Bio-Distribution, Curr. Drug Delivery, 2008, 5(2), 120–126 CrossRef PubMed.
- S. Majzoob, et al., Pectin-cysteine conjugate: synthesis and in-vitro evaluation of its potential for drug delivery, J. Pharm. Pharmacol., 2010, 58(12), 1601–1610 CrossRef PubMed.
- N. A. Hussien, N. Işıklan and M. Türk, Pectin-conjugated magnetic graphene oxide nanohybrid as a novel drug carrier for paclitaxel delivery, Artif. Cells, Nanomed., Biotechnol., 2018, 46(sup1), 264–273 CrossRef CAS PubMed.
- F. A. Oyarzun-Ampuero, et al., A new drug nanocarrier consisting of polyarginine and hyaluronic acid, Eur. J. Pharm. Biopharm., 2011, 79(1), 54–57 CrossRef CAS PubMed.
- A. Villaverde, Nanoparticles in translational science and medicine, Academic Press, 2011 Search PubMed.
- J. B. Rothbard, et al., Conjugation of arginine oligomers to cyclosporin A facilitates topical delivery and inhibition of inflammation, Nat. Med., 2000, 6(11), 1253–1257 CrossRef CAS.
- P. P. Shah, et al., Enhanced skin permeation using polyarginine modified nanostructured lipid carriers, J. Controlled Release, 2012, 161(3), 735–745 CrossRef CAS PubMed.
- S. Patra, E. Roy and R. Madhuri, The next generation cell-penetrating peptide and carbon dot conjugated nano-liposome for transdermal delivery of curcumin, Biomater. Sci., 2015, 4, 418–429 RSC.
- S. S. Kwon, et al., Cell penetrating peptide conjugated liposomes as transdermal delivery system of Polygonum aviculare L. extract, Int. J. Pharm., 2015, 483(1–2), 26–37 CrossRef CAS PubMed.
- M. R. Rekha and C. P. Sharma, Hemocompatible pullulan–polyethyleneimine conjugates for liver cell gene delivery: In vitro evaluation of cellular uptake, intracellular trafficking and transfection efficiency, Acta Biomater., 2011, 7(1), 370–379 CrossRef CAS PubMed.
- M. Dionísio, et al., Pullulan-based nanoparticles as carriers for transmucosal protein delivery, Eur. J. Pharm. Sci., 2013, 50(1), 102–113 CrossRef.
- J. M. Pereira, M. Mahoney and K. J. Edgar, Synthesis of amphiphilic 6-carboxypullulan ethers, Carbohydr. Polym., 2014, 100, 65–73 CrossRef CAS PubMed.
- L. Huang, et al., Versatile redox-sensitive pullulan nanoparticles for enhanced liver targeting and efficient cancer therapy, Nanomedicine, 2018, 14(3), 1005–1017 CrossRef CAS PubMed.
- R. Garhwal, et al., Sustained Ocular Delivery of Ciprofloxacin Using Nanospheres and Conventional Contact Lens Materials, Invest. Ophthalmol. Visual Sci., 2012, 53(3), 1341–1352 CrossRef CAS.
- J. Wang, et al., Tocopheryl pullulan-based self assembling nanomicelles for anti-cancer drug delivery, Mater. Sci. Eng. C, 2014, 43, 614–621 CrossRef CAS PubMed.
- L. Chen, et al., New bifunctional-pullulan-based micelles with good biocompatibility for efficient co-delivery of cancer-suppressing p53 gene and DOX to cancer cells, RSC Adv., 2015, 5(115), 94719–94731 RSC.
- F. Hassanzadeh and J. Varshosaz, Biotin-encoded Pullulan-Retinoic Acid Engineered Nanomicelles: Preparation, Optimization and In Vitro Cytotoxicity Assessment in MCF-7 Cells, Indian J. Pharm. Sci., 2016, 78, 557–565 CrossRef CAS.
- L. Chen, et al., Biocompatible
cationic pullulan-g-desoxycholic acid-g-PEI micelles used to co-deliver drug and gene for cancer therapy, Mater. Sci. Eng. C, 2017, 70, 418–429 CrossRef CAS PubMed.
- F. Hassanzadeh, et al., Novel NGR anchored pullulan micelles for controlled and targeted delivery of DOX to HeLa cancerous cells, Iran. Polym. J., 2018, 27(4), 263–274 CrossRef CAS.
- M. Constantin, et al., Novel cationic and hydrophobic pullulan derivatives as dna nanoparticulate carriers, Cellul. Chem. Technol., 2019, 53, 695–707 CrossRef CAS.
- H. Yuan, et al., Preparation of Cholesteryl-Modified Aminated Pullulan Nanoparticles to Evaluate Nanoparticle of Hydrophobic Degree on Drug Release and Cytotoxicity, J. Nanomater., 2020, 2020, 7171209 Search PubMed.
- K. Na, et al., Self-organized pullulan/deoxycholic acid nanogels: Physicochemical characterization and anti-cancer drug-releasing behavior, Biotechnol. Bioprocess Eng., 2006, 11(3), 262 CrossRef CAS.
- G. Shu, et al., Fucoidan-based micelles as P-selectin targeted carriers for synergistic treatment of acute kidney injury, Nanomed.: Nanotechnol. Biol. Med., 2021, 32, 102342 CrossRef CAS PubMed.
- S. Chen, et al., Nano-micelles based on hydroxyethyl starch-curcumin conjugates for improved stability, antioxidant and anticancer activity of curcumin, Carbohydr. Polym., 2020, 228, 115398 CrossRef CAS PubMed.
- G. A. Soares, et al., Blends of cross-linked high amylose starch/pectin loaded with diclofenac, Carbohydr. Polym., 2013, 91(1), 135–142 CrossRef CAS.
- N. Biswas and R. K. Sahoo, Tapioca starch blended alginate mucoadhesive-floating beads for intragastric delivery of Metoprolol Tartrate, Int. J. Biol. Macromol., 2016, 83, 61–70 CrossRef CAS PubMed.
- J. Malakar, et al., Potato starch-blended alginate beads for prolonged release of tolbutamide: Development by statistical optimization and in vitro characterization, Asian J. Pharm., 2013, 7, 43–51 CrossRef.
- A. Jha and A. Bhattacharya, Preparation and evaluation of sweet potato starch-blended sodium alginate microbeads, Asian J. Pharm., 2009, 4, 122–128 Search PubMed.
- N. Sachan and A. Bhattacharya, Feasibility of Assam bora rice based matrix microdevices for controlled release of water insoluble drug, Int. J. Pharm. Pharm. Sci., 2009, 1, 96–102 CAS.
- A. K. Nayak and D. Pal, Blends of jackfruit seed starch-pectin in the development of mucoadhesive beads containing metformin HCl, Int. J. Biol. Macromol., 2013, 62, 137–145 CrossRef CAS PubMed.
- A. K. Nayak and D. Pal, Ionotropically-gelled mucoadhesive beads for oral metformin HCl delivery: Formulation, optimization and antidiabetic evaluation, J. Sci. Ind. Res., 2013, 72, 15–22 CAS.
- A. Nayak, D. Pal and K. Santra, Development of pectinate-ispagula mucilage mucoadhesive beads of metformin HCl by central composite design, Int. J. Biol. Macromol., 2014, 66 CrossRef CAS PubMed.
- K. Liu, et al., A facile one-pot synthesis of starch functionalized graphene as nano-carrier for pH sensitive and starch-mediated drug delivery, Colloids Surf., B, 2015, 128, 86–93 CrossRef CAS PubMed.
- C. Saikia, et al., Effect of crosslinker on drug delivery properties of curcumin loaded starch coated iron oxide nanoparticles, Int. J. Biol. Macromol., 2016, 93, 1121–1132 CrossRef CAS PubMed.
- H. Hamidian and T. Tavakoli, Preparation of a new Fe3O4/starch-g-polyester nanocomposite hydrogel and a study on swelling and drug delivery properties, Carbohydr. Polym., 2016, 144, 140–148 CrossRef CAS PubMed.
- M. M. Friciu, et al., Carboxymethyl starch and lecithin complex as matrix for targeted drug delivery: I. Monolithic Mesalamine forms for colon delivery, Eur. J. Pharm. Biopharm., 2013, 85(3), 521–530 CrossRef PubMed.
- C. Wu, et al., Redox-responsive core-cross-linked mPEGylated starch micelles as nanocarriers for intracellular anticancer drug release, Eur. Polym. J., 2016, 83, 230–243 CrossRef CAS.
- V. Raj and G. Prabha, Synthesis, characterization and in vitro drug release of cisplatin loaded Cassava starch acetate–PEG/gelatin nanocomposites, J. Assoc. Arab Univ. Basic Appl. Sci., 2016, 21, 10–16 Search PubMed.
- L.-K. Zhang, et al., Bacterial cellulose based composites enhanced transdermal drug targeting for breast cancer treatment, Chem. Eng. J., 2019, 370, 749–759 CrossRef CAS.
- J. Malakar, A. K. Nayak and A. Das, Modified starch (cationized)–alginate beads containing aceclofenac: Formulation optimization using central composite design, Starch – Stärke, 2013, 65(7–8), 603–612 CrossRef CAS.
- K. A. Nayak, D. Pal and S. M. Hasnain, Development, Optimization and in vitro-in vivo Evaluation of Pioglitazone- Loaded Jackfruit Seed Starch-Alginate Beads, Curr. Drug Delivery, 2013, 10(5), 608–619 CrossRef PubMed.
- A. K. Nayak, et al., Soluble starch-blended Ca2+-Zn2+-alginate composites-based microparticles of aceclofenac: Formulation development and in vitro characterization, Future J. Pharm. Sci., 2018, 4(1), 63–70 CrossRef.
- J. Dziadkowiec, et al., Preparation, characterization and application in controlled release of Ibuprofen-loaded Guar Gum/Montmorillonite Bionanocomposites, Appl. Clay Sci., 2017, 135, 52–63 CrossRef CAS.
- S. Sharma, et al., l-Alanine induced thermally stable self-healing guar gum hydrogel as potential drug vehicle for sustained release of hydrophilic drug, Mater. Sci. Eng., C, 2019, 99, 1384–1391 CrossRef CAS PubMed.
- K. Dutta, et al., An ex situ approach to fabricating nanosilica reinforced polyacrylamide grafted guar gum nanocomposites as an efficient biomaterial for transdermal drug delivery application, New J. Chem., 2017, 41(17), 9461–9471 RSC.
- P. B. Kajjari, L. S. Manjeshwar and T. M. Aminabhavi, Novel interpenetrating polymer network hydrogel microspheres of chitosan and poly (acrylamide)-grafted-guar gum for controlled release of ciprofloxacin, Ind. Eng. Chem. Res., 2011, 50(23), 13280–13287 CrossRef CAS.
- A. Giri, et al., Tailoring carboxymethyl guargum hydrogel with nanosilica for sustained transdermal release of diclofenac sodium, Carbohydr. Polym., 2012, 87(2), 1532–1538 CrossRef CAS.
- A. Giri, et al., Polymer hydrogel from carboxymethyl guar gum and carbon nanotube for sustained trans-dermal release of diclofenac sodium, Int. J. Biol. Macromol., 2011, 49(5), 885–893 CrossRef CAS PubMed.
- A. Butt, et al., Controlled release of cephradine by biopolymers based target specific crosslinked hydrogels, Int. J. Biol. Macromol., 2019, 121, 104–112 CrossRef CAS.
- W. Lohcharoenkal, et al., Protein Nanoparticles as Drug Delivery Carriers for Cancer Therapy, BioMed Res. Int., 2014, 2014, 180549 Search PubMed.
- M. Foox and M. Zilberman, Drug delivery from gelatin-based systems, Expert Opin. Drug Delivery, 2015, 12(9), 1547–1563 CrossRef CAS PubMed.
- E. J. Lee, et al., Studies on the characteristics of drug-loaded gelatin nanoparticles prepared by nanoprecipitation, Bioprocess Biosyst. Eng., 2012, 35(1), 297–307 CrossRef CAS PubMed.
- A. O. Elzoghby, Gelatin-based nanoparticles as drug and gene delivery systems: reviewing three decades of research, J. Controlled Release, 2013, 172(3), 1075–1091 CrossRef CAS PubMed.
- G. Zhang, et al., DOX-loaded folate-mediated pH-responsive micelle based on Bletilla striata polysaccharide: Release mechanism, cellular uptake mechanism, distribution, pharmacokinetics, and antitumor effects, Int. J. Biol. Macromol., 2020, 164, 566–577 CrossRef CAS.
- A. C. d. J. Oliveira, et al., Microwave-initiated rapid synthesis of phthalated cashew gum for DDS, Carbohydr. Polym., 2021, 254, 117226 CrossRef CAS.
- Z. Negahban, S. A. Shojaosadati and S. Hamedi, A novel self-assembled micelles based on stearic acid modified schizophyllan for efficient delivery of paclitaxel, Colloids Surf., B, 2021, 199, 111524 CrossRef CAS PubMed.
- R. J. Babu, et al., Formulation of controlled release gellan gum macro beads of amoxicillin, Curr. Drug Delivery, 2010, 7(1), 36–43 CrossRef CAS PubMed.
- M. Narkar, P. Sher and A. Pawar, Stomach-specific controlled release gellan beads of acid-soluble drug prepared by ionotropic gelation method, AAPS PharmSciTech, 2010, 11(1), 267–277 CrossRef CAS PubMed.
- H. Bera, S. Boddupalli and A. K. Nayak, Mucoadhesive-floating zinc-pectinate–sterculia gum interpenetrating polymer network beads encapsulating ziprasidone HCl, Carbohydr. Polym., 2015, 131, 108–118 CrossRef CAS.
- A. Nayak, D. Pal and K. Santra, Development of calcium pectinate-tamarind seed polysaccharide mucoadhesive beads containing metformin HCl, Carbohydr. Polym., 2014, 101, 220–230 CrossRef CAS PubMed.
- P. Basim, S. Gorityala and M. Kurakula, Advances in Functionalized Hybrid Biopolymer Augmented Lipid-based Systems: A Spotlight on Their Role in Design of Gastro Retentive Delivery Systems, Arch. Gastroenterol. Res., 2021, 2(1), 35–47 Search PubMed.
- S. Nagarajan, et al., Overview of Protein-Based Biopolymers for Biomedical Application, Macromol. Chem. Phys., 2019, 220(14), 1900126 CrossRef.
- E. Quinlan, et al., Development of collagen–hydroxyapatite scaffolds incorporating PLGA and alginate microparticles for the controlled delivery of rhBMP-2 for bone tissue engineering, J. Controlled Release, 2015, 198, 71–79 CrossRef CAS.
- X. Zhang, K. Tang and X. Zheng, Electrospinning and Crosslinking of COL/PVA Nanofiber-microsphere
Containing Salicylic Acid for Drug Delivery, J. Bionic Eng., 2016, 13(1), 143–149 CrossRef.
- E. K. Tsekoura, et al., Battling bacterial infection with hexamethylene diisocyanate cross-linked and Cefaclor-loaded collagen scaffolds, Biomed. Mater., 2017, 12(3), 035013 CrossRef CAS.
- R. Khan and M. H. Khan, Use of collagen as a biomaterial: An update, J. Indian Soc. Periodontol., 2013, 17(4), 539–542 CrossRef PubMed.
- W. Liu, M. Griffith and F. Li, Alginate microsphere-collagen composite hydrogel for ocular drug delivery and implantation, J. Mater. Sci.: Mater. Med., 2008, 19(11), 3365–3371 CrossRef CAS.
- Z. Liu, et al., Study of an alginate/HPMC-based in situ gelling ophthalmic delivery system for gatifloxacin, Int. J. Pharm., 2006, 315(1–2), 12–17 CrossRef CAS PubMed.
- S. Anandhakumar, et al., Preparation of collagen peptide functionalized chitosan nanoparticles by ionic gelation method: An effective carrier system for encapsulation and release of DOX for cancer drug delivery, Mater. Sci. Eng. C, 2017, 70, 378–385 CrossRef CAS PubMed.
- D. Choi, et al., Nano-film coatings onto collagen hydrogels with desired drug release, J. Ind. Eng. Chem., 2016, 36, 326–333 CrossRef CAS.
- G. T. Tihan, et al., Chloramphenicol collagen sponges for local drug delivery in dentistry, C. R. Chim., 2015, 18(9), 986–992 CrossRef.
- G. T. Tihan, et al., Collagen-based biomaterials for ibuprofen delivery, C. R. Chim., 2016, 19(3), 390–394 CrossRef.
- G. Voicu, et al., Synthesis, characterization and bioevaluation of drug-collagen hybrid materials for biomedical applications, Int. J. Pharm., 2016, 510(2), 474–484 CrossRef CAS.
- H. Nejat, et al., Preparation and characterization of cardamom extract-loaded gelatin nanoparticles as effective targeted drug delivery system to treat glioblastoma, React. Funct. Polym., 2017, 120, 46–56 CrossRef CAS.
- A. Salerno, et al., Hybrid gelatin-based porous materials with a tunable multiscale morphology for tissue engineering and drug delivery, Eur. Polym. J., 2018, 99, 230–239 CrossRef CAS.
- L. S. Dolci, et al., Non-equilibrium atmospheric pressure plasma as innovative method to crosslink and enhance mucoadhesion of econazole-loaded gelatin films for buccal drug delivery, Colloids Surf., B, 2018, 163, 73–82 CrossRef CAS.
- R. Brito-Pereira, et al., Silk fibroin-magnetic hybrid composite electrospun fibers for tissue engineering applications, Composites, Part B, 2018, 141, 70–75 CrossRef CAS.
- S. Sotome, et al., Synthesis and in vivo evaluation of a novel hydroxyapatite/collagen–alginate as a bone filler and a drug delivery carrier of bone morphogenetic protein, Mater. Sci. Eng. C, 2004, 24(3), 341–347 CrossRef.
- M. V. Natu, et al., Controlled release gelatin hydrogels and lyophilisates with potential application as ocular inserts, Biomed. Mater., 2007, 2(4), 241–249 CrossRef CAS.
- A. Bandiera, et al., Stimuli-induced release of compounds from elastin biomimetic matrix, Biomacromolecules, 2014, 15(1), 416–422 CrossRef CAS.
- S. Nagarajan, et al., Overview of Protein-Based Biopolymers for Biomedical Application, Macromol. Chem. Phys., 2019, 220(14), 1900126 CrossRef.
- A. Bandiera, et al., Composite of Elastin-Based Matrix and Electrospun Poly(L-Lactic Acid) Fibers: A Potential Smart Drug Delivery System, Front. Bioeng. Biotechnol., 2018, 6, 127 CrossRef.
- S. H. Park, H. S. Shin and S. N. Park, A novel pH-responsive hydrogel based on carboxymethyl cellulose/2-hydroxyethyl acrylate for transdermal delivery of naringenin, Carbohydr. Polym., 2018, 200, 341–352 CrossRef CAS PubMed.
- M. Khamrai, et al., Curcumin entrapped gelatin/ionically modified bacterial cellulose based self-healable hydrogel film: An eco-friendly sustainable synthesis method of wound healing patch, Int. J. Biol. Macromol., 2019, 122, 940–953 CrossRef CAS PubMed.
- A. Anderson, et al., Electrochemically Controlled Dissolution of Nanocarbon–Cellulose Acetate Phthalate Microneedle Arrays, ACS Appl. Mater. Interfaces, 2019, 11(39), 35540–35547 CrossRef CAS.
- W. Simchareon, et al., Characterization of Natural Rubber Latex Film Containing Various Enhancers, Procedia Chem., 2012, 4, 308–312 CrossRef CAS.
- M. Rahim, M. R. H. M. Haris and N. U. Saqib, An overview of polymeric nano-biocomposites as targeted and controlled-release devices, Biophys. Rev., 2020, 1–9 Search PubMed.
- S. Han, et al., Alkylation of human hair keratin for tunable hydrogel erosion and drug delivery in tissue engineering applications, Acta Biomater., 2015, 23, 201–213 CrossRef CAS.
- Y. Dou, et al., Keratin/Polyvinyl Alcohol Blend Films Cross-Linked by Dialdehyde Starch and Their Potential Application for Drug Release, Polymers, 2015, 7(3), 580–591 CrossRef CAS.
- Y. Li, et al., Preparation and characterization of DOX loaded keratin nanoparticles for pH/GSH dual responsive release, Mater. Sci. Eng., C, 2017, 73, 189–197 CrossRef CAS.
- A. O. Elzoghby, W. M. Samy and N. A. Elgindy, Albumin-based nanoparticles as potential controlled release DDS, J. Controlled Release, 2012, 157(2), 168–182 CrossRef CAS PubMed.
- B. Elsadek and F. Kratz, Impact of albumin on drug delivery — New applications on the horizon, J. Controlled Release, 2012, 157(1), 4–28 CrossRef CAS.
- C. Ju, et al., Sequential intra-intercellular nanoparticle delivery system for deep tumor penetration, Angew. Chem., Int. Ed. Engl., 2014, 53(24), 6253–6258 CrossRef CAS PubMed.
- M. L. P. Vidallon, F. Yu and B. M. Teo, Controlling the Size and Polymorphism of Calcium Carbonate Hybrid Particles Using Natural Biopolymers, Cryst. Growth Des., 2020, 20(2), 645–652 CrossRef CAS.
- Y. Okamoto, et al., Albumin-Encapsulated Liposomes: A Novel Drug Delivery Carrier With Hydrophobic Drugs Encapsulated in the Inner Aqueous Core, J. Pharm. Sci., 2018, 107(1), 436–445 CrossRef CAS.
- S. Perteghella, et al., Stem cell-extracellular vesicles as DDS: New frontiers for silk/curcumin nanoparticles, Int. J. Pharm., 2017, 520(1), 86–97 CrossRef CAS.
- M. J. de Jesús Valle, et al., Development and In Vitro Evaluation of a Novel Drug Delivery System (Albumin Microspheres Containing Liposomes) Applied to Vancomycin, J. Pharm. Sci., 2016, 105(7), 2180–2187 CrossRef.
- Y. Iwao, et al., Inflamed site-specific drug delivery system based on the interaction of human serum albumin nanoparticles with myeloperoxidase in a murine model of experimental colitis, Eur. J. Pharm. Biopharm., 2018, 125, 141–147 CrossRef CAS.
- R. P. Das, et al., Tuning the binding, release and cytotoxicity of hydrophobic drug by Bovine Serum Albumin nanoparticles: Influence of particle size, Colloids Surf., B, 2017, 158, 682–688 CrossRef CAS PubMed.
- H. Nosrati, et al., Bovine Serum Albumin (BSA) coated iron oxide magnetic nanoparticles as biocompatible carriers for curcumin-anticancer drug, Bioorg. Chem., 2018, 76, 501–509 CrossRef CAS PubMed.
- M. Benkő, et al., Bovine serum albumin-sodium alkyl sulfates bioconjugates as DDS, Colloids Surf., B, 2015, 130, 126–132 CrossRef.
- N. Taneja and K. K. Singh, Rational design of polysorbate 80 stabilized human serum albumin nanoparticles tailored for high drug loading and entrapment of irinotecan, Int. J. Pharm., 2018, 536(1), 82–94 CrossRef CAS PubMed.
- A. A. A. Smith, et al., Albumin–Polymer–Drug Conjugates: Long Circulating, High Payload Drug Delivery Vehicles, ACS Macro Lett., 2016, 5(10), 1089–1094 CrossRef CAS PubMed.
- G. Zhou, et al., Human serum albumin nanoparticles as a novel delivery system for cabazitaxel, Anticancer Res., 2016, 36(4), 1649–1656 CAS.
- S. Sebak, et al., Human serum albumin nanoparticles as an efficient noscapine drug delivery system for potential use in breast cancer: preparation and in vitro analysis, Int. J. Nanomed., 2010, 5, 525–532 CAS.
- Y. Jiang, et al., PEGylated Albumin-Based Polyion Complex Micelles for Protein Delivery, Biomacromolecules, 2016, 17(3), 808–817 CrossRef CAS PubMed.
- L. Chen, et al., A Redox-Sensitive Micelle-Like Nanoparticle Self-Assembled from Amphiphilic Adriamycin-Human Serum Albumin Conjugates for Tumor Targeted Therapy, BioMed Res. Int., 2015, 2015, 987404 Search PubMed.
- F. P. Seib, et al., Focal therapy of neuroblastoma using silk films to deliver kinase and chemotherapeutic agents in vivo, Acta Biomater., 2015, 20, 32–38 CrossRef CAS PubMed.
- L. P. Yan, et al., Core-shell silk hydrogels with spatially tuned conformations as drug-delivery system, J. Tissue Eng. Regener. Med., 2017, 11(11), 3168–3177 CrossRef CAS PubMed.
- L. Xiao, et al., Direct formation of silk nanoparticles for drug delivery, ACS Biomater. Sci. Eng., 2016, 2(11), 2050–2057 CrossRef CAS PubMed.
- E. Wenk, et al., Silk fibroin spheres as a platform for controlled drug delivery, J. Controlled Release, 2008, 132(1), 26–34 CrossRef CAS PubMed.
- B. Mao, et al., Cyclic cRGDfk peptide and Chlorin e6 functionalized silk fibroin nanoparticles for targeted drug delivery and photodynamic therapy, Biomaterials, 2018, 161, 306–320 CrossRef CAS PubMed.
- Y. Zhou, et al., Photopolymerized maleilated chitosan/methacrylated silk fibroin micro/nanocomposite hydrogels as potential scaffolds for cartilage tissue engineering, Int. J. Biol. Macromol., 2018, 108, 383–390 CrossRef CAS PubMed.
- M. A. Marin, R. R. Mallepally and M. A. McHugh, Silk fibroin aerogels for drug delivery applications, J. Supercrit. Fluids, 2014, 91, 84–89 CrossRef CAS.
- Y. Dong, et al., Fabrication and characterization of silk fibroin-coated liposomes for ocular drug delivery, Eur. J. Pharm. Biopharm., 2015, 91, 82–90 CrossRef CAS PubMed.
- K.-L. Mao, et al., Skin-penetrating polymeric nanoparticles incorporated in silk fibroin hydrogel for topical delivery of curcumin to improve its therapeutic effect on psoriasis mouse model, Colloids Surf., B, 2017, 160, 704–714 CrossRef CAS.
- B. Subia, et al., Folate conjugated silk fibroin nanocarriers for targeted drug delivery, Integr. Biol., 2014, 6(2), 203–214 CrossRef CAS.
- A. K. M. M. Alam and Q. T. H. Shubhra, Surface modified thin film from silk and gelatin for sustained drug release to heal wound, J. Mater. Chem. B, 2015, 3(31), 6473–6479 RSC.
- H. Zhang, et al., Multifunctional iron oxide/silk-fibroin (Fe3O4–SF) composite microspheres for the delivery of cancer therapeutics, RSC Adv., 2014, 4(78), 41572–41577 RSC.
- S. Wang, et al., Colloidal Stability of Silk Fibroin Nanoparticles Coated with Cationic Polymer for Effective Drug Delivery, ACS Appl. Mater. Interfaces, 2015, 7(38), 21254–21262 CrossRef CAS PubMed.
- S. Cao, et al., Shape-dependent biodistribution of biocompatible silk microcapsules, ACS Appl. Mater. Interfaces, 2019, 11(5), 5499–5508 CrossRef CAS PubMed.
- H. Wang, et al., Fabrication of silk microcapsules by layer-by-layer desolvation, Mater. Lett., 2019, 237, 109–112 CrossRef CAS.
- J. Kundu, et al., Silk fibroin nanoparticles for cellular uptake and control release, Int. J. Pharm., 2010, 388(1–2), 242–250 CrossRef CAS PubMed.
- T. Wongpinyochit, et al., PEGylated silk nanoparticles for anticancer drug delivery, Biomacromolecules, 2015, 16(11), 3712–3722 CrossRef CAS PubMed.
- D. Hu, et al., Self-stabilized silk sericin-based nanoparticles: In vivo biocompatibility and reduced DOX-induced toxicity, Acta Biomater., 2018, 74, 385–396 CrossRef CAS PubMed.
- J. Wu, et al., Control of silk microsphere formation using polyethylene glycol (PEG), Acta Biomater., 2016, 39, 156–168 CrossRef CAS PubMed.
- Q. Wang, et al., Facile fabrication of silk fibroin microspheres via electrostatic assembly, Mater. Res. Express, 2018, 5(7), 075401 CrossRef.
- Z. Cao, et al., The preparation of regenerated silk fibroin microspheres, Soft Matter, 2007, 3(7), 910–915 RSC.
- X. Wang, et al., Injectable silk-polyethylene glycol hydrogels, Acta Biomater., 2015, 12, 51–61 CrossRef CAS.
- Z. Yin, et al., Swellable silk fibroin microneedles for transdermal drug delivery, Int. J. Biol. Macromol., 2018, 106, 48–56 CrossRef CAS PubMed.
- S. Wang, et al., Insulin-loaded silk fibroin microneedles as sustained release system, ACS Biomater. Sci. Eng., 2019, 5(4), 1887–1894 CrossRef CAS.
- K. Tsioris, et al., Fabrication of silk microneedles for controlled-release drug delivery, Adv. Funct. Mater., 2012, 22(2), 330–335 CrossRef CAS.
- S. S. Silva, et al., Fabrication and characterization of Eri silk fibers-based sponges for biomedical application, Acta Biomater., 2016, 32, 178–189 CrossRef CAS PubMed.
- E. M. Pritchard, et al., Antibiotic-releasing silk biomaterials for infection prevention and treatment, Adv. Funct. Mater., 2013, 23(7), 854–861 CrossRef CAS PubMed.
- T. Mirshahi, et al., Adaptive Immune Responses of Legumin Nanoparticles, J. Drug Targeting, 2002, 10(8), 625–631 CrossRef CAS.
- A. González, et al., Crosslinked soy protein films and their application as ophthalmic drug delivery system, Mater. Sci. Eng. C, 2015, 51, 73–79 CrossRef.
- P. Guerrero, et al., Functional properties of films based on soy protein isolate and gelatin processed by compression molding, J. Food Eng., 2011, 105(1), 65–72 CrossRef CAS.
- M. Maviah, et al., Food Protein-Based Nanodelivery Systems for Hydrophobic and Poorly Soluble Compounds, AAPS PharmSciTech, 2020, 21, 101 CrossRef CAS PubMed.
- A. Maltais, G. E. Remondetto and M. Subirade, Tabletted soy protein cold-set hydrogels as carriers of nutraceutical substances, Food Hydrocolloids, 2010, 24(5), 518–524 CrossRef CAS.
- M. A. Farooq, et al., Whey protein: A functional and promising material for DDS recent developments and future prospects, Polym. Adv. Technol., 2019, 30(9), 2183–2191 CrossRef CAS.
- S. Kumar and S. K. Singh, In silico-in vitro-in vivo studies of experimentally designed carvedilol loaded silk fibroin-casein nanoparticles using physiological based pharmacokinetic model, Int. J. Biol. Macromol., 2017, 96, 403–420 CrossRef CAS PubMed.
- J. Zhu, Bioactive modification of poly(ethylene glycol) hydrogels for tissue engineering, Biomaterials, 2010, 31(17), 4639–4656 CrossRef CAS PubMed.
- A. O. Elzoghby, et al., Swellable floating tablet based on spray-dried casein nanoparticles: near-infrared spectral characterization and floating matrix evaluation, Int. J. Pharm., 2015, 491(1–2), 113–122 CrossRef CAS PubMed.
- L. Chen and M. Subirade, Alginate–whey protein granular microspheres as oral delivery vehicles for bioactive compounds, Biomaterials, 2006, 27(26), 4646–4654 CrossRef CAS.
- G. M. Tavares, et al., Milk proteins as encapsulation devices and delivery vehicles: Applications and trends, Trends Food Sci. Technol., 2014, 37(1), 5–20 CrossRef CAS.
- A. Mohandas, et al., Chitosan based metallic nanocomposite scaffolds as antimicrobial wound dressings, Bioact. Mater., 2018, 3(3), 267–277 CrossRef.
- M. A. Arangoa, et al., Bioadhesive potential of gliadin nanoparticulate systems, Eur. J. Pharm. Sci., 2000, 11(4), 333–341 CrossRef CAS PubMed.
- C. Duclairoir, et al., Evaluation of gliadins nanoparticles as DDS: a study of three different drugs, Int. J. Pharm., 2003, 253(1), 133–144 CrossRef CAS PubMed.
- W. He, et al., Formulating food protein-stabilized indomethacin nanosuspensions into pellets by fluid-bed coating technology: physical characterization, redispersibility, and dissolution, Int. J. Nanomed., 2013, 8, 3119 Search PubMed.
- W. He, et al., Food proteins as novel nanosuspension stabilizers for poorly water-soluble drugs, Int. J. Pharm., 2013, 441(1–2), 269–278 CrossRef CAS PubMed.
- T. Geng, et al., Comparative study on stabilizing ability of food protein, non-ionic surfactant and anionic surfactant on BCS type II drug carvedilol loaded nanosuspension: Physicochemical and pharmacokinetic investigation, Eur. J. Pharm. Sci., 2017, 109, 200–208 CrossRef CAS.
- E. Assadpour, S.-M. Jafari and Y. Maghsoudlou, Evaluation of folic acid release from spray dried powder particles of pectin-whey protein nano-capsules, Int. J. Biol. Macromol., 2017, 95, 238–247 CrossRef CAS PubMed.
- W. He, et al., Food protein-stabilized nanoemulsions as potential delivery systems for poorly water-soluble drugs: preparation, in vitro characterization, and pharmacokinetics in rats, Int. J. Nanomed., 2011, 6, 521 CAS.
- M. Li, Y. Ma and J. Cui, Whey-protein-stabilized nanoemulsions as a potential delivery system for water-insoluble curcumin, LWT--Food Sci. Technol., 2014, 59(1), 49–58 CrossRef CAS.
- A. F. Esfanjani, S. M. Jafari and E. Assadpour, Preparation of a multiple emulsion based on pectin-whey protein complex for encapsulation of saffron extract nanodroplets, Food Chem., 2017, 221, 1962–1969 CrossRef PubMed.
- S. Parthasarathi and C. Anandharamakrishnan, Enhancement of oral bioavailability of vitamin E by spray-freeze drying of whey protein microcapsules, Food Bioprod. Process., 2016, 100, 469–476 CrossRef.
- Z. Fang, et al., Partition and digestive stability of α-tocopherol and resveratrol/naringenin in whey protein isolate emulsions, Int. Dairy J., 2019, 93, 116–123 CrossRef CAS.
- W. Liu, et al., On enhancing the solubility of curcumin by microencapsulation in whey protein isolate via spray drying, J. Food Eng., 2016, 169, 189–195 CrossRef CAS.
- H. Hsein, et al., Atomization of denatured whey proteins as a novel and simple way to improve oral drug delivery system properties, Int. J. Biol. Macromol., 2017, 105, 801–809 CrossRef CAS.
- E. Déat-Lainé, et al., Efficacy of mucoadhesive hydrogel microparticles of whey protein and alginate for oral insulin delivery, Pharm. Res., 2013, 30(3), 721–734 CrossRef PubMed.
- G. J. O'Neill, et al., In vitro and in vivo evaluation of whey protein hydrogels for oral delivery of riboflavin, J. Funct. Foods, 2015, 19, 512–521 CrossRef.
- F. Alavi, et al., Cold gelation of curcumin loaded whey protein aggregates mixed with k-carrageenan: Impact of gel microstructure on the gastrointestinal fate of curcumin, Food Hydrocolloids, 2018, 85, 267–280 CrossRef CAS.
- S. Owonubi, et al., Characterization and in vitro release kinetics of antimalarials from whey protein-based hydrogel biocomposites, Int. J. Ind. Chem., 2018, 9(1), 39–52 CrossRef CAS.
- L. Lv, et al., Thermally-induced whey protein isolate-daidzein co-assemblies: Protein-based nanocomplexes as an inhibitor of precipitation/crystallization for hydrophobic drug, Food Chem., 2019, 275, 273–281 CrossRef CAS.
- A. Jain, et al., Lycopene loaded whey protein isolate nanoparticles: An innovative endeavor for enhanced bioavailability of lycopene and anti-cancer activity, Int. J. Pharm., 2018, 546(1–2), 97–105 CrossRef CAS PubMed.
- F. H. MAE, Preparation and Characterization of Sustained Released Zinc Citrate Encapsulated in Whey Protein Nanoparticles, Pak. J. Biol. Sci., 2018, 21(9), 448–453 CrossRef PubMed.
- S. Shao, X. Shen and M. Guo, Zinc-loaded whey protein nanoparticles prepared by enzymatic cross-linking and desolvation, Int. J. Food Sci. Technol., 2018, 53(9), 2205–2211 CrossRef CAS.
- H. Hsein, et al., Denatured whey protein powder as a new matrix excipient: design and evaluation of mucoadhesive tablets for sustained drug release applications, Pharm. Res., 2017, 34(2), 365–377 CrossRef CAS PubMed.
- R. Zhang, et al., Succinylated whey protein isolate as a sustained-release excipient of puerarin derivative oral tablets: Preparation, optimization and pharmacokinetics, Asian J. Pharm. Sci., 2018, 13(4), 383–394 CrossRef.
- J. Mishra, et al., Whey proteins as stabilizers in amorphous solid dispersions, Eur. J. Pharm. Sci., 2019, 128, 144–151 CrossRef CAS PubMed.
- M. Mohammadian, et al., Enhancing the aqueous solubility of curcumin at acidic condition through the complexation with whey protein nanofibrils, Food Hydrocolloids, 2019, 87, 902–914 CrossRef CAS.
- Y. Zhang, et al., Endogenous albumin-mediated delivery of redox-responsive paclitaxel-loaded micelles for targeted cancer therapy, Biomaterials, 2018, 183, 243–257 CrossRef CAS PubMed.
- E. Lee, J. Lee and S. Jon, A Novel Approach to Oral Delivery of Insulin by Conjugating with Low Molecular Weight Chitosan, Bioconjugate Chem., 2010, 21(10), 1720–1723 CrossRef CAS.
- A. A. P. Mansur, S. M. de Carvalho and H. S. Mansur, Bioengineered quantum dot/chitosan-tripeptide nanoconjugates for targeting the receptors of cancer cells, Int. J. Biol. Macromol., 2016, 82, 780–789 CrossRef CAS PubMed.
- S. Lee, N. S. A. Alwahab and Z. M. Moazzam, Zein-based oral drug delivery system targeting activated macrophages, Int. J. Pharm., 2013, 454(1), 388–393 CrossRef CAS.
- K. Hu, et al., Core–shell biopolymer nanoparticle delivery systems: Synthesis and characterization of curcumin fortified zein–pectin nanoparticles, Food Chem., 2015, 182, 275–281 CrossRef CAS.
- Z. Chen, et al., Bioresponsive Hyaluronic Acid-Capped Mesoporous Silica Nanoparticles for Targeted Drug Delivery, Chem. - Eur. J., 2013, 19(5), 1778–1783 CrossRef CAS PubMed.
- T. Muthukumar, J. E. Song and G. Khang, Biological Role of Gellan Gum in Improving Scaffold Drug Delivery, Cell Adhesion Properties for Tissue Engineering Applications, Molecules, 2019, 24(24), 4514 CrossRef CAS.
- S. Dhar, et al., Biocompatible gellan gum-reduced gold nanoparticles: cellular uptake and subacute oral toxicity studies, J. Appl. Toxicol., 2011, 31(5), 411–420 CrossRef CAS PubMed.
- S. Dhar, et al., Natural gum reduced/stabilized gold nanoparticles for drug delivery formulations, Chem. – Eur. J., 2008, 14(33), 10244–10250 CrossRef CAS PubMed.
- S. Vieira, et al., Gellan gum-coated gold nanorods: an intracellular nanosystem for bone tissue engineering, RSC Adv., 2015, 5(95), 77996–78005 RSC.
- A. Gal and A. Nussinovitch, Hydrocolloid carriers with filler inclusion for diltiazem hydrochloride release, J. Pharm. Sci., 2007, 96(1), 168–178 CrossRef CAS PubMed.
- P. Vashisth, et al., Ofloxacin loaded gellan/PVA nanofibers-Synthesis, characterization and evaluation of their gastroretentive/mucoadhesive drug delivery potential, Mater. Sci. Eng. C, 2017, 71, 611–619 CrossRef CAS PubMed.
- M. Rostami, et al., Development of resveratrol loaded chitosan-gellan nanofiber as a novel gastrointestinal delivery system, Int. J. Biol. Macromol., 2019, 135, 698–705 CrossRef CAS.
- M. Arjama, et al., Sericin/RBA embedded gellan gum based smart nanosystem for pH responsive drug delivery, Int. J. Biol. Macromol., 2018, 120, 1561–1571 CrossRef CAS.
- S. Pacelli, et al., Design of a tunable nanocomposite double network hydrogel based on gellan gum for drug delivery
applications, Eur. Polym. J., 2018, 104, 184–193 CrossRef CAS.
- E. Elmowafy, et al., In situ composite ion-triggered gellan gum gel incorporating amino methacrylate copolymer microparticles: a therapeutic modality for buccal applicability, Pharm. Dev. Technol., 2019, 24(10), 1258–1271 CrossRef CAS PubMed.
- O. Novac, et al., Antibacterial quaternized gellan gum based particles for controlled release of ciprofloxacin with potential dermal applications, Mater. Sci. Eng. C, 2014, 35, 291–299 CrossRef CAS PubMed.
- H. Bera, S. Kumar and S. Maiti, Facile synthesis and characterization of tailor-made pectin-gellan gum-bionanofiller composites as intragastric drug delivery shuttles, Int. J. Biol. Macromol., 2018, 118(Pt A), 149–159 CrossRef CAS.
- Q. Liang, et al., A gum Arabic assisted sustainable drug delivery system for adult Drosophila, Biol. Open, 2020, 9(6), 052241 Search PubMed.
- B. A. Aderibigbe, et al., Kinetic release studies of nitrogen-containing bisphosphonate from gum acacia crosslinked hydrogels, Int. J. Biol. Macromol., 2015, 73, 115–123 CrossRef CAS PubMed.
- V. P. Padmanabhan, R. Kulandaivelu and S. Nellaiappan, New core-shell hydroxyapatite/Gum-Acacia nanocomposites for drug delivery and tissue engineering applications, Mater. Sci. Eng., C, 2018, 92, 685–693 CrossRef CAS PubMed.
- B. Aderibigbe, et al., Controlled dual release study of curcumin and a 4-aminoquinoline analog from gum acacia containing hydrogels, J. Appl. Polym. Sci., 2015, 132(10), 41613 CrossRef.
- B. Singh and A. Dhiman, Design of Acacia Gum–Carbopol–Cross-Linked-Polyvinylimidazole Hydrogel Wound Dressings for Antibiotic/Anesthetic Drug Delivery, Ind. Eng. Chem. Res., 2016, 55(34), 9176–9188 CrossRef CAS.
|
This journal is © The Royal Society of Chemistry 2023 |
Click here to see how this site uses Cookies. View our privacy policy here.