DOI:
10.1039/D3RA04450E
(Paper)
RSC Adv., 2023,
13, 24789-24794
One-pot, three-component, iron-catalyzed synthesis of benzimidazoles via domino C–N bond formation†
Received
3rd July 2023
, Accepted 14th August 2023
First published on 21st August 2023
Abstract
An efficient one-pot, three-component process for the synthesis of benzimidazole derivatives using a catalytic amount of Fe(III) porphyrin has been developed. The reaction proceeds via domino C–N bond formation and cyclization reactions of benzo-1,2-quinone, aldehydes and ammonium acetate as a nitrogen source to selectively produce benzimidazole. A number of benzimidazole derivatives have been synthesized using this method in high yields under mild reaction conditions.
Introduction
Multicomponent reactions (MCRs) play an essential role in the pharmaceutical industry and in modern organic synthesis, as they provide a powerful tool for the cost and time-efficient synthesis of advanced drugs and target compounds by generating a complex structure from multiple reactants in a single step.1–5 Imidazole derivatives are one of the most important groups in heterocyclic compounds that have attracted much attention because they are found in a variety of natural products. In addition, benzimidazoles are the core structure of many pharmaceuticals, ionic liquids (ILs) as more environmentally friendly solvents, and N-heterocyclic carbenes as valuable ligands in transition-metal catalysis.3,6–8 There are several known routes to benzimidazole derivatives (Scheme 1). Reaction between o-phenylenediamine and carbonyl compounds such as aldehydes,9–13 ketones,14 acids,15 acyl chlorides,16 and as well as β-ketoesters,17,18 or orthoesters,19,20 or and benzylic including benzyl alcohols,21 benzyl amines,22 and toluene derivatives23 are the main methods for the synthesis of a large number of benzimidazole derivatives. Another important approach is the reaction of 2-aminonitrobenzenes with acids,24 aldehydes,25 and activated methyl groups.26 In another strategy, benzimidazoles were obtained by coupling of 2-iodoaniline with aldehydes.27 Arylamino oximes also led to benzimidazoles in the presence of a base.28 The synthesis of benzimidazoles by intramolecular N-arylation using copper catalysts was developed.29 The intramolecular cyclization of o-bromoaryl derivatives also resulted in benzimidazoles.30
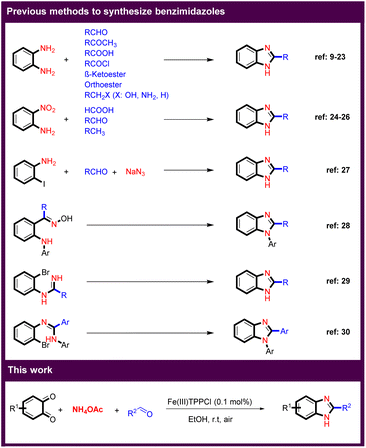 |
| Scheme 1 Different methods for the synthesis of benzimidazole scaffolds. | |
Despite numerous advances in the synthesis of benzimidazoles, the development of new synthetic methods is strongly considered because the existing methods have many drawbacks, such as low yields, complicated reaction conditions, use of toxic reagents and solvents. On the other hand, there is a high demand for efficient and clean synthesis of benzimidazole derivatives from other sources. Continuing our program on the synthesis of benzoxazoles,4,5,31,32 we would like to present here a novel and efficient protocol for the synthesis of benzimidazole derivatives. In this work, MCR of benzo-1,2-quinones, aryl aldehydes and ammonium acetate in the presence of catalytic amount of an Fe(III)-porphyrin catalyst at room temperature resulted in a benzimidazole core.
Metalloporphyrins have been used as catalyst in many organic reactions such as the hydroxylation and epoxidation of hydrocarbon compounds,33,34 the hydroxylation of aromatic compounds,35 the aziridination of olefins,36 oxidation of sulfides to sulfones,37 the ring-opening of epoxides,38 and the synthesis of heterocyclic compounds.10,39,40
Results and discussion
To optimize the new method for the synthesis of benzimidazoles, the reaction of 3,5-di-tert-butylcyclohexa-3,5-diene-1,2-dione (1), ammonium acetate (2), and 4-methoxybenzaldehyde (3a) was selected as a model reaction (Table 1). In the absence of catalyst, in ethanol as solvent and at 80 °C, only 5% product was observed (Table 1, entry 1). In an attempt to improve the reaction yield, some transition metals such as Fe, Zn, Ni, Cu, Cd and Mn were tested as catalysts (Table 1, entries 2–7). Among the catalysts tested, an increase of the reaction yield to 65% was observed using FeCl3. However, a benzoxazole by-product was also isolated using these catalysts (Table 1, entries 2–7). To enhance the yield and selectivity, different iron sources were tested (Table 1, entries 8–10). Surprisingly, the maximum product was obtained with a FeIII-porphyrin complex (FeIIITPPCl) at room temperature after only 2 h without the formation of the benzoxazole 5a side-product (Table 1, entry 11).
Table 1 Optimization of the reaction conditions for the synthesis of benzimidazoles via a one-pot multicomponent reactiona

|
# |
Cat. (mol%) |
Solv. |
T (°C) |
Time (h) |
Yield 4a/5ab (%) |
Reaction conditions: 1a (1.0 mmol), 2a (2.2 mmol), 3a (1.0 mmol), and solvent (5.0 mL) at r. t. Isolated yield. Under nitrogen atmosphere. |
1 |
— |
EtOH |
80 |
12 |
5/— |
2 |
FeCl3 (5) |
EtOH |
rt |
12 |
65/15 |
3 |
ZnCl2 (5) |
EtOH |
rt |
12 |
28/5 |
4 |
NiCl2 (5) |
EtOH |
rt |
12 |
36/10 |
5 |
CuCl2 (5) |
EtOH |
rt |
12 |
45/8 |
6 |
CdCl2 (5) |
EtOH |
rt |
12 |
25/5 |
7 |
MnCl2 (5) |
EtOH |
rt |
12 |
50/20 |
8 |
Fe(NO3)3 (5) |
EtOH |
rt |
12 |
45/20 |
9 |
FeBr3 (5) |
EtOH |
rt |
12 |
22/18 |
10 |
Fe(acac)3 (5) |
EtOH |
rt |
12 |
20/10 |
11 |
FeIIITPPCl (0.1) |
EtOH |
rt |
2 |
96/0 |
12 |
SnIITPP (0.1) |
EtOH |
rt |
7 |
65/0 |
13 |
PbIITPP (0.1) |
EtOH |
rt |
8 |
60/0 |
14 |
ZnIITPP (0.1) |
EtOH |
rt |
5 |
70/0 |
15 |
CdIITPP (0.1) |
EtOH |
rt |
9 |
60/0 |
16 |
NiIITPP (0.1) |
EtOH |
rt |
3 |
77/0 |
17 |
CuIITPP (0.1) |
EtOH |
rt |
4 |
80/0 |
18 |
FeIIITPPCl (0.05) |
EtOH |
rt |
12 |
78/0 |
19 |
FeIIITPPCl (0.15) |
EtOH |
rt |
2 |
94/0 |
20 |
FeIIITPPCl (0.1) |
MeCN |
rt |
3 |
70/10 |
21 |
FeIIITPPCl (0.1) |
H2O |
rt |
9 |
45/5 |
22 |
FeIIITPPCl (0.1) |
MeOH |
rt |
3 |
86/5 |
23 |
FeIIITPPCl (0.1) |
PhMe |
rt |
7 |
67/0 |
24 |
FeIIITPPCl (0.1) |
EtOH |
rt |
5 |
c55/0 |
Since the porphyrin complex was found to be the best catalyst for this reaction, the porphyrin complexes of other metals were also synthesized10,40 and tested to verify that iron was the best catalyst for this reaction (Table 1, entries 12–17). In the presence of SnIITPP, about 65% of the product was isolated after 7 hours at room temperature (Table 1, entry 12). With PbIITPP, 4a was prepared in 60% yield after 8 hours (Table 1, entry 13). Good yield was obtained with ZnIITPP (Table 1, entry 14). CdIITPP worked the same way as PbIITPP and 60% of the product was isolated (Table 1, entry 15). Interestingly, NiIITPP and CuIITPP gave comprisable yields of product (Table 1, entries 16 and 17). The interesting thing is that with the use of porphyrin complexes as catalysts, we have high selectivity for the formation of the benzimidazole product. Among the tested metal complexes, FeIIITPPCl showed high catalytic activity and was therefore selected as catalyst for this multicomponent synthesis of benzimidazoles.
For further optimization, different catalyst loadings were used for the reaction, and no further improvements were found (Table 1, entries 18 and 19). Different solvents were also investigated and no superiority was found (Table 1, entries 20–23). For example, in the solvents acetonitrile and methanol, 70% and 86% of the product were isolated respectively, albeit with a slight loss of selectivity. In addition, the reaction was carried out under N2 and a yield of 55% was obtained (Table 1, entry 24).
After optimizing the reaction conditions, various aryl aldehydes with electron donor and electron withdrawing groups were first investigated under optimized conditions to test the generality and scope of the method (Scheme 2).
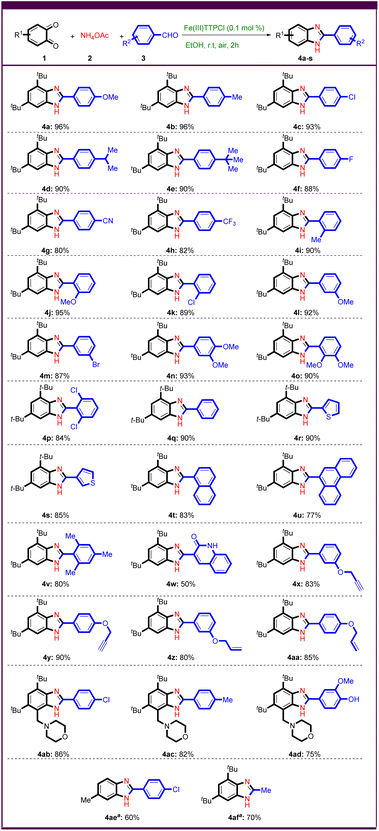 |
| Scheme 2 Synthesis of benzimidazolederivatives. Reaction conditions: 1 (1.0 mmol), 2 (2.2 mmol) and 3 (1.0 mmol) in the presence of FeTTPCl (0.1 mol%) in EtOH (5.0 mL) at room temperature under air condition for 2 h. All yields are isolated. a The reaction was performed at 60 °C, 6 h. | |
Aryl aldehydes with electron-donating groups in para position such as –OMe, –Me, –Cl, –iPr, and -tBu gave benzimidazoles 4a–e in more than 90% yield. Electron withdrawing groups such as –F, –CN, and –CF3 were tested in this reaction and gave good yields of benzimidazoles 4f–h. The ortho- or meta-substituted benzaldehyde worked well and provided the desired products in good to excellent yields, regardless of their electronic nature (Scheme 2, 4i–m).
To further investigate the reaction possibilities, aryl aldehydes with two substituents such as 3,4-dimethoxybenzaldehyde, 2,3-dimethoxybenzaldehyde and 2,6-dichlorobenzaldehyde were tested (Scheme 2, 4n–p). Remarkably, various heteroaryl aldehydes such as 2-thiophene carboxaldehyde and 3-thiophene carboxaldehyde gave good yields of the desired products (Scheme 2, 4r,s). Encouragingly, 1-naphthaldehyde, and phenanthrene-9-carbaldehyde were subjected to Fe(III)-catalyzed domino C–N bond formation as polyaromatic substrates, resulting in the corresponding benzimidazoles in 83%, and 77% yields, respectively (Scheme 2, 4t,u). Sterically hindered substrates were found to lead to the corresponding benzimidazoles in good yields (Scheme 2, 4p,v).
The use of quinoline-3-carbaldehyde as substrate gave a bis-heterocyclic product 4w in 50% yield.
Alkene and alkyne functional groups tolerated the reaction conditions well, giving allyl- and propargyl-functionalized benzimidazoles in high yields (Scheme 2, 4x–aa). To expand the scope of this method, we attempted to synthesize poly-substituted benzo-1,2-quinone derivative with heterocycle.41 The synthetic benzo-1,2-quinone derivative derived from morpholine worked quite well and yielded benzimidazoles in good yields (Scheme 2, 4ab–ad). The desired product 4ae was also successfully prepared from other benzo-1,2-quinones. Remarkably, acetaldehyde as an aliphatic substrate led to the synthesis of benzimidazole 4af in 70% yield.
To demonstrate the efficiency of the synthesis, we performed a large-scale reaction producing compound 4a in 90% yield (Scheme 3).
 |
| Scheme 3 Gram-scale Synthesis. | |
A plausible reaction mechanism for the Fe(III)-porphyrin-catalyzed formation of the benzimidazoles from benzo-1,2-quinone, ammonium acetate, and aldehydes is shown in Scheme 4.
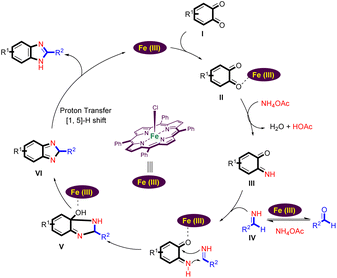 |
| Scheme 4 The proposed mechanism. | |
Coordination of the carbonyl group of benzo-1,2-quinone (I) with the Lewis acid site of Fe(III) porphyrin leads to its activation. It now reacts with ammonium acetate to form the imine intermediate (III).42,43 At the same time, aldehyde can react with an excess of ammonium acetate to form a Schiff base IV.44–48 Schiff base IV is able to react with intermediate (III) by intermolecular cyclization to form intermediate V. When the intermediate V is formed, a dehydration process could take place to obtain the intermediate (VI). Finally, the desired benzimidazole is generated by [1,5]-H shift (VI) and the Fe(III) porphyrin catalyst is regenerated to start the next cycle.2,49
Conclusions
In summary, we have demonstrated an efficient, novel, green and simple procedure for the multicomponent one-pot synthesis of benzimidazoles in the presence of Fe(III) porphyrin complexes of benzo-1,2-quinone, NH4OAc as nitrogen source and aldehydes. Key features of this process include mild reaction conditions, large-scale synthesis and the use of environmentally friendly organic solvents in the reaction process, providing an efficient method for the preparation of benzimidazoles.
Conflicts of interest
There are no conflicts to declare.
Acknowledgements
We would like to thank the financial supports of Iran National Science Foundation (INSF), Grant no. 99004864. We are thankful to the Iran's Science Elites Federation for their support. Also, the financial support from the research councils of Shiraz University is gratefully acknowledged.
References
- T. Meng, Y. Zou, O. Khorev, Y. Jin, H. Zhou, Y. Zhang, D. Hu, L. Ma, X. Wang and J. Shen, Simple and Efficient Copper(I)-Catalyzed Access to Three Versatile Aminocoumarin-Based Scaffolds Using Isocyanoacetate, Adv. Synth. Catal., 2011, 353, 918–924 CrossRef CAS.
- H. Sharghi, M. Aberi and M. M. Doroodmand, A Mild, Three-Component One-Pot Synthesis of 2,4,5-Trisubstituted Imidazoles Using Mo(IV) Salen Complex in Homogeneous Catalytic System and Mo(IV) Salen Complex Nanoparticles onto Silica as a Highly Active, Efficient, and Reusable Heterogeneous Nanocataly, Mol. Diversity, 2015, 19, 77–85 CrossRef CAS PubMed.
- M. A. Zolfigol, A. Khazaei, A. R. Moosavi-Zare, A. Zare, Z. Asgari, V. Khakyzadeh and A. Hasaninejad, Design of Ionic Liquid 1,3-Disulfonic Acid Imidazolium Hydrogen Sulfate as a Dual-Catalyst for the One-Pot Multi-Component Synthesis of 1,2,4,5-Tetrasubstituted Imidazoles, J. Ind. Eng. Chem., 2013, 19, 721–726 CrossRef CAS.
- H. Sharghi, J. Aboonajmi and M. Aberi, One-Pot Multicomponent Reaction of Catechols, Ammonium Acetate, and Aldehydes for the Synthesis of Benzoxazole Derivatives Using the Fe(III)-Salen Complex, J. Org. Chem., 2020, 85, 6567–6577 CrossRef CAS PubMed.
- J. Aboonajmi, F. Panahi and H. Sharghi, One-Pot Multicomponent Coupling Reaction of Catechols, Benzyl Alcohols/Benzyl Methyl Ethers, and Ammonium Acetate toward Synthesis of Benzoxazoles, ACS Omega, 2021, 6, 22395–22399 CrossRef CAS PubMed.
- R. Bansal, P. Soni, M. Ahirwar and A. Halve, One-Pot Multi-Component Synthesis of Some Pharmacologically Significant 2,4,5-Tri and 1,2,4,5-Tetrasubstituted Imidazoles: A Review, Int. Res. J. Pure Appl. Chem., 2016, 11, 1–26 CrossRef CAS.
- A. Nakhaei and A. Davoodnia, Application of a Keplerate Type Giant Nanoporous Isopolyoxomolybdate as a Reusable Catalyst for the Synthesis of 1,2,4,5-Tetrasubstituted Imidazoles, Chin. J. Catal., 2014, 35, 1761–1767 CrossRef CAS.
- I. Beltran-Hortelano, V. Alcolea, M. Font and S. Pérez-Silanes, The Role of Imidazole and Benzimidazole Heterocycles in Chagas Disease: A Review, Eur. J. Med. Chem., 2020, 206, 112692 CrossRef CAS PubMed.
- E. Niknam, F. Panahi, F. Daneshgar, F. Bahrami and A. Khalafi-Nezhad, Metal-Organic Framework MIL-101(Cr) as an Efficient Heterogeneous Catalyst for Clean Synthesis of Benzoazoles, ACS Omega, 2018, 3, 17135–17144 CrossRef CAS PubMed.
- H. Sharghi, M. H. Beyzavi and M. M. Doroodmand, Reusable Porphyrinatoiron(III) Complex Supported on Activated Silica as an Efficient Heterogeneous Catalyst for a Facile, One-Pot, Selective Synthesis of 2-Arylbenzimidazole Derivatives in the Presence of Atmospheric Air as a “Green” Oxidant at Ambient Temperature, Eur. J. Org. Chem., 2008, 2008, 4126–4138 CrossRef.
- H. Kottayil, S. Machingal, S. Mole Parackal, B. S. Alungal, M. L. V. Theresa, A. Govindan and S. Krishnapillai, Development of Homogeneous Polyamine Organocatalyst for the Synthesis of 2-Aryl-Substituted Benzimidazole and Benzoxazole Derivatives, J. Heterocycl. Chem., 2020, 57, 3310–3317 CAS.
- H. Sharghi, M. Aberi and M. M. Doroodmand, Reusable Cobalt(III)-Salen Complex Supported on Activated Carbon as an Efficient Heterogeneous Catalyst for Synthesis of 2-Arylbenzimidazole Derivatives, Adv. Synth. Catal., 2008, 350, 2380–2390 CrossRef CAS.
- H. Sharghi, S. F. Razavi, M. Aberi, F. Tavakoli and M. Shekouhy, The Co2+ Complex of [7-Hydroxy-4-Methyl-8-Coumarinyl]Glycine as a Nanocatalyst for the Synthesis and Biological Evaluation of New Mannich Bases of Benzimidazoles and Benzothiazoles, ChemistrySelect, 2020, 5, 2662–2671 CrossRef CAS.
- O. Ravi, A. Shaikh, A. Upare, K. K. Singarapu and S. R. Bathula, Benzimidazoles from Aryl Alkyl Ketones and 2-Amino Anilines by an Iodine Catalyzed Oxidative C(CO)-C(Alkyl) Bond Cleavage, J. Org. Chem., 2017, 82, 4422–4428 CrossRef CAS PubMed.
- S. B. Bhagat, Y. B. Sutar, Y. Manohar and V. N. Telvekar, Diphosphorus Tetraiodide (P2I4): An Efficient Catalyst for Synthesis of 2-Aryl-1,3-Benzazoles via Cyclocondensation of o-Amino/Mercaptan/Hydroxy Anilines with Aryl Acids, Asian J. Chem., 2018, 30, 376–380 CrossRef CAS.
- V. K. Tandon and M. Kumar, BF3·Et2O Promoted One-Pot Expeditious and Convenient Synthesis of 2-Substituted Benzimidazoles and 3,1,5-Benzoxadiazepines, Tetrahedron Lett., 2004, 45, 4185–4187 CrossRef CAS.
- Z. Li, J. Dong, X. Chen, Q. Li, Y. Zhou and S. F. Yin, Metal- and Oxidant-Free Synthesis of Quinazolinones from β-Ketoesters with o-Aminobenzamides via Phosphorous Acid-Catalyzed Cyclocondensation and Selective C–C Bond Cleavage, J. Org. Chem., 2015, 80, 9392–9400 CrossRef CAS PubMed.
- M. S. Mayo, X. Yu, X. Zhou, X. Feng, Y. Yamamoto and M. Bao, Convenient Synthesis of Benzothiazoles and Benzimidazoles through Brønsted Acid Catalyzed Cyclization of 2-Amino Thiophenols/Anilines with β-Diketones, Org. Lett., 2014, 16, 764–767 CrossRef CAS PubMed.
- H. Sharghi, M. Mozaffari, J. Aboonajmi, M. M. Doroodmand, P. Shiri and M. Aberi, Synergetic Effect of Iron-Doped Acidic Multi-Walled Carbon Nanotubes in the Synthesis of Diverse Substituted Five-Membered Heterocyclic Compounds, ChemistrySelect, 2018, 3, 13534–13540 CrossRef CAS.
- G. Bastug, C. Eviolitte and I. E. Markó, Functionalized Orthoesters as Powerful Building Blocks for the Efficient Preparation of Heteroaromatic Bicycles, Org. Lett., 2012, 14, 3502–3505 CrossRef CAS PubMed.
- A. J. Blacker, M. M. Farah, M. I. Hall, S. P. Marsden, O. Saidi and J. M. J. Williams, Synthesis of Benzazoles by Hydrogen-Transfer Catalysis, Org. Lett., 2009, 11, 2039–2042 CrossRef CAS PubMed.
- R. Zhang, Y. Qin, L. Zhang and S. Luo, Oxidative Synthesis of Benzimidazoles, Quinoxalines, and Benzoxazoles from Primary Amines by Ortho-Quinone Catalysis, Org. Lett., 2017, 19, 5629–5632 CrossRef CAS PubMed.
- A. Dandia, D. K. Mahawar, R. Sharma, R. S. Badgoti, K. S. Rathore and V. Parewa, Graphene Oxide-Catalyzed CSp3–H Activation of Methylarenes in Aqueous Medium: A Unified Metal-Free Access to Amides and Benzimidazoles, Appl. Organomet. Chem., 2019, 33(11), e5232 CrossRef CAS.
- E. J. Hanan, B. K. Chan, A. A. Estrada, D. G. Shore and J. P. Lyssikatos, Mild and General One-Pot Reduction and Cyclization of Aromatic and Heteroaromatic 2-Nitroamines to Bicyclic 2H-Imidazoles, Synlett, 2010, 2759–2764 CrossRef CAS.
- D. Yang, D. Fokas, J. Li, L. Yu and C. M. Baldino, A Versatile Method for the Synthesis of Benzimidazoles from O-Nitroanilines and Aldehydes in One Step via a Reductive Cyclization, Synthesis, 2005, 47–56 Search PubMed.
- T. B. Nguyen, L. Ermolenko and A. Al-Mourabit, Iron Sulfide Catalyzed Redox/Condensation Cascade Reaction between 2-Amino/Hydroxy Nitrobenzenes and Activated Methyl Groups: A Straightforward Atom Economical Approach to 2-Hetaryl-Benzimidazoles and -Benzoxazoles, J. Am. Chem. Soc., 2013, 135, 118–121 CrossRef CAS PubMed.
- Y. Kim, M. R. Kumar, N. Park, Y. Heo and S. Lee, Copper-Catalyzed, One-Pot, Three-Component Synthesis of Benzimidazoles by Condensation and C–N Bond Formation, J. Org. Chem., 2011, 76, 9577–9583 CrossRef CAS PubMed.
- B. C. Wray and J. P. Stambuli, Synthesis of N-Arylindazoles and Benzimidazoles from a Common Intermediate, Org. Lett., 2010, 12, 4576–4579 CrossRef CAS PubMed.
- J. Peng, M. Ye, C. Zong, F. Hu, L. Feng, X. Wang, Y. Wang and C. Chen, Copper-Catalyzed Intramolecular C-N Bond Formation: A Straightforward Synthesis of Benzimidazole Derivatives in Water, J. Org. Chem., 2011, 76, 716–719 CrossRef CAS PubMed.
- P. Saha, T. Ramana, N. Purkait, M. A. Ali, R. Paul and T. Punniyamurthy, Ligand-Free Copper-Catalyzed Synthesis of Substituted Benzimidazoles, 2-Aminobenzimidazoles, 2-Aminobenzothiazoles, and Benzoxazoles, J. Org. Chem., 2009, 74, 8719–8725 CrossRef CAS PubMed.
- J. Aboonajmi, F. Panahi, M. A. Hosseini, M. Aberi and H. Sharghi, Iodine-Catalyzed Synthesis of Benzoxazoles Using Catechols, Ammonium Acetate, and Alkenes/Alkynes/Ketones via C-C and C-O Bond Cleavage, RSC Adv., 2022, 12, 20968–20972 RSC.
- H. Sharghi, M. Aali Hosseini, J. Aboonajmi and M. Aberi, Use of Vitamin B12as a Nontoxic and Natural Catalyst for the Synthesis of Benzoxazoles via Catechols and Primary Amines in Water under Aerobic Oxidation, ACS Sustain. Chem. Eng., 2021, 9, 11163–11170 CrossRef CAS.
- H. Srour, P. Le Maux and G. Simonneaux, Enantioselective Manganese-Porphyrin-Catalyzed Epoxidation and C-H Hydroxylation with Hydrogen Peroxide in Water/Methanol Solutions, Inorg. Chem., 2012, 51, 5850–5856 CrossRef CAS PubMed.
- J. C. Barona-Castaño, C. C. Carmona-Vargas, T. J. Brocksom, K. T. De Oliveira, M. Graça, P. M. S. Neves, M. Amparo and F. Faustino, Porphyrins as Catalysts in Scalable Organic Reactions, Molecules, 2016, 21, 310 CrossRef PubMed.
- M. Asaka and H. Fujii, Participation of Electron Transfer Process in Rate-Limiting Step of Aromatic Hydroxylation Reactions by Compound i Models of Heme Enzymes, J. Am. Chem. Soc., 2016, 138, 8048–8051 CrossRef CAS PubMed.
- R. Vyas, G. Y. Gao, J. D. Harden and X. P. Zhang, Iron(III) Porphyrin Catalyzed Aziridination of Alkenes with Bromamine-T as Nitrene Source, Org. Lett., 2004, 6, 1907–1910 CrossRef CAS PubMed.
- A. Ghaemi, S. Rayati, S. Zakavi and N. Safari, Highly Efficient Oxidation of Sulfides to Sulfones with Tetra-n-Butylammonium Hydrogen Monopersulfate Catalyzed by β-Tri- and Tetra-Brominated Meso-Tetraphenylporphyrinatomanganese(III) Acetate, Appl. Catal., A, 2009, 353, 154–159 CrossRef CAS.
- K. Venkatasubbaiah, X. Zhu, E. Kays, K. I. Hardcastle and C. W. Jones, Co(III)-Porphyrin-Mediated Highly Regioselective Ring-Opening of Terminal Epoxides with Alcohols and Phenols, ACS Catal., 2011, 1, 489–492 CrossRef CAS.
- J. Aboonajmi, H. Sharghi, M. Aberi and P. Shiri, Consecutive Oxidation/Condensation/Cyclization/Aromatization Sequences Catalyzed by Nanostructured Iron(III)-Porphyrin Complex towards Benzoxazole Derivatives, Eur. J. Org. Chem., 2020, 2020, 5978–5984 CrossRef CAS.
- H. Sharghi, M. H. Beyzavi, A. Safavi, M. M. Doroodmand and R. Khalifeh, Immobilization of Porphyrinatocopper Nanoparticles onto Activated Multi-Walled Carbon Nanotubes and a Study of Its Catalytic Activity as an Efficient Heterogeneous Catalyst for a Click Approach to the Three-Component Synthesis of 1,2,3-Triazoles in Water, Adv. Synth. Catal., 2009, 351, 2391–2410 CrossRef CAS.
- Y. A. Sayapin, I. O. Tupaeva, V. V. Tkachev and G. V. Shilov, 6,6’-[Piperazine-1,4-Diylbis(Methylene)]Bis[3,5-Di(tert-Butyl)-1,2- Benzoquinone]: Synthesis and Properties, Russ. J. Org. Chem., 2016, 52, 214–218 CrossRef CAS.
- M. Baltrun, F. A. Watt, R. Schoch, C. Wölper, A. G. Neuba and S. Hohloch, A New Bis-Phenolate Mesoionic Carbene Ligand for Early Transition Metal Chemistry, Dalton Trans., 2019, 48, 14611–14625 RSC.
- V. I. Lodyato, I. L. Yurkova, V. L. Sorokin, O. I. Shadyro, V. I. Dolgopalets and M. A. Kisel, Synthesis and Properties of 11-(3,5-Di-tert-Butyl-2-Hydroxyphenylcarbamoyl)Undecanoic Acid, a New Amphiphilic Antioxidant, Bioorg. Med. Chem. Lett., 2003, 13, 1179–1182 CrossRef CAS PubMed.
- M. Zhou, K. Li, D. Chen, R. Xu, G. Xu, G. Xu, W. Tang, W. Tang and W. Tang, Enantioselective Reductive Coupling of Imines Templated by Chiral Diboron, J. Am. Chem. Soc., 2020, 142, 10337–10342 CrossRef CAS PubMed.
- L. Villar, N. V. Orlov, N. S. Kondratyev, U. Uria, J. L. Vicario and A. V. Malkov, Kinetic Resolution of Secondary Allyl Boronates and Their Application in the Synthesis of Homoallylic Amines, Chem.–Eur. J., 2018, 24, 16262–16265 CrossRef CAS PubMed.
- T. G. Elford and D. G. Hall, Imine Allylation Using 2-Alkoxycarbonyl Allylboronates as an Expedient Three-Component Reaction to Polysubstituted α-Exo-Methylene-γ-Lactams, Tetrahedron Lett., 2008, 49, 6995–6998 CrossRef CAS.
- S. Sen, S. R. Kamma, R. Gundla, U. Adepally, S. Kuncha, S. Thirnathi and U. V. Prasad, A Reagent Based DOS Strategy via Evans Chiral Auxiliary: Highly Stereoselective Michael Reaction towards Optically Active Quinolizidinones, Piperidinones and Pyrrolidinones, RSC Adv., 2013, 3, 2404–2411 RSC.
- Y. Chen, R. Yang, F. Xiao, T. Li, G. Mao and G. J. Deng, Four-Component Synthesis of 9H-Pyrimido[4,5-b]Indoles Using Ammonium Iodide as the Nitrogen Source, Catalysts, 2023, 13, 623 CrossRef CAS.
- N. L. Higuera, D. Peña-Solórzano and C. Ochoa-Puentes, Urea-Zinc Chloride Eutectic Mixture-Mediated One-Pot Synthesis of Imidazoles: Efficient and Ecofriendly Access to Trifenagrel, Synlett, 2019, 30, 225–229 CrossRef CAS.
Footnote |
† Electronic supplementary information (ESI) available: Experimental procedures, spectral data and copies of 1H and 13C NMR for synthesized compounds. See DOI: https://doi.org/10.1039/d3ra04450e |
|
This journal is © The Royal Society of Chemistry 2023 |
Click here to see how this site uses Cookies. View our privacy policy here.