DOI:
10.1039/D3RA04592G
(Paper)
RSC Adv., 2023,
13, 24692-24698
Study on the relationships between the oil HLB value and emulsion stabilization
Received
10th July 2023
, Accepted 1st August 2023
First published on 18th August 2023
Abstract
In order to study the relationship between the HLB value of oil and emulsion stabilization, the optimal formation of emulsification system was determined, and then, the properties of emulsion, such as particle size, stability, interfacial tension and zeta potential, were tested by laser particle analyzer, stability analyzer, and interfacial tensiometer. Experimental results showed that the optimal ratio of emulsification was Tween 80
:
Span 80 = 5
:
5. Meanwhile, when the HLB value of the emulsification system was close to that of oil, the emulsion exhibited the best stability. This phenomenon is due to the fact that when the HLB values are close, the surfactant molecules are arranged more closely on the oil–water interface, leading to smaller sized emulsion droplet, which is conducive to emulsion stability. This study provides new insights into the effective adjustment of emulsion stability.
1. Introduction
With the development of society, petroleum is consumed in a large quantity. Many scientists recognized this problem and focused to develop new energy resources, such as solar energy, wind energy, and tidal energy. Considering the progress in the new energy field, it will take a long time to replace petroleum; therefore, it is necessary to enhance oil recovery.
Nowadays, there are a lot of ways to enhance oil recovery, such as polymer flooding, surfactant flooding, alkaline flooding, and microbial flooding.1–3 In polymer flooding, improper injection blocks formation pores and causes reservoir damage.4 Alkali flooding can enhance oil recovery via generating surfactants through the reaction with petroleum acid.5 However, alkali can cause serious corrosion to pipelines. Although microbial flooding has great performance, the high cost limits its large-scale use.6,7
Owing to its low cost and great performance, surfactant flooding is attracting people's interest.8–11 Surfactant flooding enhances oil recovery via four main aspects: interfacial tension reduction, wettability alteration, foam generation, and emulsification.12,13 Several petroleum researchers have conducted excellent studies on surfactant flooding. Wu et al. investigated the possibility of sodium dodecyl sulfate (SDS) to enhance oil recovery. The core flooding experimental result showed that 0.2 wt% SDS can enhance oil recovery by 4.43%.14 Jia et al. studied SDS and 1-dodecyl-3-methylimidazolium chloride compound system's enhanced oil recovery ability.15 The experimental result showed that the combined system can enhance oil recovery by 10% under high temperature and saline reservoir. Zhang et al. using acid amidopropyl betaine (EDAB) and SDS constructed a compound surfactant system.16 Core flooding experiment was carried out under the conditions of X reservoir. Experimental results showed that the compound surfactant system have great oil displacement performance. The enhanced oil recovery ability of surfactant flooding and subsequent water flooding reached 22.6%. Recently, nanoparticles have attracted considerable attractions from petroleum researchers.17,18 Cheraghian formed a nano-TiO2/SDS compound surfactant system.19 Compared with SDS, this compound surfactant system enhanced oil recovery by 4.85%.
In surfactant flooding, emulsification is the most important among the above-mentioned four enhanced oil recovery mechanisms.20 Now surfactant flooding used in oilfields have good performance;21 however, their application range is narrow and only suitable for specific oilfields and it needs a long time to adjust for different reservoir condition. How to obtain the surfactant's screening standard for surfactant flooding with good emulsification ability is of great significance for the further use of surfactant flooding in oilfield.22 HLB value was proposed by W. C. Griffin in 1949.23 It is used to represent the balance of the magnitude and strength of hydrophilic and lipophilic groups in molecules.24 HLB value can be used to filter surfactant systems and crude oil with good matching relationship? According to the literature, a series of different HLB value surfactant solutions were prepared and emulsion was formed with crude oil. When the stability of the emulsion is the best, the HLB value of the corresponding surfactant is the HLB value of the crude oil.
In this study, the HLB value of crude oil was determined. Tween 80 and Span 80 were used to form various emulsification systems with different HLB values. Experiments such as emulsion stability and interfacial tension were performed. Through a series of experiments, the relationship between the HLB value of the emulsification system and oil was proposed.
2. Experimental
2.1. Materials
Sodium chloride (NaCl), sodium sulfate (Na2SO 4), sodium bicarbonate (NaHCO3), and calcium chloride (CaCl2) were chemically pure, purchased from Sinopharm Chemical Reagent Co., Ltd (Shanghai, China). Magnesium chloride (MgCl2·6H2O) was chemically pure, purchased from Titan Scientific Co., Ltd. (Shanghai, China). The two kinds of surfactants (Span 80 and Tween 80) were chemically pure, purchased from Sinopharm Chemical Reagent Co., Ltd (Shanghai, China). The molecular structure of these two surfactants was shown in Fig. 1. Deionized water was prepared in the lab. The experimental crude oil was provided by Bohai oilfield (Table 1 and Fig. 2).
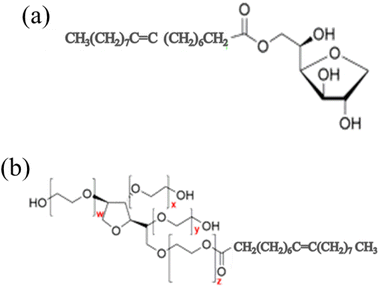 |
| Fig. 1 The molecular structure of Span 80 (a) and Tween 80 (b). | |
Table 1 SARA composition of crude oil
w (saturation)/% |
w (aromatic)/% |
w (colloid)/% |
w (asphaltene)/% |
34.65 |
30.13 |
37.05 |
0.42 |
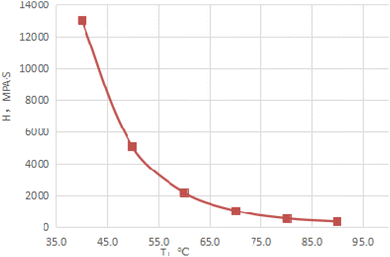 |
| Fig. 2 Crude oil viscosity under different temperature. | |
2.2. HLB value of crude oil
Firstly, a compound system with different HLB value was obtained using Span 80 and Tween 80 (Table 2). Secondly, emulsion was formed using the Span 80 and Tween 80 compound system and crude oil. Thirdly, the stability of the emulsion was observed. The experiment temperature was 50 °C.
Table 2 HLB values of Span 80 and Tween 80 compound system
Span 80 : Tween 80 |
HLB value |
2 : 8 |
12.86 |
3 : 7 |
11.79 |
4 : 6 |
10.72 |
5 : 5 |
9.65 |
6 : 4 |
8.58 |
7 : 3 |
7.51 |
8 : 2 |
6.44 |
9 : 1 |
5.37 |
2.3. Preparation of emulsification system
Firstly, Span 80 (HLB = 4.3) and Tween 80 (HLB = 15) were dissolved in simulated formation water. The formula of simulated formation water was shown in Table 3. Secondly, Span 80 and Tween 80 were mixed in different ratios to obtain emulsification systems with different HLB values. The HLB values of different emulsification systems are shown in Table 4.
Table 3 The formation of simulated formation water
Ionic |
Na+ |
Mg2+ |
Ca2+ |
Cl− |
SO42− |
HCO3− |
Total salinity |
Concentration (mg L−1) |
1394.375 |
6.08 |
120.24 |
1010.325 |
18.0113 |
3706.965 |
6255.996 |
Table 4 Composition and HLB value of emulsification systems
Span 80 : Tween 80 |
Span 80 (wt%) |
Tween 80 (wt%) |
HLB value |
2 : 8 |
0.06 |
0.24 |
12.86 |
4 : 6 |
0.12 |
0.18 |
10.72 |
5 : 5 |
0.18 |
0.12 |
9.65 |
8 : 2 |
0.24 |
0.06 |
6.44 |
9 : 1 |
0.27 |
0.03 |
5.37 |
2.4. Emulsion preparation
The emulsion was prepared by mixing crude oil and emulsification system (1
:
9, g g−1) at 50 °C using an FM-200 homogenizer (Fluko Corp., German) at a rotating speed of 3000 rpm for 15 min.
2.5. Multiple light scattering
The stability of the emulsion was characterized using a TURBISCAN Lab Expert stability analyzer (Formulaction, France) based on the principle of multiple light scattering. The experiment temperature was 50 °C.
2.6. Particular size distribution
The particle size distribution curve of dispersed oil droplets in the emulsion was determined by Rize2006 laser particle size analyzer (Jinan Runzhi Technology Co., Ltd., China) at 50 °C according to the full range Mie light scattering theory. The measuring range was 0.1–1200 μm.
The photomicrograph images of emulsion droplets were photographed using a XSJ-2 optical microscope (Chongqing Optical Instrument Corp., China).
2.7. Zeta potential
The zeta potential of emulsion was measured by Zetasizer Nano (Malvern, UK). The experiment temperature was 50 °C.
2.8. Interfacial tension
Oil–water interfacial tension between various emulsification systems and crude oil was measured at 50 °C based on spinning drop method using a TX-500C interfacial tensiometer (Bowing Industry Corp., USA) with a measuring range of 10−5 to 102 mN m−1.
3. Result and discussion
3.1. HLB value of crude oil
Fig. 3 depicts the morphology image of the dewatering of emulsions with different HLB values. When the HLB value of compound system reached 9.65, the emulsion had best stability after 90 min. At the same time, Fig. 4 shows the dewatering rate of emulsions with the variation in the HLB value of the compound system. It can be clearly seen from Fig. 4 that with the increased in the HLB value of compound system, the dewatering rate first decreased and then increased. When the HLB value of compound system was 9.65, the dewatering rate was the lowest. Based on the experimental method and HLB value of crude oil proposed by He et al.,25 the HLB value of crude oil used in this study was 9.65.
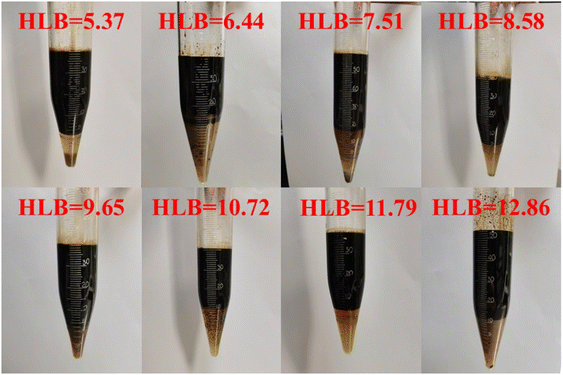 |
| Fig. 3 Morphology images of emulsions at different HLB values after 90 min (T = 50 °C). | |
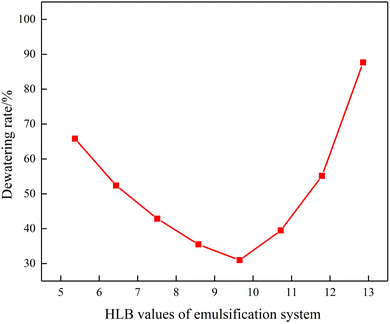 |
| Fig. 4 Dewatering rate of emulsion with the variation of HLV values of compound system after 90 min (T = 50 °C). | |
3.2. Stability comparison of emulsions
Fig. 5 shows the emulsion stability as a function of the HLB values of emulsification systems using a TURBISCAN Lab Expert stability analyzer. The TSI curves of four emulsions with different HLB values of emulsification systems increased with time. As we all know, emulsion is a thermodynamically unstable system.26 This phenomenon means these four kinds of emulsions were demulsified over time. When the experiment was completed, the TSI values of these four emulsions were 5.5, 3.4, 1.7, 2.7, and 2.1, respectively. Compared with other emulsions, the emulsion formed by the emulsification system with HLB = 9.65 was more stable. From the perspective of HLB value, when the HLB value of crude oil and emulsification system is close, the emulsion has better stability. Fig. 6 shows the morphology images of emulsion as a function of the HLB values of emulsification systems. It can be clearly seen that the formed emulsion was a water-in-oil emulsion. At the same time, when the HLB value of the emulsification systems reached 9.65, the emulsion has smaller particle size. Because the emulsion is water-in-oil emulsion and Span 80 in the emulsification system is a lipophilic surfactant, when the proportion of Span 80 in the emulsification system increases, it exhibits good emulsifying effect.
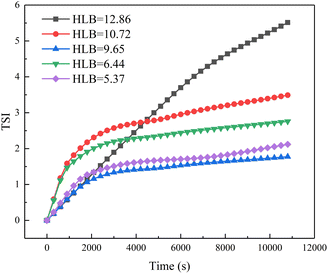 |
| Fig. 5 TSI curves of emulsion under different emulsification system's HLB value (T = 50 °C). | |
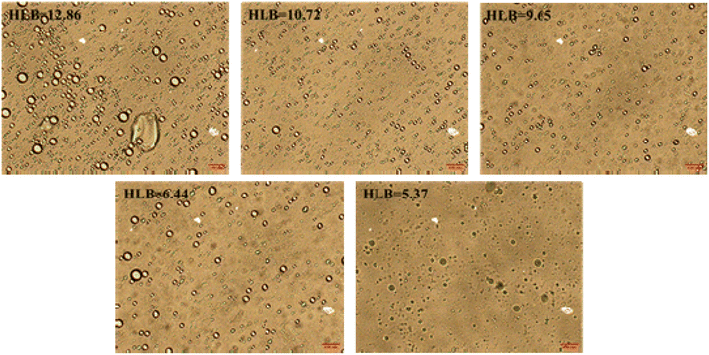 |
| Fig. 6 Emulsion morphology images under different emulsification system's HLB value. | |
3.3. Droplet size distribution of emulsions
The droplet size of emulsions is critical to the emulsion stability.27 Fig. 7 shows the droplet size distributions as a function of the HLB values of the emulsification systems. The average droplet size of HLB = 5.37, HLB = 6.44, HLB = 9.65, HLB = 10.72, and HLB = 12.86 were 475.9 nm, 333.4 nm, 319.7 nm, 659.7 nm, and 1276.1 nm, respectively. Meanwhile, the emulsion formed by emulsification system with HLB = 9.65 has narrow particle size distribution range. The same results as TSI experiment, the average droplet size was smallest when the HLB value of the emulsification system was 9.65. That is, when the HLB value of the emulsification system is close to the HLB value of oil, the average droplet size is smallest and the particle size distribution is narrower. When an appropriate HLB value emulsifier existed, crude oil can form a relatively stable emulsion. Meanwhile, compared with low HLB value emulsification system, the average droplet size of high HLB value emulsification is higher. This phenomenon can be explained as follows. When the HLB value of the emulsification system is high, the proportion of Tween 80 is high. On the oil–water interface, the volume and cross-sectional area of Tween 80 are larger than Span 80, so the emulsion has a lager radius of curvature, which means larger average droplet size. In addition, due to different molecular sizes of Tween 80 and Span 80, the adsorption kinetics on the oil–water interface are different.28 During the homogenization process, Span 80 (smaller molecular weight) has faster adsorption speed than Tween 80 (higher molecular weight).
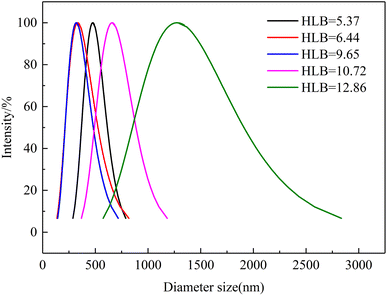 |
| Fig. 7 Droplet size distributions of emulsion as a function of emulsification system's HLB value (T = 50 °C). | |
3.4. Effect of HLB value on emulsion's zeta potential
Zeta potential represents the surface electrical properties of suspended particles, and the value can be used to determine electrical attraction or repulsion between the particle and surface.29,30 Xu et al. proposed zeta potential is one of the main factor affecting the emulsion stability.31 In this part, the zeta potential of emulsion under different emulsification systems was measured.
Fig. 8 shows the zeta potential of emulsion as a function of the HLB values of emulsification systems. The zeta potential of the emulsion was negative irrespective of the HLB value of the emulsification system. The absolute value of emulsion's zeta potential was in the order of HLB = 9.65 > HLB = 12.86 > HLB = 10.72 > HLB = 5.37 > HLB = 6.44. This result indicates when the HLB value of the emulsification system is close to the HLB value of oil, the absolute value of emulsion's zeta potential is highest. Meanwhile, high HLB value emulsification system has high absolute value of emulsion's zeta potential. This phenomenon occurred due to the presence of polyoxymethylene in emulsification system. When polyoxymethylene existence, hydrogen bonding is formed between polyoxymethylene and water and then hydroxide is generated. Therefore, when polyoxymethylene concentration is increased, the absolute value of emulsion's zeta potential is increased. When zeta potential is largest, the emulsion droplets have the best stability. However, when the polyoxymethylene concentration increased to a certain value, the polyoxymethylene get closer to emulsion surface, which produces shielding effect, and the zeta potential value decreases due to crowding effect.
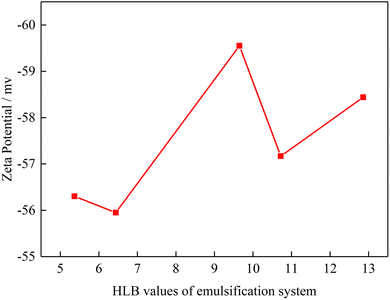 |
| Fig. 8 Zeta potential of emulsion as a function of emulsification system's HLB value (T = 50 °C). | |
3.5. Effect of HLB value on interfacial tension
Interfacial tension plays an important role on the emulsion stability.32,33 In order to explore the effect of the HLB value of the emulsification system on surface tension, interfacial tension experiments were conducted. The experimental results are shown in Fig. 9. With an increase in the HLB value of the emulsification system interfacial tension first decreased and then increased. It reached min value at HLB = 9.65 (3.99 × 10−1 mN m−1). Combined with emulsion stability experiments, the interfacial tension experiment can be explained as follows. When an emulsification system has suitable HLB value, surfactant molecules can arrange in oil–water interface film more reasonably, which reduces the free energy of oil–water interface film and can improve emulsion stability significantly. When the HLB value of the emulsification system increases, the proportion of Tween 80 in the system increases and the lipophilicity of the system is stronger, and hence it has a lower interfacial tension compared with an emulsified system with a low HLB value.
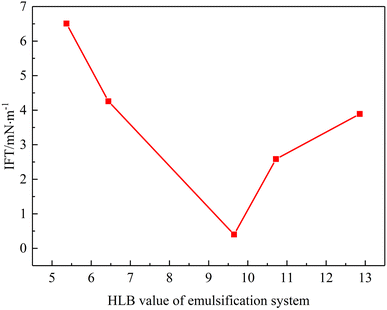 |
| Fig. 9 Interfacial tension of emulsion as a function of emulsification system's HLB value (T = 50 °C). | |
3.6. The mechanism of HLB value on emulsion stability
Based on the above results, the mechanism of HLB value on emulsion stability is illustrated in Fig. 10. When a surfactant interacts with oil, the surfactant molecules gather on the oil–water interface film spontaneously, reducing the interfacial tension and improving emulsion stability. When the HLB value of the emulsification system is close to that of crude oil, surfactant molecules arranged more closely on oil–water interface film, which leads to greater interfacial film strength. At the same time, the electrical repulsion between emulsion droplets is increased. Therefore, emulsion has better stability when the HLB value of the emulsification system is close to that of oil.
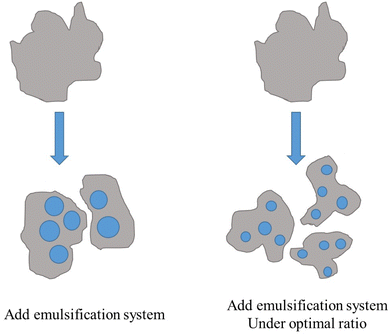 |
| Fig. 10 The mechanism of HLB value on emulsion stability. | |
4. Conclusion
Various emulsification systems were constructed using Tween 80 and Span 80 with different ratios. The HLB value of crude oil was 9.65. The relationship between the HLB value of oil and emulsion stability was proposed. When the HLB value of the emulsification system is close to that of oil, surfactant molecules are arranged more closely on the oil–water interface, leading to smaller sized emulsion droplet. At the same time, electrical repulsion between emulsion droplets is increased, through these aspects, emulsion had better stability.
Conflicts of interest
No conflict of interest exists in the submission of this manuscript, and manuscript is approved by all authors for publication. I would like to declare on behalf of my co-authors that the work described was original research that has not been published previously, and not under consideration for publication elsewhere, in whole or in part. All the authors listed have approved the manuscript that is enclosed.
References
- B. A. Bealessio, N. A. Blánquez Alonso, N. J. Mendes, A. V. Sande and B. Hascakir, A review of enhanced oil recovery (EOR) methods applied in Kazakhstan, Petroleum, 2021, 7, 1–9 CrossRef.
- G. A. R. Ramos, L. T. Akanji and W. Afzal, A Novel Surfactant–Polymer/Alkaline–Surfactant–Polymer Formulation for Enhanced Oil Recovery (EOR) Processes, Energy Fuels, 2020, 34, 1230–1239 CrossRef CAS.
- K. Mogensen and S. Masalmeh, A review of EOR techniques for carbonate reservoirs in challenging geological settings, J. Pet. Sci. Eng., 2020, 195, 107889 CrossRef CAS.
- F. Wang, H. Yang, H. Jiang, X. Kang, X. Hou, T. Wang, B. Zhou, B. Sarsenbekuly and W. Kang, Formation mechanism and location distribution of blockage during polymer flooding, J. Pet. Sci. Eng., 2020, 194, 107503 CrossRef CAS.
- D. Xie, J. Hou, A. Doda and J. Trivedi, Application of Organic Alkali for Heavy-Oil Enhanced Oil Recovery (EOR), in Comparison with Inorganic Alkali, Energy Fuels, 2016, 30, 4583–4595 CrossRef CAS.
- J. Fink, Chapter 16 - Enhanced oil recovery, in Petroleum Engineer's Guide to Oil Field Chemicals and Fluids, ed. J. Fink, Gulf Professional Publishing, 3rd edn, 2021, pp. 643–731 Search PubMed.
- D. Alfarge, M. Wei and B. Bai, Chapter 9 - Other enhanced oil recovery methods for unconventional reservoirs, in Developments in Petroleum Science, ed. D. Alfarge, M. Wei, B. Bai, B. Bai and Z. Chen, Elsevier, 2020, pp. 185–199 Search PubMed.
- W. Yang, J. Lu, B. Wei, H. Yu and T. Liang, Micromodel Studies of Surfactant Flooding for Enhanced Oil Recovery: A Review, ACS Omega, 2021, 6, 6064–6069 CrossRef CAS PubMed.
- T. Ma, H. Feng, H. Wu, Z. Li, J. Jiang, D. Xu, Z. Meng and W. Kang, Property evaluation of synthesized anionic-nonionic gemini surfactants for chemical enhanced oil recovery, Colloids Surf., A, 2019, 581, 123800 CrossRef CAS.
- M. S. Kamal, I. A. Hussein and A. S. Sultan, Review on Surfactant Flooding: Phase Behavior, Retention, IFT, and Field Applications, Energy Fuels, 2017, 31, 7701–7720 CrossRef CAS.
- C.-D. Yuan, W.-F. Pu, X.-C. Wang, L. Sun, Y.-C. Zhang and S. Cheng, Effects of Interfacial Tension, Emulsification, and Surfactant Concentration on Oil Recovery in Surfactant Flooding Process for High Temperature and High Salinity Reservoirs, Energy Fuels, 2015, 29, 6165–6176 CrossRef CAS.
- O. Massarweh and A. S. Abushaikha, The use of surfactants in enhanced oil recovery: A review of recent advances, Energy Rep., 2020, 6, 3150–3178 CrossRef.
- N. Pal, H. Hoteit and A. Mandal, Structural aspects, mechanisms and emerging prospects of Gemini surfactant-based alternative Enhanced Oil Recovery technology: A review, J. Mol. Liq., 2021, 339, 116811 CrossRef CAS.
- Y. Wu, W. Chen, C. Dai, Y. Huang, H. Li, M. Zhao, L. He and B. Jiao, Reducing surfactant adsorption on rock by silica nanoparticles for enhanced oil recovery, J. Pet. Sci. Eng., 2017, 153, 283–287 CrossRef CAS.
- H. Jia, P. Lian, X. Leng, Y. Han, Q. Wang, K. Jia, X. Niu, M. Guo, H. Yan and K. Lv, Mechanism studies on the application of the mixed cationic/anionic surfactant systems to enhance oil recovery, Fuel, 2019, 258, 116156 CrossRef CAS.
- M. Zhang, W. Kang, H. Yang, Z. Li, X. Li, B. Zhou, X. Kang, M. Li and B. Sarsenbekuly, Rheology and microstructure of zwitterionic-anionic surfactant for enhanced oil recovery, J. Mol. Liq., 2021, 116910 CrossRef CAS.
- J. A. Ali, K. Kolo, A. K. Manshad and A.
H. Mohammadi, Recent advances in application of nanotechnology in chemical enhanced oil recovery: Effects of nanoparticles on wettability alteration, interfacial tension reduction, and flooding, Egypt. J. Pet., 2018, 27, 1371–1383 CrossRef.
- H. Wu, Q. Zhou, D. Xu, R. Sun, P. Zhang, B. Bai and W. Kang, SiO2 nanoparticle-assisted low-concentration viscoelastic cationic surfactant fracturing fluid, J. Mol. Liq., 2018, 266, 864–869 CrossRef CAS.
- G. Cheraghian, S. Kiani, N. N. Nassar, S. Alexander and A. R. Barron, Silica Nanoparticle Enhancement in the Efficiency of Surfactant Flooding of Heavy Oil in a Glass Micromodel, Ind. Eng. Chem. Res., 2017, 56, 8528–8534 CrossRef CAS.
- Z. Li, D. Xu, Y. Yuan, H. Wu, J. Hou, W. Kang and B. Bai, Advances of spontaneous emulsification and its important applications in enhanced oil recovery process, Adv. Colloid Interface Sci., 2020, 277, 102119 CrossRef CAS PubMed.
- L. Y. Seng and B. Hascakir, Surfactant Flooding Performance for a Canadian Bitumen Recovery: Effect of Polarity, 2020 Search PubMed.
- Y.-B. Guo, X.-A. Yue, J. Y. Fu and B. Zhang, Relevance between Emulsification Capability and Interfacial Tension of Chemical Flooding Agents, Energy Fuels, 2018, 32, 12345–12350 CrossRef CAS.
- Z. Liu, W. Liu, C. Lang and Y. Li, Effect of surfactant HLB value on Methane hydrate formation in non-ionic surfactant-oil water emulsions systems, Energy Procedia, 2019, 158, 5275–5280 CrossRef CAS.
- T. Schmidts, D. Dobler, A. C. Guldan, N. Paulus and F. Runkel, Multiple W/O/W emulsions—Using the required HLB for emulsifier evaluation, Colloids Surf., A, 2010, 372, 48–54 CrossRef CAS.
- J. He, F. Chen, P. Duan, S. Cong and C. Zhong, Using HLB value method to screening emulsifing viscosity reducer for heavy oil, Spec. Petrochem., 2012, 29, 35–38 CAS.
- H. Zhang, H. Yang, F. Wang, H. Zhao, X. Li, B. Zhou, M. Zhang, W. Kang, B. Sarsenbekuly, S. Aidarova and M. Gabdullin, Study on the stabilization of emulsion formed by Two different inclusion Complexes, Colloids Surf., A, 2020, 594, 124651 CrossRef CAS.
- F. Wang, H. Yang, M. Li, X. Kang, X. Zhang, H. Zhang, H. Zhao, W. Kang, B. Sarsenbekuly, S. Aidarova and M. Gabdullin, Study on stabilization of emulsion formed by the supramolecular system of amphiphilic polymer and sodium polyacrylic acid, J. Mol. Liq., 2020, 314, 113644 CrossRef CAS.
- V. J. Verruto, R. K. Le and P. K. Kilpatrick, Adsorption and Molecular Rearrangement of Amphoteric Species at Oil–Water Interfaces, J. Phys. Chem. B, 2009, 113, 13788–13799 CrossRef CAS PubMed.
- D. Li, Y. Zhao, X. Wang, H. Tang, N. Wu, F. Wu, D. Yu and W. Elfalleh, Effects of (+)-catechin on a rice bran protein oil-in-water emulsion: Droplet size, zeta-potential, emulsifying properties, and rheological behavior, Food Hydrocolloids, 2020, 98, 105306 CrossRef CAS.
- Z. Wu, J. Wu, R. Zhang, S. Yuan, Q. Lu and Y. Yu, Colloid properties of hydrophobic modified alginate: Surface tension, ζ-potential, viscosity and emulsification, Carbohydr. Polym., 2018, 181, 56–62 CrossRef CAS PubMed.
- X. MingJin, L. MingYuan, P. Bo, W. ZhaoLiang, L. MeiQin, G. JiXiang and D. ZhaoXiao, Effects of Strength of Interfacial Film and Zeta potential on Oil-in-water Emulsion Stability, Chin. J. Appl. Chem., 2007, 24, 623–627 Search PubMed.
- M. Bertin, D. Gomes Rodrigues, C. Pierlot, C. Albert-Mercier, C. Davy, D. Lambertin and V. Nardello-Rataj, Influence of cetyltrimethylammonium bromide and hydroxide ions on the interfacial tension and stability of emulsions of dodecane in aqueous silicate solutions, Colloids Surf., A, 2021, 628, 127306 CrossRef CAS.
- L. Yu, Q. Sang, M. Dong and Y. Yuan, Effects of Interfacial Tension and Droplet Size on the Plugging Performance of Oil-in-Water Emulsions in Porous Media, Ind. Eng. Chem. Res., 2017, 56, 9237–9246 CrossRef CAS.
|
This journal is © The Royal Society of Chemistry 2023 |
Click here to see how this site uses Cookies. View our privacy policy here.