DOI:
10.1039/D3RA05440C
(Paper)
RSC Adv., 2023,
13, 27158-27166
Palladium-catalyzed intramolecular aza-Wacker-type cyclization of vinyl cyclopropanecarboxamides to access conformationally restricted aza[3.1.0]bicycles†
Received
11th August 2023
, Accepted 6th September 2023
First published on 11th September 2023
Abstract
A palladium(II)-catalyzed intramolecular oxidative aza-Wacker-type reaction of vinyl cyclopropanecarboxamides to access a series of conformationally restricted highly substituted aza[3.1.0]bicycles is reported. The transformation proceeded through a typical aza-Wacker reaction mechanism to forge a new C–N bond with oxygen as the terminal oxidant. The desired fused heterocycles were obtained in moderate yields. The process is tolerant of a range of functional aryl groups under mild conditions.
Introduction
The rigid 3-azabicyclo[3.1.0]hexane moiety is an essential three-dimensional building block found in alicyclic amine-based fragments of several pharmaceuticals, including the hepatitis C virus drug Victrelis, potent gyrase inhibitor Trovafloxacin, and the pesticide procymidone (Fig. 1).1 This type of small molecule is also useful as an organic synthon for the selective functionalization of a variety of bioactive molecules and is suitable for late-stage modification of drug candidates in medicinal chemistry.2
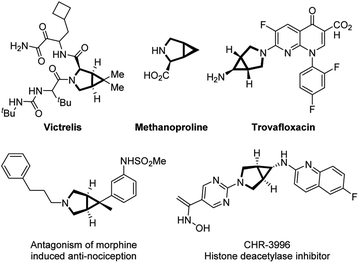 |
| Fig. 1 Examples of aza[3.1.0]bicycles-containing bioactive pharmacophores. | |
The palladium(II)-catalyzed aza-Wacker-type reaction of olefins is an important transformation given that amines, carbazoles, carbamates, and amides are commonly utilized in intermolecular palladium-catalyzed oxidative amination reactions.3 It has found widespread application in the construction of natural products and synthetic bioactive compounds. From a mechanistic aspect, after nucleopalladation of the olefin bond, a key carbon-bonded Pd(II) intermediate is generated, which can partake in subsequent reaction steps. Alkyl substituents with low steric hindrance are usually pre-attached to the terminal position of the alkene group to facilitate subsequent β-hydride elimination, rather than β′-hydride elimination (Scheme 1a).4 In particular, aryl-involved and terminal alkene-involved transformations always involve an amidopalladation intermediate or a π-allylpalladium complex to the alkylpalladium species, resulting in multiple fused products through the formation of C–C bonds via reductive elimination of the palladacycle (Scheme 1b, path a).5 Alternatively, the alkylpalladium species may afford the enamide product via a β′-hydride elimination. In addition, because the elimination occurs at locations with high steric resistance, the yields of such reactions are generally not higher than those of using non-terminal alkenes (Scheme 1b, path b).6 Several examples of transformation via path a in Scheme 1b have been established by different research groups. For example, in 2011, Yang et al.5h obtained indole derivatives 3 via Pd-catalyzed intramolecular amidoarylation of alkenes starting from α-allyl-substituted acetanilides 1. They observed a trace amount of β-hydrogen eliminated enamide by-product by 1H NMR spectroscopy (Scheme 1c). Recently, Shi's group6b–d developed a series of works on Pd(II)-catalyzed intermolecular cascade methylene C(sp3)–H alkenylation/aza-Wacker cyclization to access α/β-stereospecific γ-lactams via a π-allyl-Pd intermediate. The presence of an aryl group forces the hydrogen elimination reaction to occur regiospecifically at the β′-position (Scheme 1d).
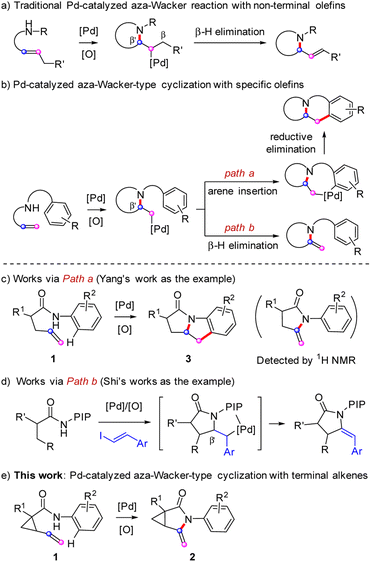 |
| Scheme 1 Reaction of allylic compounds in intramolecular aza-Wacker-type reaction. | |
Although remarkable process has been made in intramolecular aza-Wacker-type reactions in recent decades, the construction of structurally diverse heterocyclic compounds, including fused and bridged ring compounds, using this strategy remains challenging.7 To the best of our knowledge, the synthesis of conformationally restricted aza[3.1.0]bicycles from vinyl cyclopropanecarboxamides via intramolecular aza-Wacker oxidation or oxidative amination is not reported in the literature. Herein, we describe our recent efforts toward the Pd-catalyzed ligand-free intramolecular oxidative amidation of vinyl cyclopropanecarboxamides 1 to access conformationally restricted aza[3.1.0]bicycle core 2 via aza-Wacker-type reaction (Scheme 1e).
Results and discussion
Combined with our studies on derivatization reactions of amides and previous reports,8 we envisioned that fused-heterocycles 3 may be accessed by treating vinyl cyclopropanecarboxamides 1 with Pd salts to give the important cyclopropa[3,4]pyrrolo[1,2-a]indolone core 2 of natural products and prodrugs via the aza-Wacker-type oxidation amidation reaction.9 With this assumption in hand, we attempted all reaction conditions described by previous reports with model reagent 1a,10 but we found that all efforts failed to give the fused heterocycles 3, indicating that these conditions are not fit for this type of vinyl cyclopropanecarboxamide to undergo the formal [3 + 2] cyclization reaction. Fortunately, the crystal structure of the aza[3.1.0]bicycle 2a, was unambiguously confirmed by X-ray diffraction (CCDC no. 2142480, Fig. 2). This result suggests that the transformation proceeded via cascade amidopalladation/β-hydride elimination, rather than through the amidoarylation pathway. Subsequent optimization indicated that 5 mol% of Pd(PPh3)2Cl2 can promote complete consumption of the substrate in 24 h, affording 2a in 60% yield in the presence of 1.0 equiv. of K2CO3 in dimethylformamide (DMF) in a sealed tube at 50 °C using molecular oxygen as the terminal oxidant (Table 1, entry 1). Lowering the loading of the Pd(PPh3)2Cl2 catalyst to 3 mol% still furnished a 58% yield of 2a, but the reaction time was prolonged to 44 h (Table 1, entry 2). In contrast, increasing the catalyst loading to 10 mol% resulted in a yield of only 59% (Table 1, entry 3). Further efforts to increase the yield of 2a were unsuccessful, including performance of the reaction with Pd(OAc)2, Pd(TFA)2, PdCl2, PdCl2(MeCN)2, PdCl2(dppe), and Pd(dba)2 as alternative catalysts. All of these reactions gave yields of 2a that were less than that observed with Pd(PPh3)2Cl2 catalyst (Table 1, entries 4–9). Oxidant was shown to be necessary for the reaction, and a high concentration of oxygen clearly favored the rapid turn-over of the metal catalyst (Table 1, entries 10 and 11). When the reactions were carried out at slightly higher or lower temperatures, the yields were not improved (Table 1, entries 12 and 13). In addition, lowering or increasing the amount of K2CO3 also failed to improve the yield of 2a (Table 1, entries 14 and 15). Other bases including AcOK, K3PO4, and Cs2CO3 were proven less efficient than K2CO3 (Table 1, entries 16–18). Solvent screening indicated that DMF was the most appropriate solvent. Use of N-methyl-2-pyrrolidone (NMP), acetonitrile, dioxane, toluene, dimethyl sulfoxide (DMSO), or tetrahydrofuran (THF) as solvent provided diminished yields or trace amounts of the product (Table 1, entries 19–24). The conversion performed in ethanol gave a poor yield of product 2a.
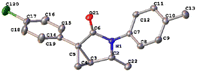 |
| Fig. 2 ORTEP drawing of 2a. | |
Table 1 Optimization of reaction conditionsa
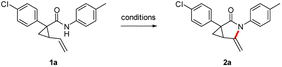
|
Entry |
[Pd]/mol% |
Solvent |
Time/h |
Yield/%b |
Unless otherwise indicated, the reaction was conducted with 1a (0.5 mmol, 1.0 equiv.), [Pd] salt (5 mol%), K2CO3 (1.0 equiv.) and solvent (2 mL) at 50 °C under O2 in a sealed tube. Recovered 1a shown in parentheses after purification by column chromatography. N2. Air. 40 °C. 60 °C. K2CO3 (0.5 equiv.). K2CO3 (1.5 equiv.). AcOK. K3PO4. Cs2CO3. |
1 |
Pd(PPh3)2Cl2 (5) |
DMF |
24 |
60 |
2 |
Pd(PPh3)2Cl2 (3) |
DMF |
44 |
58 |
3 |
Pd(PPh3)2Cl2 (10) |
DMF |
24 |
59 |
4 |
Pd(OAc)2 (5) |
DMF |
8 |
46 |
5 |
Pd(TFA)2 (5) |
DMF |
24 |
21 (31) |
6 |
PdCl2 (5) |
DMF |
24 |
19 (42) |
7 |
PdCl2(MeCN)2 (5) |
DMF |
24 |
25 (27) |
8 |
PdCl2(dppe) (5) |
DMF |
24 |
44 |
9 |
Pd(dba)2 (5) |
DMF |
24 |
13 (45) |
10 |
Pd(PPh3)2Cl2 (5) |
DMF |
24 |
Tracec |
11 |
Pd(PPh3)2Cl2 (5) |
DMF |
24 |
Traced |
12 |
Pd(PPh3)2Cl2 (5) |
DMF |
33 |
58 (10)e |
13 |
Pd(PPh3)2Cl2 (5) |
DMF |
17 |
53f |
14 |
Pd(PPh3)2Cl2 (5) |
DMF |
24 |
39 (30)g |
15 |
Pd(PPh3)2Cl2 (5) |
DMF |
24 |
60h |
16 |
Pd(PPh3)2Cl2 (5) |
DMF |
24 |
26i |
17 |
Pd(PPh3)2Cl2 (5) |
DMF |
24 |
45 (17)j |
18 |
Pd(PPh3)2Cl2 (5) |
DMF |
24 |
49 (18)k |
19 |
Pd(PPh3)2Cl2 (5) |
NMP |
24 |
45 (13) |
20 |
Pd(PPh3)2Cl2 (5) |
MeCN |
24 |
24 (43) |
21 |
Pd(PPh3)2Cl2 (5) |
Dioxane |
24 |
Trace (90) |
22 |
Pd(PPh3)2Cl2 (5) |
Toluene |
24 |
Trace (88) |
23 |
Pd(PPh3)2Cl2 (5) |
DMSO |
24 |
37 (39) |
24 |
Pd(PPh3)2Cl2 (5) |
THF |
24 |
Trace (94) |
25 |
Pd(PPh3)2Cl2 (5) |
EtOH |
24 |
29 (41) |
Related researches have already indicated the importance of pyridine and amine ligands for this aza-Wacker-type reaction.5h,10d,10e,11 Accordingly, some representative ligands L1–L14 (Scheme 2) were employed to the reaction, with 40 mol% loading under the optimal reaction conditions (Table 1, entry 1). Unfortunately, all attempts to improve the yield were unsuccessful, ligands inhibit the process, with yields of 2a not exceeding 31%, along with most of starting material was recovered after 24 h. Especially, the yield of 2a given by L4 and L6 was not higher than 31% even at 10% ligand dosage.
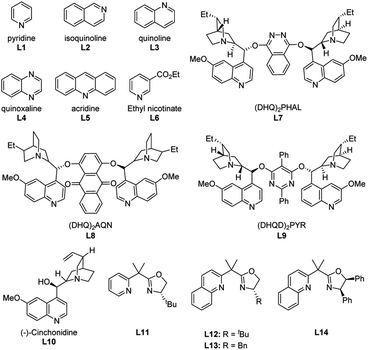 |
| Scheme 2 Structures of generally used ligands. | |
With the optimized reaction conditions in hand (Table 1, entry 1), the substrate scope and limitation of this palladium-catalyzed intramolecular aza-Wacker-type oxidation amidation of vinyl cyclopropanecarboxamides were studied. As shown in Scheme 3, various aryl R2 groups were examined, demonstrating that the reaction could tolerate aryl groups bearing an electron-donor group (EDG) (2a–e: Me, tBu, and OMe) at the para-, meta-, and ortho-position or electron-withdrawing group (EWG) at the para-position (2g: CF3 and 2h: CO2Et), as well as the unsubstituted phenyl group (2f). Notably, given that the substrate featuring an ortho-substituted methoxy group gave a relatively low yield of desired product 2e, further use of substrates with EDG at the ortho-position was not attempted. To our delight, product 2i bearing a naphthyl group on the nitrogen atom of the amide moiety was isolated in 74% yield after 5 h. However, the transformation from 1j with an aminoquinoline (AQ) group failed to give compound 2j, with 91% of the starting material recovered. We presumed that this lack of reaction was caused by poisoning of the Pd catalyst by coordination with the bidentate AQ ligand.12 However, given that steric hindrance might also be partially responsible for the low yield of the aforementioned products, we tested the reaction with the N–OMe substituted substrate 1k. Product 2k was isolated in 47% yield, indicating that steric hindrance may not be the cause of low yields. The reactions with benzyl- and nBu-substituted substrates 1l and 1m afforded products 2l and 2m, respectively, in 20% and 21% yields. Unfortunately, our efforts to expand the protocol with substrate featuring a cyclohexyl group (1n) met with failure even at high temperatures and with long reaction times, whereas the tosylate-substituted starting material (1o) gave a trace amount of desired product 2o.
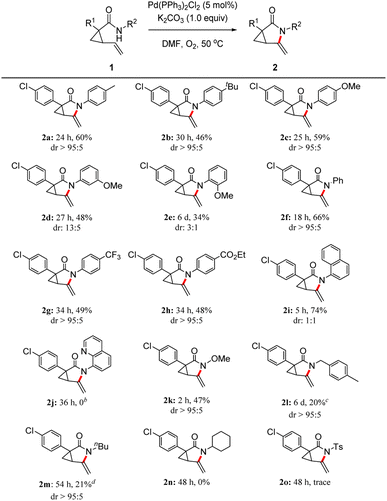 |
| Scheme 3 Extension of reaction scope with various R2 groups.a a Unless otherwise indicated, all reactions were conducted with 1 (0.5 mmol, 1.0 equiv.), Pd(PPh3)2Cl2 (5 mol%), K2CO3 (1.0 equiv.) and DMF (2 mL) at 50 °C under O2 in a sealed tube, and the dr values were determined from the 1H NMR analysis of the crude reaction mixture. b 91% of 1j was recovered. c 58% of 1l was recovered. d 71% of 1m was recovered. | |
The reaction scope was further explored with a series of substrates with varied R1 substituents see Scheme 4. The transformation was favored for various 1-aryl substituted vinyl cyclopropanecarboxamides bearing a OMe (2p and 2q), Me (2r), F (2t), Cl (2u and 2v), or Br (2w) group at the para-, meta- or ortho-position, along with the phenyl-substituted cyclopropane derivative (2s). The target compounds 2p–w were isolated in 48–57% yields. Notably, the bromobenzene moiety of product 2w remained intact as a site for further functionalization by methods including the Suzuki–Miyaura,13 Buchwald–Hartwig,14 and the Sonogashi coupling reactions.15 In addition, the starting material 1x with an EWG at the para-position readily provided the product 2x in 58% yield. Unfortunately, the reaction of 1y furnished the desired product 2y only in 16% yield. A novel alkene bisamidation product 2z with a benzene ring as the linker was also obtained in 24% yield. We also noted that all reactions were accompanied by mixtures of unidentified byproducts, which is the reason for the low yield of product 3. The reaction using terminal substituted alkene 1aa bearing a phenyl group did not reach completion after 60 h, affording 2aa in 25% yield along with 63% of recovered starting material 1aa. Notably, allyl-substituted chain substrate 1ab underwent this aza-Wacker-type cyclization reaction before oxidation and hydroxylation tandem processes formed compound 2ab, which features a hydroxyl group that is likely to be derived from symbiotic water of the reaction.
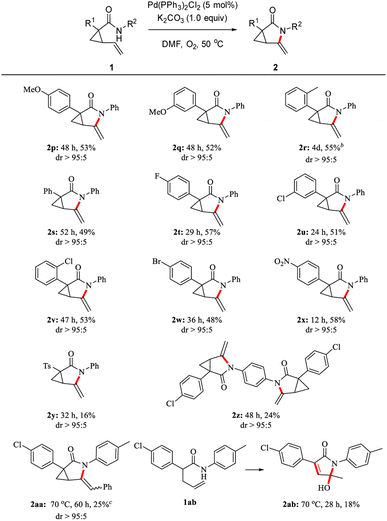 |
| Scheme 4 Extension of reaction scope with various R1 groups.a a Unless otherwise indicated, all reactions were conducted with 1 (0.5 mmol, 1.0 equiv.), Pd(PPh3)2Cl2 (5 mol%), K2CO3 (1.0 equiv.) and DMF (2 mL) at 50 °C under O2 in a sealed tube, and the dr values were determined from the 1H NMR analysis of the crude reaction mixture. b 21% of 1r was recovered. c 63% of 1aa was recovered. | |
To our delight, we found that the reaction can be readily scaled up: 5 mmol of 1-(4-chlorophenyl)-N-(p-tolyl)-2-vinylcyclopropane-1-carboxamide (1a) was treated with 5 mol% Pd(PPh3)2Cl2, 1.0 equiv. of K2CO3, and O2 in DMF to smoothly furnish a 47% yield of 2a after 46 h (Scheme 5).
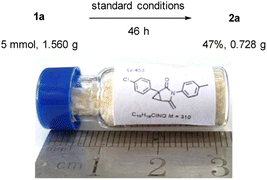 |
| Scheme 5 Gram-scale preparation of compound 2a. | |
Experimental
General information
Unless stated otherwise, reactions were conducted in flame-dried Schlenk under a positive pressure of O2. All reagents were purchased from commercial sources and used without further treatment and all substrates are homogeneous, unless otherwise indicated. Starting materials 1 were synthesized following the literature.16 DMF, MeCN, and toluene solvents were distilled under an atmosphere of dry N2. Petroleum ether (PE) used here refers to the 60–90 °C boiling point fraction of petroleum. Ethyl acetate is abbreviated as EA. 1H NMR and 13C NMR spectra were recorded on a Bruker Avance/600 (1H: 600 MHz, 13C: 150 MHz at 25 °C) or Bruker Avance/400 (1H: 400 MHz, 13C: 100 MHz at 25 °C). Fluorine nuclear magnetic resonance (19F NMR) spectra were recorded on a Bruker Avance/600 or Bruker Avance/400. NMR data are represented as follows: chemical shift, integration, multiplicity (br = broad, s = singlet, d = doublet, dd = double doublet, t = triplet, q = quartet, and m = multiplet), and coupling constants in Hertz (Hz). All high-resolution mass spectra (HRMS) were measured on a mass spectrometer by using electrospray ionization orthogonal acceleration time-of-flight (ESI-OA-TOF), and the purity of all samples used for HRMS (>95%) was confirmed by 1H NMR and 13C NMR spectroscopic analysis. Melting points were measured on a melting point apparatus equipped with a thermometer and were uncorrected. All reactions were monitored by thin-layer chromatography (TLC) with GF254 silica gel-coated plates. Flash chromatography was carried out on SiO2 (silica gel 200–300 mesh). The single crystal of 2a suitable for X-ray analysis was obtained by slowly volatilizing a mixed solution of PE and dichloromethane of 2a in a semi-sealed penicillin glass vial bottle (7 mL) at room temperature (10 mg of 2a was dissolved with 5 drops of dichloromethane before ca. 2 mL of PE was added).
General procedures for 2 (2a as an example)
In a flame-dried 10 mL Schlenk tube was added 1a (156 mg, 0.5 mmol), Pd(PPh3)2Cl2 (17 mg, 5 mol%), K2CO3 (69 mg, 1.0 equiv.), the mixture was stirred well in DMF (2 mL) and stir at 50 °C in sand bath under O2 (the whole process was closely monitored by TLC). After the completion of the reaction, the mixture was added saturated aqueous NaCl and extracted with DCM (10 mL × 3). Then the organic solvent was dried over anhydrous Na2SO4 and concentrated in vacuo. The residue was purified by flash column chromatography with PE/EA (50
:
1) as eluent to give 2a as a white solid (93 mg, 60%).
Conclusions
In summary, we developed a palladium-catalyzed intramolecular aza-Wacker-type oxidation amidation reaction starting from readily available vinyl cyclopropanecarboxamides. The transformation proceeded smoothly under mild conditions to provide a series of highly substituted aza[3.1.0]bicycles in moderate yields (27 examples). These compounds, which were conformationally restricted by their structural elements, have high potential for biological activity. The process is tolerant of a range of functional aryl groups. As the key intermediate, an alkylpalladium species, derived from an amidopalladation underwent β-hydride elimination to access the desired bicyclic 3-azabicyclo[3.1.0]hexan-2-ones. Notably, the enamide and cyclopropane moieties are valuable units for the subsequent construction of structurally diverse biologically valuable organic molecules. In addition, the interesting alkene bisamidation product 2z was synthesized for the first time. Although the yield of this product was not high in this case, this compound may prove valuable in the fields of chemical biology and medicinal chemistry. Currently, our laboratory is performing further studies aimed at improving reaction yields and exploring the reactivity of aza[3.1.0]bicyclic compounds.
Conflicts of interest
There are no conflicts to declare.
Acknowledgements
We thank the NSFC (21772032, 21877206, and 22101074), the 111 Project (D17007), Excellent Youth Foundation of Henan Scientific Committee (222300420012), China Postdoctoral Science Foundation (2019M660173), the Natural Science Foundation of Henan Province (202300410233), and Henan Key Laboratory of Organic Functional Molecules and Drug Innovation for financial support.
Notes and references
-
(a) K. Devarahosahalli Veeranna, K. Kanti Das and S. Baskaran, Reversal of polarity by catalytic SET oxidation: synthesis of azabicyclo[m.n.0]alkanes via chemoselective reduction of amidines, Org. Biomol. Chem., 2021, 19, 4054–4059 RSC;
(b) J. Qiao, Y.-S. Li, R. Zeng, F.-L. Liu, R.-H. Luo, C. Huang, Y.-F. Wang, J. Zhang, B. Quan, C. Shen, X. Mao, X. Liu, W. Sun, W. Yang, X. Ni, K. Wang, L. Xu, Z.-L. Duan, Q.-C. Zou, H.-L. Zhang, W. Qu, Y.-H.-P. Long, M.-H. Li, R.-C. Yang, X. Liu, J. You, Y. Zhou, R. Yao, W.-P. Li, J.-M. Liu, P. Chen, Y. Liu, G.-F. Lin, X. Yang, J. Zou, L. Li, Y. Hu, G.-W. Lu, W.-M. Li, Y.-Q. Wei, Y.-T. Zheng, J. Lei and S. Yang, SARS-CoV-2 Mpro inhibitors with antiviral activity in a transgenic mouse model, Science, 2021, 371, 1374–1378 CrossRef CAS PubMed;
(c) S. Ghorai and D. Lee, Strain-Induced Transformations of Bicyclo[3.1.0]hex-1-enes, Synthesis, 2021, 54, 111–123 Search PubMed;
(d) K. Futatsugi, A. C. Smith, M. Tu, B. Raymer, K. Ahn, S. B. Coffey, M. S. Dowling, D. P. Fernando, J. A. Gutierrez, K. Huard, J. Jasti, A. S. Kalgutkar, J. D. Knafels, J. Pandit, K. D. Parris, S. Perez, J. A. Pfefferkorn, D. A. Price, T. Ryder, A. Shavnya, I. A. Stock, A. S. Tsai, G. J. Tesz, B. A. Thuma, Y. Weng, H. M. Wisniewska, G. Xing, J. Zhou and T. V. Magee, Discovery of PF-06835919: A Potent Inhibitor of Ketohexokinase (KHK) for the Treatment of Metabolic Disorders Driven by the Overconsumption of Fructose, J. Med. Chem., 2020, 63, 13546–13560 CrossRef CAS PubMed;
(e) P. R. Leger, D. X. Hu, B. Biannic, M. Bui, X. Han, E. Karbarz, J. Maung, A. Okano, M. Osipov, G. M. Shibuya, K. Young, C. Higgs, B. Abraham, D. Bradford, C. Cho, C. Colas, S. Jacobson, Y. M. Ohol, D. Pookot, P. Rana, J. Sanchez, N. Shah, M. Sun, S. Wong, D. G. Brockstedt, P. D. Kassner, J. B. Schwarz and D. J. Wustrow, Discovery of Potent, Selective, and Orally Bioavailable Inhibitors of USP7 with In Vivo Antitumor Activity, J. Med. Chem., 2020, 63, 5398–5420 CrossRef CAS PubMed;
(f) L. D. Andrews, T. R. Kane, P. Dozzo, C. M. Haglund, D. J. Hilderbrandt, M. S. Linsell, T. Machajewski, G. McEnroe, A. W. Serio, K. B. Wlasichuk, D. B. Neau, S. Pakhomova, G. L. Waldrop, M. Sharp, J. Pogliano, R. T. Cirz and F. Cohen, Optimization and Mechanistic Characterization of Pyridopyrimidine Inhibitors of Bacterial Biotin Carboxylase, J. Med. Chem., 2019, 62, 7489–7505 CrossRef CAS PubMed;
(g) Z. Chen, W. Mori, H. Fu, M. A. Schafroth, A. Hatori, T. Shao, G. Zhang, R. S. Van, Y. Zhang, K. Hu, M. Fujinaga, L. Wang, V. Belov, D. Ogasawara, P. Giffenig, X. Deng, J. Rong, Q. Yu, X. Zhang, M. I. Papisov, Y. Shao, T. L. Collier, J.-A. Ma, B. F. Cravatt, L. Josephson, M.-R. Zhang and S. H. Liang, Design, Synthesis, and Evaluation of 18F-Labeled Monoacylglycerol Lipase Inhibitors as Novel Positron Emission Tomography Probes, J. Med. Chem., 2019, 62, 8866–8872 CrossRef CAS PubMed.
-
(a) P. Su, H. Li, W. Chen, G. Luo, G. Yang and Z. Chai, Lewis Acid Catalyzed [3+2] Annulations of γ-Butyrolactam-Fused Donor-Acceptor Cyclopropanes with Aromatic Aldehydes and Aldimines, Eur. J. Org Chem., 2020, 2020, 5380–5387 CrossRef CAS;
(b) B. M. Chaki, K. Takenaka, L. Zhu, T. Tsujihara, S. Takizawa and H. Sasai, Enantioselective One-pot Synthesis of 3-Azabicyclo[3.1.0]hexanes via Allylic Substitution and Oxidative Cyclization, Adv. Synth. Catal., 2020, 362, 1537–1547 CrossRef CAS;
(c) M. Lee, A. Adams, P. B. Cox and M. S. Sanford, Access to 3D Alicyclic Amine-Containing Fragments through Transannular C-H Arylation, Synlett, 2019, 30, 417–422 CrossRef CAS PubMed;
(d) M. R. Harris, Q. Li, Y. Lian, J. Xiao and A. T. Londregan, Construction of 1-Heteroaryl-3-azabicyclo[3.1.0]hexanes by sp3-sp2 Suzuki-Miyaura and Chan-Evans-Lam Coupling Reactions of Tertiary Trifluoroborates, Org. Lett., 2017, 19, 2450–2453 CrossRef CAS PubMed;
(e) J. J. Topczewski, P. J. Cabrera, N. I. Saper and M. S. Sanford, Palladium-catalysed transannular C-H functionalization of alicyclic amines, Nature, 2016, 531, 220–224 CrossRef CAS PubMed;
(f) V. A. Zapol'skii, J. C. Namyslo, A. de Meijere and D. E. Kaufmann, Chemistry of polyhalogenated nitrobutadienes, 10: Synthesis of highly functionalized heterocycles with a rigid 6-amino-3-azabicyclo[3.1.0]hexane moiety, Beilstein J. Org. Chem., 2012, 8, 621–628 CrossRef PubMed;
(g) Z. Ren, W. Cao, Y. Lu, Y. Wang and S. Wang, Ring-expansion reaction of cyclopropane: A novel process for synthesis of bicyclic dicarboximides from cyclopropanedicarboximides and carbon nucleophile, Synth. Commun., 2008, 38, 2215–2226 CrossRef CAS;
(h) D. J. Adams, A. J. Blake, P. A. Cooke, C. D. Gill and N. S. Simpkins, Highly enantioselective synthesis of chiral imides and derived products via chiral base desymmetrization, Tetrahedron, 2002, 58, 4603–4615 CrossRef CAS.
-
(a) D. Yang, J. Chen, Y. Huang, H. Pan, J. Shi, Y. Zhang, F. Wang and Z. Li, Room-temperature Formal Aza-Wacker Cyclization through Synergistic Copper/TEMPO-catalyzed Radical Relay, ACS Catal., 2021, 11, 9860–9868 CrossRef CAS;
(b) J. Muzart, Progress in the synthesis of aldehydes from Pd-catalyzed Wacker-type reactions of terminal olefins, Tetrahedron, 2021, 87, 132024 CrossRef CAS;
(c) X. Wang, Q. Wang, Y. Xue, K. Sun, L. Wu and B. Zhang, An organoselenium-catalyzed N1- and N2-selective aza-Wacker reaction of alkenes with benzotriazoles, Chem. Commun., 2020, 56, 4436–4439 RSC;
(d) H. Li, L. Liao and X. Zhao, Organoselenium-Catalyzed Aza-Wacker Reactions: Efficient Access to Isoquinolinium Imides and an Isoquinoline N-Oxide, Synlett, 2019, 30, 1688–1692 CrossRef CAS;
(e) A. Sen, K. Takenaka and H. Sasai, Enantioselective Aza-Wacker-Type Cyclization Promoted by Pd-SPRIX Catalyst, Org. Lett., 2018, 20, 6827–6831 CrossRef CAS PubMed;
(f) X. Kou, Q. Shao, C. Ye, G. Yang and W. Zhang, Asymmetric Aza-Wacker-Type Cyclization of N-Ts Hydrazine-Tethered Tetrasubstituted Olefins: Synthesis of Pyrazolines Bearing One Quaternary or Two Vicinal Stereocenters, J. Am. Chem. Soc., 2018, 140, 7587–7597 CrossRef CAS PubMed;
(g) X. Bao, Q. Wang and J. Zhu, Palladium-Catalyzed Enantioselective Desymmetrizing Aza-Wacker Reaction: Development and Application to the Total Synthesis of (-)-Mesembrane and (+)-Crinane, Angew. Chem., Int. Ed., 2018, 57, 1995–1999 CrossRef CAS PubMed;
(h) G. Liu and S. S. Stahl, Two-faced reactivity of alkenes: cis- versus trans-aminopalladation in aerobic pd-catalyzed intramolecular Aza-Wacker reactions, J. Am. Chem. Soc., 2007, 129, 6328–6335 CrossRef CAS PubMed.
-
(a) L. Shi, M. Wen and F. Li, Palladium-Catalyzed Tandem Carbonylative Aza-Wacker-Type Cyclization of Nucleophile Tethered Alkene to Access Fused N-Heterocycles, Chin. J. Chem., 2021, 39, 317–322 CrossRef CAS;
(b) X. Peng and P. Feng, Continuous-Flow Electrochemistry Promoted Aza-Wacker Cyclizations, Chinese J. Org. Chem., 2021, 41, 2918–2919 CrossRef CAS;
(c) C. Huang, Z.-Y. Li, J. Song and H.-C. Xu, Catalyst- and Reagent-Free Formal Aza-Wacker Cyclizations Enabled by Continuous-Flow Electrochemistry, Angew. Chem., Int. Ed., 2021, 60, 11237–11241 CrossRef CAS PubMed;
(d) A. A. Thomas, S. Nagamalla and S. Sathyamoorthi, Salient features of theaza-Wacker cyclization reaction, Chem. Sci., 2020, 11, 8073–8088 RSC;
(e) R.-S. Tang, L.-Y. Chen, C.-H. Lai and T.-H. Chuang, Palladium-Catalyzed Stereoselective Aza-Wacker-Heck Cyclization: One-Pot Stepwise Strategy toward Tetracyclic Fused Heterocycles, Org. Lett., 2020, 22, 9337–9341 CrossRef CAS PubMed;
(f) C. Ye, X. Kou, J. Xia, G. Yang, L. Kong, Q. Wei and W. Zhang, Pd-II-Catalyzed Oxidative Tandem aza-Wacker/Heck Cyclization for the Construction of Fused 5,6-Bicyclic N,O-Heterocycles, Chem.–Asian J., 2018, 13, 1897–1901 CrossRef CAS PubMed;
(g) P. Kocovsky and J. E. Backvall, The syn/anti-dichotomy in the palladium-catalyzed addition of nucleophiles to alkenes, Chemistry, 2015, 21, 36–56 CrossRef CAS PubMed;
(h) L. Kürti and B. Czako, Strategic Applications of Named Reactions in Organic Synthesis, Elsevier Academic Press, 2005 Search PubMed.
-
(a) Y. Zhou, H. Chen, P. Lei, C. Gui, H. Wang, Q. Yan, W. Wang and F. Chen, Palladium-catalyzed base- and solvent-controlled chemoselective allylation of amino acids with allylic carbonates, Chin. Chem. Lett., 2022, 33, 4850–4855 CrossRef CAS;
(b) S. Nagamalla, J. T. Mague and S. Sathyamoorthi, Progress towards the syntheses of Bactobolin A and C4-epi-Bactobolin A using a sulfamate-tethered aza-Wacker cyclization strategy, Tetrahedron, 2022, 128, 133112 CrossRef CAS;
(c) C.-H. Lu, S. Darvishi, V. Khakyzadeh and C. Li, Pd-Catalyzed enantioselective synthesis of 2-methyl-3-methyleneindoline, Chin. Chem. Lett., 2021, 32, 405–407 CrossRef CAS;
(d) A. H. Shinde, A. A. Thomas, J. T. Mague and S. Sathyamoorthi, Highly Regio- and Diastereoselective Tethered Aza-Wacker Cyclization of Alkenyl Phosphoramidates, J. Org. Chem., 2021, 86, 14732–14758 CrossRef CAS PubMed;
(e) S. Nagamalla, D. K. Johnson and S. Sathyamoorthi, Sulfamate-tethered aza-Wacker approach towards analogs of Bactobolin A, Med. Chem. Res., 2021, 30, 1348–1357 CrossRef CAS;
(f) M. Sun, J. Li, W. Chen, H. Wu, J. Yang and Z. Wang, Palladium-Catalyzed 4 + 2 Annulation of Aryl and Alkenyl Carboxamides with 1,3-Dienes via C-H Functionalization: Synthesis of 3,4-Dihydroisoquinolones and 5,6-Dihydropyridinones, Synthesis, 2020, 52, 1253–1265 CrossRef CAS;
(g) D. N. Garad and S. B. Mhaske, Pd(II)-Catalyzed Intramolecular Tandem Olefin Amidation/C-H Activation Protocol for the Syntheses of the Protoberberine Class of Natural Products, Org. Lett., 2016, 18, 3862–3865 CrossRef CAS PubMed;
(h) K.-T. Yip and D. Yang, Pd(II)-Catalyzed Intramolecular Amidoarylation of Alkenes with Molecular Oxygen as Sole Oxidant, Org. Lett., 2011, 13, 2134–2137 CrossRef CAS PubMed.
-
(a) R. A. Fernandes and A. J. Gangani, Palladium-Catalyzed Oxidant Dependent Switchable Aza-Wacker Cyclization and Oxidative Dimerization of Benzimidates, Org. Lett., 2022, 24, 7400–7404 CrossRef CAS PubMed;
(b) L.-S. Wu, Y. Ding, Y.-Q. Han and B.-F. Shi, Asymmetric Synthesis of gamma-Lactams Containing alpha,beta-Contiguous Stereocenters via Pd(II)-Catalyzed Cascade Methylene C(sp3)-H Alkenylation/Aza-Wacker Cyclization, Org. Lett., 2021, 23, 2048–2051 CrossRef CAS PubMed;
(c) Y. Ding, Y.-Q. Han, L.-S. Wu, T. Zhou, Q.-J. Yao, Y.-L. Feng, Y. Li, K.-X. Kong and B.-F. Shi, Pd(II)-Catalyzed Tandem Enantioselective Methylene C(sp3)-H Alkenylation-Aza-Wacker Cyclization to Access beta-Stereogenic gamma-Lactams, Angew. Chem., Int. Ed., 2020, 59, 14060–14064 CrossRef CAS PubMed;
(d) R. Chang, Y. Chen, W. Yang, Z. Zhang, Z. Guo and Y. Li, Unveiling the Mechanism, Origin of Stereoselectivity, and Ligand-Dependent Reactivity in the Pd(II)-Catalyzed Unbiased Methylene C(sp3)-H Alkenylation-Aza-Wacker Cyclization Reaction, J. Org. Chem., 2020, 85, 13191–13203 CrossRef CAS PubMed;
(e) Z. Zhang, J. Tan and Z. Wang, A facile synthesis of 2-methylquinolines via Pd-catalyzed aza-Wacker oxidative cyclization, Org. Lett., 2008, 10, 173–175 CrossRef CAS PubMed;
(f) S. W. Youn and S. R. Lee, Unusual 1,2-aryl migration in Pd(II)-catalyzed aza-Wacker-type cyclization of 2-alkenylanilines, Org. Biomol. Chem., 2015, 13, 4652–4656 RSC.
-
(a) Q. Li, X. Fang, R. Pan, H. Yao and A. Lin, Palladium-Catalyzed Asymmetric Sequential Hydroamination of 1,3-Enynes: Enantioselective Syntheses of Chiral Imidazolidinones, J. Am. Chem. Soc., 2022, 144, 11364–11376 CrossRef CAS PubMed;
(b) Q. Zhang and G. Zhang, Palladium-Catalyzed Asymmetric Sequential Hydroamination of 1,3-Enynes, Chinese J. Org. Chem., 2022, 42, 3004–3006 CrossRef;
(c) X. Wang, M. Chen, W. Zhang, Y. Zhang, Z. Ren and Z. Guan, Palladium-Catalyzed 5-exo-trig Hydroamidation of β,γ-Unsaturated Hydrazones for Synthesis of Dihydropyrazoles, Chinese J. Org. Chem., 2020, 40, 1618–1624 CrossRef CAS.
-
(a) Z. Zhang, X. Cao, X. Song, G. Wang, B. Shi, X. Li, N. Ma, L. Liu and G. Zhang, Metal-free nucleophilic 7,8-dearomatization of quinolines: Spiroannulation of aminoquinoline protected amino acids, Chin. Chem. Lett., 2023, 34, 107779 CrossRef CAS;
(b) Z. Zhang, X. Cao, G. Wang, G. Zhang and X. Zhang, Straightforward synthesis of biologically valuable nonsymmetrical malonamides under mild conditions, Green Chem., 2022, 24, 3035–3041 RSC;
(c) S. Guo, X. Wang, D. Zhao, Z. Zhang, G. Zhang, S. Tang and K. Sun, Convenient Access to Ester-Containing Quinolinones through a Sequential Radical Alkoxycarbonylation/Cyclization/Hydrolysis Process, Asian J. Org. Chem., 2022, 11, e202100812 CrossRef CAS;
(d) Z. Zhang, X. Song, G. Li, X. Li, D. Zheng, X. Zhao, H. Miao, G. Zhang and L. Liu, Synthesis of polycyclic spiro-fused indolines via IBX-mediated cascade cyclization, Chin. Chem. Lett., 2021, 32, 1423–1426 CrossRef CAS;
(e) K. Sun, S. Wang, R. Feng, Y. Zhang, X. Wang, Z. Zhang and B. Zhang, Copper-Catalyzed Radical Selenodifluoromethylation of Alkenes: Access to CF2-Containing gamma-Lactams, Org. Lett., 2019, 21, 2052–2055 CrossRef CAS PubMed.
-
(a) A. P. Maximiano, G. S. Ramos, M. V. Marques and M. M. Sá, Functionalized Cyclopropanes as Versatile Intermediates for the Diversity-Oriented Synthesis of γ-Lactones, γ-Lactams and δ-Lactams, Synthesis, 2021, 53, 2408–2421 CrossRef CAS;
(b) A. B. Mhetre, H. Lee, H. Yang, K. Lee, D.-H. Nam and D. Lim, Synthesis and anticancer activity of benzoselenophene and heteroaromatic derivatives of 1,2,9,9a-tetrahydrocyclopropa[c]benzo[e]indol-4-one (CBI), Org. Biomol. Chem., 2017, 15, 1198–1208 RSC;
(c) C. A. Kuttruff, M. Ferrara, T. Bretschneider, S. Hoerer, S. Handschuh, B. Nosse, H. Romig, P. Nicklin and G. J. Roth, Discovery of BI-2545: A Novel Autotaxin Inhibitor That Significantly Reduces LPA Levels in Vivo, ACS Med. Chem. Lett., 2017, 8, 1252–1257 CrossRef CAS PubMed;
(d) P. B. Chanda, K. E. Boyle, D. M. Brody, V. Shukla and D. L. Boger, Synthesis and evaluation of duocarmycin SA analogs incorporating the methyl 1,2,8,8a-tetrahydrocyclopropa[c]imidazolo[4,5-e]indol-4-one-6-carboxylate (CImI) alkylation subunit, Bioorg. Med. Chem., 2016, 24, 4779–4786 CrossRef PubMed;
(e) S. Jones, J. Ahmet, K. Ayton, M. Ball, M. Cockerill, E. Fairweather, N. Hamilton, P. Harper, J. Hitchin, A. Jordan, C. Levy, R. Lopez, E. McKenzie, M. Packer, D. Plant, I. Simpson, P. Simpson, I. Sinclair, T. C. P. Somervaille, H. Small, G. J. Spencer, G. Thomson, M. Tonge, I. Waddell, J. Walsh, B. Waszkowycz, M. Wigglesworth, D. H. Wiseman and D. Ogilvie, Discovery and Optimization of Allosteric Inhibitors of Mutant Isocitrate Dehydrogenase 1 (R132H IDH1) Displaying Activity in Human Acute Myeloid Leukemia Cells, J. Med. Chem., 2016, 59, 11120–11137 CrossRef CAS PubMed;
(f) H. P. Cho, D. W. Engers, D. F. Venable, C. M. Niswender, C. W. Lindsley, P. J. Conn, K. A. Emmitte and A. L. Rodriguez, A Novel Class of Succinimide-Derived Negative Allosteric Modulators of Metabotropic Glutamate Receptor Subtype 1 Provides Insight into a Disconnect in Activity between the Rat and Human Receptors, ACS Chem. Neurosci., 2014, 5, 597–610 CrossRef CAS PubMed;
(g) P. K. Mandal, F. Gao, Z. Lu, Z. Ren, R. Ramesh, J. S. Birtwistle, K. K. Kaluarachchi, X. Chen, R. C. Bast, W. S. Liao and J. S. McMurray, Potent and Selective Phosphopeptide Mimetic Prodrugs Targeted to the Src Homology 2 (SH2) Domain of Signal Transducer and Activator of Transcription 3, J. Med. Chem., 2011, 54, 3549–3563 CrossRef CAS PubMed.
-
(a) L. Ye, K.-Y. Lo, Q. Gu and D. Yang, Pd-Catalyzed Intramolecular Aminoalkylation of Unactivated Alkenes: Access to Diverse N-Heterocycles, Org. Lett., 2017, 19, 308–311 CrossRef CAS PubMed;
(b) W. Du, Q. Gu, Z. Li and D. Yang, Palladium(II)-Catalyzed Intramolecular Tandem Aminoalkylation via Divergent C(sp3)–H Functionalization, J. Am. Chem. Soc., 2015, 137, 1130–1135 CrossRef CAS PubMed;
(c) D. Xing and D. Yang, Pd(II)-Catalyzed Intramolecular 1,2-Aminoalkylation of Conjugated 1,3-Dienes for the Synthesis of Pyrrolizidines, Org. Lett., 2013, 15, 4370–4373 CrossRef CAS PubMed;
(d) K.-T. Yip, N.-Y. Zhu and D. Yang, Palladium-Catalyzed Highly Diastereoselective Oxidative Cascade Cyclization Reactions, Org. Lett., 2009, 11, 1911–1914 CrossRef CAS PubMed;
(e) W. He, K.-T. Yip, N.-Y. Zhu and D. Yang, Pd(II)/tBu-quinolineoxazoline: An Air-Stable and Modular Chiral Catalyst System for Enantioselective Oxidative Cascade Cyclization, Org. Lett., 2009, 11, 5626–5628 CrossRef CAS PubMed;
(f) P. H. Fuller and S. R. Chemler, Copper(II) Carboxylate-Promoted Intramolecular Carboamination of Alkenes for the Synthesis of Polycyclic Lactams, Org. Lett., 2007, 9, 5477–5480 CrossRef CAS PubMed.
-
(a) K.-T. Yip, M. Yang, K.-L. Law, N.-Y. Zhu and D. Yang, Pd(II)-Catalyzed Enantioselective Oxidative Tandem Cyclization Reactions. Synthesis of Indolines through C−N and C−C Bond Formation, J. Am. Chem. Soc., 2006, 128, 3130–3131 CrossRef CAS PubMed;
(b) S. B. Nallapati, R. Adepu, M. A. Ashfaq, B. Y. Sreenivas, K. Mukkanti and M. Pal, A Pd-catalyzed direct entry to 11-substituted 6H-isoindolo[2,1-a]indol-6-one derivatives as potential anticancer agents, RSC Adv., 2015, 5, 88686–88691 RSC.
-
(a) H. Wang, Z. Bai, T. Jiao, Z. Deng, H. Tong, G. He, Q. Peng and G. Chen, Palladium-Catalyzed Amide-Directed Enantioselective Hydrocarbofunctionalization of Unactivated Alkenes Using a Chiral Monodentate Oxazoline Ligand, J. Am. Chem. Soc., 2018, 140, 3542–3546 CrossRef CAS PubMed;
(b) Z. Z. Zhang, Y. Q. Han, B. B. Zhan, S. Wang and B. F. Shi, Synthesis of Bicyclo[n.1.0]alkanes by a Cobalt-Catalyzed Multiple C(sp3)-H Activation Strategy, Angew. Chem., Int. Ed., 2017, 56, 13145–13149 CrossRef CAS PubMed;
(c) G. Liao, X. S. Yin, K. Chen, Q. Zhang, S. Q. Zhang and B. F. Shi, Stereoselective alkoxycarbonylation of unactivated C(sp3)-H bonds with alkyl chloroformates via Pd(II)/Pd(IV) catalysis, Nat. Commun., 2016, 7, 12901–12910 CrossRef PubMed;
(d) Y. J. Liu, Y. H. Liu, Z. Z. Zhang, S. Y. Yan, K. Chen and B. F. Shi, Divergent and Stereoselective Synthesis of beta-Silyl-alpha-Amino Acids through Palladium-Catalyzed Intermolecular Silylation of Unactivated Primary and Secondary C-H Bonds, Angew. Chem., Int. Ed., 2016, 55, 13859–13862 CrossRef CAS PubMed;
(e) S.-Y. Zhang, Q. Li, G. He, W. A. Nack and G. Chen, Pd-Catalyzed Monoselective ortho-C-H Alkylation of N-Quinolyl Benzamides: Evidence for Stereoretentive Coupling of Secondary Alkyl Iodides, J. Am. Chem. Soc., 2015, 137, 531–539 CrossRef CAS PubMed;
(f) B. Wang, W. A. Nack, G. He, S.-Y. Zhang and G. Chen, Palladium-catalyzed trifluoroacetate-promoted mono-arylation of the beta-methyl group of alanine at room temperature: synthesis of beta-arylated alpha-amino acids through sequential C-H functionalization, Chem. Sci., 2014, 5, 3952–3957 RSC;
(g) B. Wang, C. Lu, S.-Y. Zhang, G. He, W. A. Nack and G. Chen, Palladium-Catalyzed Stereoretentive Olefination of Unactivated C(sp3)-H Bonds with Vinyl Iodides at Room Temperature: Synthesis of beta-Vinyl alpha-Amino Acids, Org. Lett., 2014, 16, 6260–6263 CrossRef CAS PubMed.
-
(a) H.-Q. Yang, Q.-Q. Chen, F. Liu, R. Shi and Y. Chen, Highly efficient photocatalytic Suzuki coupling reaction by Pd3P/CdS catalyst under visible-light irradiation, Chin. Chem. Lett., 2021, 32, 676–680 CrossRef CAS;
(b) X. Wang, S. Zhang, P. Cui and S. Li, Modular Synthesis of Drimane Meroterpenoids Leveraging Decarboxylative Borylation and Suzuki Coupling, Org. Lett., 2020, 22, 8702 CrossRef CAS PubMed;
(c) Y. Bai, X. Li, X. Xiao, J. Liu, J. Yang and J. Wang, Synthesis of Imidazolium Precursors for the Hydroxyl-Group-Modified N-Heterocyclic Carbenes and Applications of the in situ Generated Carbene Ligands in Suzuki-Miyaura and Sonogashira Coupling Reactions, Chinese J. Org. Chem., 2017, 37, 1258–1265 CrossRef CAS;
(d) Y.-L. Zhao, Y. Li, S.-M. Li, Y.-G. Zhou, F.-Y. Sun, L.-X. Gao and F.-S. Han, A Highly Practical and Reliable Nickel Catalyst for Suzuki-Miyaura Coupling of Aryl Halides, Adv. Synth. Catal., 2011, 353, 1543–1550 CrossRef CAS;
(e) M. Pudlo, D. Csányi, F. Moreau, G. Hajós, Z. Riedl and J. Sapi, First Suzuki-Miyaura type cross-coupling of ortho-azidobromobenzene with arylboronic acids and its application to the synthesis of fused aromatic indole-heterocycles, Tetrahedron, 2007, 63, 10320–10329 CrossRef CAS.
-
(a) O. Y. Yuen, M. P. Leung, C. M. So, R. W.-Y. Sun and F. Y. Kwong, Palladium-Catalyzed Direct Arylation of Polyfluoroarenes for Accessing Tetra-ortho-Substituted Biaryls: Buchwald-type Ligand Having Complementary -PPh2 Moiety Exhibits Better Efficiency, J. Org. Chem., 2018, 83, 9008–9017 CrossRef CAS PubMed;
(b) H. H. Butani, D. D. Vachhani, U. C. Bhoya, A. K. Shah and E. V. Van der Eycken, Regio- and Chemoselective Formation of Spiroindolinone-Isoindolinone by a Palladium-Catalyzed Buchwald-Hartwig Addition-Elimination Sequence, Eur. J. Org Chem., 2014, 2014, 6634–6638 CrossRef CAS;
(c) N. Sharma, Z. Li, U. K. Sharma and E. V. Van der Eycken, Facile Access to Functionalized Spiro[indoline-3,2'-pyrrole]-2,5'-diones via Post-Ugi Domino Buchwald-Hartwig/Michael
Reaction, Org. Lett., 2014, 16, 3884–3887 CrossRef CAS PubMed.
-
(a) X. Zhang, D. Qi, C. Jiao, X. Liu and G. Zhang, Nickel-catalyzed deaminative Sonogashira coupling of alkylpyridinium salts enabled by NN2 pincer ligand, Nat. Commun., 2021, 12, 4904 CrossRef CAS PubMed;
(b) R. R. Liu, Y. G. Wang, Y. L. Li, B. B. Huang, R. X. Liang and Y. X. Jia, Enantioselective Dearomative Difunctionalization of Indoles by Palladium-Catalyzed Heck/Sonogashira Sequence, Angew. Chem., Int. Ed., 2017, 56, 7475–7478 CrossRef CAS PubMed;
(c) T. Tang, X.-D. Fei, Z.-Y. Ge, Z. Chen, Y.-M. Zhu and S.-J. Ji, Palladium-Catalyzed Carbonylative Sonogashira Coupling of Aryl Bromides via tert-Butyl Isocyanide Insertion, J. Org. Chem., 2013, 78, 3170–3175 CrossRef CAS PubMed;
(d) R. Chinchilla and C. Najera, Recent advances in Sonogashira reactions, Chem. Soc. Rev., 2011, 40, 5084–5121 RSC;
(e) R. Chinchilla and C. Nájera, The Sonogashira Reaction: A Booming Methodology in Synthetic Organic Chemistry, Chem. Rev., 2007, 107, 874–922 CrossRef CAS PubMed.
-
(a) S. Wang, X. Zhao, D. Zhang-Negrerie and Y. Du, Reductive cleavage of the N–O bond: elemental sulfur-mediated conversion of N-alkoxyamides to amides, Org. Chem. Front., 2019, 6, 347–351 RSC;
(b) M. T. Knowe, M. W. Danneman, S. Sun, M. Pink and J. N. Johnston, Biomimetic Desymmetrization of a Carboxylic Acid, J. Am. Chem. Soc., 2018, 140, 1998–2001 CrossRef CAS PubMed;
(c) Z. Zhang, Y. Zhang, G. Huang and G. Zhang, Organoiodine reagent-promoted intermolecular oxidative amination: synthesis of cyclopropyl spirooxindoles, Org. Chem. Front., 2017, 4, 1372–1375 RSC;
(d) C.-H. Lin, D. Pursley, J. E. M. N. Klein, J. Teske, J. A. Allen, F. Rami, A. Köhn and B. Plietker, Non-decarbonylative photochemical versus thermal activation of Bu4N[Fe(CO)3(NO)] – the Fe-catalyzed Cloke–Wilson rearrangement of vinyl and arylcyclopropanes, Chem. Sci., 2015, 6, 7034–7043 RSC.
Footnote |
† Electronic supplementary information (ESI) available: NMR spectra, HRMS, and single-crystal X-ray diffraction data (PDF). CCDC 2142480. For ESI and crystallographic data in CIF or other electronic format see DOI: https://doi.org/10.1039/d3ra05440c |
|
This journal is © The Royal Society of Chemistry 2023 |
Click here to see how this site uses Cookies. View our privacy policy here.