DOI:
10.1039/D3RA06268F
(Paper)
RSC Adv., 2023,
13, 33453-33458
Utility of dansyl chloride for the establishment of a sensitive spectrofluorimetric approach for estimating midodrine hydrochloride: application to content uniformity testing
Received
14th September 2023
, Accepted 1st November 2023
First published on 14th November 2023
Abstract
A new, straightforward spectrofluorimetric approach with high sensitivity was established for determining midodrine hydrochloride based on derivatizing this drug through its reaction with 5-(dimethylamino)naphthalene-1-sulfonyl chloride (dansyl chloride). The highly fluorescent product was extracted with methylene chloride, and then its emission was measured at 532 nm after excitation at 339 nm. The reaction was conducted in aqueous medium containing 0.1 M borate buffer (pH 8.2). The results showed that the proposed method is sensitive with high linearity in the range from 0.1 to 3 μg mL−1. The lower limits of detection and quantitation were 29 and 88 ng mL−1, respectively. Furthermore, the proposed approach was analytically assessed by applying the ICH guidelines. The suggested approach was effectively utilized for the estimation of the medicine in its marketable tablet formulations with excellent recovery and without any interfering effect from excipients. Moreover, the presented approach was utilized to test the content uniformity of commercial tablets following the USP guidelines.
1 Introduction
Midodrine hydrochloride is 2-amino-N-[2-(2,5-dimethoxyphenyl)-2-hydroxyethyl]-acetamide monohydrochloride. This drug undergoes a biotransformation, resulting in the formation of desglymidodrine, which is the active metabolite. The action of the formed desglymidodrine mimics the sympathetic nervous system, resulting in the elevation of the blood pressure with a rise in vascular tone. Midodrine is also used for the treatment of hypotension related to dialysis, orthostatic hypotension, and desautonomia.1–5
To date, many analytical methods have been reported for estimating the above-mentioned drug, including chromatographic,6–15 spectrofluorimetric,16–19 spectrophotometric,20–22 and electrochemical methods.23–25 However, the conventional methods employed for the quantification of midodrine hydrochloride, such as high-performance liquid chromatography (HPLC), often suffer from several limitations, such as time-consuming sample preparation and the use of expensive and highly pure organic solvents, while ultraviolet-visible (UV-vis) spectroscopy has low sensitivity and possible interference from excipients present in the formulation. Therefore, it is necessary to develop sensitive, accurate, and efficient analytical methods for the estimation of the midodrine hydrochloride content in formulations.
This study aimed to develop an alternative spectrofluorimetric method for the estimation of the above-mentioned drug via derivatization with dansyl chloride. This method is simple, fast, sensitive, and relatively inexpensive. Furthermore, it is highly suitable for analyzing this drug in tablets and examining the uniformity in its content.
The proposed spectrofluorimetric method offers several advantages compared to the reported techniques. First, the derivatization reaction facilitates the conversion of midodrine hydrochloride into a fluorophore, which results in a significantly enhanced fluorescence signal, thereby improving the method's sensitivity. Secondly, the difference between the excitation and emission wavelengths (Stokes shift) of the dansyl chloride derivative is large, which can minimize background interference from excipients, enhancing the specificity of the method. Additionally, this method offers simplicity and rapidity in terms of sample preparation and analysis, making it suitable for routine quality control applications.
2. Experimental
2.1. Instrument
A Jasco FP-8350 spectrofluorimeter was employed to perform the spectrofluorimetric measurements. The apparatus had a 150 W Xenon-arc lamp and 400 V PMT voltage. A 5 nm slit width was set for both the emission and excitation monochromators, and a scan rate of 1000 nm min−1 was used.
2.2. Chemicals and reagents
Dansyl chloride was purchased from Sigma (St. Louis, USA). Briefly, 20 mg of the reagent was dissolved in 100 mL acetone to get a 0.02% w/v solution. Boric acid, sodium hydroxide, sodium bicarbonate, methylene chloride, 1,2-dichloroethane, acetone, chloroform, n-hexane, isobutyl methyl ketone, isopropyl alcohol, ethanol, methanol, and 1-butanol were purchased from El Nasr Company for Chemicals (Cairo, Egypt). The Nile Company for Pharmaceuticals and Chemical Industries (Cairo, Egypt) graciously donated midodrine hydrochloride as a gift. Midodrine® tablets having 2.5 mg of drug per tablet were bought from a local pharmacy in Egypt. To prepare the borate buffer solution, various portions of 0.1 M boric acid and 0.1 M NaOH were mixed to achieve the intended pH.
2.3. Standard drug solution
For the preparation of the midodrine hydrochloride stock solution, an accurate weight of 10.0 mg of pure drug was transferred to a 100 mL calibrated flask and dissolved in distilled water. The volume was completed to the mark using the same solvent. Aliquots of the stock solution were further diluted to 100 mL with distilled water to obtain the desired working concentration.
2.4. Recommended analytical procedure
Different volumes of working drug solution in the range of 0.1–3.0 μg mL−1 were placed in 10 mL stoppered glass tubes, followed by the addition of 0.4 mL of 0.1 M borate buffer solution (pH 8.2) and 0.45 mL of 0.02% dansyl chloride solution. The tubes were left at ambient temperature for 25 min. The fluorescent product was extracted with 3 × 3 mL methylene chloride and its fluorescence was recorded at an emission wavelength of 532 nm upon excitation at 339 nm. A blank was performed in parallel with the test experiment.
2.5. Procedure for commercial tablets
Twenty tablets of the marketed Midodrine dosage form were accurately weighed, ground, and made homogenous. An accurate weight equivalent to 10.0 mg of the powdered medication was sonicated with a suitable volume of distilled water in a 100 mL calibrated flask for 20 min. After dilution with distilled water to the mark, the solution was filtered. After dilution of the filtrate to get solutions in the required concentration range, the recommended analytical procedure was employed.
2.6. Procedure for content uniformity test
Ten tablets of midodrine containing 2.5 mg each was separately treated as previously stated. Then, a portion of filtered solution from each tablet was diluted individually to the desired working concentration before being subjected to general analytical procedures. The acceptance value was computed for testing the content uniformity using the USP guidelines.26
3. Results and discussion
Due to the presence of a primary amine moiety in the aliphatic side chain of midodrine, it could be derivatized into a fluorescent product. Dansyl chloride was reacted with the above-mentioned drug, yielding a product with high fluorescence (Fig. 1). The product was extracted in methylene chloride and its emission measured at 532 nm upon excitation at 339 nm (Fig. 2).
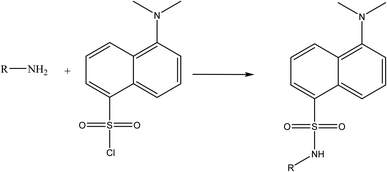 |
| Fig. 1 Suggested reaction pathway between the primary amine group of midodrine and dansyl chloride. | |
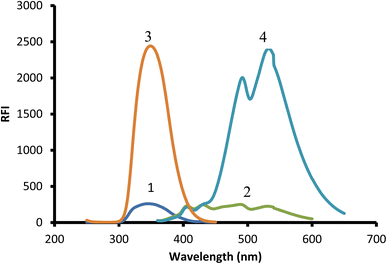 |
| Fig. 2 Excitation and emission spectra of 2 μg mL−1 midodrine-dansyl chloride reaction product (3 and 4) against blank (1 and 2). | |
3.1. Optimization of reaction conditions
The variables that may have an impact on the interaction of midodrine with dansyl chloride such as pH, reagent concentration, buffer volume, reaction time, and diluting solvent were investigated independently to determine the best reaction conditions.
3.1.1. Influence of buffer type and its pH. The recommended procedure was performed using different pH (7.0–12.0) using either 0.1 M borate buffer (pH 7.0–10.0) or 0.1 M bicarbonate buffer (9.0–12.0). In the case of borate buffer, the fluorescence of the product increased by increasing the pH up to pH 8.0, which remained nearly constant up to pH 8.5. A further increase in pH led to a remarkable decrease in fluorescence intensity. Bicarbonate buffer resulted in a lower fluorescence intensity, and thus borate buffer (pH 8.2) was chosen as the optimal condition for this reaction (Fig. 3).
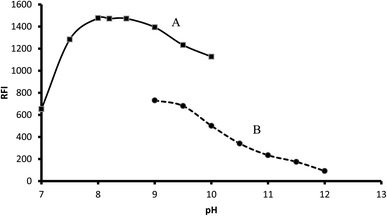 |
| Fig. 3 Influence of buffer type and pH on the fluorescence of 2 μg mL−1 midodrine-dansyl chloride reaction product using borate (A) and carbonate buffer (B). | |
3.1.2. Influence of buffer volume. Various aliquots of 0.1 M borate buffer (pH 8.2) were tested to determine their effect on the fluorescence of the product in the range of 0.02 mL to 1 mL. The results showed that an increase in the buffer volume resulted in an increase in the fluorescence intensity up 0.2 mL, which remained nearly unchanged up to 0.6 mL. A further increase in the buffer volume decreased the fluorescence intensity, and thus 0.3 mL was selected as the optimal buffer volume for the reaction (Fig. 4).
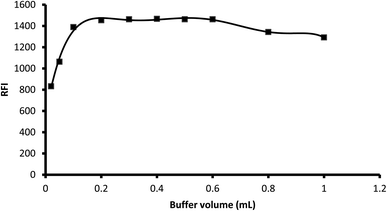 |
| Fig. 4 Influence of buffer volume on the fluorescence intensity of the reaction product of 2 μg mL−1 midodrine with dansyl chloride. | |
3.1.3. Influence of time. The fluorescence of the formed product was scanned at various periods (1–50 min) after mixing the reagents with the drug. The results showed that the reaction was completed after 20 min and the product was stable for another 10 min. Standing for a longer time resulted in a remarkable inhibition of fluorescence, and thus 25 min was selected as the optimal reaction time (Fig. 5).
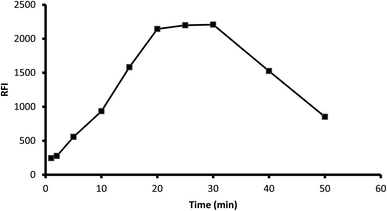 |
| Fig. 5 Influence of reaction time on the fluorescence of the reaction product of 2 μg mL−1 midodrine and dansyl chloride. | |
3.1.4. Influence of reagent concentration. Various volumes of dansyl chloride (0.02% g w/v) ranging from 0.01 mL to 1 mL were tested for their impact on the fluorescence of the product. The results showed that the fluorescence increased as the volume of the reagent increased up to 0.4 mL. After that, there was no remarkable change in the fluorescence intensity up to 0.5 mL. However, a distinct reduction in the fluorescence intensity was observed upon the addition of larger reagent volumes. Thus, 0.45 mL was selected as optimal for the present reaction (Fig. 6).
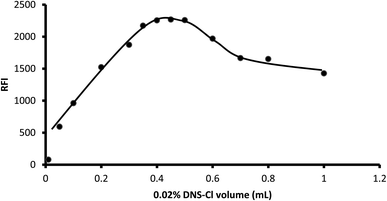 |
| Fig. 6 Influence of dansyl chloride volume on the fluorescence of its reaction product with 2 μg mL−1 midodrine. | |
3.1.5. Influence of extracting solvent. Numerous solvents were used for the extraction of the reaction product such as methyl isobutyl ketene, chloroform, methylene chloride (dichloromethane), 1,2-dichloroethane and n-hexane. High fluorescence intensity values were observed with all solvents except for isobutyl methyl ketene, which gave slightly lower fluorescence (Fig. 7). However, methylene chloride was chosen as the extracting solvent given that it is less toxic. The reaction mixture was directly diluted with different water-miscible solvents without subsequent extraction and measuring the fluorescence in the resulting solution. It was found that water and alcoholic solvents such as methanol, ethanol, isopropyl alcohol and 1-butanol showed very high blank values, and thus these solvents were excluded.
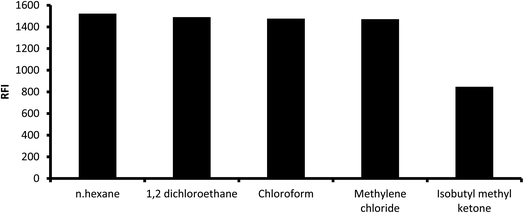 |
| Fig. 7 Influence of extracting solvent on the fluorescence intensity of the reaction product of 2 μg mL−1 midodrine and dansyl chloride. | |
3.2. Method validation
The proposed method was validated following the ICH guidelines27 with respect to range, linearity, LOD, LOQ, robustness, accuracy and precision.
3.2.1. Linearity and range. The outlined analytical method was used to analyze several standard drug solutions at various concentrations. By plotting the relative fluorescence intensity at 532 nm with drug concentration in μg mL−1, a calibration curve for the spectrofluorimetric technique was produced. The suggested method showed linearity in the concentration range of 0.1 to 3.0 μg mL−1. The obtained data, which are listed in Table 1, reflect the good linearity of the suggested approach.
Table 1 Analytical parameters for the analysis of the cited drug using the suggested method
Parameter |
Value |
Linear range (μg mL−1) |
0.1–3.0 |
Slope |
1140.4 |
Intercept |
−32.235 |
Standard deviation of slope |
6.121 |
Standard deviation of residuals |
15.902 |
Standard deviation of intercept |
10.042 |
Determination coefficient (r2) |
0.9999 |
Correlation coefficient (r) |
0.9999 |
LOQ (ng mL−1) |
88 |
LOD (ng mL−1) |
29 |
3.2.2. LOD and LOQ. The limit of detection (LOD) and limit of quantitation (LOQ) were computed obeying the ICH guidelines27 by applying the equation: LOD = 3.3 σ s−1 and LOQ = 10 σ s−1, where σ represents the standard deviation of the intercept and s is the slope of the graph. The LOQ value was 0.088 μg mL−1 and the LOD was 0.029 μg mL−1.
3.2.3. Accuracy. The % recovery of three distinct midodrine concentrations following measurement using the suggested method was used to assess the accuracy. Three replicates of each experiment were performed. The results are shown in percentage recovery mean and standard deviation, as shown in Table 2. The proximity of the results of % recovery to 100% at all the studied concentrations provided evidence of the acceptable level of accuracy of the current approach.
Table 2 Accuracy evaluation of the proposed spectrofluorimetric method
Sample number |
Concentration level (μg mL−1) |
% recoverya ± SD |
Values are the average of 3 measurements, SD is the standard deviation. |
1 |
0.10 |
98.7 ± 2.2 |
2 |
1.00 |
104.98 ± 0.23 |
3 |
2.00 |
101.93 ± 0.86 |
3.2.4. Precision. For the determination of interday and intraday precision, three different concentrations in the linearity range were examined using the suggested method within the same day for the evaluation of the intraday precision and in three successive days for the evaluation of the interday precision. All experiments were carried out in triplicate. The good precision of the suggested approach was proven by the small value of computed % RSD, as listed in Table 3.
Table 3 Precision evaluation of the suggested spectrofluorimetric method
Sample number |
Concentration level (μg mL−1) |
Intra-day precision |
Inter-day precision |
%recovery a ± SD |
RSD |
%recovery a ± SD |
RSD |
Values are the average of 3 measurements, RSD is the relative standard deviation and SD is the standard deviation. |
1 |
0.10 |
99.58 ± 0.5 |
0.50 |
98.7 ± 1.01 |
1.03 |
2 |
1.00 |
104.95 ± 0.5 |
0.48 |
104.63 ± 0.23 |
0.22 |
3 |
2.00 |
103.27 ± 0.25 |
0.24 |
102 ± 1.2 |
1.18 |
3.2.5. Robustness. The robustness of the current analytical protocol was assessed by incorporating small variations in the conditions of the experiment including buffer volume, pH, and dansyl chloride volume, and then calculating the percent recovery. The data presented in Table 4 show that a small change in these parameters had no significant effect on the performance of the method.
Table 4 Robustness evaluation of the proposed spectrofluorimetric method
Parameter |
%recoverya ± SD |
Values are the average of 3 measurements and SD is the standard deviation. |
pH |
8.0 |
102.17 ± 0.49 |
8.2 |
101.57 ± 0.39 |
8.4 |
102.62 ± 0.14 |
Buffer volume |
0.2 |
102.66 ± 0.22 |
0.3 |
101.7 ± 0.24 |
0.4 |
102.37 ± 1.27 |
0.02% dansyl chloride volume |
0.4 |
102.33 ± 0.07 |
0.45 |
102.28 ± 0.09 |
0.5 |
102.44 ± 0.15 |
3.3. Application of the suggested method
3.3.1. Assay of midodrine commercial tablets. The proposed spectrofluorimetric approach was effectively applied for the analysis of midodrine hydrochloride in its tablet dosage forms and a high value of % recovery (99.11 ± 0.28) was obtained. By comparing the suggested and reported method16 by calculating the t-value and F-value, it was observed that the calculated value of each parameter did not significantly exceed its corresponding theoretical value at the 95% confidence interval. This revealed the good accuracy and precision of the current approach. Moreover, the high % recovery provided evidence of non-significant interference from common excipients, as shown in Table 5.
Table 5 Application of the proposed spectrofluorimetric method to tablet dosage form and comparison with the reported method
Dosage form |
Labelled content |
Mean %recovery a± SD |
t-valueb |
F-valueb |
Proposed method |
Reported method |
Results are the average value of three determinations, while SD represents the standard deviation. The tabulated t-value and F-value at the 95% confidence limit are 2.78 and 6.39, respectively. |
Midodrine® tablets |
2.5 mg |
99.11 ± 0.29 |
99.2 ± 0.3 |
0.386 |
1.158 |
3.3.2. Application to content uniformity test. Generally, a content uniformity test is carried out if the content of the active constituent of the tablet is less than 25 mg or if the active constituent content does not exceed 25% of total tablet weight. In the present work, the content uniformity test was carried out for 10 tablets of midodrine, each was labeled to contain 2.5 mg of midodrine hydrochloride. The recommended procedure was applied for individual tablets in triplicate and the % recovery, in addition to the standard deviation was estimated for each tablet. The test was performed according to the USP guidelines.26 The acceptance value was computed and found to be less than the maximum permitted value, as shown in Table 6. Therefore, the examined dosage forms are considered uniform.
Table 6 Application of the proposed spectrofluorimetric method to content uniformity testing of Midodrine® 2.5 mg tablets
Tablet no |
% recovery a |
Results are the average value of three determinations. |
1 |
98.99 |
2 |
99.94 |
3 |
99.36 |
4 |
99.93 |
5 |
99.17 |
6 |
99.30 |
7 |
100.02 |
8 |
100.03 |
9 |
99.62 |
10 |
100.17 |
Mean |
99.65 |
SD |
0.42 |
RSD |
0.42 |
Acceptance value AV |
1.01 |
Max allowed AV(L1) |
15 |
3.4. Comparison of the proposed method with the published fluorimetric methods
Four spectrofluorimetric methods16–19 were previously reported for the determination of MDR. A comparison of the current method with the published spectrofluorimetric methods is presented in Table 7. As shown in this table, two of the reported methods17,18 have lower sensitivity for MDR than the proposed spectrofluorimetric method. In addition, no heating step was required compared to the method based on ninhydrin condensation.17 Furthermore, the utilized reagent (DNS-Cl) is relatively cheap compared to the extremely expensive fluorescamine reagent.18 Although the reported method16 based on the measurement of the native fluorescence of the drug had a comparable analytical performance to the proposed method, it suffered from a low Stokes shift. The excitation/emission wavelengths were 290/315 (Δλ = 25 nm) in the reported method and 339/532 nm in the proposed method, respectively. Thus, the proposed method has a very large Stokes shift, (Δλ = 193 nm). Some problems are associated with a low Stokes shift in fluorimetric measurements. A low Stokes shift may result in significant spectral overlap between the excitation and emission spectra. It can also increase the probability of scattering and reabsorption of the excitation light by the sample. Thus, it can cause a decrease in the observed fluorescence signal. Furthermore, it can lead to increased background noise and decreased signal-to-noise ratio, which potentially affect the sensitivity and accuracy of the measurements. Therefore, the proposed method has the merits of high sensitivity, simple, and cost-effectiveness.
Table 7 Analysis of the developed approach against the reported spectrofluorimetric methods
No. |
Reagent |
λex/λem (nm) |
Conditions |
Linear range (μg mL−1) |
LOD (μg mL−1) |
LOQ (μg mL−1) |
Ref. |
1 |
Native |
290/315 |
Room temp |
0.1–2.0 |
0.03 |
0.10 |
16 |
2 |
Ninhydrin/phenylacetaldehyde |
388/450 |
Heated at 70 °C for 12 min |
0.5–6.0 |
0.16 |
0.48 |
17 |
3 |
Fluorescamine |
388/462 |
5 min/room temp |
0.2–3.0 |
0.06 |
0.19 |
18 |
4 |
Ortho-phthalaldehyde/2-mercapto-ethanol |
334/451 |
15 min/room temp |
0.1–1.5 |
0.031 |
0.094 |
19 |
5 |
DNS-Cl |
339/532 |
25 min/room temp |
0.1–3.0 |
0.029 |
0.088 |
This work |
4. Conclusion
Based on the interaction of the primary amine of midodrine with dansyl chloride, a highly sensitive spectrofluorimetric approach was established. The present approach was found to be suitable for the analysis of the cited drug in its pure form and tablet formulations, in addition to content uniformity testing. This approach offers the benefits of being simple, rapid, sensitive and performed at ambient temperature with no need for heating. Thus, it can be applied for the routine analysis of the drug in quality-control units.
Conflicts of interest
The authors declare that they have no competing financial interests.
References
- F. B. Axelrod, L. Krey, J. S. Glickstein, J. W. Allison and D. Friedman, J. Auton. Nerv. Syst., 1995, 55, 29–35 CrossRef CAS PubMed.
- P. A. Low, Jama, 1997, 277, 1046 CrossRef CAS.
- S. Prakash, A. X. Garg, A. P. Heidenheim and A. A. House, Nephrol., Dial., Transplant., 2004, 19, 2553–2558 CrossRef CAS PubMed.
- R. Karwa and C. B. Woodis, Ann. Pharmacother., 2009, 43, 692–699 CrossRef CAS PubMed.
- O. Jans, J. Mehlsen, P. Kjærsgaard-Andersen, H. Husted, S. Solgaard, J. Josiassen, T. H. Lunn and H. Kehlet, Anesthesiology, 2015, 123, 1292–1300 CrossRef CAS PubMed.
- W. Poscht and W. Lindners, Biomed. Chromatogr., 1989, 3, 153–156 CrossRef.
- M. G. Quaglia, A. Farina, E. Bossù and V. Cotichini, J. Pharm. Biomed. Anal., 1998, 18, 171–177 CrossRef CAS.
- J. Jia, G. Liu, S. Li, X. Li, W. Yuan, C. Yu and Y. Wang, J. Pharm. Biomed. Anal., 2006, 40, 715–719 CrossRef CAS PubMed.
- T. Aomori, N. Matsumoto, K. Nakamura, Y. Fujita, M. Sekizuka, T. Araki, T. Nakamura and K. Yamamoto, J. Liq. Chromatogr. Relat. Technol., 2011, 34, 2411–2419 CrossRef CAS.
- T. Barth, J. Aleu, M. T. Pupo, P. S. Bonato and I. G. Collado, J. Chromatogr. Sci., 2013, 51, 460–467 CAS.
- A. A. Ali, M. A. Al-Ghobashy, S. F. Farid and M. A. Kassem, J. Chromatogr. B: Anal. Technol. Biomed. Life Sci., 2015, 991, 34–40 CrossRef CAS PubMed.
- D. P. Patel, S. Nair, B. N. Suhagia and B. M. Patel, J. Pharm. Biomed. Anal., 2016, 131, 355–363 CrossRef CAS PubMed.
- H. K. Jain and A. A. Gunjal, Int. J. Appl. Pharm., 2017, 9, 121–127 CrossRef CAS.
- G. Byran, J. Ashwini, K. Lakshmanan, K. Rajagopal, G. Subramanian and S. N. Meyyanathan, Drug Dev. Ind. Pharm., 2021, 47, 1072–1078 CrossRef CAS PubMed.
- M. Abdelgaleel, D. M. Nagi, S. M. Derayea and P. Y. Khashaba, J. Pharm. Biomed. Anal., 2023, 229, 115322 CrossRef PubMed.
- M. A. Omar, D. M. Nagy and M. E. Halim, Luminescence, 2019, 34, 854–858 CrossRef CAS.
- M. A. Omar, D. M. Nagy and M. E. Halim, Microchem. J., 2019, 146, 544–547 CrossRef CAS.
- M. A. Omar, D. M. Nagy and M. E. Halim, Luminescence, 2019, 34, 84–89 CrossRef CAS PubMed.
- P. Y. Khashaba, M. Abdelgaleel, S. M. Derayea and D. M. Nagy, Spectrochim. Acta, Part A, 2022, 273, 121046 CrossRef CAS PubMed.
- M. S. Elazazy, RSC Adv., 2015, 5, 48474–48483 RSC.
- S. G. Nair, J. V. Shah, P. A. Shah, M. Sanyal and P. S. Shrivastav, Cogent Chem., 2015, 1, 1075852 CrossRef.
- S. G. Nair, J. V Shah, P. A. Shah, M. Sanyal and P. S. Shrivastav, Eurasian J. Anal. Chem., 2015, 10, 68–83 CAS.
- E. S. Elzanfaly, H. E. Zaazaa and H. A. Merey, Acta Chim. Slov., 2013, 60, 256–262 CAS.
- W. M. Salem, Egypt. J. Chem., 2020, 63, 2185–2199 Search PubMed.
- S. D. Al-Qahtani, A. Hameed, E. Aljuhani, R. Shah, A. Alharbi, B. H. Asghar and N. M. El-Metwaly, Arabian J. Chem., 2021, 14, 103446 CrossRef CAS.
- M. Rockville, Electron. version, 2007, pp. 2287–2288 Search PubMed.
- I. C. H. H. T. Guideline and others, Q2, 2005, 1, 5.
|
This journal is © The Royal Society of Chemistry 2023 |
Click here to see how this site uses Cookies. View our privacy policy here.