DOI:
10.1039/D3RA06678A
(Paper)
RSC Adv., 2023,
13, 32772-32777
One-pot four-component synthesis of novel isothiourea-ethylene-tethered-piperazine derivatives†
Received
1st October 2023
, Accepted 1st November 2023
First published on 7th November 2023
Abstract
An efficient metal-free four-component approach for the synthesis of piperazine derivatives tethered to an isothiourea group through an ethylene link was developed. 1,4-Diazabicyclo[2.2.2]octane (DABCO) salts, generated in situ through the reactions of DABCO with various alkyl bromides, reacted with phenylisothiocyanate (PITC) and amines in a one-pot manner to give the target products. Initially, through two parallel nucleophilic paths, DABCO and the secondary amine adds to the alkyl bromide and PITC, respectively. The process is followed by the combination of the two respective intermediates to produce the final products by forming a new C–S bond with the expense of a C–N bond cleavage. Consequently, various DABCO salts and secondary amines were tolerated well in this protocol to afford the isothiourea-ethylene-tethered-piperazine compounds in good to high yields.
Introduction
Multicomponent reactions (MCRs) have emerged as a promising synthetic strategy in organic chemistry in recent decades, since they furnish one-pot routes to convert commercially available reactants to complex structures.1,2 In addition, MCRs are attractive from economical, operational simplicity, and green chemistry points of view.3,4 Consequently, MCRs are widely employed in the synthesis of various natural products,5 heterocyclic systems,6 and other molecules of interest.7–9 However, earlier MCRs often involved the use of limited starting materials, higher reaction temperatures, and toxic reagents. Thus, there is an ongoing demand for further improvement of MCRs by designing new processes with lower overall costs, better selectivity, higher efficiency, enhanced environmental aspects, and improved atom-economy.
Molecules containing isothiourea moieties constitute important structures in medicinal,10,11 biological,12,13 and agricultural chemistry.14,15 In addition, they are also employed as catalysts16,17 or reactive intermediates18 in other synthetic procedures. Illustrative related structures are highlighted in Fig. 1. Various methods are reported so far for the synthesis of these molecules. The majority of these reports are carried out using conventional stepwise approaches,19,20 while a few recent studies deal with three-component procedures. For instance, Sun et al. developed a three-component synthesis of isothioureas via the combination of isocyanides and amines with disulfides, where the latter component was initially activated by N-halogen succinimides using (2,2,6,6-tetramethylpiperidin-1-yl)oxyl (TEMPO).21 Alternatively, Maes devised a copper(I) catalyzed three-component reaction between thiosulfonates, amines, and isocyanides, resulting in the synthesis of isothiourea derivatives.22 Other important related reports include a tandem process by Wu,23 a three-component reaction by Mishra,24 and a binuclear aluminium complex mediated synthesis of carbodiimides by Panda.25
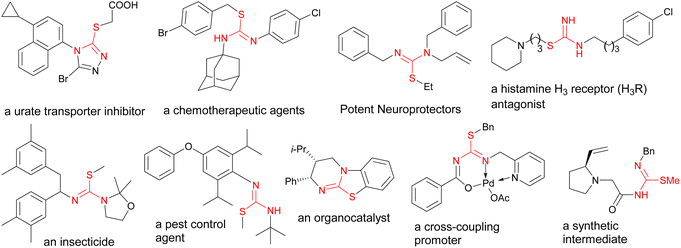 |
| Fig. 1 Important molecules containing the isothiourea functional group. | |
Despite all the studies carried out on isothioureas, many traditional methods suffer from the use of complicated steps, toxic reagents or additives, and poor reactivity of the starting materials. Therefore, there is a need for further development of efficient and sustainable synthetic methods involving isothiourea chemistry. In the framework of our program on MCRs26,27 and heterocyclic chemistry,28,29 we would like to report a novel four-component procedure for the synthesis of a new series of isothiourea containing piperazines, as exemplified in Scheme 1 for the reaction of diethylamine (Et2NH), phenyl isothiocyanate (PITC), 1,4-diazabicyclo[2.2.2]octane (DABCO), and 1-bromo-2-methylpropane.
 |
| Scheme 1 One-pot four-component approach for the synthesis of isothiourea-ethylene-tethered piperazine derivatives. | |
Although the use of DABCO bond cleavage for the synthesis of various piperazine derivatives has precedence,30–32 the current work is the first application of DABCO salts in the synthesis of the isothiourea functional group. For this purpose, we planned to use two very reactive species, the electrophilic isothiocyanate (PITC) moiety and the sterically hindered tertiary amine (DABCO), whose reactive natures can trigger parallel nucleophilic combinations of the reactants, which is a useful tool for launching multicomponent reactions. Consequently, this leads to a concurrent C–N bond cleavage and C–S bond formation reactions to produce the target products, in which the isothiourea and piperazine functional groups are placed in vicinity and would be interesting candidates for further biological studies.
Results and discussion
For simplicity, we first synthesized the DABCO salts 2 separately to start the study with a three-component process. Thus, to optimize the reaction, we subjected Et2NH 1a and PITC to combine with 2a under various conditions (Table 1). Treatment of a 1.0
:
1.0
:
1.0 mixture of the three reactants and K2CO3 at refluxing temperature in THF after 5 h led to the formation of 3a in 93% yield (entry 1). In the absence of the base (entry 2) or at lower temperatures (entries 3–4), the yield was diminished even at a longer reaction time. Similarly, use of other inorganic bases (entries 5–8) did not led to higher conversion of the reactants to 3a. Alternatively, no better results were achieved for conducting the reaction in other protic (entries 9–11) or aprotic (entries 12–16) solvents, conveying that K2CO3/THF/reflux conditions would provide the highest conversion of the reactants to the desired product.
Table 1 Three-component optimization of the synthesis of 3a

|
Entry |
Base |
Solvent |
T (°C) |
Time (h) |
Yielda (%) |
Isolated yields. |
1 |
K2CO3 |
THF |
Reflux |
5 |
93 |
2 |
— |
THF |
Reflux |
24 |
28 |
3 |
K2CO3 |
THF |
50 |
24 |
49 |
4 |
K2CO3 |
THF |
25 |
24 |
20 |
5 |
Na2CO3 |
THF |
Reflux |
24 |
59 |
6 |
K3PO4 |
THF |
Reflux |
24 |
54 |
7 |
KOH |
THF |
Reflux |
24 |
39 |
8 |
NaOH |
THF |
Reflux |
24 |
37 |
9 |
K2CO3 |
EtOH |
Reflux |
24 |
64 |
10 |
K2CO3 |
MeOH |
Reflux |
24 |
70 |
11 |
K2CO3 |
H2O |
Reflux |
24 |
16 |
12 |
K2CO3 |
DMF |
Reflux |
24 |
37 |
13 |
K2CO3 |
DMSO |
Reflux |
24 |
<5 |
14 |
K2CO3 |
CHCl3 |
Reflux |
24 |
26 |
15 |
K2CO3 |
CH2Cl2 |
Reflux |
24 |
<5 |
16 |
K2CO3 |
n-Hexane |
Reflux |
24 |
18 |
With these results in hand, next we extended the process into a four-component combination by primarily subjecting DABCO to react with various alkyl bromides to provide the required salts 2 for the following steps (Table 2). Consequently, when DABCO, Me2CHCH2Br, Et2NH, and PITC were reacted in this manner, 3a was produced after 12 h in 91% yield (entry 1). By using this approach, Et2NH and PITC reacted with other in situ generated derivatives of 2 to give 3b–e efficiently (entries 2–5). Similarly, products 3f–j were obtained in the same manner to emphasize the generality of the process (entries 6–10).
Table 2 Diversity scope of the process by 4-component synthesis of derivatives of 3a,b
Reaction conditions: DABCO (10.0 mmol), THF (30 mL) R′Br (10.0 mmol), rt, 10–12 h. Then added to this mixture R2NH (10.0 mmol), PITC (10.0 mmol), K2CO3 (10.0 mmol), THF (10 mL) reflux 4–6 h. Isolated yields. |
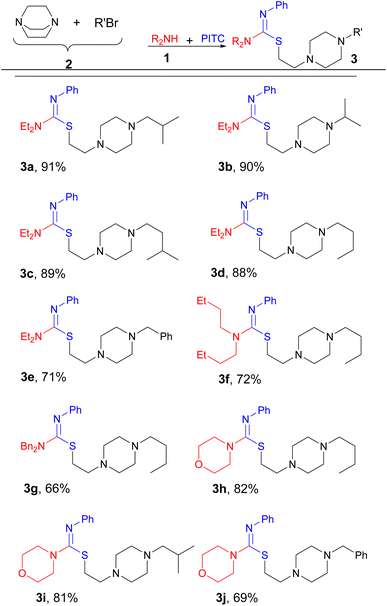 |
Based on these results, a mechanism would be proposed for this process. Initially, DABCO is alkylated via a nucleophilic attack on the alkyl bromide moiety to produce 2, while in a parallel reaction, Et2NH adds to PITC. The two resulting intermediates of the initial steps then would combine through K2CO3-activated attack of the diethyl-phenylthiourea species to the DABCO salt to access to the final products. The stereochemistry of the isothiourea functional group was assigned as Z (as seen in Fig. 2 (top) for the product with R = Et and R′ = CH2Ph). Such assignment for similar molecules resulting from the same chemistry is reported before in the literature.15,33 To further support the assignment, we engaged molecular mechanics calculations to verify the proposed stereochemistry. The results arising from molecular mechanics (MM2) calculations using ChemSoft's ChemOffice Pro (version 20) clearly show that the Z configuration (Fig. 2, bottom-left) is more stable that the E counterpart (Fig. 2, bottom-right).
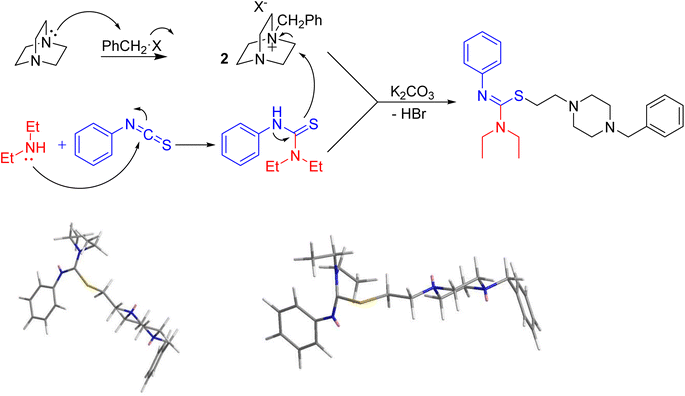 |
| Fig. 2 Proposed mechanism for the synthesis of the products (top) and configurational projection of MM2-energy-minimised 3e (bottom) obtained from ChemDraw Pro. | |
Experimental
General
FT-IR spectra were recorded using KBr disks on a Bruker Vector-22 spectrometer. NMR spectra were obtained on a Bruker AMX (300 MHz for 1H and 75 MHz for 13C) as CDCl3 solutions using TMS as internal standard reference. Elemental analyses were performed using a Thermo Finnigan Flash EA 1112 instrument. MS spectra were obtained on a Fisons 8000 Trio instrument at ionization potential of 70 eV. TLC experiments were carried out on pre-coated silica gel plates using petroleum ether/EtOAc as the eluent. Starting materials and reagents were purchased from commercial sources.
Synthesis of ammonium salts 2a from DABCO
To a solution of DABCO (1.12 g, 10.0 mmol) in THF (20 mL) was added Me2CHCH2Br (10.0 mmol), and the solution was stirred at room temperature for 12 h. A precipitate was formed, which was filtered, washed with ethyl acetate (10 mL) and dried under vacuum to get 2a.
Typical procedure for three-component synthesis of functionalized piperazines 3a
A mixture of 2a (0.11 g, 0.5 mmol), Et2NH (57.0 μL, 0.55 mmol), PITC (0.07 g, 0.5 mmol), and K2CO3 (0.069 g, 0.5 mmol) in THF (5 mL) was stirred at refluxing temperature for 5 h. After completion of the reaction, the solvent was evaporated under vacuum and the concentrated crude mixture was fractionated by column chromatography on silica gel (EtOAc/petroleum ether; 60/40) to afford the pure product. The isolated product was fully characterized by various spectroscopic methods.
Typical procedure for four-component synthesis of functionalized piperazines 3a
To a solution of DABCO (1.12 g, 10.0 mmol) in THF (30 mL) was added Me2CHCH2Br (10.0 mmol), and the solution was stirred at room temperature for 12 h to get 2a. To this was added Et2NH (1140 μL, 10.0 mmol), PITC (1.4 g, 10.0 mmol), and K2CO3 (1.4 g, 10.0 mmol) in THF (10 mL) and the mixture was stirred at refluxing temperature for 5 h. After completion of the reaction, the solvent was evaporated under vacuum and the concentrated crude mixture was fractionated by column chromatography on silica gel (EtOAc/petroleum ether; 60/40) to afford the pure product. The isolated product was fully characterized by various spectroscopic methods.
2-(4-Isobutylpiperazin-1-yl)ethyl (Z)-N,N-diethyl-N′-phenylcarbamimidothioate 3a
1H NMR (300 MHz, CDCl3) δ 7.21–7.16 (m, 2H), 6.92–6.86 (m, 3H), 3.54 (q, J = 7.0 Hz, 4H), 2.47–2.41 (m, 2H), 2.38–2.29 (m, 10H), 2.04 (d, J = 7.0 Hz, 2H), 1.70 (t, septet, J = 7.0, 6.5 Hz, 1H), 1.17 (t, J = 7.0 Hz, 6H), 0.85 (d, J = 6.5 Hz, 6H) ppm; 13C NMR (75 MHz, CDCl3) δ 152.3, 149.8, 128.3, 121.4, 121.2, 66.5, 57.4, 53.1, 52.4, 44.0, 28.6, 25.0, 20.7, 20.6, 13.5 ppm; IR (KBr) ν = 2953, 2807, 1568, 1227, 1112 cm−1; MS (70 eV) m/z 376 [M+], 235, 168, 125; anal. calcd for C21H36N4S: C, 66.97; H, 9.64; N, 14.88; S, 8.51. Found: C, 66.76; H, 9.80; N, 14.97; S, 8.67.
2-(4-Isopropylpiperazin-1-yl)ethyl (Z)-N,N-diethyl-N′-phenylcarbamimidothioate 3b
1H NMR (300 MHz, CDCl3) δ 7.29–7.23 (m, 2H), 6.97–6.91 (m, 3H), 3.53 (q, J = 7.0 Hz, 4H), 2.78 (septet, J = 6.5 Hz, 1H), 1.69–1.58 (m, 4H), 1.43–1.36 (m, 8H), 1.10 (d, J = 6.5 Hz, 6H), 0.99 (t, J = 7.0 Hz, 6H) ppm; 13C NMR (75 MHz, CDCl3) δ 153.2, 150.3, 128.6, 121.5, 121.1, 68.1, 49.8, 48.7, 30.7, 29.7, 29.6, 20.1, 14.0 ppm; IR (KBr) ν = 2954, 2809, 1577, 1227 cm−1; MS (70 eV) m/z 362 [M+], 279, 248, 192; anal. calcd for C20H34N4S: C, 66.25; H, 9.45; N, 15.45; S, 8.84. Found: C, 66.14; H, 9.23; N, 15.60; S, 9.05.
2-(4-Isopentylpiperazin-1-yl)ethyl (Z)-N,N-diethyl-N′-phenylcarbamimidothioate 3c
1H NMR (300 MHz, CDCl3) δ 7.21–7.15 (m, 2H), 6.94–6.84 (m, 3H), 3.53 (q, J = 7.0 Hz, 4H), 2.45–2.40 (m, 4H), 2.38–2.33 (m, 4H), 2.31–2.24 (m, 6H), 1.53 (t, septet, J = 7.0, 6.5 Hz, 1H), 1.36–1.29 (m, 2H), 1.16 (t, J = 7.0 Hz, 6H), 0.86 (d, J = 6.5 Hz, 6H) ppm; 13C NMR (75 MHz, CDCl3) δ 152.5, 149.9, 128.4, 121.4, 121.1, 57.4, 56.7, 53.0, 52.4, 44.1, 35.6, 28.7, 26.5, 22.6, 13.7 ppm; IR (KBr) ν = 2953, 2808, 1578, 1227, 1112 cm−1; MS (70 eV) m/z 390 [M+], 350, 318, 214, 182; anal. calcd for C22H38N4S: C, 67.64; H, 9.81; N, 14.34; S, 8.21. Found: C, 67.82; H, 10.08; N, 14.58; S, 8.05.
2-(4-Butylpiperazin-1-yl)ethyl (Z)-N,N-diethyl-N′-phenylcarbamimidothioate 3d
1H NMR (300 MHz, CDCl3) δ 7.21–7.15 (m, 2H), 6.92–6.86 (m, 3H), 3.54 (q, J = 7.0 Hz, 4H), 2.48–2.36 (m, 4H), 2.36–2.19 (m, 10H), 1.47–1.37 (m, 2H), 1.33–1.27 (m, 2H), 1.17 (t, J = 7.0 Hz, 6H), 0.88 (t, J = 7.0 Hz, 3H) ppm; 13C NMR (75 MHz, CDCl3) δ 152.6, 149.9, 128.4, 121.5, 121.3, 58.3, 57.4, 52.9, 52.4, 44.1, 28.8, 28.7, 20.6, 13.8, 13.6 ppm; IR (KBr) ν = 2957, 2808, 1577, 1227 cm−1; MS (70 eV) m/z 376 [M+], 264, 168, 125; anal. calcd for C21H36N4S: C, 66.97; H, 9.64; N, 14.88; S, 8.51. Found: C, 66.77; H, 9.83; N, 14.99; S, 8.43.
2-(4-Benzylpiperazin-1-yl)ethyl (Z)-N,N-diethyl-N′-phenylcarbamimidothioate 3e
1H NMR (300 MHz, CDCl3) δ 7.36–7.30 (m, 5H), 7.25–7.20 (m, 2H), 6.95–6.93 (m, 3H), 3.58 (q, J = 7.0 Hz, 4H), 3.52 (s, 2H), 2.57–2.37 (m, 12H), 1.21 (t, J = 7.0 Hz, 6H) ppm; 13C NMR (75 MHz, CDCl3) δ 152.8, 150.0, 129.3, 128.7, 128.2, 127.1, 121.7, 121.5, 121.3, 62.9, 57.5, 52.7, 52.4, 44.3, 28.8, 13.7 ppm; IR (KBr) ν = 2931, 2807, 1578, 1227 cm−1; MS (70 eV) m/z 410 [M+], 264, 202, 175, 146; anal. calcd for C24H34N4S: C, 70.20; H, 8.35; N, 13.64; S, 7.81. Found: C, 70.41; H, 8.57; N, 13.49; S, 7.95.
2-(4-Butylpiperazin-1-yl)ethyl (Z)-N,N-dibutyl-N′-phenylcarbamimidothioate 3f
1H NMR (300 MHz, CDCl3) δ 7.22–7.17 (m, 2H), 6.91–6.87 (m, 3H), 3.48 (t, J = 7.5 Hz, 4H), 2.46–2.41 (m, 4H), 2.36–2.35 (m, 10H), 1.62–1.52 (m, 4H), 1.48–1.36 (m, 2H), 1.34–1.22 (m, 6H), 0.92 (t, J = 7.5 Hz, 3H), 0.89 (t, J = 7.0 Hz, 6H) ppm; 13C NMR (75 MHz, CDCl3) δ 153.1, 150.0, 128.5, 121.5, 121.2, 58.3, 57.4, 53.0, 52.4, 49.7, 30.5, 28.8, 28.7, 20.6, 19.9, 13.9, 13.8 ppm; IR (KBr) ν = 2930, 1578, 1376, 1161 cm−1; MS (70 eV) m/z 432 [M+], 261, 207, 168, 125; anal. calcd for C25H44N4S: C, 69.39; H, 10.25; N, 12.95; S, 7.41. Found: C, 69.19; H, 10.41; N, 13.08; S, 7.57.
2-(4-Benzylpiperazin-1-yl)ethyl (Z)-N,N-dibutyl-N′-phenylcarbamimidothioate 3g
1H NMR (300 MHz, CDCl3) δ 7.34–7.30 (m, 5H), 7.25–7.19 (m, 3H), 6.95–6.89 (m, 3H), 3.52 (s, 2H), 3.51 (t, J = 7.5 Hz, 3H), 2.53–2.45 (m, 2H), 2.45–2.35 (m, 10H), 1.60 (t, t, J = 7.5, 7.5 Hz, 4H), 1.32 (t, q, J = 7.0, 7.5 Hz, 4H), 0.95 (t, J = 7.0 Hz, 6H) ppm; 13C NMR (75 MHz, CDCl3) δ 153.3, 150.1, 134.0, 129.3, 128.6, 128.2, 127.1, 121.6, 121.4, 62.9, 57.5, 52.7, 52.4, 49.9, 30.7, 20.1, 14.0 ppm; IR (KBr) ν = 2941, 2808, 1578, 1159 cm−1; MS (70 eV) m/z 466 [M+], 320, 265, 202, 146; anal. calcd for C28H42N4S: C, 72.06; H, 9.07; N, 12.00; S, 6.87. Found: C, 71.90; H, 9.15; N, 12.11; S, 6.97.
2-(4-Butylpiperazin-1-yl)ethyl (Z)-N-phenylmorpholine-4-carbimidothioate 3h
1H NMR (300 MHz, CDCl3) δ 7.21 (d, t, J = 7.5, 2.0 Hz, 2H), 6.94 (t, t, J = 7.5, 2.0 Hz, 1H), 6.87–6.84 (m, 2H), 3.69 (t, J = 4.5 Hz, 4H), 3.59 (t, J = 4.5 Hz, 4H), 2.53–2.47 (m, 4H), 2.38–2.34 (m, 6H), 2.28–2.24 (m, 4H), 1.46–136 (m, 2H), 1.27 (t, q, J = 7.0, 7.5 Hz, 2H), 0.87 (t, J = 7.0 Hz, 3H) ppm; 13C NMR (75 MHz, CDCl3) δ 154.9, 149.2, 128.4, 121.3, 121.0, 66.4, 58.2, 57.6, 52.8, 52.4, 48.5, 28.9, 28.7, 20.5, 13.9 ppm; IR (KBr) ν = 2955, 2807, 1582, 1158 cm−1; MS (70 eV) m/z 390 [M+], 336, 281, 168, 125; anal. calcd for C21H34N4OS: C, 64.58; H, 8.77; N, 14.34; S, 8.21. Found: C, 64.62; H, 8.91; N, 14.27; S, 8.40.
2-(4-Isobutylpiperazin-1-yl)ethyl (Z)-N-phenylmorpholine-4-carbimidothioate 3i
1H NMR (300 MHz, CDCl3) δ 7.24 (d, t, 7.5, 1.5 Hz, 2H), 7.25 (d, t, 7.5, 1.5 Hz, 1H), 6.81–6.88 (m, 2H), 3.71 (t, 4.5Hz, 4H), 3.63 (t, 4.5Hz, 4H), 2.56–2.51 (m, 2H), 2.52–2.34 (m, 10H), 2.05 (d, J = 7.0 Hz, 2H), 1.77 (t, septet, J = 7.0, 6.5 Hz, 1H), 0.88 (d, J = 6.5 Hz, 6H) ppm; 13C NMR (75 MHz, CDCl3) δ 154.8, 149.2, 128.3, 121.9, 121.0, 66.5, 66.3, 57.6, 53.0, 52.5, 48.4, 28.9, 25.0, 20.6 ppm; IR (KBr) ν = 2953, 2807, 1583, 1159 cm−1; MS (70 eV) m/z 390 [M+], 249, 189, 168, 125; anal. calcd for C21H34N4OS: C, 67.58; H, 8.77; N, 14.34; S, 8.21. Found: C, 67.65; H, 8.89; N, 14.47; S, 8.39.
2-(4-Benzylpiperazin-1-yl)ethyl (Z)-N-phenylmorpholine-4-carbimidothioate 3j
1H NMR (300 MHz, CDCl3) δ 7.35–7.31 (m, 5H), 7.29–7.24 (m, 2H), 7.02 (dd, J = 7.0, 1.5 Hz, 1H), 6.92–6.90 (m, 2H), 3.76 (t, J = 4.5 Hz, 4H), 3.65 (t, J = 4.5 Hz, 4H), 3.53 (s, 2H), 2.59–2.54 (m, 2H), 2.49–2.40 (m, 10H) ppm; 13C NMR (75 MHz, CDCl3) δ 155.2, 149.5, 129.3, 128.7, 128.2, 127.1, 122.3, 121.5, 121.3, 66.7, 62.9, 57.8, 52.7, 52.5, 48.7, 29.1 ppm; IR (KBr) ν = 2934, 2807, 1581, 1156 cm−1; MS (70 eV) m/z 424 [M+], 278, 202, 146; anal. calcd for C24H32N4OS: C, 67.89; H, 7.60; N, 13.20; S, 7.55. Found: C, 67.70; H, 7.81; N, 13.32; S, 7.74.
Conclusions
In summary, we developed a method for the synthesis of a novel series of isothiourea-ethylene-tethered piperazine derivatives. The process can be performed in the same vessel using all the four required reactants in a one-pot manner. The operation is convenient, the process is multicomponent and atom economy, no expensive reagent is required, and each reaction gives a sole product in good yields. The products are expected to exhibit biological properties and would be assessed accordingly in due course.
Author contributions
Conceptualization, study design, data validation, supervision, project administration, and funding acquisition: Mohammed M. Mojtahedi; methodology; investigation, resources, software, formal analysis, and data curation: Fatima Hajizadeh; writing, original draft preparation, review and editing: M. Saeed Abaee. All authors have read and agreed to the published version of the manuscript.
Conflicts of interest
There are no conflicts to declare.
Acknowledgements
We would like to thank CCERCI for financial support of this work (grant number 1402-102).
Notes and references
- J. Zhu, Q. Wang and M. Wang, Multicomponent Reactions in Organic Synthesis, Wiley-VCH, Weinheim, 2014 Search PubMed.
- U. K. Sharma, P. Ranjan, E. V. Van der Eycken and S.-L. You, Chem. Soc. Rev., 2020, 49, 8721–8748 RSC.
- R. C. Cioc, E. Ruijter and R. V. A. Orru, Green Chem., 2014, 16, 2958–2975 RSC.
- A. F. de la Torre, A. Ali, B. Westermann, G. Schmeda-Hirschmann and M. W. Pertino, New J. Chem., 2018, 42, 19591–19599 RSC.
- B. B. Touré and D. G. Hall, Chem. Rev., 2009, 109, 4439–4486 CrossRef PubMed.
- R. Javahershenas and S. Nikzat, RSC Adv., 2023, 13, 16619–16629 RSC.
- S. Zhi, X. Ma and W. Zhang, Org. Biomol. Chem., 2019, 17, 7632–7650 RSC.
- A. R. Sheikh, A. Arif and M. M. Khan, RSC Adv., 2023, 13, 11652–11684 RSC.
- A. F. de la Torre, A. Ali, O. Concepcion, A. L. Montero-Alejo, F. M. Muñiz, C. A. Jiménez, J. Belmar, J. L. Velázquez–Libera, E. W. Hernández–Rodríguez and J. Caballero, New J. Chem., 2019, 43, 12804–12813 RSC.
- S. M. Hoy, Drugs, 2016, 76, 509–516 CrossRef CAS PubMed.
- F. A. M. Al-Omary, N. Alvarez, L. S. Al-Rasheed, N. Veiga, H. M. Hassan and A. A. El-Emam, ACS Omega, 2023, 8, 13465–13477 CrossRef CAS PubMed.
- G. L. Perlovich, A. N. Proshin, T. V. Volkova, S. V. Kurkov, V. V. Grigoriev, L. N. Petrova and S. O. Bachurin, J. Med. Chem., 2009, 52, 1845–1852 CrossRef CAS PubMed.
- H. Yoneyama, T. Magata, K. Uemura, Y. Usami, S. Tanaka, M. Takaoka and S. Harusawa, Synthesis, 2015, 47, 1291–1302 CrossRef CAS.
- W. T. Lambert, M. E. Goldsmith and T. C. Sparks, Pest Manage. Sci., 2017, 73, 743–751 CrossRef CAS PubMed.
- A. Pascual and A. Rindlisbacher, Pestic. Sci., 1994, 42, 253–263 CrossRef CAS.
- Y. Wang, C. M. Young, D. B. Cordes, A. M. Z. Slawin and A. D. Smith, J. Org. Chem., 2023, 88, 7784–7799 CrossRef CAS PubMed.
- K. Srinivas, P. Srinivas, P. S. Prathima, K. Balaswamy, B. Sridhar and M. M. Rao, Catal. Sci. Technol., 2012, 2, 1180–1187 RSC.
- T. Watanabe, J. Pisano, C. Mangione and J. S. Madalengoitia, J. Org. Chem., 2023, 88, 2851–2868 CrossRef CAS PubMed.
- N. Dawra and R. N. Ram, Synthesis, 2016, 48, 4199–4206 CrossRef CAS.
- T. Horinouchi, H. Nakagawa, T. Suzuki, K. Fukuhara and N. Miyata, Bioorg. Med. Chem. Lett., 2011, 21, 2000–2002 CrossRef CAS PubMed.
- X. Lei, Y. Wang, E. Fan and Z. Sun, Org. Lett., 2019, 21, 1484–1487 CrossRef CAS PubMed.
- S. Abou-Shehada, P. Mampuys, B. Maes, J. Clark and L. Summerton, Green Chem., 2017, 19, 249–258 RSC.
- S. Wu, X. Lei, E. Fan and Z. Sun, Org. Lett., 2018, 20, 522–525 CrossRef CAS PubMed.
- D. Mishra and P. Phukan, J. Org. Chem., 2021, 86, 17581–17593 CrossRef CAS PubMed.
- K. Bano, J. Sharma, A. Jain, H. Tsurugi and T. K. Panda, RSC Adv., 2023, 13, 3020–3032 RSC.
- M. M. Mojtahedi, K. Darvishi, M. S. Abaee, M. R. Halvagar and M. Ghasemzadeh, Tetrahedron Lett., 2022, 98, 153810 CrossRef CAS.
- H. Mollabagher, S. Taheri, M. M. Mojtahedi and S. Seyedmousavi, RSC Adv., 2020, 10, 1995–2003 RSC.
- L. Pourabdi, T. T. Küçükkılınç, F. Khoshtale, B. Ayazgök, H. Nadri, F. F. Alashti, H. Forootanfar, T. Akbari, M. Shafiei, A. Foroumadi, M. Sharifzadeh, M. S. Ardestani, M. S. Abaee, L. Firoozpour, M. Khoobi and M. M. Mojtahedi, Front. Chem., 2021, 9, 810233 CrossRef CAS PubMed.
- S. Bagherpour, M. M. Mojtahedi and M. S. Abaee, J. Sulfur Chem., 2020, 41, 301–309 CrossRef CAS.
- A. Z. Halimehjani and E. Badali, RSC Adv., 2019, 9, 36386–36409 RSC.
- F. J. Asar, F. Soleymani, S. E. Hooshmand and A. Z. Halimehjani, Tetrahedron Lett., 2020, 61, 152610 CrossRef CAS.
- Q. Zhu, Q. Yuan, M. Chen, M. Guo and H. Huang, Angew. Chem., Int. Ed., 2017, 56, 5101–5105 CrossRef CAS PubMed.
- D. Leusser, J. Henn, N. Kocher, B. Engels and D. Stalke, J. Am. Chem. Soc., 2004, 126, 1781–1793 CrossRef CAS PubMed.
|
This journal is © The Royal Society of Chemistry 2023 |
Click here to see how this site uses Cookies. View our privacy policy here.