DOI:
10.1039/D3RA06737H
(Paper)
RSC Adv., 2023,
13, 31346-31352
Visible-light-mediated synthesis of oxime esters via multicomponent reactions of aldehydes, aryl amines, and N-hydroxyphthalimide esters†
Received
4th October 2023
, Accepted 21st October 2023
First published on 26th October 2023
Abstract
Oxime esters are useful scaffolds in many organic chemistry transformations. Herein, a novel visible-light-mediated three-component reaction for synthesis of oxime esters is reported. Aldehydes, aniline, and N-hydroxyphthalimide (NHPI) esters were used as substrates in this three-component reaction, and eosin Y was used as a crucial photocatalyst for the reaction. Wide ranges of aldehydes and NHPI esters were well tolerated in this reaction method, generating various oxime esters with high efficiency under mild reaction conditions. This visible-light-mediated methodology will be a promising approach to synthesize useful oxime esters in a single step.
Introduction
Oxime esters have been found in various areas. For example, a wide range of biologically active compounds with anticancer,1 anti-inflammatory,2 antifungal,3 antioxidant and antimicrobial4 activities contain oxime ester moieties. Additionally, oxime esters are an important scaffold for many organic synthesis processes such as C–C bond cleavage,5 cyanoalkylation,6 hydroesterification,7 cross coupling,8 sulfonylamination,9 and cyclization.10 Recently, oxime esters were used as photoinitiators in photoreactions for various transformations.11 Due to their utility, several methods have been reported for the synthesis of oxime esters (Scheme 1). Oxime esters can be prepared using two-step procedures: the formation of aldoximes and ketoximes via reactions of aldehydes or ketones with NH2OH, followed by esterification of oximes with carboxylic acids,12 aldehydes,13 or esters.14
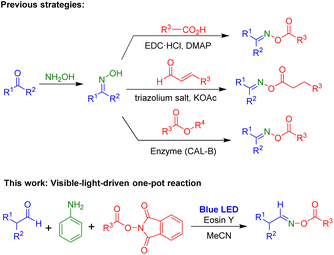 |
| Scheme 1 Synthetic procedures affording oxime esters. | |
Light has been employed for numerous chemical transformations.15 Photochemical reactions are initiated by the absorption of light as the source of energy, and they have become useful methods with many applications in organic chemistry and materials.16 Additionally, photochemical reactions are usually carried out at room temperature and can tolerate a wide range of transformations.17
N-Hydroxyphthalimide (NHPI) esters also are useful substrates in a variety of reactions because they can be prepared with ease and at low cost from carboxylic acids and N-hydroxyphthalimides. NHPI esters have been used in many reactions for the formation of C–C bonds.18 Reactions of NHPI esters to generate C–B and C–Si bonds have been developed.19 Various photoredox reactions employing NHPI esters also have been reported. These reactions included formation of C–C bonds such as alkylation of heterocycles20 and alkenylation,21 formation of C–O and C–S bonds,22 and remote C–H oxidation.23 Additionally, NHPI esters were successfully employed in annulation reactions under visible-light irradiation to produce saturated heterocycles.24
We were interested in developing practical and useful synthetic methods for oxime ester compounds. Specifically, utilization of a visible-light-mediated reaction to yield oxime esters can be a promising strategy. Herein, we describe a visible-light-induced one-pot reaction via three-component reaction of aldehyde, aniline, and NHPI esters for the preparation of oxime ester compounds (Scheme 1).
Results and discussion
One-pot reactions are useful approaches to produce organic compounds with many advantages including minimal waste and reduced cost and effort.25 Thus, we tried to use multicomponent reactions for the preparation of oxime esters. For the present study, we assumed that reaction of aldehydes with amines could also produce the radical acceptors imines, and, under irradiation by visible light, NHPI esters could be converted into carboxylic radicals. Then, target oxime esters could be formed through reaction between imines and carboxylic radicals.
To assess our idea, novel visible-light-induced multicomponent reactions using aldehydes, aryl amine, and NHPI ester in the presence of a photocatalyst were examined. In the initial experiments, benzaldehyde, aniline, and 1,3-dioxoisoindolin-2-yl butyrate (NHPI ester of butyric acid) were selected as the model substrates, and eosin Y was employed as a photocatalyst for optimization of the reaction condition (Table 1) because eosin Y has been used for visible-light induced reactions.26 The reaction using eosin Y in MeCN was conducted under irradiation of blue LEDs at room temperature. Under standard conditions, (E)-benzaldehyde O-butyryl oxime, the target product, was obtained in 92% yield in 16 hours (Table 1, entry 1). The other photocatalysts were tested (Table 1, entries 2–8). The reaction using Ru(bpy)3Cl2·6H2O did not produce the desired product (Table 1, entry 2), and the use of 2,2-bipyridine or benzophenone resulted in preparation of the target product in less than 30% yield (Table 1, entries 3 and 4). Photocatalysts 5,5-dimethyl-2,2-bipyridine, salicylaldehyde, sodium anthraquinone-2-sulfonate (SAQS), and eosin B afforded the corresponding products in yields ranging from 64% to 90% (Table 1, entries 5–8).
Table 1 Screening of reaction conditions for synthesis of ethyl (E)-2-phenyldiazene-1-carboxylatea
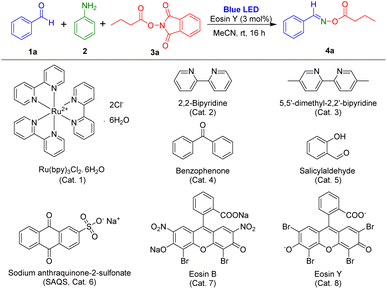
|
Entry |
Deviation from the standard conditions |
Yieldb (%) |
Reaction conditions: aldehyde 1a (1.0 mmol), aniline 2 (1.0 mmol), N-hydroxyphthalimide ester (NHPI ester) 3a (1.2 mmol), photocatalyst (0.03 mmol), solvent (2 mL), room temperature, blue LEDs (5 W × 2 bulbs), 16 h. Isolated yield after purification by flash column chromatography. No reaction. |
1 |
Standard conditions, blue LEDs |
92 |
2 |
Cat. 1 instead of Cat. 8 |
NRc |
3 |
Cat. 2 instead of Cat. 8 |
27 |
4 |
Cat. 3 instead of Cat. 8 |
28 |
5 |
Cat. 4 instead of Cat. 8 |
64 |
6 |
Cat. 5 instead of Cat. 8 |
76 |
7 |
Cat. 6 instead of Cat. 8 |
77 |
8 |
Cat. 7 instead of Cat. 8 |
90 |
9 |
CH2Cl2 instead of MeCN |
15 |
10 |
DCE instead of MeCN |
16 |
11 |
Toluene instead of MeCN |
18 |
12 |
THF instead of MeCN |
58 |
13 |
DMF instead of MeCN |
79 |
14 |
1,4-Dioxane instead of MeCN |
81 |
15 |
Ethyl acetate instead of MeCN |
85 |
16 |
Benzylamine instead of 2 |
3 |
17 |
Propylamine instead of 2 |
Trace |
18 |
tert-Butylamine instead of 2 |
NR |
19 |
No photocatalyst |
NR |
20 |
No light |
14 |
21 |
No photocatalyst, no light |
NR |
Several solvents including CH2Cl2, DCE, and toluene were also tested in photoreactions, but the reactions in these solvents produced oxime ester in less than 20% yield (Table 1, entries 9–11). Other solvents of THF, DMF, 1,4-dioxane, and ethyl acetate provided improved reaction efficiencies (Table 1, entries 12–15). However, MeCN was the most suitable solvent for this multi-component reaction. In addition, photoreactions using other nitrogen sources were also conducted. However, these reactions were not successful (Table 1, entries 16–18). Notably, using these basic aliphatic amines led to the hydrolysis of NHPI ester substrates and benzaldehyde substrate remained unreacted, indicating the vital role of aniline in the reaction. The influences of catalyst and light on the reaction were examined. The desired product was not prepared and only imine intermediate was generated in the reaction in the absence of photocatalyst (Table 1, entries 19 and 21). Reaction in the absence of light was performed, and less than 15% of product was obtained (Table 1, entry 20).
Next, several different light types were tested to examine their influence on the reaction (Table S1†). Irradiation with white LEDs, green LEDs, and compact fluorescent lights (CFLs) led to generation of products with yields of 71%, 87%, and 88%, respectively. However, no other additional improvements of reaction were observed compared to the reaction using blue LEDs. Different amounts of photocatalyst were investigated to determine optimal reaction conditions (Table S2†). Use of 1 mol% of eosin Y as a catalyst yielded the desired oxime ester in 76% yield, and a reaction using 3 mol% of eosin Y boosted the yield to 92%. However, greater than 3 mol% of eosin Y did not show an additional increase. Amounts of 1,3-dioxoisoindolin-2-yl butyrate in the reaction were also examined, and reaction using 1.2 equiv. of 1,3-dioxoisoindolin-2-yl butyrate afforded the target product in high yield (Table S3†).
Based on the optimized conditions, we explored the scope of the visible-light-induced reaction. First, various aldehydes were reacted with aniline and 1,3-dioxoisoindolin-2-yl butyrate (Scheme 2). Aryl aldehydes containing electron-donating (methyl) or electron-withdrawing substituents (chloro and nitro) in the para-position on the phenyl ring produced the desired products 4a–4d in high yields (89–93%). Reaction of aryl aldehydes with electron-donating or electron-withdrawing groups at the ortho-position of the aromatic ring also yielded the corresponding products 4e–4g at 83–91%. Notably, reaction of heterocyclic aromatic substrates 2-pyridinecarboxaldehyde and 2-thiophenecarboxaldehyde provided the target oxime esters (4i and 4j) in yields of 89% and 84%, respectively. Additionally, several alkyl aldehydes were tested for this reaction protocol, and they were tolerated in the reaction to afford the target oxime esters (4k–4n) in high yields.
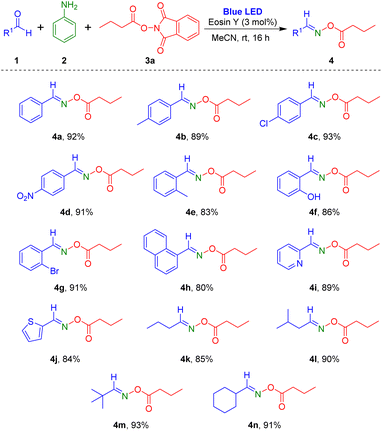 |
| Scheme 2 Substrate scope of aldehydes. aReaction conditions: aldehyde 1 (1.0 mmol), aniline 2 (1.0 mmol), N-hydroxyphthalimide ester (NHPI ester) 3a (1.2 mmol), eosin Y (0.03 mmol), MeCN (2 mL), blue LEDs (5 W × 2 bulbs), 16 h. bIsolated yield after purification by flash column chromatography. | |
Subsequently, reactions of a variety of NHPI esters with benzaldehyde and aniline were carried out (Scheme 3). Aromatic carboxylic acid-derived NHPI esters with electron-donating (methyl and methoxy) or electron-withdrawing substituents (chloro, bromo, trifluoromethyl, and nitro) readily produced the desired oxime esters (5a–5h). Particularly, reaction of NHPI ester of 2,4,6-trimethylbenzoic acid, which has steric hindrance, gave the target product 5d in 90% yield. Using the process, NHPI esters of the two aromatic carboxylic acids 1-naphthoic acid and biphenyl-4-carboxylic acid were also transformed to the corresponding oxime ester products (5i and 5j). Additionally, various alkyl carboxylic acid derived NHPI esters were employed in the reaction, and they were tolerated in this reaction method to give the desired products (5k–5n) in high yields. Notably, the reaction of NHPI esters prepared from undecanoic acid, which contains a long carbon chain, generated the target oxime ester compound 5m in 92% yield.
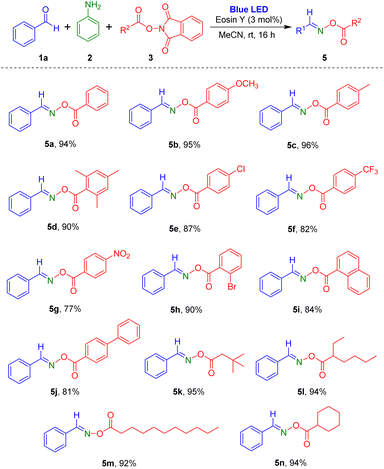 |
| Scheme 3 Substrate scope of NHPI esters. aReaction conditions: aldehyde 1a (1.0 mmol), aniline 2 (1.0 mmol), N-hydroxyphthalimide ester (NHPI ester) 3 (1.2 mmol), eosin Y (0.03 mmol), MeCN (2 mL), blue LEDs (5 W × 2 bulbs), 16 h. bIsolated yield after purification by flash column chromatography. | |
Next, gram-scale synthesis of oxime esters from aldehyde, aniline, and 1,3-dioxoisoindolin-2-yl butyrate was performed to evaluate the visible-light-mediated multi-component reaction (Scheme 4). Benzaldehyde 1a (10.0 mmol, 1.07 g) readily reacted with aniline and 1,3-dioxoisoindolin-2-yl butyrate to generate the target oxime ester 4a in 83% yield.
 |
| Scheme 4 Gram-scale reaction of three components substrate scope of aldehydes. | |
Several control experiments were carried out to understand the reaction mechanism (Scheme 5). When 5.0 equiv. of TEMPO (2,2,6,6-tetramethyl-1-piperidinyloxy), a radical scavenger, was added to this reaction, formation of the target oxime ester was prevented, which suggested that the synthesis used a radical pathway. Reaction of imine and 1,3-dioxoisoindolin-2-yl butyrate in the presence of photocatalyst was performed under the irradiation of blue LEDs, and the target product was successfully obtained. Also, the reaction between aldehyde and aniline readily afforded the imine.
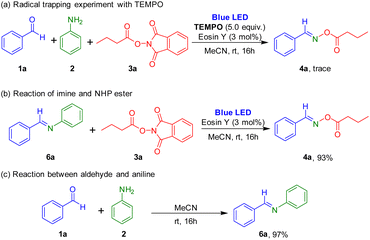 |
| Scheme 5 Control experiments. | |
Moreover, light on/off experiments were conducted to assess the effect of visible light on this synthesis (Fig. 1). Synthesis of oxime ester was remarkably slow in the dark, while irradiation by blue LEDs readily generated the desired product 4a from starting substrates. This suggests that irradiation of visible light played a crucial role in this reaction.
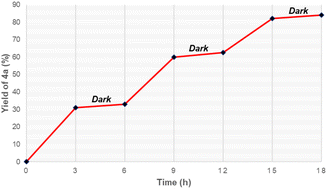 |
| Fig. 1 Light on/off experiments. | |
We proposed a plausible catalytic cycle mechanism for this process (Scheme 6) based on the results of control experiments and previously published reports.27 Photocatalyst eosin Y2− forms eosin Y2−* under irradiation of blue LEDs. NHPI ester 3a is transformed to radical anion A via reaction of eosin Y2−*. Then, radical anion A yields carboxylic radical B by release of a phthalimide anion. Carboxylic radical B reacts with imine C, which is prepared by treatment of aldehyde 1a and amine 2, to give C-centered radical intermediate D. Intermediate D provides the imine-like cation E via single electron transfer with eosin Y˙− while photocatalyst eosin Y2− is recovered to close the catalytic cycle. The desired product 4a is finally produced by the elimination of the phenyl group with phthalimide anion.
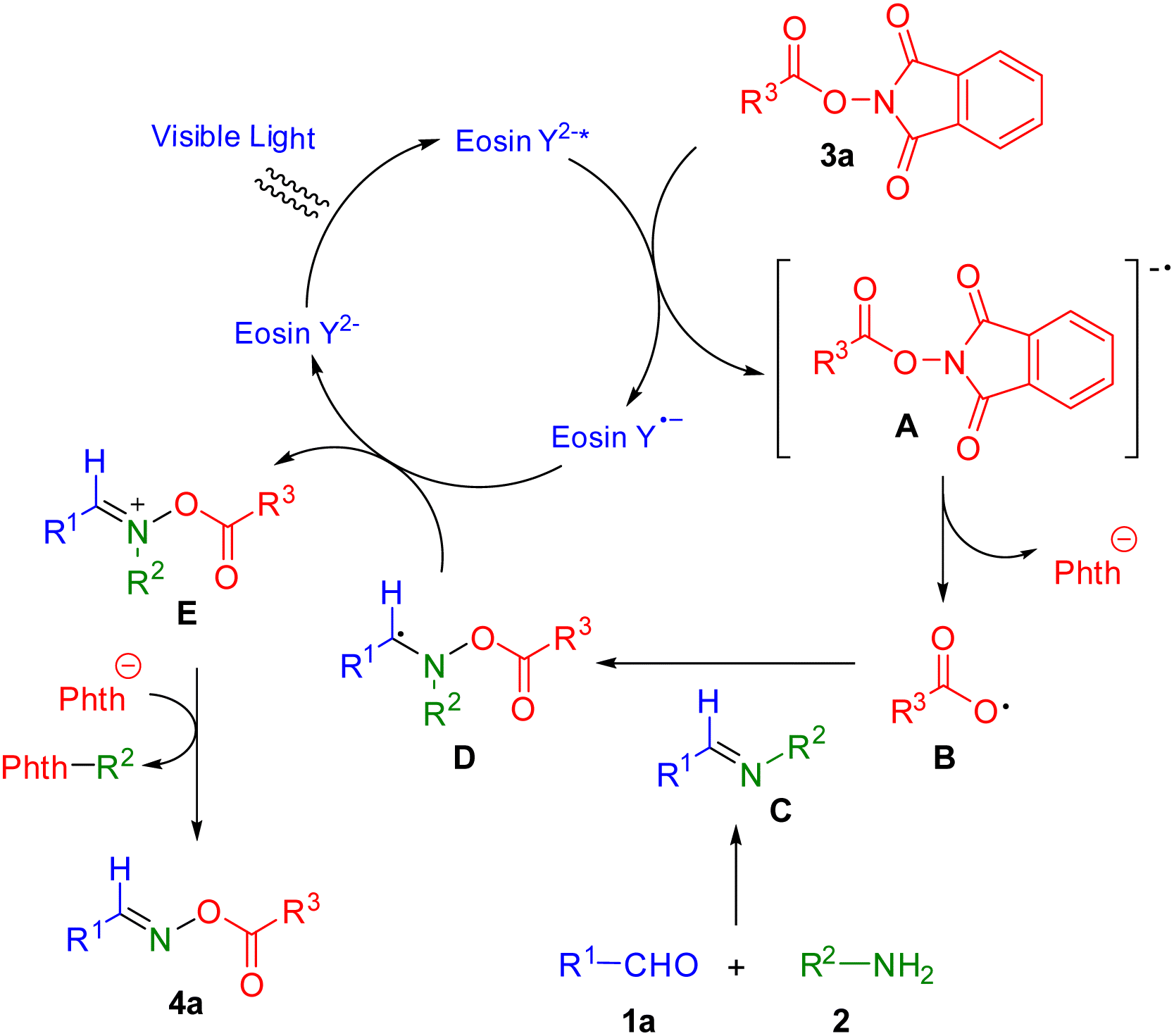 |
| Scheme 6 A plausible mechanism. | |
Experimental
General procedure of the synthesis of oxime esters (4a–4n, 5a–5n)
Benzaldehyde 1a (0.106 g, 1.0 mmol, 1.0 equiv.), aniline 2 (0.093 g, 1.0 mmol), 1,3-dioxoisoindolin-2-yl butyrate (NHPI ester) 3a (0.233 g, 1.2 mmol), and eosin Y (0.021 g, 0.03 mmol) were added to acetonitrile (2 mL). The reaction mixture was stirred under irradiation of 5 W blue LEDs (×2) at room temperature for 16 hours. The reaction product was extracted with EtOAc (50 mL) and washed with aqueous NaHCO3 (50 mL), followed by water (50 mL). The organic layer was dried with anhydrous sodium sulfate and was concentrated under reduced pressure. The residue was purified by flash column chromatography on silica gel eluting with hexane–EtOAc as eluent to afford the target product (4a) as a yellow oil (0.175 g, 92%).
Conclusions
In summary, efficient visible-light-mediated method for the preparation of oxime esters through one-pot multicomponent reaction employing aldehydes, aniline, and NHPI esters was described. Eosin Y was used as a suitable photocatalyst for the synthesis of oxime esters. A wide range of oxime esters bearing aliphatic and aromatic groups was successfully obtained by novel visible-light-mediated reaction under mild conditions. The method has advantages such as use of a wide scope of substrates, simple operation, and mild reaction conditions. Thus, this method allows easy and effective preparation of oxime esters in one step.
Author contributions
H.-K. Kim: conceptualization, supervision, data interpretation, writing – original draft, review, and editing. A. T. Nguyen: investigation, methodology, data curation, data interpretation, writing – original draft, and editing.
Conflicts of interest
There are no conflicts to declare.
Acknowledgements
This research was supported by the National Research Foundation of Korea (NRF) grant funded by the Korea government (MSIT) (NRF-2021R1A2C1011204).
Notes and references
-
(a) H.-P. Chen, Z.-Z. Zhao, Z.-H. Li, Z.-J. Dong, K. Wei, X. Bai, L. Zhang, C.-N. Wen, T. Feng and J.-K. Liu, Novel natural oximes and oxime esters with a vibralactone backbone from the basidiomycete Boreostereum vibrans, ChemistryOpen, 2016, 5, 142 CrossRef CAS PubMed
;
(b) B.-A. Song, X.-H. Liu, S. Yang, D.-Y. Hu, L.-H. Jin and H. Zhang, Synthesis and anticancer activity of 2,3,4-trimethoxyacetophenoxime ester containing benzothiazole moiety, Chin. J. Chem., 2005, 23, 1236–1240 CrossRef CAS
;
(c) A. Karakurt, İ. Bozbey, H. Uslu, S. Sari, Z. Özdemir and E. Şalva, Synthesis and cytotoxicity studies on new pyrazole-containing oxime ester derivatives, Trop. J. Pharm. Res., 2019, 18, 1315–1322 CrossRef CAS
;
(d) S.-R. Chena, F.-J. Shena, G.-L. Fenga and R.-X. Yuan, Synthesis and anticancer activity of 4-azasteroidal-20-oxime derivatives, J. Chem. Res., 2015, 39, 527–530 CrossRef
. -
(a) Q. Li, J. Zhang, L. Z. Chen, J. Q. Wang, H. P. Zhou, W. J. Tang, W. Xue and X. H. Liu, New pentadienone oxime ester derivatives: synthesis and anti-inflammatory activity, J. Enzyme Inhib. Med. Chem., 2018, 33, 130–138 CrossRef CAS PubMed
;
(b) E. Antoniadou-Vyza, N. Avramidis, A. Kourounakis and L. Hadjipetrou, Anti-inflammatory properties of new adamantane derivatives. Design, synthesis, and biological evaluation, Arch. Pharm., 1998, 331, 72–78 CrossRef CAS
. - Z. Fan, W. Duan, G. Lin, M. Chen and M. Huang, Synthesis and biological activities of novel (Z)-/(E)-anisaldehyde-based oxime ester compounds, Chem. Biodiversity, 2021, 18, e2100235 CrossRef CAS PubMed
. - S. T. Harini, H. V. Kumar, J. Rangaswamy and N. Naik, Synthesis, antioxidant and antimicrobial activity of novel vanillin derived piperidin-4-one oxime esters: preponderant role of the phenyl ester substituents on the piperidin-4-one oxime core, Bioorg. Med. Chem. Lett., 2012, 22, 7588–7592 CrossRef CAS PubMed
. -
(a) Y. He, J. Lou, K. Wu, H. Wang and Z. Yu, Copper-catalyzed radical C–C bond cleavage and [4+1] annulation cascade of cycloketone oxime esters with enaminothiones, J. Org. Chem., 2019, 84, 2178–2190 CrossRef CAS PubMed
;
(b) W. Zhou, Y. Long, H. Xiang, B. Xu and X. Zhou, Iron-catalyzed C–C bond cleavage of oximes for direct coupling of benzothiazole in water, J. Org. Chem., 2023, 88, 4875–4879 CrossRef CAS PubMed
. -
(a) L. Yang, P. Gao, X.-H. Duan, Y.-R. Gu and L.-N. Guo, Direct C–H cyanoalkylation of quinoxalin-2(1H)-ones via radical C–C bond cleavage, Org. Lett., 2018, 20, 1034–1037 CrossRef CAS PubMed
;
(b) W. Zhang, Y.-L. Pan, C. Yang, L. Chen, X. Li and J.-P. Cheng, Metal-free direct C–H cyanoalkylation of quinoxalin-2(1H)-ones by organic photoredox catalysis, J. Org. Chem., 2019, 84, 7786–7795 CrossRef CAS PubMed
. - T. Sang, C. Zhou, J. Li, X. Liu, Y. Yuan, X. Bao, X. Zhao and C. Huo, Photosensitized anti-Markovnikov hydroesterification of unactivated alkenes, Org. Lett., 2023, 25, 4371–4376 CrossRef CAS PubMed
. - G.-Q. Li, F.-R. Meng, W.-J. Xiao and J.-R. Chen, Photoinduced copper-catalyzed asymmetric radical three-component cross-coupling of 1,3-enynes with oxime esters and carboxylic acids, Org. Chem. Front., 2023, 10, 2773–2781 RSC
. - C.-P. Yuan, Y. Zheng, Z.-Z. Xie, K.-Y. Deng, H.-B. Chen, H.-Y. Xiang, K. Chen and H. Yang, Photosensitized vicinal sulfonylamination of alkenes with oxime ester and DABCO·(SO2)2, Org. Lett., 2023, 25, 1782–1786 CrossRef CAS PubMed
. - S.-C. Wang, Y.-T. Shen, T.-S. Zhang, W.-J. Hao, S.-J. Tu and B. Jiang, Cyclic oxime esters as deconstructive bifunctional reagents for cyanoalkyl esterification of 1,6-enynes, J. Org. Chem., 2021, 86, 15488–15497 CrossRef CAS PubMed
. - F. Hammoud, A. Hijazi, M. Schmitt, F. Dumur and J. Lalevée, A review on recently proposed oxime ester photoinitiators, Eur. Polym. J., 2023, 188, 111901 CrossRef CAS
. -
(a) C. S. Kumar, N. V. Kumar, P. Srinivas and B. K. Bettadaiah, A convenient practical synthesis of alkyl and aryl oxime esters, Synthesis, 2014, 46, 1847–1852 CrossRef
;
(b) T. Patra, M. Das, C. G. Daniliuc and F. Glorius, Nat. Catal., 2021, 4, 54–61 CrossRef CAS
;
(c) W. J. Lee, H. S. Kwak, D. Lee, C. Oh, E. K. Yum, Y. An, M. D. Halls and C.-W. Lee, Design and synthesis of novel oxime ester photoinitiators augmented by automated machine learning, Chem. Mater., 2022, 34, 116–127 CrossRef CAS
. - D. Enders, A. Grossmann and D. V. Craen, N-Heterocyclic carbene catalyzed synthesis of oxime esters, Org. Biomol. Chem., 2013, 11, 138–141 RSC
. - S. Lal and T. J. Snape, Exploitation of a Candida antarctica lipase B-catalysed in situ carboxylic acid activation method for the synthesis of acetanilides, J. Mol. Catal. B: Enzym., 2012, 83, 80–86 CrossRef CAS
. -
(a) D. V. Patil, Y. T. Hong, H. Y. Kim and K. Oh, Visible-light-induced three-component selenofunctionalization of alkenes: an aerobic selenol oxidation approach, Org. Lett., 2022, 24, 8465–8469 CrossRef CAS PubMed
;
(b) T. G. Luu, Y. Jung and H. K. Kim, Visible-light-induced catalytic selective halogenation with photocatalyst, Molecules, 2021, 26, 7380 CrossRef CAS PubMed
;
(c) T. G. Luu and H. K. Kim, Visible-light-driven copper-catalyzed synthesis of sulfonamides from Aryl-N2BF4 through one-pot tricomponent reaction, Adv. Synth. Catal., 2023, 365, 1671–1677 CrossRef
;
(d) J. Singh, R. I. Patel and A. Sharma, Visible-light-mediated C-2 functionalization and deoxygenative strategies in heterocyclic N-oxides, Adv. Synth. Catal., 2022, 364, 2289–2306 CrossRef CAS
;
(e) T. T. Bui and H. K. Kim, Recent advances in photo-mediated radiofluorination, Chem.–Asian J., 2021, 16, 2155–2167 CrossRef CAS PubMed
;
(f) T. T. Bui, V. H. Tran and H. K. Kim, Adv. Synth. Catal., 2022, 364, 341–347 CrossRef CAS
. -
(a) G. S. Kumar and Q. Lin, Light-triggered click chemistry, Chem. Rev., 2021, 121, 6991–7031 CrossRef CAS PubMed
;
(b) K. Jung, N. Corrigan, M. Ciftci, J. Xu, S. E. Seo, C. J. Hawker and C. Boyer, Designing with light: advanced 2D, 3D, and 4D materials, Adv. Mater., 2020, 32, 1903850 CrossRef CAS PubMed
;
(c) S. R. Atriardi, J.-Y. Kim, Y. Anita and S. K. Woo, Synthesis of gem-difluoroalkenes via photoredox-catalyzed defluoroaryloxymethylation of α-trifluoromethyl alkenes, Bull. Korean Chem. Soc., 2023, 44, 50–54 CrossRef CAS
;
(d) C. Brieke, F. Rohrbach, A. Gottschalk, G. Mayer and A. Heckel, Light-controlled tools, Angew. Chem., Int. Ed., 2012, 51, 8446–8476 CrossRef CAS PubMed
;
(e) T. G. Luu and H. K. Kim, Visible-light-driven sulfonamidation of arylazo sulfonesthrough one-pot multicomponent reaction, Asian J. Org. Chem., 2023, 12, e202300301 CrossRef CAS
;
(f) S. D. Tambe and E. J. Cho, Organophotocatalytic oxidation of alcohols to carboxylic acids, Bull. Korean Chem. Soc., 2022, 43, 1226–1230 CrossRef CAS
;
(g) T. Luo, Y.-F. Guo, T.-T. Xu and H. Dong, Visible-light-promoted desulfurization to synthesize deoxyglycosides, J. Org. Chem., 2023, 88, 8024–8033 CrossRef CAS PubMed
;
(h) T. G. Luu and H. K. Kim, Visible-light-driven reactions for the synthesis of sulfur dioxide-inserted compounds: generation of S–F, S–O, and S–N bonds, RSC Adv., 2023, 13, 14412–14434 RSC
. -
(a) Y. Liu, T. Pang, W. Yao, F. Zhong and G. Wu, Visible-light-induced radical gem-iodoallylation of 2,2,2-trifluorodiazoethane, Org. Lett., 2023, 25, 1958–1962 CrossRef CAS PubMed
;
(b) S. P. Blum, K. Hofman, G. Manolikakes and S. R. Waldvogel, Advances in photochemical and electrochemical incorporation of sulfur dioxide for the synthesis of value-added compounds, Chem. Commun., 2021, 57, 8236–8249 RSC
;
(c) C. Park and S. Lee, One-pot sulfa-Michael addition reactions of disulfides using a pyridine-borane complex under blue light irradiation, Bull. Korean Chem. Soc., 2022, 43, 951–954 CrossRef CAS
;
(d) T. T. Bui, W. P. Hong and H. K. Kim, Recent advances in visible light-mediated fluorination, J. Fluorine Chem., 2021, 247, 109794 CrossRef CAS
;
(e) U. D. Newar, S. Borra and R. A. Maurya, Visible-light 2,4-dinitrophenol-mediated photoannulation of α-azidochalcones into 2,5-diaryloxazoles, Org. Lett., 2022, 24, 4454–4458 CrossRef CAS PubMed
;
(f) P.-C. Cui, Z.-C. Yin and G.-W. Wang, Visible-light-promoted C4-selective phosphorylation of pyridine and quinoline derivatives, Org. Lett., 2023, 25, 2663–2668 CrossRef CAS PubMed
;
(g) V. H. Tran and H. K. Kim, Visible-light-driven SAQS-catalyzed aerobic oxidative dehydrogenation of alkyl 2-phenylhydrazinecarboxylates, RSC Adv., 2022, 12, 30304–30309 RSC
;
(h) N. A. Romero and D. A. Nicewicz, Organic photoredox catalysis, Chem. Rev., 2016, 116, 10075–10166 CrossRef CAS PubMed
;
(i) J. Park and D. Y. Kim, Synthesis of selenated γ-lactones via photoredox-catalyzed selenylation and ring closure of alkenoic acids with diselenides, Bull. Korean Chem. Soc., 2022, 43, 941–945 CrossRef CAS
;
(j) T. G. Luu, T. T. Bui and H. K. Kim, Visible-light-induced one-pot synthesis of sulfonic esters via multicomponent reaction of arylazo sulfones and alcohols, RSC Adv., 2022, 12, 17499–17504 RSC
. -
(a) K. Kang and D. J. Weix, Nickel-catalyzed C(sp3)–C(sp3) cross-electrophile coupling of in situ generated NHP esters with unactivated alkyl bromides, Org. Lett., 2022, 24, 2853–2857 CrossRef CAS PubMed
;
(b) M. S. West, A. L. Gabbey, M. P. Huestis and S. A. L. Rousseaux, Ni-Catalyzed reductive cross-coupling of cyclopropylamines and other strained ring NHP esters with (hetero)aryl halides, Org. Lett., 2022, 24, 8441–8446 CrossRef CAS PubMed
;
(c) D. C. Salgueiro, B. K. Chi, I. A. Guzei, P. García-Reynaga and D. J. Weix, Control of redox-active ester reactivity enables a general cross-electrophile approach to access arylated strained rings, Angew. Chem., Int. Ed., 2022, 61, e202205673 CrossRef CAS PubMed
;
(d) F. Sandfort, M. J. O'Neill, J. Cornella, L. Wimmer and P. S. Baran, Alkyl−(hetero)aryl bond formation via decarboxylative cross-coupling: a systematic analysis, Angew. Chem., Int. Ed., 2017, 56, 3319–3323 CrossRef CAS PubMed
;
(e) J. Wang, T. Qin, T.-G. Chen, L. Wimmer, J. T. Edwards, J. Cornella, B. Vokits, S. A. Shaw and P. S. Baran, Nickel-catalyzed cross-coupling of redox-active esters with boronic acids, Angew. Chem., Int. Ed., 2016, 55, 9676–9679 CrossRef CAS PubMed
;
(f) J. T. Edwards, R. R. Merchant, K. S. McClymont, K. W. Knouse, T. Qin, L. R. Malins, B. Vokits, S. A. Shaw, D.-H. Bao, F.-L. Wei, T. Zhou, M. D. Eastgate and P. S. Baran, Decarboxylative alkenylation, Nature, 2017, 545, 213–218 CrossRef CAS PubMed
. -
(a) C. Li, J. Wang, L. M. Barton, S. Yu, M. Tian, D. S. Peters, M. Kumar, A. W. Yu, K. A. Johnson, A. K. Chatterjee, M. Yan and P. S. Baran, Decarboxylative borylation, Science, 2017, 356, eaam7355 CrossRef PubMed
;
(b) W.-M. Cheng, R. Shang, B. Zhao, W.-L. Xing and Y. Fu, Isonicotinate ester catalyzed decarboxylative borylation of (hetero)aryl and alkenyl carboxylic acids through n-hydroxyphthalimide esters, Org. Lett., 2017, 19, 4291–4294 CrossRef CAS PubMed
;
(c) W. Xue and M. Oestreich, Copper-catalyzed decarboxylative radical silylation of redox-active aliphatic carboxylic acid derivatives, Angew. Chem., Int. Ed., 2017, 56, 11649–11652 CrossRef CAS PubMed
. -
(a) L. M. Kammer, A. Rahman and T. Opatz, A visible light-driven Minisci-type reaction with n-hydroxyphthalimide esters, Molecules, 2018, 23, 764 CrossRef PubMed
;
(b) N. E. Behnke, Z. S. Sales, M. Li and A. T. Herrmann, Dual photoredox/nickel-promoted alkylation of heteroaryl halides with redox-active esters, J. Org. Chem., 2021, 86, 12945–12955 CrossRef CAS PubMed
;
(c) S. Das, S. K. Parida, T. Mandal, S. K. Hota, L. Roy, S. De Sarkar and S. Murarka, An organophotoredox-catalyzed redox-neutral cascade involving N-(acyloxy)phthalimides and maleimides, Org. Chem. Front., 2021, 8, 2256–2262 RSC
. - H.-Y. Wang, L.-J. Zhong, G.-F. Lv, Y. Li and J.-H. Li, Photocatalytic dual decarboxylative alkenylation mediated by triphenylphosphine and sodium iodide, Org. Biomol. Chem., 2020, 18, 5589 RSC
. -
(a) S. Shibutani, T. Kodo, M. Takeda, K. Nagao, N. Tokunaga, Y. Sasaki and H. Ohmiya, Organophotoredox-catalyzed decarboxylative C(sp3)–O bond formation, J. Am. Chem. Soc., 2020, 142, 1211–1216 CrossRef CAS PubMed
;
(b) Z. Xiao, L. Wang, J. Wei, C. Ran, S. H. Liang, J. Shang, G.-Y. Chen and C. Zheng, Visible-light induced decarboxylative coupling of redox-active esters with disulfides to construct C–S bonds, Chem. Commun., 2020, 56, 4164–4167 RSC
. - M. Luo, S. Zhu, C. Shi, Y. Du, C. Yang, L. Guo and W. Xia, Photoinduced remote C(sp3)–H cyanation and oxidation enabled by a vinyl radical-mediated 1,5-HAT strategy, Org. Lett., 2022, 24, 6560–6565 CrossRef CAS PubMed
. - P. R. D. Murray, I. N.-M. Leibler, S. M. Hell, E. Villalona, A. G. Doyle and R. R. Knowles, Radical redox annulations: a general light-driven method for the synthesis of saturated heterocycles, ACS Catal., 2022, 12, 13732–13740 CrossRef CAS PubMed
. - Y. Hayashi, Pot economy and one-pot synthesis, Chem. Sci., 2016, 7, 866–880 RSC
. -
(a) V. Srivastava, P. K. Singh and P. P. Singh, Recent advances of visible-light photocatalysis in the functionalization of organic compounds, J. Photochem. Photobiol., C, 2022, 50, 100488 CrossRef CAS
;
(b) T. Qin, J. Sun, F. Wang, J.-Y. Wang, H. Wang and M.-D. Zhou, Visible-light-induced C2 alkylation of heterocyclic N-oxides with N-hydroxyphthalimide esters under metal-free conditions, Adv. Synth. Catal., 2020, 362, 4707 CrossRef
;
(c) S. Soni, P. Pali, M. A. Ansari and M. S. Singh, Visible-light photocatalysis of eosin Y: HAT and complementing MS-CPET strategy to trifluoromethylation of β-Ketodithioesters with Langlois' reagent, J. Org. Chem., 2020, 85, 10098–10109 CrossRef CAS PubMed
. -
(a) Y. Cai, R. Zhang, D. Sun, S. Xu and Q. Zhou, Eosin Y-sensitized photocatalytic reaction of tertiary aliphatic amines with arenesulfonyl chlorides under visible-light irradiation, Synlett, 2017, 28, 1630–1635 CrossRef CAS
;
(b) S. M. Hell, C. F. Meyer, G. Laudadio, A. Misale, M. C. Willis, T. Noël, A. A. Trabanco and V. Gouverneur, Silyl Radical-mediated activation of sulfamoyl chlorides enables direct access to aliphatic sulfonamides from alkenes, J. Am. Chem. Soc., 2020, 142, 720–725 CrossRef CAS PubMed
.
|
This journal is © The Royal Society of Chemistry 2023 |
Click here to see how this site uses Cookies. View our privacy policy here.