DOI:
10.1039/D3SC01526B
(Edge Article)
Chem. Sci., 2023,
14, 7279-7284
Photocrystallography of [Ru(bpy)2(dmso)2]2+ reveals an O-bonded metastable state†
Received
23rd March 2023
, Accepted 6th June 2023
First published on 8th June 2023
Abstract
We report the first instance of observing the phototriggered isomerization of dmso ligands on a bis sulfoxide complex, [Ru(bpy)2(dmso)2], in the crystalline solid state. The solid-state UV-vis spectrum of the crystal demonstrates an increase in optical density around 550 nm after irradiation, which is consistent with the solution isomerization results. Digital images of the crystal before and after irradiation display a notable color change (pale orange to red) and cleavage occurs along planes, (
01) and (100), during irradiation. Single crystal X-ray diffraction data also confirms that isomerization is occurring throughout the lattice and a structure that contains a mix of the S,S and O,O/S,O isomer was attained from a crystal irradiated ex situ. In situ irradiation XRD studies reveal that the percentage of the O-bonded isomer increases as a function of 405 nm exposure time.
Introduction
Photocrystallography has emerged as an indispensable tool for the structural characterization of metastable states of photochromic compounds.1 Irradiation of single crystals of these compounds or complexes yields a mixture of ground state and metastable state isomers. While complete conversion of a single crystal can be achieved, it is much more common to observe partial conversion, and thus the isomerization is modelled as a disorder in the crystal. Success of this experiment requires that the solid-state photochemistry mimic the solution photochemistry. Moreover, crystal engineering to manage density, moduli, molecular structural changes, and heat dissipation is often required for photocrystallography to be successful. Indeed, irradiation of many crystalline materials can yield explosions (termed photosalient),2–4 dramatic cracking or cleaving of planes,5,6 and even disintegration of the crystal.7 In stark contrast, other crystalline samples simply change color upon irradiation without any significant or substantial photochemistry beyond the surface of the crystal, revealing a complicated relationship between crystal structure and molecular photochemistry. Nevertheless, photocrystallography has yielded the molecular structures of metastable states of a wide range of transition metal complexes with NO+,8–11 N2,12 SO2,13–15 NO2− (ref. 16–18) ligands as well as diarylethenes,19 fulgides, spiro-compounds, and has recently been employed to observe reaction intermediates in C–H bond activation.20–22 We have created a large family of photochromic ruthenium sulfoxides by conjoining the electrochemistry of [Ru(NH3)5(dmso)]2+ (dmso is dimethylsulfoxide) with the photochemistry of [Ru(bpy)3]2+ (bpy is 2,2′bipyridine).23–25 In 1982, Henry Taube first proposed an ECEC (Electrochemical Chemical–Electrochemical Chemical) mechanism to explain the peculiar cyclic voltammogram of [Ru(NH3)5(dmso)]2+, where oxidation of Ru2+ to produce Ru3+ triggers intramolecular S → O isomerization of the sulfoxide, and reduction of Ru3+ to yield Ru2+ prompts intramolecular O → S isomerization.26 While the ground state S-bonded isomer is well characterized by infrared spectroscopy and single crystal X-ray diffraction,27,28 evidence for the formation of the isomerization product was provided by comparison of the O-bonded Ru3+/2+ coupled with other O-bonded coordination complexes and from its reactivity with exogenous ligands. Despite claims of X-ray data of the O-bonded isomer in [Ru(NH3)5(dmso)]3+, the O-bonded structure produced from isomerization to our knowledge has not been reported.29,30
The MLCT (Metal-to-Ligand Charge-Transfer) transition in ruthenium polypyridine complexes yields an excited state best formulated as an oxidized ruthenium ion and a reduced bipyridine ligand (e.g., [Ru(bpy)2(bpy−)]2+).31 The phototriggered S → O isomerization in ruthenium polypyridine sulfoxides is activated by the formation of Ru3+ in the MLCT state of these heteroleptic complexes (Scheme 1). Characterization of the metastable state of these photochromic complexes has included 1H NMR spectroscopy,32 infrared spectroscopy,33,34 DFT calculations,35–41 and X-ray absorption spectroscopy.42 While these techniques reflect molecular structure, none of these data provide the molecular structure of the metastable state. While there are many structures of S-bonded or O-bonded sulfoxides, we do not know of any X-ray diffraction study that characterizes the conversion of an S-bonded sulfoxide to an O-bonded sulfoxide.43,44 Herein, we report the structure of the metastable state of a photochromic ruthenium sulfoxide complex and show that the structure is unequivocally an O-bonded sulfoxide (Scheme 1).
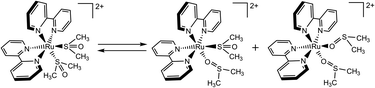 |
| Scheme 1 Proposed isomerization products of S,S-[Ru(bpy)2(dmso)2]2+ to yield a mixture of O,O bonded and S,O bonded isomers upon visible light irradiation. | |
Results and discussion
We chose to investigate [Ru(bpy)2(dmso)2][B(Ph)4]2 as the large size, flexibility, weak basicity, and lack of functional groups would increase the likelihood of observing isomerization of the dmso ligands in the single crystalline phase. As expected, the tetraphenylborate salt exhibits a limited number of short contacts to the dmso. For dmso1 (containing S1), and dmso2 (containing S2; Fig. 1, left), three short contacts to the anion were observed (contacts not shown); however, for dmso2 two of these contacts were to a symmetry related dmso2.
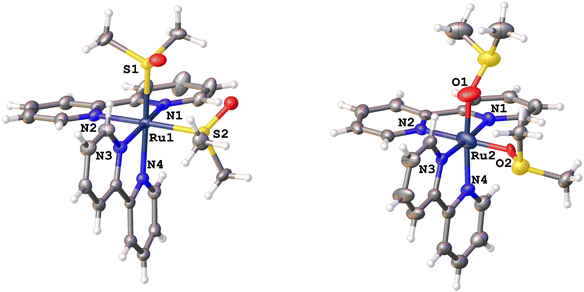 |
| Fig. 1 Ground state structure of bis-[Ru(bpy)2(dmso)2]2+(left). Isolated molecular structure of bis-[O,O]-[Ru(bpy)2(dmso)2]2+ after irradiation with 405 nm light (right). Ruthenium, navy; nitrogen, blue; oxygen, red; sulfur, yellow; carbon, grey; hydrogen, white. | |
Our previous investigations of bis sulfoxides have revealed that solvent and the nature of the chelate (if any) can be important in the quantum yield of isomerization and product distribution (one sulfoxide isomerization vs. two sulfoxide isomerizations) following visible light irradiation.22 These studies have demonstrated the presence of separate one isomerization (S,O-product isomer) and two isomerization (O,O-product isomer) reaction pathways. We are interested in capturing the mechanistic details of isomerization within a crystalline environment and correlating these structural changes with electronic spectroscopic results within photochromic crystals.
Previous reports establish that the S,S-, S,O- and O,O-isomers each feature unique absorption maxima in solution. Thus, irradiation of solutions of this complex at much lower concentrations than those present in a crystal show a distinct darkening of the solution from colorless or pale yellow to a deep burgundy or purple color.
The solid-state UV-visible spectra of the crystal during irradiation demonstrates similar features to that seen in the solution data (Fig. S1†). Prior to irradiation, the crystal of the S,S-isomer absorbs at ∼460 nm. As the crystal is irradiated with 405 nm light, a new broad absorption feature appears at ∼550 nm, which is indicative of the formation of the O,O-isomer. These changes in the optical density of the crystal are consistent with the solution isomerization results and are suggestive of isomerization. Upon careful examination of the crystals during irradiation, the crystal not only demonstrates dramatic changes in color (Fig. 2 and S2;† consistent with solution observations), but fracture lines along (
01) are also evident. The emergence of fracture planes in the crystal indicate strain in the lattice presumably caused by the stress associated with the proposed sulfoxide isomerization (deep coloration) in the crystal. This is supported by the fact that the dmso ligands align along (
01) (see Fig. S3†). If the crystal is irradiated with 405 nm light for a prolonged time (>30 minutes), delamination occurs at the surface seemingly parallel to (100) (Fig. S4†).
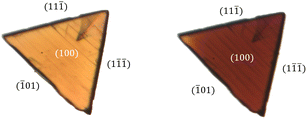 |
| Fig. 2 Digital images of the irradiated crystal before (left) and after 600 seconds of irradiation with 405 nm light (right). | |
Single-crystal X-ray diffraction data of [Ru(bpy)2(dmso)2][B(Ph)4]2 study corroborates that the dmso ligands isomerize within the lattice. Photodifference maps are a useful tool for the visualization of light-induced electron density distribution changes in photocrystallography experiments.45 A photodifference map calculated using the experimental intensities from the initial and final data collections (details in ESI†) reveals regions of positive and negative density changes near the dmso ligands consistent with S → O isomerization (Fig. 3). Red surfaces, corresponding to negative changes in electron density, are observed near the initial ground state positions of the sulfur, oxygen and carbon atoms of both dmso ligands indicating a reduction of electron density at these positions. As expected, the approximate volume of these surfaces tracks with atom size (S > O > C). The green surfaces near the dmso ligands, corresponding to positive changes, are observed in close proximity to the ground-state Ru–S and S–C bonds. These regions are consistent with the approximate new positions of the sulphur and oxygen atoms following light-induced S–O isomerization and served as the basis for the subsequent refinement of the position and orientation of the dmso groups.
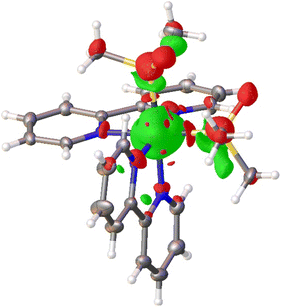 |
| Fig. 3 Photodifference map of the Ru–dmso complex with iso-meshes of 0.766 e Å−3 showing regions of positive and negative density changes near the dmso ligands. The positive and negative electron density iso-meshes are represented in green and red respectively. | |
Shown in Fig. 1 is the ground state structure of [S,S]-[Ru(bpy)2(dmso)2][B(Ph)4]2 (tetraphenylborate anion not shown) prior to laser irradiation. After ex situ irradiation with 405 nm light, the crystal structure revealed a mixture of S,S- and O,O-isomers. When modelling the disorder for partly isomerized structure (S,O-isomer), the dmso groups and the Ru were each refined independently. The dmso trans to N4 contains 10.0% in the O bonded conformation whereas the dmso cis to N4 contains 6.0%. The observation of disorder in both dmso ligands clearly indicates that both ligands isomerize, though given the differences in isomerization for both sulfoxides both S,O and O,O products are likely formed in the crystal lattice. The structure of the O,O-isomer is displayed in Fig. 1, right. This structure clearly features two O-bonded dmso ligands with a tetrahedral geometry about the sulfur, indicating that the sulfur has not changed oxidation state. We note that the unit cell volume of the crystal is essentially unchanged between the two structures (ΔV ∼ 0.13%; VS,S = 6139.9(4) Å3; VO,O = 6148.3(4) Å3; Table S1†). In addition to the new coordination mode of dmso, there are other changes in the new O,O-bonded structure relative to the initial S,S-bonded complex.
Table 1 summarizes the structural parameters of each isomer. Notably, there is a distinctive shortening of the bond between the Ru and the dmso from ∼2.3 Å to ∼1.8 Å, which is consistent with isomerization from Ru–S to Ru–O coordination modes. We find Ru–S bond distances of 2.291 and 2.285 Å, which are consistent with that found in other salts of [Ru(bpy)2(dmso)2]2+ as well as related bis(sulfoxides).46–48 Moreover, Calligaris has categorized geometric and infrared data for a wide variety of metal sulfoxide complexes in both S- and O-bonded coordination modes. He reports that the average Ru(II)–S bond distance is 2.260(2) Å from 474 independent ruthenium sulfoxide structures, which is in accord with our present findings.43 Whereas an average Ru(II)–O bond distance of 2.10(1) Å is obtained from statistical analysis of 291 independent structures. The bond distances of the Ru(II)–O bond reported here differ slightly from literature, which is likely a consequence of modelling low occupancy disorder. For example, the Ru–O bond distances in cis-[Ru(bpy)2(OH2)2]2+ are 2.147(4) and 2.152(4) Å.49 In [Ru(bpy)2(ox)]2+ (ox is oxalate), the Ru–O bond distances are 2.087(7) and 2.093(6) Å.50 Lastly, Ru–O bond distances of 2.050(8) and 2.030(8) Å were found in [Ru(bpy)2(DBSQ)]2+, where DBSQ is 3,5-di-tert-butyl-1,2-semiquinato.51
Table 1 Crystallographically determined bond lengths and angles for the ground state and metastable state isomers
S,S-Bonded isomer |
O,O-Bonded isomer |
Bond |
Distance (Å) |
Bond |
Distance (Å) |
Ru1–S1 |
2.291 |
Ru2–O1 |
1.813 |
Ru1–S2 |
2.285 |
Ru2–O2 |
1.803 |
Ru1–N1 |
2.073 |
Ru2–N1 |
2.028 |
Ru1–N2 |
2.099 |
Ru2–N2 |
1.983 |
Ru1–N3 |
2.080 |
Ru2–N3 |
2.099 |
Ru1–N4 |
2.083 |
Ru2–N4 |
1.883 |
S1–O1 |
1.481 |
S1–O1 |
1.561 |
S2–O2 |
1.482 |
S2–O2 |
1.533 |
Bond angle |
Angle (°) |
Bond angle |
Angle (°) |
N2–Ru1–N4 |
86.763° |
N2–Ru2–N4 |
96.23° |
S1–Ru1–N2 |
91.186° |
O1–Ru2–N2 |
90.569° |
S2–R1–N4 |
94.207° |
O2–Ru2–N4 |
81.314° |
S1–Ru1–S2 |
88.067° |
O1–Ru2–O2 |
92.514° |
Ru1–S1–O1 |
116.518° |
Ru2–O1–S1 |
136.729° |
Ru1–S2–O2 |
115.338° |
Ru2–O2–S2 |
126.280° |
The S–O bond distances for the ground state S,S-isomer (1.481 Å and 1.482 Å) are in accord with many existing structures (1.489(1) Å) and the S–O bond distances for the O,O-isomer are similar (1.561 Å and 1.533 Å) to what is typically observed (1.545(3) Å).43,44 The S–C bond distances for a Ru(II) S-bonded and O-bonded sulfoxide are 1.784(1) and 1.783(5) Å, respectively. The ground state structure reveals commensurate distances (1.768–1.786 Å) while the S–C bond distances for the O-bonded metastable state are close to the expected value (ranging from 1.659 to 1.804 Å). Likewise, the Ru–S–O angle (116.5° and 115.3°) is in good agreement with the literature data (Ru–S–O: 117.6°), while the Ru–O–S angles (136.7° and 126.2°) in the metastable state are just slightly wider than that outlined by Calligaris (122.0°).
There is also a distortion of the geometry about Ru between the ground state and metastable state structures. Specifically, the N2–Ru1–N4 angle is 86.763° for the S,S-isomer, while this angle is 96.23° for the O,O-isomer (Fig. 1). Similarly, the S1–Ru1–S2 angle for the S,S-isomer is 88.067°, whereas the corresponding angle in the O,O-isomer (O1–Ru2–O2) is 92.514°. We are in the process of preparing new complexes to define these distances and angles more precisely of the metastable state. Nevertheless, the diffraction data unequivocally show changes in the coordination mode of dmso following irradiation of the crystal.
We performed an in situ SC-XRD data collection study to determine if the extent of dmso isomerization was related to the total exposure to visible light (405 nm). Five complete data sets were collected sequentially on the same crystal during continuous irradiation (405 nm), and then the crystal structure was solved after each run to determine the percent of the O-bonded isomer as a function of total time irradiated. It was difficult to reproducibly observe the isomerization at cryogenic temperatures, so these data sets were collected at room temperature unlike the ex situ studies described above. This led to lower quality data sets; however, relevant conclusions may be drawn from this experiment. Chiefly, we confirmed that the concentration of the bis O-bonded isomer increased as a function of total light exposure (Fig. S5†). From the first data set to the final data set, the percent of the O-bonded dmso gradually increased from ∼10.1% to ∼12.2% and ∼7.7% to ∼10.4% for dmso1 and dmso2, respectively. Although XRD is unable to determine the precise distribution of S and O bonded isomers in the lattice (e.g., whether it is a mix of S,S and O,O or a mix of S,O/O,S), the dmso ligands refined independently and only differ in occupancies of the S- and O-bonded isomer by ∼3% throughout the entire data collection. This suggests that the isomerization of the dmso ligands is correlated with light exposure. The plateau observed is consistent with achieving a photostationary state within the crystalline environment.
Consistent with solution measurements, the isomerization is thermally reversible. When a dark red crystal containing a mixture of isomers (∼10% and 6%) was isolated in the dark for a week, the crystal reverted to a pale orange color, characteristic of the S-bonded isomer (Fig. S6†). SC-XRD measurements on this crystal (in ESI†) revealed conversion back to the ground state structure. Subsequent refinement showed only 2.6% of dmso1 remained O-bonded, whereas dmso2 displayed no evidence of the O-bonded isomer.
Conclusions
In aggregate, the data presented here establish that the metastable product from irradiation of photochromic [Ru(bpy)2(dmso)2][BPh4]2 is an O-bonded sulfoxide. This result is consistent with the proposal first outlined by Taube in 1982. This study is enabled by the incorporation of large, flexible, weakly coordinating anion within the salt. Future studies will focus on revealing the mechanistic details of the isomerization by photocrystallography and transient absorption spectroscopy in this and related complexes.
Data availability
Crystallographic data for the structures reported herein have been deposited as CCDC 2226309–2226311. Additional experimental details and data are provided in the ESI,† including a full description of synthetic procedures, crystallographic modelling techniques, UV-visible spectra of single crystals, and images showing changes to a single crystal upon irradiation.
Author contributions
ZYM, RTM, JBB, and JJR were responsible for conceptualization. ZYM, RTM, and BF were responsible for the investigation. ZYM and BF for formal analysis. JBB and JJR for funding acquisition. All authors participated in writing – review and editing.
Conflicts of interest
There are no conflicts to declare.
Acknowledgements
This research was supported by the National Science Foundation (NSF) through a collaborative grant to JBB (DMR 2003932) and JJR (DMR 2003336). JJR acknowledges NSF for an MRI grant for the purchase of mass spectrometric instrumentation (CHE 1920229).
Notes and references
- P. Coppens, I. Novozhilova and A. Kovalevsky, Photoinduced linkage isomers of transition-metal nitrosyl compounds and related complexes, Chem. Rev., 2002, 102, 861–883 CrossRef CAS PubMed.
- R. Medishetty, S. C. Sahoo, C. E. Mulijanto, P. Naumov and J. J. Vittal, Photosalient Behavior of Photoreactive Crystals, Chem. Mater., 2015, 27, 1821–1829 CrossRef CAS.
- P. Naumov, S. Chizhik, M. K. Panda, N. K. Nath and E. Boldyreva, Mechanically Responsive Molecular Crystals, Chem. Rev., 2015, 115, 12440–12490 CrossRef CAS PubMed.
- P. Naumov, D. P. Karothu, E. Ahmed, L. Catalano, P. Commins, J. M. Halabi, M. B. Al-Handawi and L. Li, The Rise of the Dynamic Crystals, J. Am. Chem. Soc., 2020, 142, 13256–13272 CrossRef CAS PubMed.
- K. R. Chalek, X. N. Dong, F. Tong, R. A. Kudla, L. Y. Zhu, A. D. Gill, W. W. Xu, C. Yang, J. D. Hartman, A. Magalhaes, R. O. Al-Kaysi, R. C. Hayward, R. J. Hooley, G. J. O. Beran, C. J. Bardeen and L. J. Mueller, Bridging photochemistry and photomechanics with NMR crystallography: the molecular basis for the macroscopic expansion of an anthracene ester nanorod, Chem. Sci., 2021, 12, 453–463 RSC.
- T. Kim, L. Y. Zhu, R. O. Al-Kaysi and C. J. Bardeen, Organic Photomechanical Materials, ChemPhysChem, 2014, 15, 400–414 CrossRef CAS PubMed.
- D. J. Shields, D. P. Karothu, K. Sambath, I. Ranaweera, S. Schramm, A. Duncan, B. Duncan, J. A. Krause, A. D. Gudmundsdottir and P. Naumov, Cracking under Internal Pressure: Photodynamic Behavior of Vinyl Azide Crystals through N-2 Release, J. Am. Chem. Soc., 2020, 142, 18565–18575 CrossRef CAS PubMed.
- M. D. Carducci, M. R. Pressprich and P. Coppens, Diffraction studies of photoexcited crystals: Metastable nitrosyl-linkage isomers of sodium nitroprusside, J. Am. Chem. Soc., 1997, 119, 2669–2678 CrossRef CAS.
- A. Y. Kovalevsky, G. King, K. A. Bagley and P. Coppens, Photoinduced oxygen transfer and double-linkage isomerism in a cis-(NO)(NO2) transition-metal complex by photocrystallography, FT-IR spectroscopy and DFT calculations, Chem.–Eur. J., 2005, 11, 7254–7264 CrossRef CAS PubMed.
- A. A. Mikhailov, E. Wenger, G. A. Kostin and D. Schaniel, Room-Temperature Photogeneration of Nitrosyl Linkage Isomers in Ruthenium Nitrosyl Complexes, Chem.–Eur. J., 2019, 25, 7569–7574 CrossRef CAS PubMed.
- G. A. Kostin, A. O. Borodin, A. A. Mikhailov, N. V. Kuratieva, B. A. Kolesov, D. P. Pishchur, T. Woike and D. Schaniel, Photocrystallographic, Spectroscopic, and Calorimetric Analysis of Light-Induced Linkage NO Isomers in RuNO(NO2)(2)(pyridine)(2)OH, Eur. J. Inorg. Chem., 2015, 4905–4913, DOI:10.1002/ejic.201500702.
- D. V. Fomitchev, I. Novozhilova and P. Coppens, Photo-induced linkage isomerism of transition metal nitrosyl and dinitrogen complexes studied by photocrystallographic techniques, Tetrahedron, 2000, 56, 6813–6820 CrossRef CAS.
- J. M. Cole, J. d. J. Velazquez-Garcia, D. J. Gosztola, S. G. Wang and Y.-S. Chen, Eta(2)-SO2 Linkage Photoisomer of an Osmium Coordination Complex, Inorg. Chem., 2018, 57, 2673–2677 CrossRef CAS PubMed.
- J. M. Cole, J. d. J. Velazquez-Garcia, D. J. Gosztola, S. G. Wang and Y.-S. Chen, Light-Induced Macroscopic Peeling of Single Crystal Driven by Photoisomeric Nano-Optical Switching, Chem. Mater., 2019, 31, 4927–4935 CrossRef CAS.
- S. O. Sylvester, J. M. Cole and P. G. Waddell, Photoconversion Bonding Mechanism in Ruthenium Sulfur Dioxide Linkage Photoisomers Revealed by in Situ Diffraction, J. Am. Chem. Soc., 2012, 134, 11860–11863 CrossRef CAS PubMed.
- L. E. Hatcher, J. Christensen, M. L. Hamilton, J. Trincao, D. R. Allan, M. R. Warren, I. P. Clarke, M. Towrie, S. Fuertes, C. C. Wilson, C. H. Woodall and P. R. Raithby, Steady- State and Pseudo-Steady-State Photocrystallographic Studies on Linkage Isomers of Ni(Et(4)dien)(n(2)-O,ON)(n(1)-NO2): Identification of a New Linkage Isomer, Chem.–Eur. J., 2014, 20, 3128–3134 CrossRef CAS PubMed.
- L. E. Hatcher and P. R. Raithby, Dynamic single-crystal diffraction studies using synchrotron radiation, Coord. Chem. Rev., 2014, 277, 69–79 CrossRef.
- M. R. Warren, S. K. Brayshaw, A. L. Johnson, S. Schiffers, P. R. Raithby, T. L. Easun, M. W. George, J. E. Warren and S. J. Teat, Reversible 100% Linkage Isomerization in a Single-Crystal to Single-Crystal Transformation: Photocrystallographic Identification of the Metastable Ni(dppe)(eta(1)-ONO)Cl Isomer, Angew. Chem., Int. Ed., 2009, 48, 5711–5714 CrossRef CAS PubMed.
- J. M. Cox, I. M. Walton, D. G. Patel, M. Xu, Y.-S. Chen and J. B. Benedict, The Temperature Dependent Photoswitching of a Classic Diarylethene Monitored by in Situ X-ray Diffraction, J. Phys. Chem. A, 2015, 119, 884–888 CrossRef CAS PubMed.
- M. I. Gonzalez, D. Gygi, Y. Qin, Q. Zhu, E. J. Johnson, Y.-S. Chen and D. G. Nocera, Taming the Chlorine Radical: Enforcing Steric Control over Chlorine-Radical-Mediated C-H Activation, J. Am. Chem. Soc., 2022, 144, 1464–1472 CrossRef CAS PubMed.
- D. Gygi, M. I. Gonzalez, S. J. Hwang, K. T. Xia, Y. Qin, E. J. Johnson, F. Gygi, Y.-S. Chen and D. G. Nocera, Capturing the Complete Reaction Profile of a C-H Bond Activation, J. Am. Chem. Soc., 2021, 143, 6060–6064 CrossRef CAS PubMed.
- D. C. Powers, S. J. Hwang, B. L. Anderson, H. Yang, S.-L. Zheng, Y.-S. Chen, T. R. Cook, F. P. Gabbai and D. G. Nocera, Stereoelectronic Effects in Cl-2 Elimination from Binuclear Pt(III) Complexes, Inorg. Chem., 2016, 55, 11815–11820 CrossRef CAS PubMed.
- A. W. King, L. Wang and J. J. Rack, Excited State Dynamics and Isomerization in Ruthenium Sulfoxide Complexes, Acc. Chem. Res., 2015, 48, 1115–1122 CrossRef CAS PubMed.
- J. J. Rack, Electron transfer triggered sulfoxide isomerization in ruthenium and osmium complexes, Coord. Chem. Rev., 2009, 253, 78–85 CrossRef CAS.
- S. B. Vittardi, R. T. Magar, D. J. Breen and J. J. Rack, A Future Perspective on Phototriggered Isomerizations of Transition Metal Sulfoxides and Related Complexes, J. Am. Chem. Soc., 2021, 143, 526–537 CrossRef CAS PubMed.
- A. Yeh, N. Scott and H. Taube, S to O and O to S Linkage Isomerization in Sulfoxide Complexes of Pentaammine-Ruthenium, Inorg. Chem., 1982, 21, 2542–2545 CrossRef CAS.
- F. C. March and G. Ferguson, Crystal Structure of Dimethylsulfoxidepentaammineruthenium(II) Hexafluorophosphate, Can. J. Chem., 1971, 49, 3590–3595 CrossRef CAS.
- C. V. Senoff, E. Maslowsk and R. G. Goel, Dimethylsulfoxidepentaammineruthenium(II) Hexafluorophosphate - Spectroscopic Study, Can. J. Chem., 1971, 49, 3585–3589 CrossRef CAS.
- A. Tomita and M. Sano, Linkage Isomerizations of (Sulfoxide)Ammineruthenium Complexes Induced by Electrochemical Processes, Inorg. Chem., 1994, 33, 5825–5830 CrossRef CAS.
- A. Tomita and M. Sano, Preparations and electrochemical properties of pyrazine- bridged ruthenium-binuclear complexes exhibiting molecular hysteresis, Inorg. Chem., 2000, 39, 200–205 CrossRef CAS PubMed.
- E. M. Kober and T. J. Meyer, Concerning the Absorption-Spectra of the Ions M(Bpy)32+ (M=Fe,Ru,Os, Bpy=2,2'-Bipyridine), Inorg. Chem., 1982, 21, 3967–3977 CrossRef CAS.
- D. P. Butcher Jr, A. A. Rachford, J. L. Petersen and J. J. Rack, Phototriggered S -> O isomerization of a ruthenium-bound chelating sulfoxide, Inorg. Chem., 2006, 45, 9178–9180 CrossRef PubMed.
- C. S. Keating, B. A. McClure, J. J. Rack and I. V. Rubtsov, Sulfoxide stretching mode as a structural reporter via dual-frequency two-dimensional infrared spectroscopy, J. Chem. Phys., 2010, 133, 144513 CrossRef PubMed.
- C. S. Keating, B. A. McClure, J. J. Rack and I. V. Rubtsov, Mode Coupling Pattern Changes Drastically Upon Photoisomerization in Ru-II Complex, J. Phys. Chem. C, 2010, 114, 16740–16745 CrossRef CAS.
- D. A. Lutterman, A. A. Rachford, J. J. Rack and C. Turro, Theoretical Insight on the S -> O Photoisomerization of DMSO Complexes of Ru(II), J. Phys. Chem. A, 2009, 113, 11002–11006 CrossRef CAS PubMed.
- D. A. Lutterman, A. A. Rachford, J. J. Rack and C. Turro, Electronic and Steric Effects on the Photoisomerization of Dimethylsulfoxide Complexes of Ru(II) Containing Picolinate, J. Phys. Chem. Lett., 2010, 1, 3371–3375 CrossRef CAS.
- A. J. Goettle, I. M. Dixon, F. Alary, J.-L. Heully and M. Boggio-Pasqua, Adiabatic Versus Nonadiabatic Photoisomerization in Photochromic Ruthenium Sulfoxide Complexes: A Mechanistic Picture from Density Functional Theory Calculations, J. Am. Chem. Soc., 2011, 133, 9172–9174 CrossRef PubMed.
- I. Ciofini, C. A. Daul and C. Adamo, Phototriggered linkage isomerization in ruthenium -dimethylsulfoxyde complexes: insights from theory, J. Phys. Chem. A, 2003, 107, 11182–11190 CrossRef CAS.
- H. Li, L. Zhang, X. Fan and Y. Zhao, Theoretical Studies on the Redox-Stimulated Isomerization in Electrochromic Osmium Sulfoxide Complexes, J. Phys. Chem. A, 2015, 119, 4244–4251 CrossRef CAS PubMed.
- H. Li, L. Zhang, H. Lin and X. Fan, A DFT-D study on the electronic and photophysical properties of ruthenium (II) complex with a chelating sulfoxide group, Chem. Phys. Lett., 2014, 604, 10–14 CrossRef CAS.
- H. Li, L. Zhang, M. Ye and X. Fan, A Comparative Study of the Redox-Induced Linkage Isomerization of Ruthenium and Osmium Complexes, Eur. J. Inorg. Chem., 2015, 5074–5080, DOI:10.1002/ejic.201500666.
- A. A. Cordones, J. H. Lee, K. Hong, H. Cho, K. Garg, M. Boggio-Pasqua, J. J. Rack, N. Huse, R. W. Schoenlein and T. K. Kim, Transient metal-centered states mediate isomerization of a photochromic ruthenium sulfoxide complex, Nat. Commun., 2018, 9, 1989 CrossRef PubMed.
- M. Calligaris, Structure and bonding in metal sulfoxide complexes: an update, Coord. Chem. Rev., 2004, 248, 351–375 CrossRef CAS.
- M. Calligaris and O. Carugo, Structure and bonding in metal sulfoxide complexes, Coord. Chem. Rev., 1996, 153, 83–154 CrossRef CAS.
- B. Fournier and P. Coppens, On the assessment of time-resolved diffraction results, Acta Crystallogr., Sect. A: Found. Adv., 2014, 70, 291–299 CrossRef CAS PubMed.
- M. K. Smith, J. A. Gibson, C. G. Young, J. A. Broomhead, P. C. Junk and F. R. Keene, Photoinduced Ligand Isomerization in Dimethyl Sulfoxide Complexes of Ruthenium(II), Eur. J. Inorg. Chem., 2000, 2000, 1365–1370 CrossRef.
- B. A. McClure and J. J. Rack, Two-Color Reversible Switching in a Photochromic Ruthenium Sulfoxide Complex, Angew. Chem., Int. Ed., 2009, 48, 8556–8558 CrossRef CAS PubMed.
- N. V. Mockus, D. Rabinovich, J. L. Petersen and J. J. Rack, Femtosecond Isomerization in a Photochromic Molecular Switch, Angew. Chem., Int. Ed., 2008, 47, 1458–1461 CrossRef CAS PubMed.
- M. G. Sauaia, E. Tfouni, R. H. d. A. Santos, M. T. d. P. Gambardella, M. P. F. M. Del Lama, L. F. Guimarães and R. S. da Silva, Use of HPLC in the identification of cis and trans-diaquabis(2,2′-bipyridine)ruthenium(II) complexes: crystal structure of cis-[Ru(H2O)2(bpy)2](PF6)2, Inorg. Chem. Commun., 2003, 6, 864–868 CrossRef.
- H.-Y. Shen, W.-M. Bu, D.-Z. Liao, Z.-H. Jiang, S.-P. Yan and G.-L. Wang, A new three-dimensional ruthenium(II)
complex via hydrogen bonds: Ru(bpy)2(ox)·4H2O (bpy=2,2′-bipyridine, ox=oxalate ion), Inorg. Chem. Commun., 1999, 2, 615–618 CrossRef CAS.
- S. R. Boone and C. G. Pierpont, Charge delocalization in ruthenium-quinone complexes. Structural characterization of bis(bipyridine)(3,5-di-tert-butylsemiquinonato)ruthenium(II) perchlorate and trans-bis(4-tert-butylpyridine)bis(3,5-di-tert-butylquinone)ruthenium, Inorg. Chem., 1987, 26, 1769–1773 CrossRef CAS.
|
This journal is © The Royal Society of Chemistry 2023 |
Click here to see how this site uses Cookies. View our privacy policy here.