DOI:
10.1039/D3SD00020F
(Paper)
Sens. Diagn., 2023,
2, 665-675
A benzimidazole-derived fluorescent chemosensor for Cu(II)-selective turn-off and Zn(II)-selective ratiometric turn-on detection in aqueous solutions†
Received
14th January 2023
, Accepted 6th March 2023
First published on 6th March 2023
1. Introduction
The design and development of organic small-molecule fluorescent chemosensors for the detection of various metal ions has attracted extensive attention.1–4 Zn2+ and Cu2+ are known to be important trace elements that are involved in many physiological processes.5,6 However, it has also been noted that an imbalance of Zn2+ and Cu2+ concentrations can lead to various diseases, such as metabolic disorders, Parkinson's disease, and coronary heart disease.7–10 Hence, it would be of great significance to develop a highly selective and sensitive fluorescent chemosensor to quantitatively detect Cu2+ and Zn2+ in biological and environmental fields. In this regard, a number of fluorescent sensors for Cu2+ and Zn2+ detection have been designed based on various coordination scaffolds.9–27 For example, a naphthalene Schiff-base system was designed as a fluorescent sensor for Cu2+ and Zn2+, where the fluorescence of the sensor was quenched by Cu2+or enhanced by Zn2+ over other metal cations in DMSO, respectively.11 Kim et al. reported a phenanthrene-based multifunctional chemosensor that showed a slightly enhanced and blue-shifted (45 nm) fluorescence emission in the presence of Zn2+, whereas Cu2+ caused a serious quenching of the original fluorescence.18 Recently, a dual-functional Schiff-base fluorescent sensor containing the coumarin fluorophore was developed for Zn2+ and Cu2+ detection, in which Zn2+ induced a red-shift (46 nm) of the emission wavelength, allowing it to be used for the construction of a molecular logic gate with metal ion inputs and fluorescence signal outputs.22 Even the dual recognition of different Cu2+ and Zn2+ ions by a single sensor has been achieved, but most of their fluorescence emission showed no distinct or just a slight shift from the original one over binding events. It is practically undesirable for a dual-functional fluorescent chemosensor to be used for two different metal ions due to the discrimination being more difficult to as ascertain from a fluorescence intensity change than from a color change. Moreover, it has been considered that the fluorescence intensity is usually dependent on the measurement conditions, such as the concentration, excitation light intensity, and instrument efficiency, and that a ratiometric fluorescent sensor could overcome such shortcomings.3,28,29 Therefore, a dual-functional fluorescent sensor for Cu2+ and Zn2+ that could exhibit a large emission wavelength shift from the original one upon binding with metal ions would be promising for a ratiometric sensor, but would be challenging to achieve.30
Recently, benzimidazole-containing fluorescent sensors have attracted much interest due to their nice coordination ability toward metal ions.31–36 Anbu et al. reported a benzimidazole-based Schiff-base-type fluorescent sensor that could differentially detect Cu2+ and Zn2+ through a fluorescence on–off and enhancement manner, respectively.36 However, in the case of Zn2+ detection, the fluorescence emission was only blue-shifted by 10 nm. In this work, we designed and developed the novel benzimidazole-derived fluorescent sensor BBMP (Scheme 1) for Cu2+ and Zn2+. The present BBMP exhibited an excited-state intramolecular proton-transfer (ESIPT) emission at 542 nm and fluorescence quenching upon binding to Cu2+ and Zn2+ due to the inhibition of ESIPT. Notably, the BBMP also showed an 82 nm blue-shifted fluorescence emission upon binding to Zn2+, allowing ratiometric detection. Thus a benzimidazole-derived fluorescent sensor was developed for Cu2+-selective turn-off and Zn2+-selective ratiometric turn-on detection in aqueous solution. Furthermore, this was successfully used for Cu2+ and Zn2+ detection in real water samples and for fluorescence imaging in living cells, respectively.
 |
| Scheme 1 Synthesis of BBMP. | |
2. Experimental
2.1. Materials and methods
4-Nethoxyphenol, hexamethylenetetramine, o-phenylenediamine, trifluoroacetic, hydrochloride acid, and all organic solvents were analytical grade and purchased from Sinopharm Chemical Reagents Co. (Shanghai, China). The solutions of metal cations were prepared by dissolving the corresponding perchlorates (Mg2+, Hg2+), chlorides (Ca2+, Al3+, Mn2+, Co2+, Pd2+), sulfates (Fe2+, Cu2+), or nitrates (Fe3+, Ni2+, Zn2+, Ag+, Cd2+, Pb2+) salts with deionized water (10 mM). The stock solution of the BBMP sensor (1 mM) was dissolved in DMSO and the working solution was obtained by dilution with Tris-HCl buffer solution (10 mM, pH 7.4). The prepared solution was used for spectral testing and cellular imaging.
An Edinburgh FS5 spectrofluorometer was employed to measure the fluorescence spectra at room temperature (the slit width was set to 5 nm for both excitation and emission). Absolute fluorescence quantum yield measurements were carried out on an Edinburgh FS5 spectrofluorometer equipped with an integrating sphere (EI-FS5-SC-30). The UV-vis absorption spectra were collected on a Shimadzu UV-1800 spectrophotometer. The 1H and 13C NMR spectra were recorded on a Bruker AVANCE III 400 MHz spectrometer with TMS as the internal standard. The high-resolution mass spectra were obtained on Micro TOFII 10257, Agilent 7250, and JEOL-JMS-T100LP AccuTOF mass spectrometers, respectively.
For analysis of real water samples, lake water and drinking water samples were collected from Songjiang Campus of Donghua University and a water dispenser, respectively. Zinc gluconate oral liquid (Harbin Pharm Group Sanjing Pharmaceutical Co. Ltd, China) was purchased from a local medical store. Fluorescence imaging in living HeLa cells was observed by an Axio Vert, A1 Carl Zeiss fluorescence microscopy system (Jena, Germany).
2.2. Synthesis of BBMP
The BBMP sensor was synthesized by a concise two-step process, as summarized in Scheme 1. First, 2,6-diformyl-4-methoxyphenol was synthesized according to our previously works.37–39 Specifically, under a N2 atmosphere, 4-methoxyphenol (15 mmol) and hexamethylenetetramine (45 mmol) were dissolved in trifluoroacetic acid (25 mL) and refluxed at 110 °C for 72 h. The mixture was cooled to 90 °C and 1 M HCl solution was added and stirring was continued for 24 h. The mixture was filtered and the filtrate was extracted with dichloromethane (25 mL × 3). Then the filtered solid and the extract were combined and washed with water until neutral pH. The organic phase was dried over anhydrous Na2SO4 for half an hour and concentrated under reduced pressure evaporation. The crude product was further purified by column chromatography (silica gel, ethyl acetate
:
petroleum ether = 1
:
10). Finally, a yellow flocculent solid was obtained. Yield, 30%. 1H NMR (400 MHz, chloroform-d), δ (ppm): 11.13 (s, 1H), 10.22 (s, 2H), 7.51 (s, 2H), 3.86 (s, 3H).
Subsequently, under a N2 atmosphere, 2,6-diformyl-4-methoxyphenol (1 mmol) and o-phenylenediamine (2.4 mmol) were dissolved in dichloromethane (15 mL) and refluxed for 63 h. After the reaction was completed, the mixture was cooled to room temperature, and the crude product was obtained by filtration, and washed with dichloromethane three times to obtain the target BBMP as an orange solid. Yield, 16.7%. 1H NMR (400 MHz, DMSO-d6), δ (ppm): 13.50 (s, 3H), 7.95 (s, 2H), 7.71 (dd, J = 6.0, 3.0 Hz, 4H), 7.29 (dd, J = 6.0, 3.0 Hz, 4H), 3.92 (s, 3H); 13C NMR (101 MHz, DMSO-d6), δ (ppm): 152.26, 150.86, 150.37, 138.01, 123.20, 116.38, 115.57, 115.08, 56.37. MS: m/z calcd for C21H16N4O2 357.1347 (M + H)+; found 357.1352 (M + H)+.
3. Results and discussion
3.1. Photophysical properties of BBMP
The absorption and fluorescence spectra of BBMP were first studied in various solvents (Fig. 1). It could be seen that BBMP showed a yellowish-green fluorescence emission at 548 nm and two absorption peaks around 300 nm as well as 375 nm in the weak polar solvents DEE, CHCl3, and THF, respectively. Notably, the fluorescence emission of BBMP at 548 nm was slightly blue-shifted several nanometers in the strong polar solvents DMSO, DMF, and MeOH, accompanied by the appearance of weak fluorescence around 470 nm, respectively (Fig. 1a). Meanwhile, a new and broad absorption peak was discernable at 400–500 nm in these polar solvents (Fig. 1b). The large Stokes shift (ca. 8400 cm−1) and similar molecular structure feature to 2-(2-hydroxyphenyl)benzimidazole most probably indicated an ESIPT emission nature.40 Therefore, the solvent-dependent photophysical properties could be tentatively rationalized according to a subtle solvent effect on the intramolecular hydrogen-bond interaction between the imidazole N atom and phenolic OH in BBMP. The weak polar solvents DEE, CHCl3, and THF had little effect on the intramolecular hydrogen bonds in BBMP, and thus normal ESIPT emission was observed at 548 nm, without disturbance to the absorption spectra. In contrast, the strong polar solvents DMSO, DMF, and MeOH, could cause competition between the intermolecular and intramolecular hydrogen-bond interactions, as confirmed by the observation of a substantial change in the absorption and fluorescence spectra in these polar solvents. The more prominent change in DMF and MeOH could be related to their basic and protic nature, respectively. ESIPT emission only underwent a slight disturbance in the polar solvents, indicating that strong intramolecular hydrogen-bond interactions existed in BBMP. Interestingly, when NaOH was introduced into the THF solution of BBMP, a largely blue-shifted fluorescence emission at 490 nm and a red-shifted absorption band at 407 nm were observed (Fig. 1). It could be ascribed to the ESIPT being completely inhibited due to the anionic BBMP formed by the deprotonation in the presence of a strong base, as has previously been observed in 2-(2-hydroxyphenyl)benzothiazole derivatives.41 In addition, the absolute fluorescence quantum yields of BBMP were measured to be in the range of 0.6–0.9 in various solvents (Table S1†).
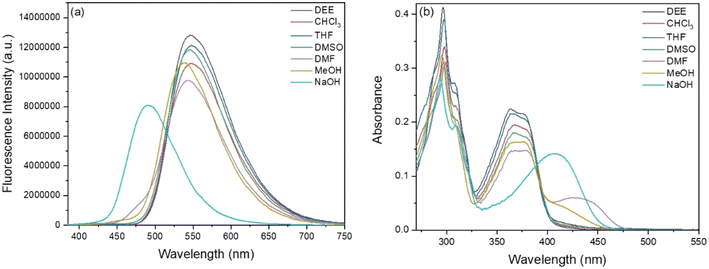 |
| Fig. 1 Fluorescence (a) and absorption (b) spectra of BBMP (10 μM) in various solvents. The excitation wavelength was 370 nm. | |
3.2. Selectivity toward Zn2+ and Cu2+ detection
Then the selectivity of BBMP toward various metal ions was investigated in aqueous solution (pH 7.4 Tris-HCl buffer containing 70% THF) as shown in Fig. 2. Without metal ions, the BBMP sensor showed a strong yellowish-green ESIPT fluorescence at 542 nm under excitation at 370 nm. Significant fluorescence quenching was observed in the presence of Cu2+, whereas Zn2+ selectively induced only a moderate quenching of the original ESIPT emission accompanied by the appearance of new blue fluorescence at 462 nm (Fig. 2a). In contrast, the fluorescence of BBMP showed almost no obvious change in the presence of other metal ions (Ca2+, Mg2+, Al3+, Mn2+, Fe3+, Fe2+, Ni2+, Pd2+, Ag+, Cd2+, Hg2+, Pb2+), except for Co2+, which caused a slight quenching effect. This demonstrated that the present BBMP could serve as a novel fluorescent sensor for Cu(II)-selective turn-off and Zn(II)-selective ratiometric turn-on detection in aqueous solution. Fig. 2b shows the absorption spectra of BBMP in the absence and presence of various metal ions. Obviously red-shifted absorption was observed upon the addition of Cu2+, Zn2+, and Co2+ respectively, suggesting that BBMP could substantially coordinate with these metal ions. Combined with the photophysical properties of BBMP in various solvents (Fig. 1), it could tentatively be concluded that the ESIPT emission was inhibited upon binding to the metal ions.
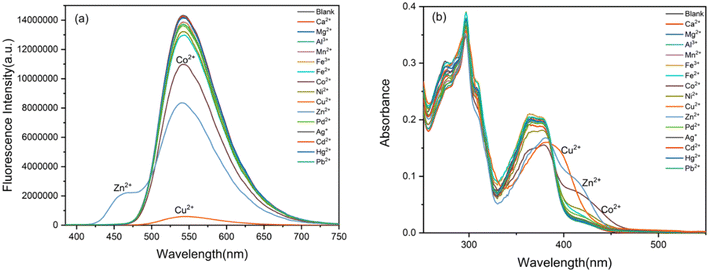 |
| Fig. 2 Fluorescence (a) and absorption (b) spectra of BBMP (10 μM) upon the addition of 1 equiv. of various metal ions in Tris-HCl aqueous solution (10 mM, pH 7.4, containing 70% THF). The excitation wavelength was 370 nm. | |
To further examine the potential interference of others metal ions toward Cu2+ and Zn2+ detection, competition experiments were also conducted by measuring the fluorescence intensity at 542 nm and the intensity ratio at 462/541 nm. Fig. 3 shows that no significant interference was observed in the turn-off detection of Cu2+ and ratiometric turn-on detection of Zn2+, respectively. Thus, the present BBMP sensor could selectively and differentially detect Cu2+ and Zn2+ in aqueous solution.
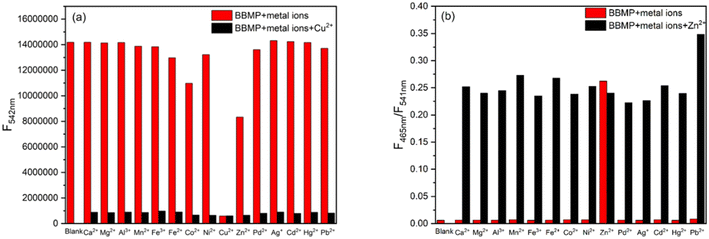 |
| Fig. 3 Competition experiments for Cu2+ (a) and Zn2+ (b) in aqueous Tris-HCl solution (10 mM, pH 7.4, 70% THF). The excitation wavelength was 370 nm. | |
3.3. Response time and effect of the pH
To obtain the optimal detection conditions, the response time and effect of the pH were investigated. As shown in Fig. 4a, upon the addition of 1 equiv. Cu2+, the fluorescence of BBMP (10 μM) at 542 nm rapidly declined but became stable within 2 min. In the case of Zn2+, the fluorescence intensity ratio of 465/541 nm increased to the maximum within 3 min (Fig. 4b). This indicated that the response of BBMP toward Cu2+ and Zn2+ was fast, and all the solution measurements were therefore performed after standing for 3 min.
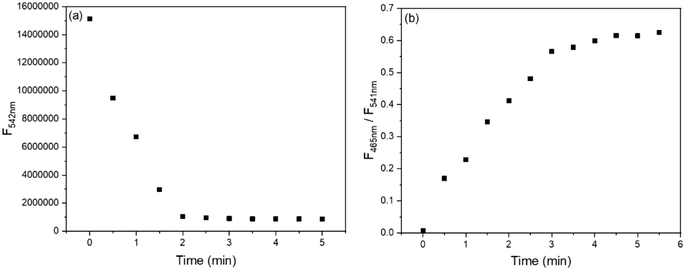 |
| Fig. 4 Change in the fluorescence intensity of BBMP (10 μM) with time upon the addition of 1 equiv. Cu2+ (a) and Zn2+ (b) in aqueous Tris-HCl solution (10 mM, pH 7.4, 70% THF), respectively. The excitation wavelength was 370 nm. | |
Subsequently, the fluorescence of BBMP in the absence and presence of 1 equiv. Cu2+ and Zn2+ was tested in the pH range of 2–11, respectively. It was found that the yellowish-green fluorescence of BBMP almost remained constant at pH <9, while the intensity at 542 nm slightly declined between pH 9–10, and a blue fluorescence emission appeared at 489 nm at pH >10 (Fig. 5a and S1a†). This could be attributed to deprotonation of the phenolic OH in BBMP and as anionic BBMP was produced at pH >10. In the presence of Cu2+, the fluorescence of BBMP was drastically quenched at pH <10, and also a blue fluorescence at 489 nm was observed at pH >10 originating from the anionic BBMP (Fig. 5a and S1b†). Notably, upon the addition of 1 equiv. Zn2+, the yellowish-green fluorescence of BBMP was slightly changed at pH <6 and moderate quenching was observed between pH 7–10, accompanied by the appearance of new blue fluorescence emission at 465 nm (Fig. 5b and S1c†). This allowed the ratiometric fluorescence detection of Zn2+. Similarly, blue fluorescence at 489 nm from the anionic BBMP was also observed at pH >10 (Fig. S1c†). In this work, pH 7.4 Tris-HCl buffer solution was then selected to meet the requirements for physiological environment applications.
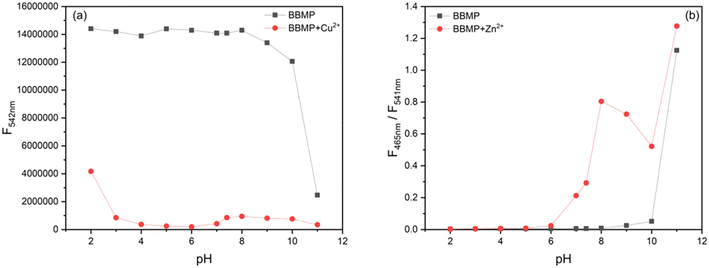 |
| Fig. 5 Change in the fluorescence intensity of BBMP (10 μM) at different pH 2–11 upon the addition of 1 equiv. Cu2+ (a) and Zn2+ (b) in aqueous Tris-HCl solution (10 mM, pH 7.4, 70% THF), respectively. The excitation wavelength was 370 nm. | |
3.4. Fluorescence and absorption titrations
Under the optimal experimental conditions, fluorescence and absorption titrations were performed to evaluate the sensing performance. Fig. 6 shows the fluorescence spectra and titration curves with the continuous increase in the concentration of Cu2+ and Zn2+ in Tris-HCl buffer (10 mM, pH 7.4, 70% THF), respectively. It could be seen that the fluorescence intensity of the BBMP sensor at 542 nm gradually decreased with increasing the concentration of Cu2+, and a good linear relationship was obtained (R2 = 0.99027) within the concentration range 0–5 μM (Fig. 6a and b), with a detection limit of 0.16 μM (S/N = 3). As shown in Fig. 6c and d, upon the continuous addition of Zn2+, the fluorescence intensity decreased at 541 nm and was enhanced at 465 nm, and a good linear relationship (R2 = 0.99037) was obtained between the ratio of the fluorescence intensity (F465nm/F541nm) and the Zn2+ concentration within the concentration range 0–10 μM, with a detection limit of 0.1 μM (S/N = 3). When compared against the previously reported sensing systems, the present sensor showed a comparable and even better performance (Table S2†).
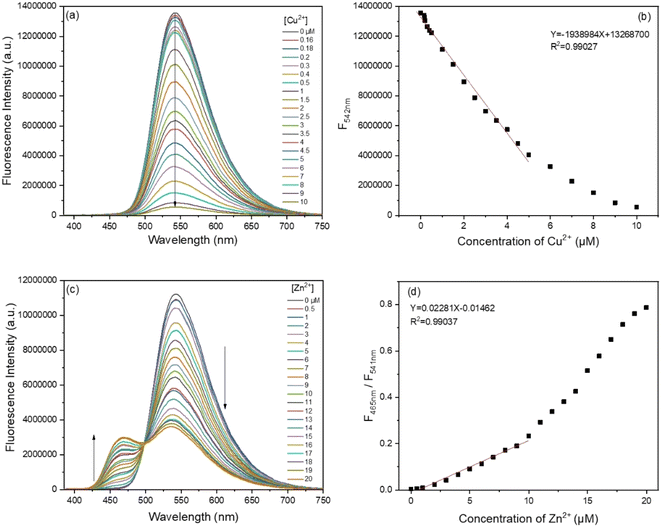 |
| Fig. 6 Fluorescence titration spectra of BBMP (10 μM) upon the addition of different concentrations of Cu2+ (a) and Zn2+ (c) in aqueous Tris-HCl solution (10 mM, pH 7.4, 70% THF), as well as a corresponding linear correlation between the fluorescence intensity and concentrations of Cu2+ (b) and Zn2+ (d), respectively. The excitation wavelength was 370 nm. | |
In addition, absorption titrations of Cu2+ and Zn2+ were also performed in Tris-HCl buffer (10 mM, pH 7.4, 70% THF), respectively. It was found that the absorption gradually decreased at 375 nm and increased at 420 nm with increasing the concentration of Cu2+ and Zn2+, respectively (Fig. S2†). A well-defined isosbestic point was observed at 386 nm, suggesting a complex was formed between the BBMP sensor and metal ions.
3.5. Binding reversibility and modes of BBMP to Cu2+ and Zn2+
Binding reversibility was investigated by the addition of ethylene diamine tetraacetic acid (EDTA) into the mixed solution of BBMP with Cu2+ or Zn2+. It was observed that the introduction of EDTA could almost recover the fluorescence of BBMP at 542 nm, indicting the competitive chelation between EDTA and Cu2+ or Zn2+ would release the free BBMP and thus restore its fluorescence. The further addition of Cu2+ or Zn2+ could then cause fluorescence quenching again, confirming the binding between BBMP and Cu2+ or Zn2+ was reversible (Fig. S3†).
The coordination stoichiometric ratio between BBMP and Cu2+ or Zn2+ was investigated by the Job's plot method, with the absorption spectra and results shown in Fig. 7. Obviously, a 2
:
1 or 1
:
1 binding mode between BBMP and Cu2+ or Zn2+ was obtained. This was further confirmed by ESI-MS analysis of the mixture solution of BBMP with Cu2+ or Zn2+ in MeOH, respectively. A prominent peak was found at m/z 837.08774, which could be readily assigned to [2BBMP–2H + Cu2+ + 2MeOH]+ species (Fig. S4a and c†). In addition, the mixture solution of BBMP with Zn2+ exhibited a complex peak at m/z 609.22409, which was attributed to [BBMP + Zn(NO3)2 + 2MeOH]+ species (Fig. S4b and d†). This demonstrated that BBMP formed a 2
:
1 complex with Cu2+ and a 1
:
1 complex with Zn2+. To further elucidate the binding mode, the ground-state molecular geometries of BBMP and its complexes with Cu2+ or Zn(NO3)2 were optimized by DFT calculations using the Gaussian 09 program package at the B3LYP/6-31 + G(d) level,42 and the results are displayed in Fig. 8. The optimized BBMP showed that one benzimidazole unit was near coplanar with the phenol ring and the distance of the imidazole N⋯HO was 1.7199 Å, indicating the existence of intramolecular hydrogen-bonding that facilitated the ESIPT (Fig. 8a). However, the other benzimidazole unit in BBMP was found to be twisted about 53.8° from the phenol plane. Regarding the optimized complexes, both the imidazole N and phenolic O atoms were involved in coordination with metal ions, where the distances of O⋯Cu, N⋯Cu, O⋯Zn, and N⋯Zn were found to be 2.2186, 1.9812, 1.9968, and 2.0880 Å, respectively (Fig. 8b and c). Notably, the benzimidazole units involved in coordination were twisted 22.4° (Cu complex) or 16.2° (Zn complex) from the phenol plane. However, the non-coordinated benzimidazole units were almost planar with the phenol ring, where the distance of the phenolic O⋯HN (imine) was 1.8285 Å (Cu complex) or 1.9075 Å (Zn complex), suggesting intramolecular hydrogen-bonding was most probably involved in the complexes (Fig. 8b and c).
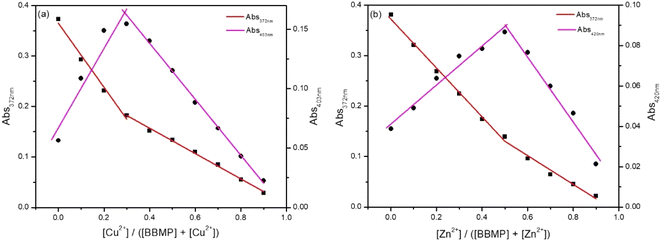 |
| Fig. 7 Job plots for BBMP + Cu2+ (a) and BBMP + Zn2+ (b) complexes in aqueous Tris-HCl solution (10 mM, pH 7.4, 70% THF), respectively. Both the total concentrations of [BBMP] + [Cu2+] = 20 μM and [BBMP] + [Zn2+] = 20 μM. | |
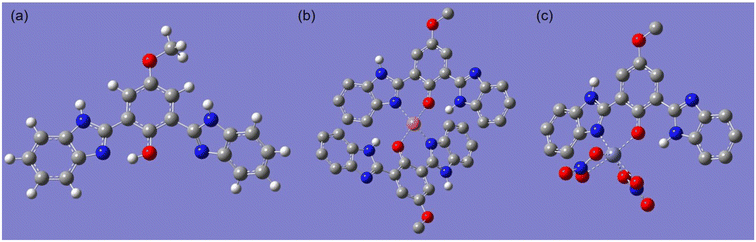 |
| Fig. 8 Calculated molecular structures of BBMP (a) and its complexes with Cu2+ (b) and Zn(NO3)2 (c). Gray, blue, red, pink, light blue, and white colors denote C, N, O, Cu, Zn, and H atoms, respectively. All the H atoms except imine NH in (b) and (c) were omitted for clarity. | |
Therefore, the sensing mechanism could be understood by considering both the coordination interaction and natural property of the metal cation. Upon the addition of Cu2+/Zn2+, the complex formed between BBMP and the metal ion via the imidazole N atom and deprotonated phenolic group coordination could inhibit the ESIPT effect.16 A turn-off response was observed in the presence Cu2+ due to its paramagnetic character, whereas a blue-shifted and turn-on fluorescence response with Zn2+ was observed, possibly resulting from a chelation-enhanced fluorescence effect.
3.6. Fluorescence detection of Cu2+ and Zn2+ in real samples
In order to investigate the practical application of the BBMP sensor, the fluorescence detection of Cu2+ and Zn2+ was performed in real water samples and zinc gluconate oral liquid, respectively. The lake water samples were collected from Songjiang Campus of Donghua University and filtrated to remove solid impurities before use. A standard addition method was used and five measurements were collected to estimate the relative standard deviation (RSD) and recovery rate. The analysis results are summarized in Tables 1 and 2. It was found that the recovery rate and RSD were 106–127.5% and 0.41–1.76% for Cu2+ detection in the real water samples (Table 1). The Zn2+ amount in zinc gluconate oral liquid was determined to be 4.12 μM, which matched well with the real value of 4 μM. The recovery rate and RSD were 99.5–108.14% and 1.19–6.02% for Zn2+ detection in the real samples (Table 2). Therefore, this demonstrated that the present BBMP sensor can be used as a promising fluorescent sensor for the rapid detection of Cu2+ and Zn2+ in practical water samples.
Table 1 Detection of Cu2+ in real water samples (n = 5)
Samples |
Added Cu2+ (μM) |
Detected Cu2+ (μM) |
Recovery (%) |
RSD (%) |
Lake water |
2 |
2.55 ± 0.09 |
127.50 |
1.76 |
4 |
4.31 ± 0.05 |
107.75 |
0.41 |
Drinking water |
2 |
2.48 ± 0.04 |
124.00 |
0.59 |
4 |
4.24 ± 0.08 |
106.00 |
0.74 |
Table 2 Detection of Zn2+ in real samples (n = 5)
Samples |
Added Zn2+ (μM) |
Detected Zn2+ (μM) |
Recovery (%) |
RSD (%) |
Lake water |
4 |
4.65 ± 0.42 |
108.14 |
3.02 |
7 |
7.60 ± 0.43 |
104.11 |
2.07 |
Zinc gluconate oral liquid |
0 |
4.12 ± 0.41 |
— |
3.90 |
2 |
6.09 ± 0.21 |
99.50 |
1.19 |
3.7. Fluorescence imaging in living cells
The application for Cu2+ and Zn2+ imaging in living cells was also investigated by confocal fluorescence microscopy. HeLa cells were first incubated with BBMP (10 μM) at 37 °C for 30 min, and subsequently incubated with 20 μM Cu2+ or Zn2+ and then observed under the confocal fluorescence microscope. Bright green fluorescence was observed in living cells incubated only with BBMP (Fig. 9a–c). Further incubation with Cu2+ caused a quenching of the green fluorescence (Fig. 9d–f), whereas a brilliant blue fluorescence was observed in living cells with further Zn2+ incubation (Fig. 9g–i). Thus the BBMP sensor is a promising sensor for Cu2+ and Zn2+ imaging in living cells.
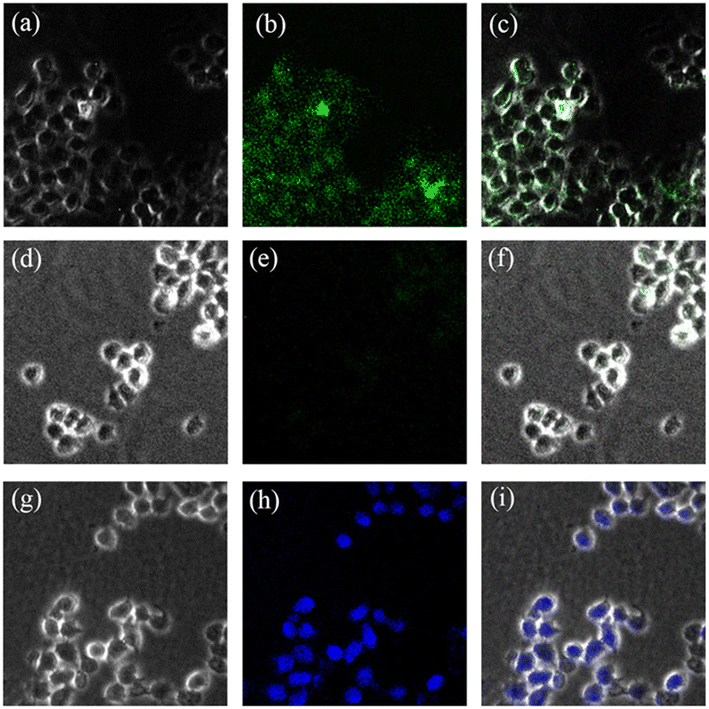 |
| Fig. 9 Bright-field (a, d and g), dark-field (b, e and h), and merged (c, f and i) images of living HeLa cells. The HeLa cells were incubated with BBMP (10 μM) (a–c), BBMP and further with Cu2+ (20 μM) (d–f), and BBMP and further with Zn2+ (20 μM) (g–i) at 37 °C for 30 min, respectively. | |
4. Conclusions
We successfully developed a new benzimidazole-derived fluorescence chemosensor BBMP for Cu2+ and Zn2+ detection in aqueous solution. The BBMP exhibited an ESIPT fluorescence emission, which was quenched upon binding to Cu2+ and Zn2+ due to an inhibition of ESIPT. Notably, the BBMP also showed an 82 nm blue-shifted fluorescence from the original ESIPT emission upon binding Zn2+, allowing the ratiometric detection. A Cu2+-selective turn-off and Zn2+-selective ratiometric turn-on fluorescent sensor was thus achieved. The binding mode was studied with Job's plot, mass analysis, and DFT calculation, where a 2
:
1 or 1
:
1 complex was formed between BBMP and Cu2+ or Zn2+ with imidazole N and phenolic O atoms coordination. The linear range and detection limit were found to be 0–5 μM and 0.16 μM for Cu2+, and 0–10 μM and 0.1 μM for and Zn2+, respectively. Furthermore, the sensor was also successfully used for Cu2+ and Zn2+ detection in real water samples and fluorescence imaging in living cells.
Author contributions
Wan-Yu Zhu: investigation, data curation, and writing – original draft. Kai Liu: writing – review & editing. Xuan Zhang: conceptualization, methodology, writing – review & editing, supervision, funding acquisition.
Conflicts of interest
The authors declare that they have no known competing financial interests or personal relationships that could have appeared to influence the work reported in this paper.
Acknowledgements
This work was financially supported by the Research Foundation from National Innovation Center of Advanced Dyeing & Finishing Technology (No. 2022GCJJ10).
References
- L. Li, J. Wang, S. Xu, C. Li and B. Dong, Recent progress in fluorescent probes for metal ion detection, Front. Chem., 2022, 10, 875241 CrossRef CAS PubMed.
- K. P. Carter, A. M. Young and A. E. Palmer, Fluorescent sensors for measuring metal ions in living systems, Chem. Rev., 2014, 114, 4564–4601 CrossRef CAS PubMed.
- M. H. Lee, J. S. Kim and J. L. Sessler, Small molecule-based ratiometric fluorescence probes for cations, anions, and biomolecules, Chem. Soc. Rev., 2015, 44, 4185–4191 RSC.
- P. Piyanuch, S. Wangngae, A. Kamkaew, W. Wattanathana, S. Wannapaiboon, S. Impeng, W. Maneeprakorn, V. Promarak and K. Chansaenpak, Ultrasensitive fluorogenic chemosensor based on ESIPT phenomenon for selective determination of Cu2+ ion in aqueous system and its application in environmental samples and biological imaging, Dyes Pigm., 2022, 205, 110532 CrossRef CAS.
- M. Raju, R. R. Nair, I. H. Raval, S. Haldar and P. B. Chatterjee, A water soluble Cu2+-specific colorimetric probe can also detect Zn2+ in live shrimp and aqueous environmental samples by fluorescence channel, Sens. Actuators, B, 2018, 260, 364–370 CrossRef CAS.
- E. A. B. Pajarillo, E. Lee and D. K. Kang, Trace metals and animal health: Interplay of the gut microbiota with iron, manganese, zinc, and copper, Animal, Nutrition, 2021, 7, 750–761 CAS.
- A. Prabhu and M. Gadgil, Trace metals in cellular metabolism and their impact on recombinant protein production, Process Biochem., 2021, 110, 251–262 CrossRef CAS.
- B. Vellingiri, A. Suriyanarayanan, P. Selvaraj, K. S. Abraham, M. Y. Pasha, H. Winster, A. V. Gopalakrishnan, J. K. Reddy, N. Ayyadurai, N. Kumar, B. Giridharan, K. R. S. S. Rao, S. K. Nachimuthu, A. Narayanasamy, I. Mahalaxmi and D. Venkatesan, Role of heavy metals (copper (Cu), arsenic (As), cadmium (Cd), iron (Fe) and lithium (Li)) induced neurotoxicity, Chemosphere, 2022, 301, 134625 CrossRef CAS PubMed.
- Y. Sun, W. Ding, J. Li, Y. Jia, G. Guo and Z. Deng, A novel and simple fluorescent chemical sensor SX based on AIE for relay recognition of Zn2+ and Cu2+ in aqueous system and analysis in logic gates, J. Mol. Struct., 2022, 1252, 132219 CrossRef CAS.
- B. Zha, S. Fang, H. Chen, H. Guo and F. Yang, An effective dual sensor for Cu2+ and Zn2+ with long-wavelength fluorescence in aqueous media based on biphenylacrylonitrile Schiff-base, Spectrochim. Acta, Part A, 2022, 269, 120765 CrossRef CAS PubMed.
- J. C. Qin and Z. Y. Yang, Fluorescent chemosensor for detection of Zn2+ and Cu2+ and its application in molecular logic gate, J. Photochem. Photobiol., A, 2016, 324, 152–158 CrossRef CAS.
- P. Ghorai, S. Banerjee, D. Nag, S. K. Mukhopadhyay and A. Saha, Design and synthesis of a novel fluorescent-colorimetric chemosensor for selective detection of Zn(II) and Cu(II) ions with applications in live cell imaging and molecular logic gate, J. Lumin., 2019, 205, 197–209 CrossRef CAS.
- Q. Niu, T. Sun, T. Li, Z. Guo and H. Pang, Highly sensitive and selective colorimetric / fluorescent probe with aggregation induced emission characteristics for multiple targets of copper, zinc and cyanide ions sensing and its practical application in water and food samples, Sens. Actuators, B, 2018, 266, 730–743 CrossRef CAS.
- H. Thomas, J. Naimhwaka, P. Endjala and V. Uahengo, ESIPT-influenced C3-symmetry, disk-shaped fluorescence turn-on probe for Zn2+ based on melamine-naphthyl moiety with high affinity towards Cu2+ in CH3CN, Results in Optics, 2022, 6, 100215 CrossRef.
- H. Fang, P. C. Huang and F. Y. Wu, A highly sensitive fluorescent probe with different responses to Cu2+ and Zn2+, Spectrochim. Acta, Part A, 2019, 214, 233–238 CrossRef CAS PubMed.
- J. S. Ganesan, S. Gandhi, K. Radhakrishnan, A. Balasubramaniem, M. Sepperumal and S. Ayyanar, Execution of julolidine based derivative as bifunctional chemosensor for Zn2+ and Cu2+ ions: Applications in bio-imaging and molecular logic gate, Spectrochim. Acta, Part A, 2019, 219, 33–43 CrossRef CAS PubMed.
- A. Jayaraj, M. S. Gayathri, G. Sivaraman and C. A. Swamy P, A highly potential acyclic Schiff base fluorescent turn on sensor for Zn2+ ions and colorimetric chemosensor for Zn2+, Cu2+ and Co2+ ions and its applicability in live cell imaging, J. Photochem. Photobiol., B, 2022, 226, 112371 CrossRef CAS PubMed.
- S. M. Hwang and C. Kim, Fluorescent detection of Zn2+ and Cu2+ by a phenanthrene-based multifunctional chemosensor that acts as a basic pH indicator, Inorg. Chim. Acta, 2018, 482, 375–383 CrossRef CAS.
- R. Arabahmadi, Antipyrine-based Schiff base as fluorogenic chemosensor for recognition of Zn2+, Cu2+ and H2PO4− in aqueous media by comparator, half subtractor and integrated logic circuits, J. Photochem. Photobiol., A, 2022, 426, 113762 CrossRef CAS.
- M. S. Kim, T. G. Jo, M. Yang, J. Han, M. H. Lim and C. Kim, A fluorescent and colorimetric Schiff base chemosensor for the detection of Zn2+ and Cu2+: Application in live cell imaging and colorimetric test kit, Spectrochim. Acta, Part A, 2019, 211, 34–43 CrossRef CAS PubMed.
- V. Kumar, U. Diwan, N. Tyagi, R. K. Mishra, M. K. Singh and K. K. Upadhyay, A coumarin based fluorescent probe enabling nanomolar detection of Zn2+ and Cu2+, J. Photochem. Photobiol., A, 2022, 425, 113692 CrossRef CAS.
- X. He, Q. Xie, J. Fan, C. Xu, W. Xu, Y. Li, F. Ding, H. Deng, H. Chen and J. Shen, Dual-functional chemosensor with colorimetric / ratiometric response to Cu(II) / Zn(II) ions and its applications in bioimaging and molecular logic gates, Dyes Pigm., 2020, 177, 108255 CrossRef CAS.
- J. C. Qin and Z. Y. Yang, Design of a novel coumarin-based multifunctional fluorescent probe for Zn2+/Cu2+/S2− in aqueous solution, Mater. Sci. Eng., C, 2015, 57, 265–271 CrossRef CAS PubMed.
- C. Liu, J. C. Qin, J. Xue, L. M. Tian, T. R. Li and Z. Y. Yang, Development of a novel fluorescent probe for Zn2+/Cu2+/S2− in different solutions based on benzocoumarin derivative, J. Photochem. Photobiol., A, 2019, 385, 112091 CrossRef CAS.
- Y. Wang, P. D. Mao, W. N. Wu, X. J. Mao, Y. C. Fan, X. L. Zhao, Z. Q. Xu and Z. H. Xu, New pyrrole-based single-molecule multianalyte sensor for Cu2+, Zn2+, and Hg2+ and its AIE activity, Sens. Actuators, B, 2018, 255, 3085–3092 CrossRef CAS.
- T. S. Aysha, M. B. I. Mohamed, M. S. El-Sedik and Y. A. Youssef, Multi-functional colorimetric chemosensor for naked eye recognition of Cu2+, Zn2+ and Co2+ using new hybrid azo-pyrazole / pyrrolinone ester hydrazone dye, Dyes Pigm., 2021, 196, 109795 CrossRef CAS.
- C. M. Pang, S. H. Luo, K. Jiang, B. W. Wang, S. H. Chen, N. Wang and Z. Y. Wang, A dual-channel sensor containing multiple nitrogen heterocycles for the selective detection of Cu2+, Hg2+ and Zn2+ in same solvent system by different mechanism, Dyes Pigm., 2019, 170, 107651 CrossRef CAS.
- X. Pei, Y. Pan, L. Zhang and Y. Lv, Recent advances in ratiometric luminescence sensors, Appl. Spectrosc. Rev., 2021, 56, 324–345 CrossRef CAS.
- S.-H. Park, N. Kwon, J.-H. Lee, J. Yoon and I. Shin, Synthetic ratiometric fluorescent probes for detection of ions, Chem. Soc. Rev., 2020, 49, 143–179 RSC.
- P. S. Kumar and K. P. Elango, A simple organic probe for ratiometric fluorescent detection of Zn(II), Cd(II) and Hg(II) ions in aqueous solution via varying emission colours to distinguish one another, Spectrochim. Acta, Part A, 2020, 241, 118610 CrossRef CAS PubMed.
- F. A. S. Chipem, S. K. Behera and G. Krishnamoorthy, Ratiometric fluorescence sensing ability of 2-(2′-hydroxyphenyl)benzimidazole and its nitrogen substituted analogues towards metal ions, Sens. Actuators, B, 2014, 191, 727–733 CrossRef CAS.
- Y. Wu, J. You, L. Guan, J. Shi, L. Cao and Z. Wang, Progress in the synthesis and application of benzimidazole-based fluorescent chemosensors, Chin. J. Org. Chem., 2015, 35, 2465–2486 CrossRef CAS.
- M. Qin, D. Jin, W. Che, Y. Jiang, L. Zhang, D. Zhu and Z. Su, Fluorescence response and detection of Cu2+ with 2-(2-hydroxyphenyl)benzimidazole in aqueous medium, Inorg. Chem. Commun., 2017, 75, 25–28 CrossRef CAS.
- L. Wang, X.-Y. Zheng, X. Zhang and Z.-J. Zhu, A quinoline-based fluorescent chemosensor for palladium ion (Pd2+)-selective detection in aqueous solution, Spectrochim. Acta, Part A, 2021, 249, 119283 CrossRef CAS PubMed.
- L. Wang, W.-Y. Zhu and X. Zhang, Selective and sensitive fluorescence detection of Pd (II) in 100% water and imaging application in living cells, Chin. J. Anal. Chem., 2022, 50, 100155 Search PubMed.
- S. Anbu, A. Paul, K. Surendranath, N. S. Solaiman and A. J. L. Pombeiro, A benzimidazole-based new fluorogenic differential/sequential chemosensor for Cu2+, Zn2+, CN−, P2O74−, DNA, its live-cell imaging and pyrosequencing applications, Sens. Actuators, B, 2021, 337, 129785 CrossRef CAS.
- X. Zhang, J.-Y. Liu, W.-W. Ma and M.-L. Yang, Near-infrared fluorescence of π-conjugation extended benzothiazole and its application for biothiol imaging in living cells, J. Mater. Chem. B, 2016, 4, 6662–6669 RSC.
- Y. Zhou, L. Zhang, X. Zhang and Z.-J. Zhu, Development of a near-infrared ratiometric fluorescent probe for glutathione using an intramolecular charge transfer signaling mechanism and its bioimaging application in living cells, J. Mater. Chem. B, 2019, 7, 809–814 RSC.
- X. Zhang, L. Zhang, W.-W. Ma, Y. Zhou, Z.-N. Lu and S. Xu, A near-infrared ratiometric fluorescent probe for highly selective recognition and bioimaging of cysteine, Front. Chem., 2019, 7, 32 CrossRef PubMed.
- K. Takagi, Y. Yamada, R. Fukuda, M. Ehara and D. Takeuchi, ESIPT emission behavior of methoxy-substituted 2-hydroxyphenylbenzimidazole isomers, New J. Chem., 2018, 42, 5923–5928 RSC.
- X. Zhang and J.-Y. Liu, Solvent dependent photophysical properties and near-infrared solid-state excited state intramolecular proton transfer (ESIPT) fluorescence of 2,4,6-tris- benzothiazolylphenol, Dyes Pigm., 2016, 125, 80–88 CrossRef CAS.
-
M. J. Frisch, G. W. Trucks, H. B. Schlegel, G. E. Scuseria, M. A. Robb, J. R. Cheeseman, G. Scalmani, V. Barone, B. Mennucci, G. A. Petersson, H. Nakatsuji, M. Caricato, X. Li, H. P. Hratchian, A. F. Izmaylov, J. Bloino, G. Zheng, J. L. Sonnenberg, M. Hada, M. Ehara, K. Toyota, R. Fukuda, J. Hasegawa, M. Ishida, T. Nakajima, Y. Honda, O. Kitao, H. Nakai, T. Vreven, J. A. Montgomery Jr., J. E. Peralta, F. Ogliaro, M. Bearpark, J. J. Heyd, E. Brothers, K. N. Kudin, V. N. Staroverov, T. Keith, R. Kobayashi, J. Normand, K. Raghavachari, A. Rendell, J. C. Burant, S. S. Iyengar, J. Tomasi, M. Cossi, N. Rega, J. M. Millam, M. Klene, J. E. Knox, J. B. Cross, V. Bakken, C. Adamo, J. Jaramillo, R. Gomperts, R. E. Stratmann, O. Yazyev, A. J. Austin, R. Cammi, C. Pomelli, J. W. Ochterski, R. L. Martin, K. Morokuma, V. G. Zakrzewski, G. A. Voth, P. Salvador, J. J. Dannenberg, S. Dapprich, A. D. Daniels, O. Farkas, J. B. Foresman, J. V. Ortiz, J. Cioslowski and D. J. Fox, Gaussian 09, Revision C.01, Gaussian, Inc., Wallingford CT, 2010 Search PubMed.
|
This journal is © The Royal Society of Chemistry 2023 |
Click here to see how this site uses Cookies. View our privacy policy here.