DOI:
10.1039/D3TB01287E
(Paper)
J. Mater. Chem. B, 2023,
11, 9163-9178
A new method for treating chronic pancreatitis and preventing fibrosis using bioactive calcium silicate ion solution†
Received
5th June 2023
, Accepted 14th August 2023
First published on 16th August 2023
Abstract
Chronic pancreatitis (CP) is a multifactorial fibroinflammatory syndrome. At present, there is no effective way to treat it clinically. In this study, we proposed a new approach by application of a highly active calcium silicate ion solution derived from calcium silicate (CS) bioceramics, which effectively inhibited the development of CP. This bioceramic derived bioactive ionic solution mainly regulated pancreatic acinar cells (PACs), macrophages and pancreatic stellate cells (PSCs) by SiO32− ions to inhibit inflammation and fibrosis and promote acinar regeneration. The possible mechanism of the therapeutic effect of CS ion solution mainly includes the inhibition of PAC apoptosis by down-regulating the c-caspase3 signal pathway and promotion of the regeneration of PACs by up-regulating the WNT/β-catenin signaling pathway. In addition, the CS ion solution also effectively down-regulated the NF-κB signaling pathway to reduce macrophage infiltration and PAC inflammatory factor secretion, thereby reducing PSC mediated pancreatic fibrosis. This bioceramics-based ion solution provides a new idea for disease treatment using biomaterials, which may have the potential for the development of new therapy for CP.
1. Introduction
Chronic pancreatitis (CP) is a kind of fibroinflammatory syndrome caused by many factors. Its main pathological features include atrophy, destruction of pancreatic acini and interstitial fibrosis.1 The clinical manifestations include recurrent epigastric pain, internal and external pancreatic secretion insufficiency and pancreatic duct stones, which ultimately lead to lower quality of life and shorter life expectancy of patients.1 The prevalence of CP in many countries has increased significantly in recent years.2,3 Furthermore, the risk of pancreatic cancer in CP patients has also been significantly increased, with the cumulative risk 10 and 20 years after CP of 1.8% and 4%, respectively.4,5 Unfortunately, there is no effective way to treat CP, and patients can only try to regulate their daily life by giving up drinking and smoking, thereby relieving their symptoms. In recent years, stem cell therapy and chemical small molecule drugs have been investigated to treat CP.6,7 Although some significant progress has been achieved, stem cell therapy has not been applied to clinical practice because of the serious risk of tumorigenicity after large-scale expansion in vitro,8 and small molecule drugs mainly improve the fibrosis of CP by inhibiting macrophage polarization and activation of pancreatic stellate cells (PSCs) through down-regulation of the MAPK pathway, but their clinical application is limited due to the toxicological factors.6,7 Therefore, it is necessary to develop a new safe and effective method to treat CP.
The challenge for CP treatment is mainly due to the complex pathogenesis. First, some harmful habits such as heavy drinking and smoking can cause damage and necrosis of pancreatic acinar cells (PACs).9 Then, the NF-κB inflammatory signal in PACs will be activated to induce an inflammatory cascade reaction and produce a large number of inflammatory factors.10,11 Next, the secreted inflammatory factors will cause continuous high expression of transforming growth factor-β (TGF-β),12 and activate the PSCs.13 Finally, the activated PSCs can induce a large amount of extracellular matrix deposition, promote the formation of pancreatic fibrosis, and ultimately lead to interlobular and intralobular fibrosis. In addition, the activated PSCs can also hinder the growth of PACs, lead to apoptosis of PACs, and gradually result in the atrophy of pancreas.13 Therefore, CP is a multifactorial injury disease, mainly involving harmful stimulus injury, persistent inflammatory reactions and fibrosis. Considering the complex pathogenesis, we speculate that a multi-functional agent may be more effective in treating CP, if it can regulate the whole microenvironment in the damaged part of the pancreas including inhibiting inflammation, PAC apoptosis and fibrosis, and promoting tissue regeneration simultaneously.
Silicate bioceramic is a special bioactive material and is widely used in the repair of many different tissues.14,15 Huang et al.16 found that the SiO32− released from nagelschmidtite can reduce the inflammatory response of macrophages and promote bone regeneration by inhibiting the activation of the NF-κB signaling pathway. Because the NF-κB signaling pathway is the core pathway in regulating the inflammatory response of CP,6,7,10,11 we hypothesize that silicate bioceramics may also inhibit the inflammatory response of CP by regulating this signaling pathway. In addition, many studies showed that SiO32− can enhance the cell viability of umbilical vein endothelial cells, bone marrow mesenchymal stem cells, myocardial cells, alveolar epithelial cells and other soft tissue related cells. Its unique bioactivity not only effectively prevents cell apoptosis but also promotes the repair and regeneration of various tissues.17–19 Yi et al.20 developed SiO32− ion therapy to treat myocardial infarction, which significantly reduced fibrosis and repaired myocardium at the injured site. Considering that SiO32− has unique effects in enhancing regeneration of soft tissues such as myocardium and skin, we also hypothesize that it may also promote pancreatic regeneration in the therapy of CP.
In order to verify this hypothesis, we use the most typical silicate bioceramic, calcium silicate (CS), to prepare bioactive CS ion solution and establish CP animal models in mice to observe the therapeutic effects of CS ion solution. Furthermore, the effects of CS ion solution on apoptosis and regeneration of PACs, macrophage polarization and inflammatory reactions, activation of PSCs and intercellular interactions were evaluated to explore the possible mechanism in treating CP (Scheme 1).
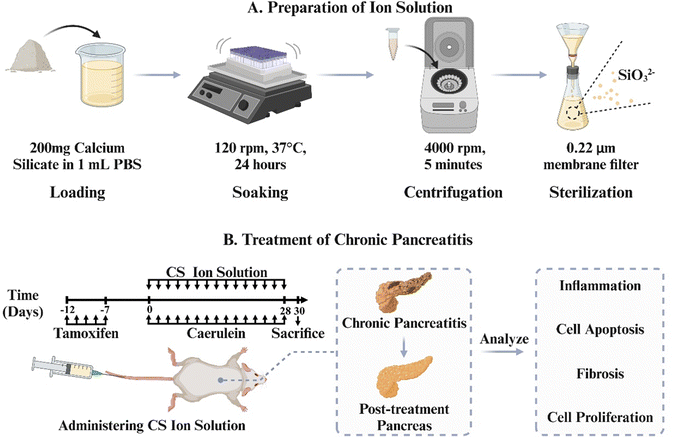 |
| Scheme 1 (A) Preparation of ion solution. CS powders are soaked in PBS to prepare the CS ion solution. (B) Treatment of chronic pancreatitis. Chronic pancreatitis in mice was treated by administering the CS ion solution via tail vein injection to inhibit inflammation, cell apoptosis, and fibrosis, while promoting cell proliferation. | |
2. Materials and methods
2.1. Preparation of calcium silicate bioceramics
Calcium silicate bioceramics were synthesized using a chemical coprecipitation method. Calcium nitrate tetrahydrate (Ca(NO3)2·4H2O) and sodium metasilicate nonahydrate (Na2SiO3·9H2O) were employed as raw materials and obtained from Sinopharm Chemical Reagent Co., Ltd, Shanghai, China. In brief, 59.04 g of Ca(NO3)2·4H2O was dissolved in 500 mL of deionized water. Simultaneously, 71.05 g of Na2SiO3·9H2O was also added to 500 mL of deionized water. After vigorous stirring for 24 hours at room temperature, the mixture was filtered to collect precipitates. The precipitates were then washed three times with deionized water and ethanol, followed by drying at 60 °C for 24 hours. Subsequently, the dried material was calcined at 800 °C for 3 hours and finally ground to obtain the calcium silicate bioceramics.
2.2. Preparation of calcium silicate (CS) ion solution
The ion extracts of CS were prepared according to the previously reported protocol, which was adapted from the standard procedure in ISO10993-1.21–23 Briefly, CS powders were added into the serum-free Dulbecco's modified Eagle's medium (DMEM; Gibco) or PBS (Gibco) at a solid/liquid ratio of 200 mg mL−1 at 37 °C for 24 hours. Then, the mixture was centrifuged at 4000 rpm for 5 min. The supernatant was collected and sterilized through a filter membrane (Millipore; 0.22 μm) to obtain CS ion solution. ICP-AES was employed to determine the concentrations of Ca2+ and SiO32− ions in the CS solution. Serial dilutions of CS ion solution (1/2, 1/4, 1/8, 1/16,1/32, 1/64, 1/128, and 1/256) were prepared by using the acinar DMEM medium (containing 2.5% FBS, 1% penicillin–streptomycin mixture (PS), and 0.25 mg mL−1 of trypsin inhibitor) or the DMEM supplemented with 10% FBS and 1% PS for cell experiments.
2.3. Establishment, treatment and performance evaluation of the animal model
LSL-K-RasG12D mice (LSL) and Ela-CreERT (Cre) mice were obtained from the University of Texas MD Anderson Cancer Centre. Cre mice express tamoxifen-regulated CreERT specifically in pancreatic acinar cells (PACs) under the control of a full-length elastase gene promoter. To specifically express K-RasG12D in PACs, LSL mice were bred with Cre mice to generate LSL-K-Ras/Ela-CreERT double-transgenic mice (LSL/Cre). Cre and LSL/Cre mice (23–29 g, 14–18 weeks old) randomly divided into the following four groups after matching body weight: (1) Cre PBS group: Cae + PBS, (2) Cre CS group: Cae + CS, (3) LSL/Cre PBS group: Cae + PBS, and (4) LSL/Cre CS group: Cae + CS. Mice in each group were given tamoxifen (3 mg/40 g body weight) for 5 days to induce CreERT gene expression and injected caerulein into an abdominal cavity (4 hourly of 50 μg kg−1, every 2 d for 4 weeks, Cae) after 7 days of rest. CS (8 μL of CS stock solution per unit mouse body weight, every 2 d for 4 weeks) was given 1 hour after the last injection of caerulein. The dosing regimen for the CS solution in this study was determined based on our previous work.20 Mice were then sacrificed and analyzed 2 days after the last caerulein injection. Ethical approval for experimental procedures and the handling of mice had been approved by the Animal Care Committee of Changhai hospital.
2.4. Histology and immunohistochemistry staining
Tissues were immediately fixed in 4% paraformaldehyde (PFA) and embedded in paraffin. The 5 μm sections were taken and stained with haematoxylin and eosin(H&E) for histopathological evaluation. For acinar atrophy and fibrosis of CP, a pathologist unaware of the origin of the specimens scored the degree of pancreatic injury by light microscopy based on a previously published study.24 Immunohistochemical staining for Sirius Red staining (ab150681, Abcam), Ki67(ab15580, Abcam) and β-catenin (A19657, ABclonal) was performed in pancreatic paraffin sections. IHC staining was performed for infiltrating macrophages by F4/80 antibodies. Quantification of the staining was performed using the ImageJ analysis software.
2.5. Analysis of TUNEL staining
The apoptosis of mouse pancreas acinar cells (PACs) was detected by TUNEL staining. The pancreatic tissues were harvested after surgery and fixed in 4% paraformaldehyde at room temperature before embedding in paraffin. Pancreas samples were sectioned to 5 μm and then stained using the TUNEL Apoptosis Assay Kit (Novazan, Jiangsu, China) according to the manufacturer's protocol. Cells were counterstained with DAPI (blue) and TUNEL positive nuclei (green). A fluorescence microscope (Olympus, Japan) was used to observe the apoptosis of PACs. Three fields were randomly selected for the total nuclei and TUNEL+ nuclei counts in each section, and the index of apoptosis (number of TUNEL+ nuclei/total number of nuclei × 100%) was calculated.
2.6. Cell isolation and culture
PACs were freshly prepared from LSL/Cre mice using a collagenase digestion method as previously described.25 In short, after washing twice in Hank's Balanced Salt Solution (HBSS), the mouse pancreas was quickly dissected and cut into small pieces of 1 to 3 mm3. Then, 10 mL of collagenase IA solution (HBSS 1× containing 10 mM HEPES, 200 U mL−1 of collagenase IA, and 0.25 mg mL−1 of trypsin inhibitor) was added to digest pancreas sections. When the pancreatic tissue was completely separated, the enzymatic reaction was stopped with cold buffer washing solution (HBSS 1× containing 5% fetal bovine serum (FBS) and 10 mM HEPES). The cell mixture was filtrated using a 100 μm filter and seeded into 6-well plates with the DMEM medium, containing 2.5% FBS, 1% penicillin–streptomycin mixture (PS), and 0.25 mg mL−1 of a trypsin inhibitor. Primary pancreatic acinar cells were used for the next experiment. Primary mice pancreatic stellate cells (PSCs) were isolated from mice pancreas obtained from iCell (MIC-iCELL-g003). In short,26 the mouse pancreatic tissue was extracted using an aseptic procedure; after cleaning with the fetal bovine serum (Gibco), the pancreatic tissues were cut into 0.5–1 mm3 tissue blocks and cultured in 6-well plates. The culture medium was changed on the second day. After the primary PSCs crawled out, the tissue blocks were placed in a new 6-well plate for further culture, and the primary PSCs were cultured and passed. PSCs below passages 2–5 were used in this study. Raw 264.7 cells were obtained from the American Type Culture Collection (ATCC, VA, USA). PSCs and Raw 264.7 cells were cultured with the DMEM supplemented with 10% FBS and 1% PS in a humid atmosphere of 5% CO2 at 37 °C. The culture medium was changed every other day. Each generation of Raw 264.7 cells could be used for further experiments.
2.7. Cell viability
PACs (3 × 105 per well), PSCs (1 × 104 per well), and Raw 264.7 cells (1 × 104 per well) were seeded into 96-well plates with the prepared culture medium containing diluted CS ions solution at different concentrations (1,1/2, 1/4, 1/8, 1/16,1/32, 1/64, 1/128, and 1/256). At the pre-set time point, the culture medium was removed by the fresh culture medium containing CCK8 assay (Topscience, China) solution (10
:
1) and cultured at 37 °C for another 2 hours. The absorbance of the as-mentioned medium was measured using a microplate reader (SpectraMax, Molecular Devices) at a wavelength of 450 nm.
2.8. Quantitative real-time PCR
Total RNA was extracted from cells or mouse pancreas tissues using the Trizol reagent (Novazan, China) and the concentration was measured using a nanodrop 1000 reader (Thermo Scientific). Then, 1 μg of RNA was reverse transcribed into cDNA using the reverse transcription kit (Novazan, China). Highly purified primers were commercially synthesized (Shenggong, Shanghai, China) and the qRT-PCR was performed in triplicate using a Light Cycler® 480 II System (Roche, Sandhofer, Germany). The primer sequences are listed in Table S1 (ESI†).
2.9. Western blotting
Cells were rinsed with cold PBS and isolated using RIPA lysis buffer (Beyotime Biotechnology, China) according to the standard protocol.27 Protein contents were determined using the BCA kit (Shanghai Epizyme Biomedical Technology, China). The total proteins from 20 mg of samples were loaded onto 4–20% polyacrylamide gels and transferred to the PVDF membrane (Merck Millipore, Darmstadt, Germany). After soaking in a closed buffer at room temperature for 1 hour, the membrane was then incubated with various primary antibodies overnight at 4 °C, and then incubated with secondary antibodies for 1 hour. Anti-tubulin was used as the loading control. The target proteins were then visualized using the ECL system (Amersham Imager 600, GE). Primary antibodies used in this study were described as follows: NF-κB p65 (Cell Signaling Technology CST, #8242, 1
:
1000), p-NF-κB p65 (CST, #3033, 1
:
1000), IL-1β (CST, #12242, 1
:
1000), β-catenin (A19657, ABclonal, 1
:
1000), GSK3β (CST, #12456, 1
:
1000), p-GSK3β (ABclonal, AP1088, 1
:
1000), caspase-3 (ABclonal, AP11040, 1
:
1000), cleaved caspase-3 (CST, #9664, 1
:
1000), β-tubulin (GenScript, 2G7D4, 1
:
1000), α-SMA (CST, #19245, 1
:
1000), and Col1α1 (CST, #72026, 1
:
1000).
2.10. Immunofluorescence staining of α-SMA and Col1α1
Cell slides were fixed in 4% paraformaldehyde for 30 min, rinsed with PBS three times, permeabilized with 1% Triton X-100 for 30 min, blocked with 5% BSA at 37 5% BSA at 37 three times, pean antibody Col1α1 (CST, #72026, 1
:
100) and an anti-α-smooth muscle actin antibody (CST, #19245, 1
:
100) overnight at 4 °C in a dark humidity chamber. Next, the cell slides were incubated with the secondary antibody (Alexa Fluor 488 goat anti-rabbit IgG or Cy3 goat anti-rabbit (Servicebio, China)) in the dark at room temperature for 2 hours, the nuclei were stained with DAPI, and the cell slides were sealed with glycerol. Finally, glycerol was used to seal the cell slides. Cells were observed using a fluorescence microscope (Olympus, Japan).
2.11. Effects of CS ion solution on regeneration, apoptosis and expression of inflammatory factors of pancreatic acinar cells (PACs)
First, PACs (1.5 × 106 cell per well) were seeded into 6-well plates, and stimulated with 10 nM mL−1 cholecystokinin (CCK) for 30 min to induce the injured model. The injured PACs were cultured with the CS ion extract (1/16 CS and 1/64 CS) or the control DMEM. The expressions of β-catenin, GSK3β, p-GSK3β, caspase3, c-caspase3, t-P65, p-P65 and IL-1β were evaluated by western blot, and the expressions of IL-1β, IL-6, TNF-α and TGF-β were evaluated by the qRT-PCR.
2.12. Effects of CS ion solution on inhibiting the NF-κB signaling pathway in macrophages (Raw 264.7)
Raw 264.7 cells (1 × 105 cells per well) were seeded into 6-well plates and 100 ng mL−1 LPS (Sigma, USA) was used for 1 h to induce M1 polarized macrophages. Then, the cell cultured medium was changed as CS ion solution (1/8CS and 1/16CS) or the control DMEM. After 24 h, the cells were harvested and the expressions of t-P65, p-P65 and IL-1β were evaluated by western blot and the expressions of IL-1β, IL-6, TNF-α and iNOS were evaluated by the qRT-PCR.
2.13. CD68 and CD206 expressions of Raw 264.7 cells evaluated by flow cytometry
Raw 264.7 cells were stimulated with LPS (100 ng mL−1) for 1 h and incubated with the DMEM (Ctrl), DMEM + 1/8CS (CS), PSC conditioned supernatant (PSC CM) and PSC conditioned supernatant after 1/8CS treatment for 24 h (PSC CM + CS), respectively. In particular, PSC CM and PSC CM + CS were extracted from PSCs incubated with the DMEM and DMEM + 1/8CS for 24 hours, respectively. The collected Raw 264.7 cells were washed and stained with surface markers APC anti-mouse CD68 (BioLegend, USA) and PE anti-mouse CD206 (BioLegend, USA) at 4 °C for 30 min. Data were obtained using a NovoCyte flow cytometer (Agilent, China) and analysed using the NovoExpress Software (Agilent, China).
2.14. Expressions of α-SMA and Col1α1 in injured PACs and the PSC co-cultured system
PSCs (4 × 104 cells per well) in the logarithmic growth phase were seeded into 6-well plates or polylysine-treated cell slides. After 12 hours, PSCs were divided into the following four groups: (1) ctrl group, (2) CS group (1/64CS ion solution), (3) PACs group (PSCs co-cultured with injured PACs), and (4) PACs + CS group (PSCs co-cultured with injured PACs and cultured with 1/64CS ion solution). PACs were stimulated with 10 nM mL−1 cholecystokinin (CCK) for 30 min to induce the injured model, and co-cultured with PSCs in a 1
:
1 ratio. The cells in the 6-well plates were collected to evaluate the expressions of α-SMA and col1α1 by western blot. The cells in polylysine-treated cell slides were collected for immunofluorescence staining (α-SMA and Col1α1).
2.15. Expressions of α-SMA and Col1α1 in M1 conditioned supernatant and the PSC co-cultured system
PSCs (4 × 104 cells per well) in the logarithmic growth phase were seeded into 6-well plates or polylysine-treated cell slides. After 12 hours, PSCs were divided into the following four groups: (1) ctrl group, (2) CS group (PSCs cultured with 1/8CS ion solution), (3) Raw group (PSCs cultured with the M1 conditioned supernatant), and (4) Raw + CS group (PSCs cultured with the M1 conditioned supernatant after 1/8CS treatment for 24 h). The cells in the 6-well plates were collected to evaluate the expressions of α-SMA and col1α1 by western blot. The cells in polylysine-treated cell slides were collected for immunofluorescence staining (α-SMA and col1α1).
2.16. Biosafety evaluation of CS ion solution
Organs such as heart, liver, spleen, lungs and kidneys were collected after CS treatment for one month. The organs were chemically digested in concentrated nitric acid (spectral purity) and used inductively coupled plasma mass spectrometry (ICP-MS; PerkinElmer NexION 350×, USA) to determine the accumulation and distribution of SiO32− ions in these important organs.
2.17. Statistical analysis
The GraphPad Prism software 7.0 was used to analyze statistics. Data were presented as mean ± standard deviation. The Shapiro Wilk normality test was used for the normality test. The statistical significance between two groups was determined by Student's t test. One-way ANOVA was used for comparison between groups that conform to the normal distribution, Tukey's multiple comparisons test was used for comparison between groups, and the Kruskal–Wallis test and Dunn's multiple comparisons test were used for comparison that do not conform to the normal distribution. P < 0.05 represents a statistical difference (** stands for P < 0.01, * stands for P < 0.05, NS stands for P > 0.05).
3. Results
3.1. The ion concentration in the CS solution
The ion concentrations in the prepared CS solutions were assessed using ICP-AES, and the results are presented in Table S2 (ESI†). The concentrations of SiO32− ions in the CS solution were determined to be 119.2 ± 0.74 μg mL−1 in PBS and 121.2 ± 0.69 μg mL−1 in the DMEM. Furthermore, the concentrations of Ca2+ ions in the CS solution were found to be 0.05 ± 0.03 μg mL−1 in PBS and 5.2 ± 0.05 μg mL−1 in the DMEM. These values align with the ion concentrations reported in our previous study.20 Importantly, our previous studies have demonstrated that the CS solution at this concentration does not induce any toxic side effects in mice and exhibits favorable biological activity. Based on these findings, we employed this concentration of CS solution for our in vivo animal experiments.
3.2. CS ion solution inhibited acinar atrophy and fibrosis in mice with various degree chronic pancreatitis (CP)
To test our hypothesis, the CP model was constructed by injecting caerulein (Cae) in elastase-CreERT transgenic tool mice (Cre) and elastase-CreERT + KrasG12D dual transgenic mice (LSL/Cre). In particular, the pancreatitis model constructed in LSL/Cre mice is more severe and irreversible than that in the ordinary mice (Cre), and its phenotype is more stable.28 Therefore, we used Cre and LSL/Cre mice to construct mild and severe chronic pancreatitis mouse models to verify the therapeutic effect of CS. The injection protocol of caerulein (Cae) and calcium silicate ion solution (CS) is shown in Scheme 1. It is clear to see that, with the development of CP, the body weight of LSL/Cre mice decreased gradually in the PBS group. In contrast, the weight of Cre mice did not change significantly. This may be due to the fact that CP is more severe in LSL/Cre mice than in Cre mice, which makes the weight loss trend more obvious. Interestingly, we found that the treatment of CS ion solution prevented the weight loss of the mice significantly in LSL/Cre mice (Fig. S1, ESI†). In the optical photographs, we clearly observed that CS ion solution effectively reduced the atrophy of pancreas in both Cre and LSL/Cre mice (Fig. 1A). Quantitative analysis also showed that, compared with the CS group, the percentage of the pancreas weight/body weight in the PBS group was significantly lower (0.37 ± 0.03% in Cre mice and 0.51% ± 0.03% in LSL/Cre mice). More importantly, the percentage of pancreas weight/body weight in the CS group was close to the normal value (1%),27 which indicated that CS ion solution can alleviate pancreatic injury during CP (Fig. 1B). To further verify the effect of CS ion solution on CP, the histopathological changes of pancreas in each group of mice were examined by H&E staining. The results showed that, compared with the CS group, there were a large number of inflammatory cells infiltrating the pancreas in the PBS group, resulting in fibrotic lesions, and acinar tissues began to atrophy (Fig. 1C). Furthermore, CS ion solution significantly reduced Sirius red positive staining in pancreatic tissues compared with the PBS group, indicating that CS ion solution effectively inhibited the excessive deposition of collagen to avoid pancreatic fibrosis (Fig. 1D). Besides, the histological score in the CS group was more than two times lower than that in the PBS group (Fig. 1E), and the percentage of the collagen positive area in the CS group was also more than three times lower than that in the PBS group (CS: 2.6 ± 0.29% vs. PBS: 19.17 ± 1.14% in Cre mice, CS: 7.04 ± 1.13% vs. PBS: 23.57 ± 1.17% in Cre/LSL mice, Fig. 1F). Meanwhile, our data showed that the mRNA levels of fibrosis related genes (fibronectin, α-SMA, Col1α1 and TGF-β) were also significantly reduced in the CS group in both Cre and LSL/Cre mice (Fig. S2, ESI†).
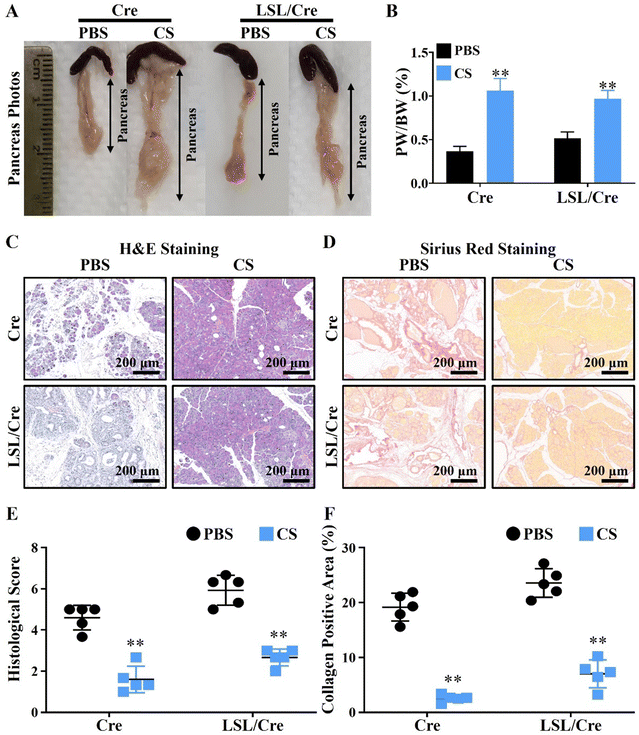 |
| Fig. 1 Treatment of chronic pancreatitis (CP) in Cre and LSL/Cre mice using CS ion solution and its therapeutic effects. (A) Representative photographs of the pancreas collected on the 30th day in CP mice with or without CS ion solution treatment (CS: CS ion solution treatment). (B) Percentage of pancreas weight/body weight in Cre and LSL/Cre CP mice on the 30th day (day of sacrifice). (C) Representative images of H&E staining of pancreas. (D) Representative images of Sirius red staining of pancreatic tissues. (E) Pathological score of H&E staining. (F) Quantitative analysis of Sirius red positive staining to assess the degree of pancreatic fibrosis (** p < 0.01 compared with the PBS group; n = 5). | |
3.3. CS ion solution inhibited the apoptosis of pancreas acinar cells (PACs) and promoted regeneration
Since CS ion solution significantly reduced acinar atrophy in Cre and LSL/Cre mice, we further explored the effect of CS on the apoptosis and regeneration of PACs. TUNEL staining was used to identify apoptotic PACs and Ki67 staining was used to label the regenerated PACs. Interestingly, we found that CS ion solution significantly reduced TUNEL positive cells (Fig. 2A) and increased Ki67 positive cells in both Cre and LSL/Cre mice (Fig. 2B). Especially, in the LSL/Cre group, CS reduced the percentage of TUNEL positive cells from 15.25% to 2.99%, and increased the percentage of Ki67 positive cells from 6.00% to 31.86% (Fig. 2C and D). Since β-catenin in the typical WNT signaling pathway is essential for the proliferation of PACs after injury,29 the expression of β-catenin in Cre and LSL/Cre CP mice was also examined in this study. In comparison to the PBS group, CS ion solution significantly increased β-catenin expression (Fig. 2E and F), which suggested that CS ion solution may regulate PAC regeneration through the regulating Wnt/β-catenin pathway.
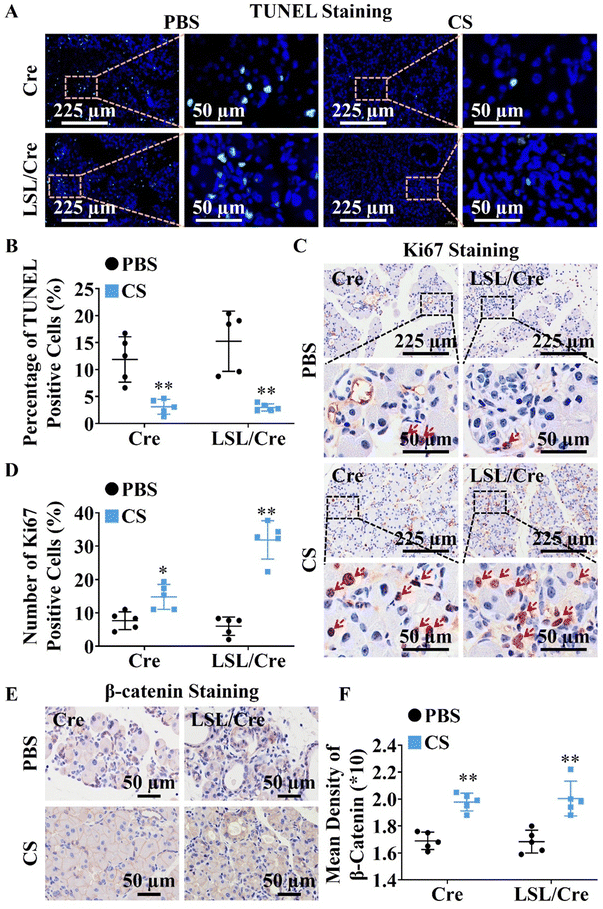 |
| Fig. 2 Effects of CS ion solution on apoptosis and proliferation of pancreatic acinar cells (PACs). (A) Representative images pf TUNEL staining of pancreatic tissues (green: apoptotic PACs). (B) The number of apoptotic PACs was quantitatively analyzed according to TUNEL staining. (C) Representative images of Ki67 staining of pancreatic tissues (red: proliferated PACs). (D) The number of proliferated PACs was quantitatively analyzed according to Ki67 staining. (E) Representative images of β-catenin staining of pancreatic tissues (brown: β-catenin positive staining; the darker brown color indicates higher β-catenin expression, which means the stronger promoting effect on the proliferation of PACs.). (F) Quantitative analysis of the promoting effect on the proliferation of PACs based on β-Catenin staining (* p < 0.05 compared with the PBS group; ** p < 0.01 compared with the PBS group; n = 5). | |
3.4. CS ion solution inhibited infiltration and M1 polarization of macrophages
Macrophages are closely related to the progression of pancreatic fibrosis.30,31 Therefore, F4/80 staining was used to evaluate the effect of CS ion solution on inhibiting macrophage infiltration. Interestingly, it is clear to see that CS ion solution significantly decreased F4/80 positive cells in both Cre and LSL/Cre mice (Fig. 3A). Quantitative analysis showed that, CS reduced the F4/80 positive staining area of Cre mice by more than 9.8 times and Cre/LSL mice by more than 7.3 times compared with the PBS group (Fig. 3B). In addition to macrophage infiltration, the effect of CS ion solution on macrophage polarization was determined by the qRT-PCR. It is clearly observed that, compared with the PBS group, the mRNA levels of M1 macrophage-associated genes including interleukin-1β (IL-1β), interleukin-6 (IL-6), tumor necrosis factor-α (TNF-α), and inducible nitric oxide synthase (iNOS) were significantly decreased (Fig. 3C–F), while M2 macrophage-associated genes including arginase 1 (Arg1), macrophage mannose receptor 1 (CD206), and C-type lectin domain family 10 member (CD301) did not change after treatment with CS ion solution (Fig. 3G–I). These results indicate that CS ion solution effectively inhibited infiltration and M1 polarization of macrophages.
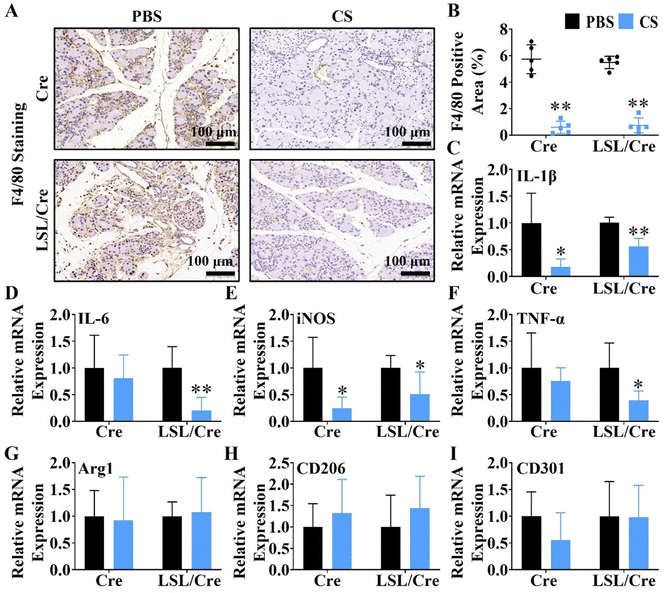 |
| Fig. 3 CS ion solution reduces macrophage infiltration and inhibits the polarization of macrophages into M1 phenotype. (A) Representative images of F4/80 staining of mouse pancreatic tissues (brown: positive staining of F4/80; the darker brown color indicates more macrophage infiltration and stronger inflammation). (B) Quantitative analysis of the proportion of inflammatory cell infiltration according to F4/80 staining. (C)–(F) qRT-PCR analysis of the expression of macrophage M1 phenotypic related genes (IL-1β, IL-6, iNOS and TNF-α) in pancreatic tissues. (G)–(I) qRT-PCR analysis of the expression of M2 phenotypic related genes (Arg1, CD206 and CD301) in pancreatic tissues (* p < 0.05 compared with the PBS group; ** p < 0.01 compared with the PBS group; n = 5). | |
3.5. The regulatory effect of CS on CP-related cell viability
To further explore the biological mechanism of CS in treating CP, the cell viability of CP's pathogenesis related cells, including pancreatic acinar cells (PACs), macrophages (Raw264.7) and pancreatic stellate cells (PSCs), was detected in the CS ion solution with different concentrations. The results revealed that the cell viability of PACs was increased after being treated for 24 h in media containing CS ion solution with 1/8, 1/16, 1/32, and 1/64 dilutions. Similarly, 1/4, 1/8, 1/16, 1/32 and 1/64 CS dilutions of the CS ion solution significantly stimulated the cell viability of Raw 264.7 after 24 h and 48 h. This result suggests that CS ion solution can promote the viability of PACs and RAW 264.7 in a wide concentration range. In addition, CS ion solution did not promote the cell viability of PSCs compared to the control group (Fig. S3, ESI†). Furthermore, the cell viability of all three types cells was inhibited under the original concentration of CS ion solution, which was possibly due to its high pH value.23,32
3.6. CS ion solution affected the regeneration and apoptosis of PACs by regulating the Wnt/β-catenin signaling pathway and apoptosis-related proteins
In order to further explore the mechanism of CS ion solution in promoting the regeneration of PACs, we investigated the effect of CS ion solution on Wnt/β-catenin, a signaling pathway related to pancreatic acinar regeneration,29 by western blot in vitro. Since 1/16CS and 1/64CS have the highest cell viability, these two concentrations were used to intervene PACs under cholecystokinin damage conditions. Interestingly, we found that 1/16 and 1/64 dilutions of CS ion solutions significantly inhibited the phosphorylation of GSK3β in PACs, but stimulated the expression of nonphosphorylated GSK3β and β-catenin (Fig. 4A and B). This result suggests that CS ion solution could inhibit the hydrolysis of β-catenin by phosphorylated GSK3β, leading to the accumulation of β-catenin, thereby promoting acinar regeneration. In addition, we found that the cleaved caspase-3 (c-caspase-3) protein in PACs was decreased by 1/16 and 1/64 dilutions of CS ion solution (Fig. 4C and D), indicating that CS ion solution reduces the apoptosis of PACs possibly by down-regulating the c-caspase-3 protein. Since a nuclear factor kappa B (NF-κB) is a transcription factor involved in cell apoptosis,33 we believed that CS ion solution may mediate the apoptosis of PACs through this signaling pathway. We found that 1/16 and 1/64 dilutions of CS ion solution indeed reduced the expression of phosphorylated NF-κB-P65 and IL-1β in PACs after cholecystokinin stimulation (Fig. 4E and F). Considering that the 1/64 dilution of CS ion solution had the strongest inhibitory effect on NF-κB protein expression in PACs, we chose this concentration to further evaluate the regulatory effect of CS ion solution on inflammatory cytokines. It was found that CS ion solution reduced the mRNA levels of inflammatory cytokines (IL-1β, IL-6, TNF-α and TGF-β) in PACs (Fig. 4G). Studies have shown that the activation of the NF-κB signaling pathway is the key cause of PAC injury, and after injury PACs will secrete monocyte chemoattractant protein-1 (MCP1) to recruit macrophages.34 However, CS ion solution effectively inhibited the NF-κB signaling pathway and reduced the secretion of inflammatory factors in PACs, which might be another reason why macrophage infiltration in pancreatic tissues was reduced after the treatment of CS ion solution.
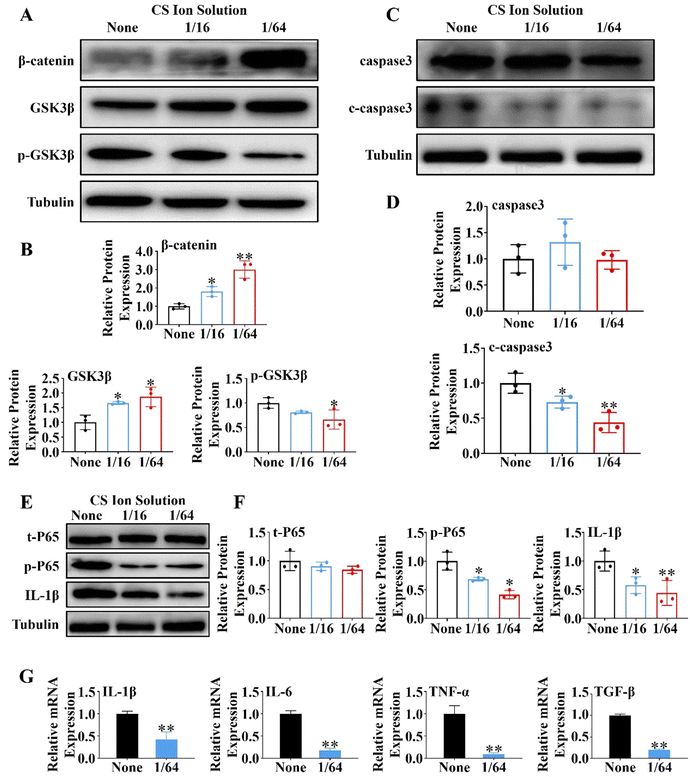 |
| Fig. 4 Effects of CS ion solution on proliferation, apoptosis and expression of inflammatory factors of pancreatic acinar cells (PACs). (A) and (B) The western blot expression and quantitative analysis of β-catenin, GSK3β and p-GSK3β proteins in PACs cultured with different concentrations of CS ion solution under the condition of CCK stimulation. (C) and (D) The western blot expression and quantitative analysis of caspase3 and c-caspase3 proteins in PACs cultured with different concentrations of CS ion solution under the condition of CCK stimulation. (E) and (F) The western blot expression and quantitative analysis of t-P65, p-P65 and IL-1β proteins in PACs cultured with different concentrations of CS ion solution under the condition of CCK stimulation. (G) qPCR detection of the expression of inflammatory cytokines IL-1β, IL-6, TNF-α and TGF-β in PACs intervened with different concentrations of CS ion solution (* p < 0.05 compared with the none group; ** p < 0.01 compared with the none group; n = 3). | |
3.7. CS ion solution directly or indirectly affected macrophage polarization through the NF-κB signaling pathway and intercellular communication
It is well-known that NF-κB signaling plays an essential role in immune regulation and macrophage polarization.35,36 Here, we used 1/8 and 1/16 dilutions of CS ion solution (both concentrations were the most effective concentration for promoting Raw 264.7 cell viability) to treat Raw 264.7 cells induced by lipopolysaccharide (LPS). The results revealed that the IL-1β and phosphorylation of NF-κB-P65 (p-P65) were significantly inhibited by 1/8 and 1/16 dilutions of CS ion solution, while the nonphosphorylated NF-κB-P65 (t-P65) was not affected (Fig. 5A–D). In particular, the 1/8 dilution of CS ion solution had the strongest inhibitory effect on the NF-κB signaling pathway, so that this concentration was used in subsequent experiments. Interestingly, we found that the 1/8 dilution of CS ion solution significantly decreased the mRNA levels of IL-1β, TNF-α and iNOS in Raw 264.7 cells (Fig. 5E). Above results suggest that CS ion solution may regulate M1 polarization of macrophages by inhibiting the NF-κB signaling pathway. To further clarify the direct and indirect effects of CS ion solution on macrophage polarization, M1-type macrophages induced by LPS were treated with the DMEM, 1/8CS, PSC conditioned medium (PSC CM) and PSC CM after 1/8CS treatment for 24 h (PSC CM + CS). Then, flow cytometry was performed to detect the expression of macrophage surface markers (CD68 and CD206). As shown in Fig. 5F–I and Fig. S4 (ESI†), 1/8CS decreased the expression of the M1 marker (CD68: from 90.44 ± 1.39% to 59.1 ± 2.63%) and increased the expression of the M2 marker (CD206: from 23.91 ± 1.0% to 53.89 ± 3.53%), which was consistent with our previous findings in bone regeneration related studies.37 Similarly, PSC CM also decreased the expression of the M1 marker (CD68: from 90.40% to 71.04 ± 3.64%) but not changed the expression of the M2 marker. Interestingly, PSC CM + CS decreased both the M1 marker and the M2 marker (CD68: from 90.40% to 65.98 ± 4.29%; CD206: from 28.37 ± 1.86% to 13.61 ± 1.68%). These results showed that CS ion solution could directly reduce the polarization of M1 macrophages by inhibiting the NF-κB signal pathway, and indirectly reduce the polarization of M2 macrophages through regulating intercellular communication between PSCs and macrophages.
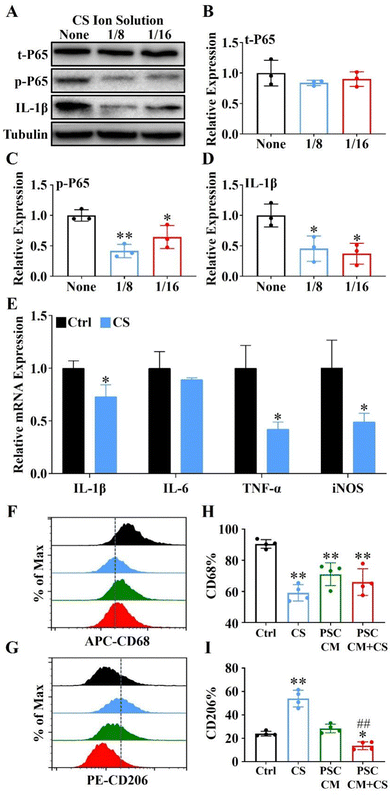 |
| Fig. 5 Inhibition of the NF-κB signaling pathway using CS ion solution reduced the secretion of M1-type macrophage inflammatory cytokines. (A) The western blot analysis of t-P65, p-P65 and IL-1β proteins in M1-type macrophages after cultured with the CS ion solution for 24 hours. (B)–(D) Quantitative analysis of expression levels of t-P65, p-P65 and IL-1β according to the western blot analysis. (E) The qRT-PCR analysis of the expression of phenotypic M1-related cytokines (IL-1β, IL-6, TNF-α and iNOS) in macrophages treated with different concentrations of CS ion solution after induction with M1 macrophages by LPS. (F) and (G) Flow cytometry analysis of the expressions of macrophage surface markers CD68 and CD206 in macrophages treated with CS ion solution, the pancreatic stellate cell supernatant (PSC CM), and the PSC conditioned supernatant after 1/8CS treatment (PSC CM + CS) for 24 h. (H and I) Quantitative analysis of the expression levels of macrophage surface markers CD68 and CD206 (* p < 0.05 compared with the none group and the ctrl group; ** p < 0.01 compared with the none group and the ctrl group; ## p < 0.01 compared with the PCS CM group; n = 4). | |
3.8. CS ion solution down-regulated the activation of PSCs to reduce the ECM production by modulating intercellular communication
Injured PACs and M1 macrophages could activate PSCs through paracrine secretion, leading to pancreatic fibrosis.38,39 However, since CS ion solution have positive effects on PACs and macrophages, we speculated that CS ion solution may be able to inhibit pancreatic fibrosis by regulating the interaction between PACs/PSCs or M1 macrophages/PSCs. To test this hypothesis, we first used CS ion solution to treat injured PACs and co-cultured with PSCs. The results showed that when PSCs were co-cultured with injured PACs, the expression of α-SMA and Col1α1 was increased compared with the ctrl group, indicating that injured PACs could activate PSCs and promote collagen deposition. However, the 1/64 dilution of CS ion solution significantly reduced the secretion of α-SMA and Col1α1 in PSCs with or without co-culture with injured PACs (Fig. 6A). Similar results were further confirmed by western blot and quantitative analysis in the protein level (Fig. 6B–D). Furthermore, the M1 macrophage conditioned medium (Raw), 1/8 CS ion solution (CS) and conditioned medium of M1 macrophage cultured with 1/8 CS ion solution (Raw + CS) were used to culture PSCs for the evaluation of the effect of CS ion solution on cell interactions between M1 macrophages and PSCs. The results showed that, compared with PSCs without any treatment, after 1/8 CS ion solution intervention, the expressions of α-SMA and Col1α1 in PSCs were clearly decreased, while the M1 macrophage conditioned medium enhanced these expressions. However, the expressions of α-SMA and Col1α1 decreased significantly after PSCs were cultured with raw + CS (Fig. 6E). Western blot and quantitative analysis further confirmed that the M1 macrophage conditioned medium significantly increased the protein expression levels of α-SMA and Col1α1 compared with PSCs without any treatment, while the conditioned medium of M1 macrophage cultured in 1/8 CS ion solution reduced the expressions of α-SMA and Col1α1 in PSCs (Fig. 6F–H). The above results indicated that CS ion solution could directly inhibit the activation of PSCs to inhibit pancreatic fibrosis. More importantly, CS ion solution could also reduce the activation of PSCs through regulating the intercellular communication between injured PACs/PSCs and M1 macrophages/PSCs, thereby indirectly inhibiting pancreatic fibrosis.
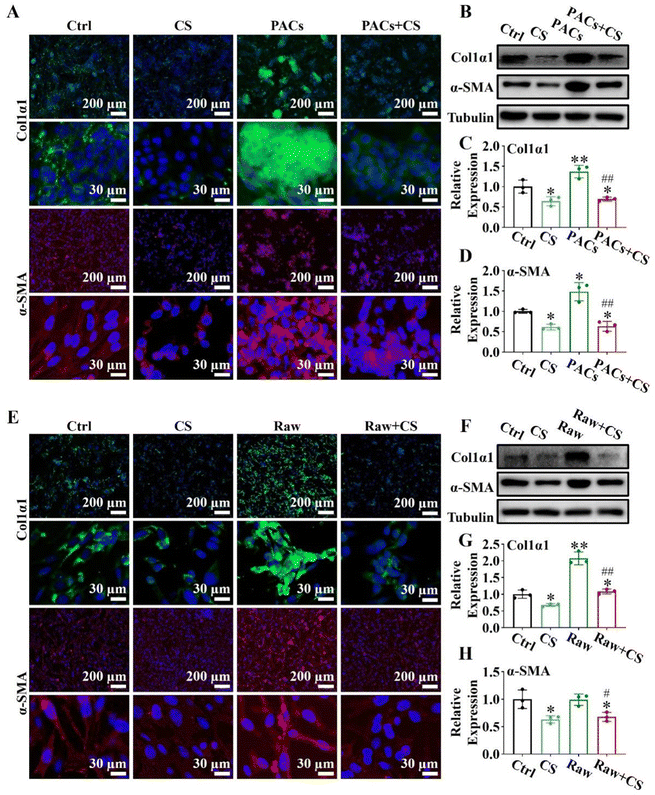 |
| Fig. 6 CS ion solution regulates intercellular communication and reduces pancreatic stellate cell (PSC) activation and extracellular matrix deposition. (A) The effect of 1/64CS ion solution on the expression of Col1α1 and α-SMA immunofluorescence staining after injured pancreatic acinar cells (PACs) co-cultured with PSCs: DAPI (blue), Col1α1 (green), and α-SMA (red). (B) Representative western blot bands of Col1α1 and α-SMA in PSCs co-cultured with injured PACs for 24 hours. (C) and (D) Quantitative analysis of protein levels quantified from protein bands of Col1α1 and α-SMA versus tublin in PSCs co-cultured with injured PACs for 24 hours. (ctrl group: PSCs cultured with the normal culture medium; CS group: PSCs cultured with 1/64CS ion solution; PAC group: PSCs co-cultured with injured PACs; PACs + CS group: PSCs co-cultured with injured PACs and cultured with 1/64CS ion solution). (E) The effect of 1/8CS ion solution on the expression of Col1α1 and α-SMA immunofluorescence staining after PSCs cultured with the macrophage conditioned medium: DAPI (blue), Col1α1 (green), and α-SMA (red). (F) Representative western blot bands of Col1α1 and α-SMA in PSCs co-cultured with the M1 macrophage conditioned medium for 24 hours. (G) and (H) Quantitative analysis of protein levels quantified from protein bands of Col1α1 and α-SMA versus tublin in PSCs co-cultured with the M1 macrophage conditioned medium for 24 hours. (ctrl group: PSCs cultured with the normal culture medium; CS group: PSCs cultured with 1/8CS ion solution; raw group: PSCs cultured with the M1 macrophage conditioned medium; raw + CS group: PSCs cultured with the M1 macrophage conditioned medium after 1/8CS treatment for 24 h) (* p < 0.05 compared with the ctrl group; ** p < 0.01 compared with the ctrl group; # p < 0.05 compared with the PAC group or raw group; ## p < 0.01 compared with the PAC group or raw group; n = 3). | |
3.9. Biosafety evaluation of CS ion solution
To evaluate the biosafety of CS ion solution, we collected important organs of mice such as heart, liver, spleen, lungs and kidneys for H&E staining and found that there were no histological differences in all organs after the treatment of CS ion solution through intraperitoneal injection compared with PBS (Fig. S5, ESI†). Furthermore, after treatment with CS ion solution for 1 month, there was no residual silicon found in the heart, liver, spleen, lungs and kidneys of mice (Fig. S6, ESI†). This result indicates that the CS ion solution has no acute toxicity and might be safe for treating CP.
4. Discussion
Chronic pancreatitis (CP) is a multifactorial fibroinflammatory syndrome. The repeated inflammatory reaction leads to pancreatic acinar atrophy and fibrosis, which seriously affects the quality of life of patients with a reduced life span.40 However, the current clinical treatment of CP is mainly to alleviate symptoms, which cannot fundamentally inhibit the progress of the disease.40 In this study, we applied a biomaterial-based approach by preparation of bioactive SiO32− ion solution from calcium silicate (CS) bioceramics for the treatment of CP, and for the first time demonstrated that CS ion solution has a unique therapeutic effect on CP in mice. It can reduce pancreatic acinar atrophy, regulate immune response, and inhibit inflammation and pancreatic fibrosis (Fig. 7). In this study, we specially selected Cre mice and LSL/Cre transgenic mice to construct two different animal models of CP. In particular, LSL/Cre transgenic mice were hybridized between Cre mice and Loxp-STOP-Loxp (LSL) mice with endogenous KrasG12D mutations. Since the specific K-ras point of PACs mutates in this kind of mouse, pancreas will start to suffer from persistent inflammation and gradually become severe pancreatitis after being injected with caerulein for a short time.28,41 In contrast, Cre mice need to be treated with caerulein repeatedly to induce acute inflammation of the pancreas to cause persistent acinar injury, and finally develop into CP.42 However, the degree of inflammatory reaction in the model of CP constructed in this way is relatively low. Interestingly, we found that CS ion solution has a unique therapeutic effect on both mild and severe CP, which indicates a stable and efficient therapeutic effect.
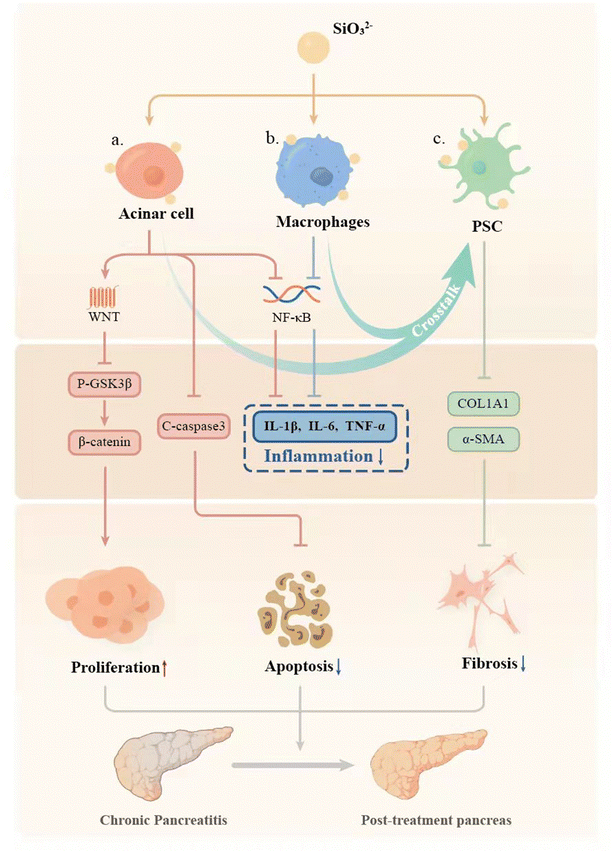 |
| Fig. 7 Schematic mechanism of CS ion solution in the treatment of CP. (a) SiO32− in CS ion solution could inhibit c-caspase3 related PACs apoptosis, regulate the WNT/β-catenin signaling pathway to promote pancreas acinar cell (PAC) proliferation. (b). SiO32− reduces macrophage infiltration and inhibits the NF-κb signaling pathway to reduce the secretion of inflammatory factors in M1 macrophages and PACs. (c) In addition, SiO32− effectively reduces the activation and ECM production of pancreatic stellate cells (PSCs) directly or by mediating the cell–cell crosstalk. | |
Pancreatic acinar cells (PACs) will gradually develop apoptosis and necrosis in CP patients, leading to pancreatic atrophy.43–45 Therefore, how to protect PACs effectively may be one of the key points for treating CP. Interestingly, in our study, we found that CS ion solution significantly reduced the number of TUNEL positive PACs, and decreased the expression of the apoptosis-related protein c-caspase3 in PACs. This result shows that CS ion solution can effectively inhibit the apoptosis of PACs in the progress of CP. Previous studies have shown that NF-κB is one of the main signaling pathways involved in apoptosis.33 The activated NF-κB signaling pathway can promote the production of TNF-α, thereby mediating cell apoptosis.46 Interestingly, in our study, we found that CS ion solution effectively inhibited the expression of phosphorylated NF-κB-P65. This result suggests that the inhibition of PACs apoptosis by CS ion solution may be associated with the inhibition of the NF-κB signaling pathway, thus avoiding the atrophy of pancreas. In addition to inhibiting apoptosis of PACs, promoting PAC proliferation may also be an important way to protect the pancreas from atrophy for CP.47 Although the regeneration promoting activity of CS bioceramics has been found in bone, skin, myocardium, lungs and other tissues,48–51 it is unclear whether CS bioceramics derived ionic solution has the proliferation activity on pancreas. Interestingly, we observed that CS ion solution indeed significantly increased the number of Ki67 positive acinar cells, indicating that it has activity to stimulate PAC proliferation. Since Wnt/β-catenin is one of the most critical signaling pathways in tissue regeneration,29,52 we speculated that CS ion solution may promote the proliferation of PACs mainly through this pathway. Interestingly, the results showed that CS ion solution could indeed inhibit the expression of phosphorylated GSK3 in PACs to increase the accumulation of β-catenin, thus upregulating the Wnt/β-catenin signaling pathway. Many studies have shown that most drugs only inhibit the apoptosis of PACs, but cannot promote cell proliferation.53,54 This might be the main reason why these drugs could only be used to relieve CP symptoms. Unlike most organic drugs, which only regulated a single cell pathway,55,56 CS ion solution systematically regulated the whole microenvironment of cells.57 Our results in this study demonstrated that CS ion solution significantly regulated both NF-κB and Wnt/β-catenin signaling pathways in PACs simultaneously, which not only inhibited apoptosis of PACs but also promoted cell proliferation, which may contribute to its therapeutic effects on CP.
The infiltration of macrophages determines the degree of inflammation in CP.58–60 It is known that more M1 macrophages infiltration will lead to more serious inflammatory reactions in the tissues, which will further aggravate the progress of CP.61 Therefore, reducing M1 macrophage infiltration is also one of the effective ways to improve the inflammatory response of CP.7,62 Since the NF-κB signaling pathway plays an important role in immune regulation and macrophage polarization35,36 and previous studies have shown that CS reduces the inflammatory response of macrophages and the immune response between bone tissue implants and hosts by inhibiting the NF-κB signaling pathway,16 we speculated that CS ion solution may also regulate the NF-κB signaling pathway to reduce M1 macrophages infiltration in CP. Interestingly, we found that CS ion solution indeed inhibited the activation of the NF-κB signaling pathway to down-regulate the expression of phenotype related cytokines of M1 macrophages, such as IL-1β, TNF-α, TGF-β and iNOS, which may be one of the most important reasons for CS ion solution to reduce the persistent damage of the inflammatory reaction to the pancreas. In addition, studies have shown that M1 and M2 macrophages can also promote PSCs to produce collagen, leading to pancreatic fibrosis.31,63 Michalski et al.38 induced M1 polarization of macrophages with LPS and found that the amount of collagen expressed was significantly increased after co-cultured with PSCs. Hou et al.64 found that M2 polarized macrophages can activate myofibroblast viability and accelerate the progress of tissue fibrosis. Although CS ion solution significantly inhibited M1 polarization of macrophages, our previous study found that the ions released by the CS bioceramic scaffold also significantly promoted the M2 polarization of macrophages during bone regeneration and repair.65 Therefore, whether CS ion solution can accelerate pancreatic fibrosis by inducing M2 polarization of macrophages is a matter of concern. However, interestingly, in this study, we found that CS ion solution did not increase the number of M2 macrophages in pancreatic tissues. Therefore, we used flow cytometry to analyze the expression of surface markers CD68 and CD206 of macrophages, which were treated with CS ion solution, the supernatant of PSCs cultured in the medium or the supernatant of PSCs cultured in CS ion solution. The results showed that CS ion solution alone could indeed inhibit M1 polarization and promote M2 polarization of macrophages. However, the supernatant of PSCs cultured in CS ion solution not only reduced M1 polarized, but also decreased M2 polarized macrophages. This indicates that CS ion solution may produce the paracrine effect through PSCs to make macrophages enter the undifferentiated state of M0 again. Since M0 macrophages do not participate in the process of pancreatic fibrosis,31,63 this may also be one of the reasons why CS ion solution has excellent anti-fibrosis effect in the treatment of CP.
Excessive fibrosis is the important problem of chronic pancreatitis. When a large number of pancreatic stellate cells (PACs) were activated, the secretion of an extracellular matrix could increase quickly, and the original function of the pancreas would be damaged.66 Therefore, inhibiting pancreatic fibrosis is also a key issue in the treatment of CP. Since the activation of pancreatic stellate cells (PSCs) plays a central role in the fibrosis of chronic pancreatitis,67 some small molecule drugs have been designed to reduce the viability of PSCs, thereby inhibiting the fibrosis of CP,68,69 which inevitably causes damage to other cells. In contrast, CS ion solution did not inhibit the cell viability of PSCs, but significantly inhibited CP fibrosis. Studies have shown that the α-SMA expression in activated PSCs is significantly increased, leading to a large number of ECM secretion and deposition, and ultimately leading to the formation of pancreatic fibrosis.70 Therefore, we speculated that CS ion solution may inhibit CP fibrosis by inhibiting the expression of fibrosis factors in PSCs. Interestingly, we did find that CS ion solution inhibited the expressions of α-SMA and col1α1 in PSCs, indicating that CS ion solution had a direct inhibitory effect on pancreatic fibrosis mediated by PSCs. Furthermore, some studies have shown that the activation of PSCs was also affected by the pancreatic microenvironment71 and was mainly regulated by injured PACs and M1 macrophages.72,73 Therefore, the interaction between PSCs and injured PACs or M1 macrophages is critical for CP treatment, and we indeed found that CS ion solution significantly inhibited the progress of fibrosis by regulating the interaction between PACs, macrophages and PSCs, so the CS ion solution not only has a certain directly anti-fibrosis effect, but also indirectly inhibit the fibrosis process mediated by injured PACs and M1 macrophages.
5. Conclusions
In this study, a biomaterial-based treatment scheme for chronic pancreatitis (CP) was proposed for the first time. CS bioceramics with unique biological activity were used to prepare a highly bioactive CS ion solution. This “ion therapy” effectively inhibited the inflammatory reaction in CP and protected the pancreas from atrophy. The unique therapeutic effect of the CS ion solution is attributed to the biological activity of SiO32− ions, which were effective at a concentration of 121.2 ± 0.69 μg mL−1, and systematically modulated the cell–cell interactions of different cell types in pancreatic tissues, including pancreatic acinar cells (PACs), macrophages, and pancreatic stellate cells (PSCs). Our research has proved that this new CP treatment method based on CS bioceramic biomaterials is effective and provides a theoretical basis and guiding significance for the development of new CP therapies.
Author contributions
H. Huang, J. Chang and Z. Li designed this study. H. Yin, Z. Zhang, C. Xia, L. Peng, D. Zhang, Xinyue Wang and X. Yang performed the search. H. Yin, Z. Zhang and D. Zhang collected and analyzed the data. H. Yin and Z. Zhang wrote the manuscript. All authors read and approved the final manuscript.
Conflicts of interest
The authors declare that the research was conducted in the absence of any commercial or financial relationships that could be construed as a potential conflict of interest.
Acknowledgements
This work was supported by the National Natural Science Foundation of China (Grant No. 82020108005), the National Outstanding Youth Science Fund Project of National Natural Science Foundation of China (Grant No. 82022008) and Natural Science Foundation of Ningxia Province (Grant No. 2023AAC03524).
References
- G. Beyer, A. Habtezion, J. Werner, M. M. Lerch and J. Mayerle, The Lancet, 2020, 396, 499–512 CrossRef PubMed
.
- S. S. Olesen, L. H. Mortensen, E. Zinck, U. Becker, A. M. Drewes, C. Nojgaard, S. Novovic, D. Yadav and J. S. Tolstrup, United Eur. Gastroenterol. J., 2021, 9, 82–90 CrossRef PubMed
.
- D. M. Spagnolo, P. J. Greer, C. S. Ohlsen, S. Mance, M. Ellison, C. Breze, B. Busby, D. C. Whitcomb and M. Haupt, Clin. Transl. Gastroenterol., 2022, 13, e00455 CrossRef PubMed
.
- V. K. Singh, D. Yadav and P. K. Garg, JAMA, 2019, 322, 2422–2434 CrossRef CAS PubMed
.
- S. Midha, V. Sreenivas, M. Kabra, T. K. Chattopadhyay, Y. K. Joshi and P. K. Garg, Pancreas, 2016, 45, 1478–1484 CrossRef PubMed
.
- Z. Sun, W. Gou, D. S. Kim, X. Dong, C. Strange, Y. Tan, D. B. Adams and H. Wang, Mol. Ther., 2017, 25, 2490–2501 CrossRef CAS PubMed
.
- X. P. Zeng, L. J. Wang, H. L. Guo, L. He, Y. W. Bi, Z. L. Xu, Z. S. Li and L. H. Hu, Pharmacol. Res., 2019, 147, 104357 CrossRef CAS PubMed
.
- Z. Ma, J. Zhou, T. Yang, W. Xie, G. Song, Z. Song and J. Chen, Med. Res. Rev., 2021, 41, 2474–2488 CrossRef PubMed
.
- H. Gu, J. Werner, F. Bergmann, D. C. Whitcomb, M. W. Büchler and F. Fortunato, Cell Death Dis., 2013, 4, e816 CrossRef CAS PubMed
.
- A. J. Hietaranta, A. K. Saluja, L. Bhagat, V. P. Singh, A. M. Song and M. L. Steer, Biochem. Biophys. Res. Commun., 2001, 280, 388–395 CrossRef CAS PubMed
.
- J. Lee, J. Seo, H. Kim, J. B. Chung and K. H. Kim, Ann. N. Y. Acad. Sci., 2003, 1010, 104–108 CrossRef CAS PubMed
.
- M. Apte, R. Pirola and J. Wilson, Antioxid. Redox Signaling, 2011, 15, 2711–2722 CrossRef CAS PubMed
.
- T. Watanabe, M. Kudo and W. Strober, Mucosal Immunol., 2017, 10, 283–298 CrossRef CAS PubMed
.
- C. Wu and J. Chang, Biomed. Mater., 2013, 8, 032001 CrossRef CAS PubMed
.
- Q. Yu, J. Chang and C. Wu, J. Mater. Chem. B, 2019, 7, 5449–5460 RSC
.
- Y. Huang, C. Wu, X. Zhang, J. Chang and K. Dai, Acta Biomater., 2018, 66, 81–92 CrossRef CAS PubMed
.
- H. Li, K. Xue, N. Kong, K. Liu and J. Chang, Biomaterials, 2014, 35, 3803–3818 CrossRef CAS PubMed
.
- T. Chen, Z. Zhang, D. Weng, L. Lu, X. Wang, M. Xing, H. Qiu, M. Zhao, L. Shen, Y. Zhou, J. Chang and H. P. Li, Bioact. Mater., 2021, 6, 3194–3206 CrossRef CAS PubMed
.
- X. Y. Wang, L. Gao, Y. Han, M. Xing, C. C. Zhao, J. L. Peng and J. Chang, Adv. Sci., 2018, 5, 1800776 CrossRef PubMed
.
- M. Yi, H. K. Li, X. Y. Wong, J. Y. Yan, L. Gao, Y. Y. He, X. L. Zhong, Y. B. Cai, W. J. Feng, Z. P. Wen, C. T. Wu, C. W. Ou, J. Chang and M. S. Chen, Adv. Sci., 2019, 6, 1801260 CrossRef PubMed
.
- H. Li and J. Chang, Acta Biomater., 2013, 9, 6981–6991 CrossRef CAS PubMed
.
- H. Li, J. He, H. Yu, C. R. Green and J. Chang, Biomaterials, 2016, 84, 64–75 CrossRef CAS PubMed
.
- M. Xing, X. Wang, E. Wang, L. Gao and J. Chang, Acta Biomater., 2018, 72, 381–395 CrossRef CAS PubMed
.
- G. X. Zhang, M. X. Wang, W. Nie, D. W. Liu, Y. Zhang and H. B. Liu, Pancreas, 2017, 46, 1327–1335 CrossRef CAS PubMed
.
- J. Gout, R. M. Pommier, D. F. Vincent, B. Kaniewski, S. Martel, U. Valcourt and L. Bartholin, J. Vis. Exp., 2013, 13, 50514 Search PubMed
.
- M. G. Bachem, E. Schneider, H. Gross, H. Weidenbach, R. M. Schmid, A. Menke, M. Siech, H. Beger, A. Grünert and G. Adler, Gastroenterology, 1998, 115, 421–432 CrossRef CAS PubMed
.
- H. Huang, J. Chen, L. Peng, Y. Yao, D. Deng, Y. Zhang, Y. Liu, H. Wang, Z. Li, Y. Bi, A. N. Haddock, X. Zhan, W. Lu, C. D. Logsdon and B. Ji, Am. J. Physiol.: Gastrointest. Liver Physiol., 2019, 316, G179–G186 CrossRef CAS PubMed
.
- J. Daniluk, Y. Liu, D. Deng, J. Chu, H. Huang, S. Gaiser, Z. Cruz-Monserrate, H. Wang, B. Ji and C. D. Logsdon, J. Clin. Invest., 2012, 122, 1519–1528 CrossRef CAS PubMed
.
- M. D. Keefe, H. Wang, O. J. De La, A. Khan, M. A. Firpo and L. C. Murtaugh, Dis. Models Mech., 2012, 5, 503–514 CAS
.
- H. Zhang, B. Liu, X. F. Xu, T. T. Jiang, X. Q. Zhang, Y. L. Shi, Y. Chen, F. Liu, J. Gu, L. J. Zhu and N. Wu, World J. Gastroenterol., 2016, 22, 2960–2970 CrossRef CAS PubMed
.
- J. Xue, V. Sharma, M. H. Hsieh, A. Chawla, R. Murali, S. J. Pandol and A. Habtezion, Nat. Commun., 2015, 6, 7158 CrossRef CAS PubMed
.
- M. Meenapriya, Biosci. Biotechnol. Res. Commun., 2020, 13, 286–290 CrossRef
.
- M. Bhatia, J. Cell. Mol. Med., 2004, 8, 402–409 CrossRef CAS PubMed
.
- C. Petrella, S. Agostini, G. S. Alema, P. Casolini, F. Carpino, C. Giuli, G. Improta, G. Linari, V. Petrozza and M. Broccardo, Neurogastroenterol. Motil., 2010, 22(1248–1256), e1323 Search PubMed
.
- C. Yunna, H. Mengru, W. Lei and C. Weidong, Eur. J. Pharmacol., 2020, 877, 173090 CrossRef PubMed
.
- U. Juhas, M. Ryba-Stanisławowska, P. Szargiej and J. Myśliwska, Postepy Hig. Med. Dosw., 2015, 69, 496–502 CrossRef PubMed
.
- H. Li, W. Wang and J. Chang, Regener. Biomater., 2021, 8, rbab056 CrossRef PubMed
.
- C. W. Michalski, A. Gorbachevski, M. Erkan, C. Reiser, S. Deucker, F. Bergmann, T. Giese, M. Weigand, N. A. Giese, H. Friess and J. Kleeff, J. Transl. Med., 2007, 5, 63 CrossRef PubMed
.
- H. Huang, Y. Liu, J. Daniluk, S. Gaiser, J. Chu, H. Wang, Z. S. Li, C. D. Logsdon and B. Ji, Gastroenterology, 2013, 144, 202–210 CrossRef CAS PubMed
.
- P. A. Hart and D. L. Conwell, Am. J. Gastroenterol., 2020, 115, 49–55 CrossRef PubMed
.
- B. Ji, L. Tsou, H. Wang, S. Gaiser, D. Z. Chang, J. Daniluk, Y. Bi, T. Grote, D. S. Longnecker and C. D. Logsdon, Gastroenterology, 2009, 137, 1072–1082 CrossRef CAS PubMed
, 1082 e1071-1076.
- M. M. Lerch and F. S. Gorelick, Gastroenterology, 2013, 144, 1180–1193 CrossRef PubMed
.
- N. I. Walker, C. M. Winterford, R. M. Williamson and J. F. Kerr, Pancreas, 1993, 8, 443–449 CrossRef CAS PubMed
.
- M. Kornmann, T. Ishiwata, H. Maruyama, H. G. Beger and M. Korc, Pancreas, 2000, 20, 123–128 CrossRef CAS PubMed
.
- P. H. Jiang, Y. Motoo, M. I. Vaccaro, J. L. Iovanna, G. Okada and N. Sawabu, Pancreas, 2004, 29, 225–230 CrossRef CAS PubMed
.
- I. Gukovsky, A. S. Gukovskaya, T. A. Blinman, V. Zaninovic and S. J. Pandol, Am. J. Physiol., 1998, 275, G1402–1414 CAS
.
- L. C. Murtaugh and M. D. Keefe, Annu. Rev. Physiol., 2015, 77, 229–249 CrossRef CAS PubMed
.
- S. Saravanan, S. Vimalraj, M. Vairamani and N. Selvamurugan, J. Biomed. Nanotechnol., 2015, 11, 1124–1138 CrossRef CAS PubMed
.
- T. Chen, Z. Zhang, D. Weng, L. Lu, X. Wang, M. Xing, H. Qiu, M. Zhao, L. Shen, Y. Zhou, J. Chang and H.-P. Li, Bioactive Materials, 2021, 6, 3194–3206 CrossRef CAS PubMed
.
- Z. Zhang, W. Li, Y. Liu, Z. Yang, L. Ma, H. Zhuang, E. Wang, C. Wu, Z. Huan, F. Guo and J. Chang, Bioact. Mater., 2021, 6, 1910–1920 CrossRef CAS PubMed
.
- Z. Zhang, Y. Zhang, W. Li, L. Ma, E. Wang, M. Xing, Y. Zhou, Z. Huan, F. Guo and J. Chang, Appl. Mater. Today, 2021, 23, 101065 CrossRef
.
- A. Strom, C. Bonal, R. Ashery-Padan, N. Hashimoto, M. L. Campos, A. Trumpp, T. Noda, Y. Kido, F. X. Real, F. Thorel and P. L. Herrera, Development, 2007, 134, 2719–2725 CrossRef CAS PubMed
.
- A. A. Mrazek, V. Bhatia, M. Falzon, H. Spratt, C. Chao and M. R. Hellmich, Pancreas, 2019, 48, 711–718 CrossRef CAS PubMed
.
- W. S. Park, K. Paik, K. J. Yang and J. O. Kim, Pancreas, 2020, 49, 281–289 CrossRef CAS PubMed
.
- S. B. Su, M. J. Xie, N. Sawabu and Y. Motoo, Pancreatology, 2007, 7, 28–36 CrossRef PubMed
.
- Y. M. Yang, M. Ramadani and Y. T. Huang, World J. Gastroenterol., 2003, 9, 2828–2831 CrossRef CAS PubMed
.
- Y. Que, Z. Zhang, Y. Zhang, X. Li, L. Chen, P. Chen, C. Ou, C. Yang and J. Chang, Bioact. Mater., 2022, 25, 716–731 CrossRef PubMed
.
- L. Sun, M. Xiu, S. Wang, D. R. Brigstock, H. Li, L. Qu and R. Gao, J. Cell. Mol. Med., 2018, 22, 2346–2356 CrossRef CAS PubMed
.
- A. S. Gukovskaya, I. Gukovsky, H. Algül and A. Habtezion, Gastroenterology, 2017, 153, 1212–1226 CrossRef CAS PubMed
.
- C. Shi, M. K. Washington, R. Chaturvedi, Y. Drosos, F. L. Revetta, C. J. Weaver, E. Buzhardt, F. E. Yull, T. S. Blackwell, B. Sosa-Pineda, R. H. Whitehead, R. D. Beauchamp, K. T. Wilson and A. L. Means, Lab. Invest., 2014, 94, 409–421 CrossRef CAS PubMed
.
- C. Peng, G. P. Tu, L. Yu, P. Wu, X. L. Zhang, Z. Li, Z. Q. Li and X. Yu, Front. Immunol., 2022, 13, 840887 CrossRef CAS PubMed
.
- N. Wang, T. T. Zhao, S. M. Li, Y. H. Li, Y. J. Wang, D. S. Li and W. F. Wang, Exp. Cell Res., 2019, 382, 111457 CrossRef CAS PubMed
.
- A. Hashimoto, M. R. Karim, M. Kuramochi, T. Izawa, M. Kuwamura and J. Yamate, Toxicol. Pathol., 2020, 48, 509–523 CrossRef CAS PubMed
.
- J. W. Hou, J. Y. Shi, L. Chen, Z. Y. Lv, X. Chen, H. H. Cao, Z. Xiang and X. D. Han, Cell Commun. Signaling, 2018, 16, 89 CrossRef CAS PubMed
.
- T. Li, M. Z. Peng, Z. Z. Yang, X. J. Zhou, Y. Deng, C. Jiang, M. Xiao and J. W. Wang, Acta Biomater., 2018, 71, 96–107 CrossRef CAS PubMed
.
- G. Kloppel, S. Detlefsen and B. Feyerabend, Virchows Arch., 2004, 445, 1–8 CrossRef PubMed
.
- R. R. Bynigeri, A. Jakkampudi, R. Jangala, C. Subramanyam, M. Sasikala, G. V. Rao, D. N. Reddy and R. Talukdar, World J. Gastroenterol., 2017, 23, 382–405 CrossRef CAS PubMed
.
- W. Q. Xiao, W. L. Jiang, J. Shen, G. J. Yin, Y. T. Fan, D. Q. Wu, L. Qiu, G. Yu, M. Xing, G. Y. Hu, X. P. Wang and R. Wan, PLoS One, 2015, 10, e0141462 CrossRef PubMed
.
- L. J. Wang, L. He, L. Hao, H. L. Guo, X. P. Zeng, Y. W. Bi, G. T. Lu, Z. S. Li and L. H. Hu, J. Cell.
Mol. Med., 2020, 24, 9667–9681 CrossRef CAS PubMed
.
- X. Zhang, T. Jin, X. Huang, X. Liu, Z. Liu, Y. Jia and J. Hao, Exp. Cell Res., 2018, 373, 132–144 CrossRef CAS PubMed
.
- L. Tian, Z. P. Lu, B. B. Cai, L. T. Zhao, D. Qian, Q. C. Xu, P. F. Wu, Y. Zhu, J. J. Zhang, Q. Du, Y. Miao and K. R. Jiang, Int. J. Oncol., 2016, 48, 783–792 CrossRef CAS PubMed
.
- X. P. Zeng, L. J. Wang, H. L. Guo, L. He, Y. W. Bi, Z. L. Xu, Z. S. Li and L. H. Hu, Pharmacol. Res., 2019, 147, 104357 CrossRef CAS PubMed
.
- Y. F. Miao, J. Li, Y. M. Zhang, L. Zhu, H. Chen, L. Yuan, J. Hu, X. L. Yi, Q. T. Wu, M. H. Wan and W. F. Tang, World J. Gastroenterol., 2018, 24, 4448–4461 CrossRef CAS PubMed
.
Footnotes |
† Electronic supplementary information (ESI) available. See DOI: https://doi.org/10.1039/d3tb01287e |
‡ Hua Yin, Zhaowenbin Zhang and Deyu Zhang contributed equally to this work. |
|
This journal is © The Royal Society of Chemistry 2023 |
Click here to see how this site uses Cookies. View our privacy policy here.