DOI:
10.1039/D3TC03134A
(Paper)
J. Mater. Chem. C, 2023,
11, 16982-16991
New RM734-like fluid ferroelectrics enabled through a simplified protecting group free synthesis†
Received
30th August 2023
, Accepted 22nd November 2023
First published on 22nd November 2023
Abstract
We report a novel and simplified synthetic procedure for making analogues of the widely studied ferroelectric nematogen RM734. Our new procedure focuses on building materials starting from the nitro-terminus and eschewing protecting groups, in contrast to previously reported syntheses. This new synthetic approach confers two principal advantages: firstly, the synthesis of the variants described herein is expedient, being a single step as opposed to three or more via the classical route. Secondly, by forgoing the use of benzyl groups as utilised in the original synthesis we can include functionality that is incompatible with hydrogenolysis conditions (e.g. olefins, late halogens, unsaturated heterocycles). Several of the RM734-like materials we report exhibit ferroelectric nematic phases, and we rationalise the behaviour of these materials with aid of electronic structure calculations, potential energy surface scans and atomistic molecular dynamics simulations.
Introduction
Nematic liquid crystals possess a degree of orientational order of their constituent molecules (or particles) along a direction termed the director (
) and are widely exploited in display technology. It had long been considered that nematic (N) phases, even when comprised of extremely polar molecules, do not show a preference for bulk polar order; the molecular electric dipole vectors have an equal probability of orientation parallel or antiparallel with the director (i.e.
= −
, Fig. 1a). A nematic phase with polar ordering would also be ferroelectric; having the vast majority of its constituent electric dipole moments oriented along a single direction (−
≠ −
, Fig. 1b). In 2017 Mandle et al.1 and Nishikawa et al.2 simultaneously and independently reported highly polar liquid crystalline materials that exhibited multiple nematic phases. The lower temperature nematic phase is now understood to be polar and is termed the ferroelectric nematic (NF) phase. The NF phase is of special scientific interest3–12 due to the presence of polar order in a fluid system coupled with a strong linear electrooptical response13,14 and large polar domains.15 The NF phase may also be aligned using existing alignment techniques originally developed for conventional nematics,16 and potential applications are beginning to emerge, including photo-variable capacitors17 and electrostatic actuators.18
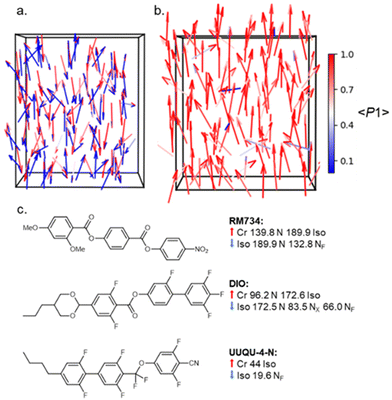 |
| Fig. 1 Molecular organization in the apolar nematic (a) and ferroelectric nematic (b) phases; arrows represent molecular electric dipole moments and are coloured according to the polar order parameter 〈P1〉 which takes a value of 0.06 in (a) and 0.89 in (b), while 〈P2〉 is ∼0.65 in both cases. (c) The molecular structures and transition temperatures (°C) of the three of the archetypal ferroelectric nematogens: RM734,1 DIO,2 and UUQU-4-N.19 | |
Despite a growing number of reported materials which exhibit the NF phase (for example20–29) studies into the phase are still in their infancy and little is known about how certain structural features drive NF phase formation. As a result, the majority of reported ferroelectric nematogens can be broadly classed as derivatives of one of the three archetypal structures (Fig. 1c): RM734,1 DIO2 and UUQU-4-N.19 For RM734, the synthesis is typically performed left-to-right, installing the 4-nitrophenyl unit last and employing a benzyl protecting strategy (Fig. 2(a)). The benzyl group enables high yields, but the hydrogenolysis deprotecting step brings issues of functional group compatibility. An alternative synthetic strategy has using a tetrahydropyranyl protecting group,21 suffers from low yields (Fig. 2(b), circa 40% over 3 steps). Herein we report a new synthetic pathway to ‘RM734-like’ compounds which installs the aromatic rings in the opposite order to the original synthesis of RM734 right-to-left (Fig. 3(d)). We eschew the need for any protecting groups entirely and allows for the highest possible functional group compatibility. We utilize this new methodology to report a series of novel RM734 derivatives, including those with halogens and alkenes at the terminus, some of which exhibit the NF phase.
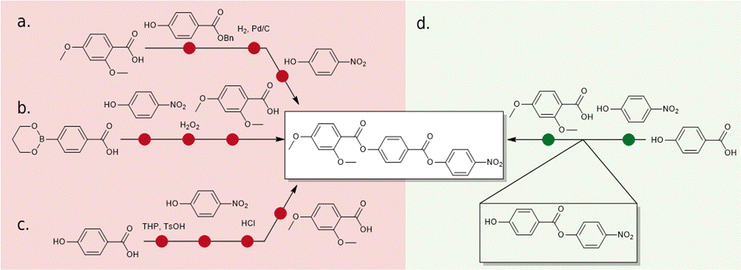 |
| Fig. 2 (a) Original synthesis of RM734 reported by Mandle et al.,1 (b) synthesis of RM734 and related materials reported Kumar et al.;30 (c) the synthetic route reported by Li et al.24 and Stepanafas et al.31 which employs the use of the THP protecting group; and (d) synthetic route developed and used in this work. | |
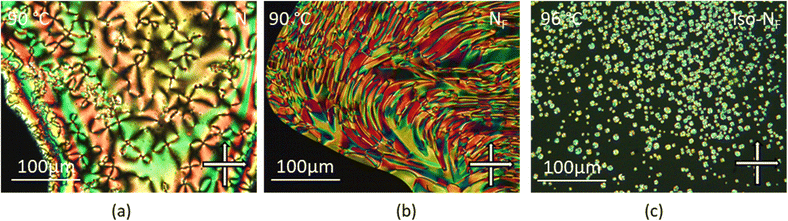 |
| Fig. 3 A nematic Schlieren texture containing both 2- and 4-point brush defects observed for compound 4 (a), an example of the banded texture of the NF phase observed for compound 3 (b), and the growth of point singularities at the I–NF phase transition observed on cooling for compound 2 (c). All textures were observed between untreated glass slides. | |
Experimental
Unless otherwise noted, all chemicals were purchased from commercial suppliers and used as received. Solvents were purchased from Merck. Reactions were monitored by TLC using UV light (254 nm). Chromatographic purification was performed using a Combiflash NextGen 300+ system (Teledyne Isco) using silica gel cartridges as the stationary phase and a hexane/DCM gradient as the mobile phase. The chromatographed materials were then recrystallized from the indicated solvent system, collected by filtration, and dried under reduced pressure. The materials were characterised by 1H, 13C{1H} and, where appropriate, 19F NMR. Electronic structure calculations were performed with the B3LYP hybrid functional and the LAN2DZ basis set32 in Gaussian G09 revision D01.33 Bimolecular PES scans employed additional GD3BJ dispersion34 and counterpoise correction terms. Following geometry optimisation we performed a frequency calculation, with the absence of imaginary frequencies taken to indicate the optimisation was at a minimum. We generated electrostatic potential (ESP) isosurfaces using the Gaussian formchk and cubegen utilities; the resulting cube files were then rendered using VMD. The interactions strength was quantified by performing a relaxed potential energy scan of the separation between two molecules arranged head-to-tail, from an initial separation of 2.5 Å to a maximum of 7 Å in 0.1 Å steps. Full experimental details, including synthetic procedures, structural characterisation and purity analysis are provided in the ESI.†
34
Results and discussion
The previously reported synthesis of RM734 employs a benzyl (OBn) protecting strategy, beginning from 2,4-dimethoxybenzoic acid and eventually affording the title compound in high yield over three linear steps (Fig. 2(a)). The nitrophenyl unit is installed last, owing to the incompatibility of this group with the hydrogenolysis conditions employed for debenzylation. Saha et al. have also reported a four-step synthetic pathway to the synthesis of RM734-like materials in which they forgo the use of the OBn protecting group, by instead obtaining the acid via aldehyde oxidation prior to the final esterification.35 Li et al.24 and Stepanafas et al.31 instal the ring units of RM734 in reverse order (Fig. 2(b)); utilising a tetrahydropyranyl (THP) protecting group to synthesise 4-nitrophenyl 4-hydroxybenzoate and similar derivatives in ∼ 40% yield, this then being subjected to terminal esterification to afford RM734. The modest yields afforded using this method are likely due to issues regarding chemical selectivity due to the presence of both the phenolic and acidic moieties as well as issues associated with cleavage of the group during purification by SiO2 gel chromatography as the group is easily cleaved under mildly acidic conditions.36 This makes the THP group non-ideal for in the case of the synthesise of RM734. Kumar et al. have also employed a synthetic strategy in which the rings are installed in the reverse order.30 The method presented by Kumar et al. contains only three synthetic steps using a boronic ester as the protecting group (Fig. 2(c)). Whist containing only three-synthetic steps from the boronic ester, the group is hydrolytically labile which may lead to unwanted side reactions occurring.
Our initial synthetic effort was to focus on improving this yield by utilizing a tert-butyldimethylsilyl (TBDMS) protecting group. Regrettably, the TBAF mediated cleavage invariably led to significant ester hydrolysis and this method was promptly abandoned as unworkable due to the lability of the 4-nitrophenyl group. We found success by avoiding protecting groups entirely (Fig. 2(d)). Our initial hint was that esterification of 4-hydroxybenzoic acid and 4-nitrophenol in a 1
:
1 ratio on a mmol scale gave good conversion to the desired product. As had been previously reported however, separation by standard re-crystallisation techniques from residual 4-nitrophenol was challenging.37–39 Gratifyingly, large scale attempts using a 1
:
2 mixture of 4-hydroxybenzoic acid and 4-nitrophenol reacted equally smoothly under Steglich conditions with EDC/DMAP which was successfully purified by flash chromatography using a gradient elution of Hexane/DCM to afford the title compound in 55% yield in a single step. After chromatography, we note that the material is somewhat unstable on silica which explains the modest yield achieved however, this instability is relieved following subsequent esterification. Subsequent Steglich esterification of 4-nitrophenyl 4-hydroxybenzoate with a library of substituted benzoic acids afforded compounds 1–11 in moderate to good yield (67–88%) in a method ultimately requiring only two synthetic steps.
We first synthesised five RM734-analogues which have various modifications made to the 2,4-dimethoxybenzoate unit. Transition temperatures and phase assignments (made via polarized optical microscopy (POM)) for compounds 1–5 are given in Table 1. The conventional N phases are assigned based upon the observation of characteristic Schlieren textures containing 2- and 4-point brush singularities (Fig. 3(a)). The NF phases have been assigned based on the observation of a banded textures (Fig. 3(b)), now thought to be characteristic of the phase.24,28,40 In cases where a direct NF–isotropic(I) phase transition is observed, the NF phase is seen to grow from point singularity defects (Fig. 3(c)) which coalesce to form the characteristic bulk texture. This observation has been reported previously for the NF–I transition.19,41 To further affirm the assignments of the NF phase, binary mixtures of the ferroelectric nematogens and RM734 have been prepared showing good miscibility across all concentrations. The NF phase is observed for all concentrations studied with an approximately linear dependence of the transition temperatures. The phase diagram for 2/RM734 is presented in Fig. 4 (top) whilst the data for other materials is given in the ESI† to this article. Calculated phase diagram data (melting points, transition temperatures) were obtained using data from DSC experiments on pure materials using the Eutectic software tool.42 The transition temperatures and associated enthalpy changes are extracted from their respective DSC thermograms (for example Fig. 4 (bottom)), measured at a heat/cool rate of 20 °C min−1 under a nitrogen atmosphere.
Table 1 Transition temperatures (T, °C) and associated enthalpies (ΔH, kJ mol−1) for compounds 1–5?
No. |
R = |
|
Melt |
NF–N/Iso |
N–Iso |
RM734
|
|
T
|
139.8 |
132.7 |
187.9 |
ΔH |
34.8 |
0.20 |
0.60 |
1
|
|
T
|
181.9 |
— |
— |
ΔH |
43.0 |
— |
— |
2
|
|
T
|
136.4 |
96.3 |
— |
ΔH |
32.9 |
1.7 |
— |
3
|
|
T
|
157.3 |
125.0 |
151.6 |
ΔH |
32.2 |
1.0 |
0.5 |
4
|
|
T |
151.1 |
— |
182.0 |
ΔH |
36.8 |
— |
0.5 |
5
|
|
T |
137.8 |
— |
119.8 |
ΔH |
38.7 |
— |
0.3 |
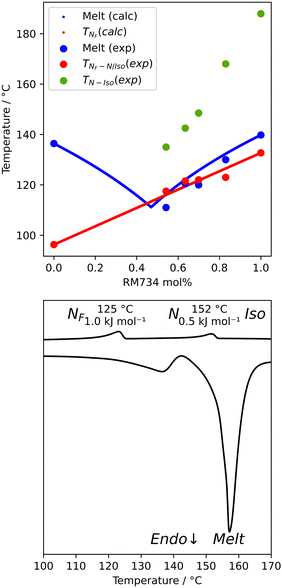 |
| Fig. 4 Phase diagram constructed by mixing 2 and RM734 (top), and DSC thermogram for compound 3 showing the N–I and NF–N phase transitions (bottom). | |
Compared to RM734, compound 1 has an additional OMe group in the 6-position and this sees a significant increase in the melting point and the total loss of mesogenic behaviour. Contrast this with the removal of the terminal methoxy group (4-position) of RM734; this gives compound 2 material, which exhibits a direct NF–Iso transition (albeit with an onset below that of the parent material) as well as a small reduction in melting point. This suggests that, whilst a terminal chain is beneficial for ‘classical’ non-polar nematics, it is not a requirement for the polar NF phase; and indeed the deleterious effect of long terminal chains on the stability of the NF phase is well known.25,27,28,40,43,44 Crudely put, this reflects the origins of the NF phase being in electrostatic (dipole–dipole) forces, whereas the conventional nematics result from shape anisotropy.
We next study the loss of oxygen atoms from the terminal/lateral methoxy group(s) of RM734 and compound 1. Compound 3, in which the terminal (4-) methoxy group is replaced with a methyl group has only a modest reduction in TNF–N, a sharp reduction in TN–Iso and a modest increase in melting point. Compound 4 is closely related to 3, having both the terminal and lateral methoxy groups replaced with methyl; however, the mesomorphic behaviour of 4 is rather different, with this material only exhibiting a conventional nematic phase. To a first approximation, this can be understood to be a consequence of the reduced dipole moment that results from the loss of the lateral methoxy group, and we will demonstrate this shortly (inter alia). In 5 we add an additional methyl group to 4 in the 2-position (or alternatively, we replace the methoxy units of 1 with methyl); with the resulting material exhibiting a conventional nematic phase.
We optimised the geometries of RM734 and compounds 1–5 at the B3LYP/LAN2LDZ level of DFT in order to rationalise the trends in observed mesophase behaviour (Table 2). Compound 1 is non mesogenic, despite being of comparable electrical polarity to RM734. In 1 the additional breadth that results from the two lateral methoxy units is reflected in a low value of the shape anisotropy parameter (ShAP). Compounds 2 and 3 both exhibit the NF phase, albeit at reduced temperature to the parent; they have comparable electric dipole moments to RM734, as well as being sufficiently anisotropic in shape to permit the formation of mesophases. Compounds 4 and 5 lack the lateral methoxy unit, and as such exhibit notably reduced electric dipole moments which are perhaps below the threshold required to exhibit the NF phase in this system. This result demonstrates the apparent necessity of the lateral methoxy group in promoting NF behaviour in RM734-like materials.
Table 2 Properties from DFT calculations on RM734 and compounds 1–5: molecular electric dipole moment (μ, Debye); anisotropy of polarizability (Δα/Å3); Shape Anisotropy Parameter (ShAP), defined here as
, where l/w/b are the molecular length, width, and breadth, respectively; Rotational Anisotropy Parameter (RotAP) defined here as
, where R1/2/3 are the rotational constants
No. |
R = |
μ/D |
Δα/Å3 |
ShAP |
RotAP |
RM734
|
|
12.78 |
51.1 |
4.8 |
21.1 |
1
|
|
12.23 |
46.2 |
3.5 |
21.0 |
2
|
|
11.55 |
43.8 |
4.6 |
18.9 |
3
|
|
12.46 |
47.6 |
4.6 |
19.8 |
4
|
|
10.65 |
47.9 |
5.7 |
19.9 |
5
|
|
9.88 |
46.6 |
3.9 |
19.8 |
The inclusion of late-halogen atoms in RM734 is complicated by the prior use of hydrogenolysis, with these often being labile under the H2/Pd conditions employed. Our new synthetic approach makes the inclusion of halogen atoms on the -left- and central-rings synthetically straightforward. To demonstrate this, we elected to synthesis compounds 6–9 which contain I, Br, Cl and, F, respectively, in the 4-position on the left-hand ring. The transitional temperatures and phase assignments of these compounds are shown in Table 3. Halogen atom are known for their ability to exhibit Sigma-holes,45,46 as well as being able to partake in a wide range of synthetic transformations.
Table 3 Transition temperatures (T, °C) and associated enthalpies (ΔH, kJ mol−1) for compounds 6–9
All the halogen terminated homologues exhibit monotropic nematic phases with only the iodine terminated derivative exhibiting the NF phase (Fig. 5). The values of TN–I remain almost constant for the three largest halogens, with a significant decrease observed for the fluorine terminated derivative. We optimised the geometries of RM734 and compounds 6–9 at the B3LYP/LAN2LDZ level of DFT in order to rationalise the trends in observed mesophase behaviour (Table 4). Immediately it is clear that a halogen atom at the 4-position leads to the expected reduction in molecular electric dipole moment relative to the parent RM734. The shape anisotropy parameter is almost constant across this series, while the rotational anisotropy parameter follows the expected trend as a consequence of the large mass of the halogen atom.
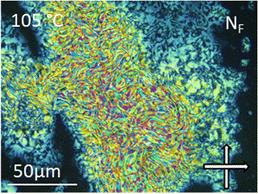 |
| Fig. 5 The banded texture observed by POM between untreated glass slides for the NF phase of compound 6 at 105 °C. | |
Table 4 Properties from DFT calculations on RM734 and compounds 6–9 molecular electric dipole moment (μ, Debye); anisotropy of polarizability (Δα/Å3); Shape Anisotropy Parameter (ShAP); Rotational Anisotropy Parameter (RotAP)
No. |
R = |
μ/D |
Δα/Å3 |
ShAP |
RotAP |
RM734
|
|
12.78 |
51.1 |
4.8 |
21.1 |
6
|
|
9.83 |
55.9 |
4.8 |
19.9 |
7
|
|
9.40 |
52.2 |
4.8 |
19.7 |
8
|
|
9.04 |
49.2 |
4.8 |
19.6 |
9
|
|
9.01 |
44.4 |
4.7 |
19.1 |
It is somewhat surprising that the iodo-terminated 6 exhibits the NF phase, while 7–9 do not; we therefore computed the molecular electrostatic potential on the 0.001 au electron density for each of these compounds (and also RM734). The ESP isosurface of RM734 is as expected, with the nitro- and carboxylate-groups giving rise to regions of enhanced electron density. Materials incorporating a terminal iodo-group feature a large Sigma hole – a region of positive charge which can partake in non-covalent interactions – with a smaller feature being seen for the bromo- terminated material (shown in Fig. 6a and c). For the fluoro- and chloro- terminated materials we do not find such a feature. Experimentally, the observation of an NF phase for the iodo-terminated materials suggests a propensity for head-to-tail ordering which implies the existence of electrostatic interactions between the terminal group and the nitro group at the other terminus.
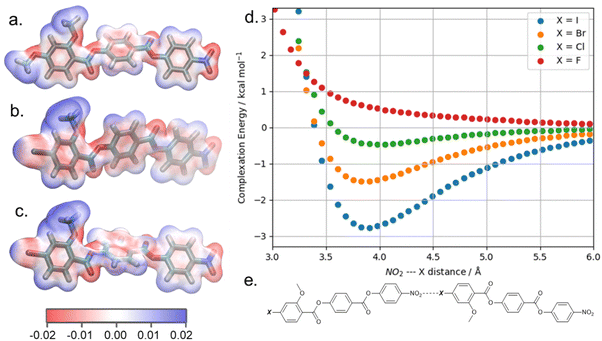 |
| Fig. 6 Optimized geometries of RM734 ((a) –OMe terminus), 6 ((b) –I terminus), 7 ((c) –Br terminus), at the B3LYP-GD3BJ/LAN2DZ level of DFT, with the electrostatic potential (ESP, isovalue = 0.04 a.u.) on the 0.001 au electron density isosurface. (d) Complexation energy (kcal mol−1) as a function of nitro-‘X’ separation (Å) for homodimers of compounds 6–9 (X = I, Br, Cl, F) as computed at the B3LYP-GD3BJ/LAN2DZ level of DFT; (e) diagram illustrating the distance referenced in (d) for a homodimer. | |
To quantify the potential for interactions between these groups we performed potential energy scans for head-to-tail homodimers of RM734, and compounds 6–9 Our calculations employ the B3LYP hybrid functional, GD3BJ dispersion correction, the LAN2DZ basis set, and additional counterpoise correction. We start from a homodimer of each compound, with the Ph-NO2 and Ph-X bonds – where X is OMe, F, Cl, Br, or I – being oriented on the same vector and with a separation of 2.5 Å between the nitro and X groups, and increase the separation in 0.1 Å steps.
We compute the complexation energy as function of the distance between the nitro group and the terminal group in question by performing relaxed scans about the potential energy surface (Fig. 6d). The iodo terminated material 6 has the largest Sigma hole and this displays a large complexation energy (∼2.7 kcal mol−1) at a separation of 3.7 Å. In contrast, the bromo-terminated material has a much smaller complexation energy (∼1.3 kcal mol−1) and the chloro- is smaller still (∼0.4 kcal mol−1). Owing to the electronegativity of its terminal fluorine group, 9 experiences electrostatic repulsion (i.e. positive complexation energy).
The final compounds we elected to synthesise using our new synthetic approach are compounds 10 and 11. Like the halogen containing compounds discussed previously, both compounds are reactive under the hydrogenolysis conditions previously employed in the synthesis of RM734 making them difficult if not impossible to obtain using the previously reported methodologies. The transitional temperatures of compounds 10 and 11 are shown in Table 5. Compound 10 may be compared to the parent RM734 molecule with an additional olefin inserted between the ester-link and left-hand ring. Inclusion of the unsaturated moiety significantly increases the melting point and N–I transition temperature and sees the extinction of the NF phase. The increase in melting point and isotropisation temperature probably results from both the increased molecular length and the enhanced intermolecular interactions (relative to RM734) that result from incorporation of the cinnamate unit. The short range of supercooling precludes the observation of a monotropic NF phase. Compound 11 shows an enantiotropic NF phase which transitions directly into the isotropic phase, as determined by POM (Fig. 7). As will be shown shortly, the later is probably a consequence of increased electric dipole moment.
Table 5 Transition temperatures (T, °C) and associated enthalpies (ΔH, kJ mol−1) for compounds 10 and 11
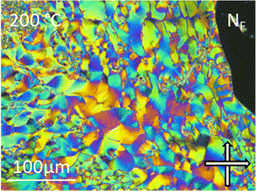 |
| Fig. 7 The banded texture observed by POM between untreated glass slides for the NF phase of compound 11 at 200 °C. | |
We optimised the geometries of RM734 and compounds 10 and 11 at the B3LYP/LAN2LDZ level of DFT (Table 6). Utilisation of the cinnamate ester in 10 leads to an increase in molecular length; in turn, this gives a larger electric dipole moment (as a consequence of the larger separation of charges) and larger values of ShAP/RotAP. Under experimental conditions 10 does not exhibit the NF phase, rather it crystallises on cooling prior to reaching this point. In compound 11 the presence of the 2-ethoxyfuran unit leads to a slightly larger electric dipole moment than the parent material which, inter alia, leads to a direct NF–Iso transition at high temperature, beyond even the isotropisation temperature of the parent material. The high melting point of 11 defied our expectations that the bent (∼120°) geometry of this unit would lead to a ‘low’ melting point, nevertheless the high TNF–N is a welcome observation.
Table 6 Properties from DFT calculations on RM734 and compounds 10–11 molecular electric dipole moment (μ, Debye); anisotropy of polarizability (Δα/Å3); Shape Anisotropy Parameter (ShAP); Rotational Anisotropy Parameter (RotAP)
No. |
R = |
μ/D |
Δα/Å3 |
ShAP |
RotAP |
RM734
|
|
12.78 |
51.1 |
4.8 |
21.1 |
10
|
|
14.74 |
68.9 |
6.8 |
23.5 |
11
|
|
13.22 |
46.0 |
5.1 |
18.8 |
Experimentally, compound 11 shows low solubility in with other NF materials and so we are unable to confirm the phase identification through binary mixtures or contact preparations. Compound 11 also begins to degrade above its melting point, making detailed physical study complicated, and so we consider the phase assignment as NF to be tentative. We therefore opted to explore the unusually high TNF–Iso in this material computationally, through the use of fully atomistic molecular dynamics simulations. Full details on simulation setup and parametrisation are given in the ESI† to this article; briefly, simulations comprised 600 molecules of 11, and commenced from a polar nematic starting configuration and at various temperatures with a production MD run of 250 ns. Our MD simulations appear to underestimate the experimental clearing point by 10–20 °C; at and below temperatures of 463 K we find polar nematic order to be stable, although the values of 〈P1〉 we obtain are persistently lower than those obtained under the same methodology for RM734, DIO, and UUQU-4-N (Fig. 8(a)).4,43
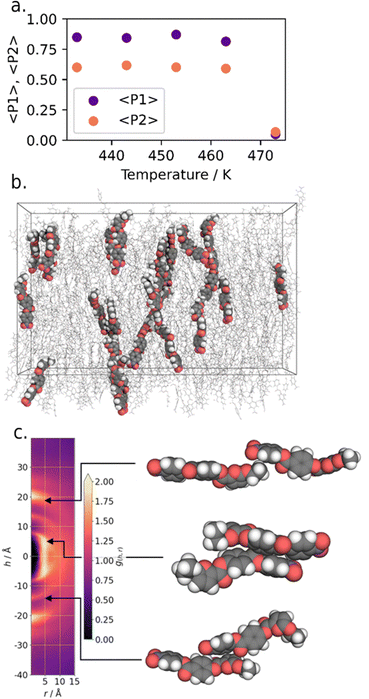 |
| Fig. 8 (a) plot of the polar (〈P1〉) and orientational (〈P2〉) order parameters as a function of simulation temperature for fully atomistic MD simulations of 11. (b) Instantaneous configuration of the polar (NF) nematic configuration of 11 at a temperature of 443 K (170 °C) at a simulation time of 231 ns. Molecules are shown as wireframe with 25 randomly selected and shown as space filling. (c) Cylindrical distribution function (CDF) of the polar (NF) nematic configuration of 11 at a temperature of 413 K (140 °C). | |
We gained additional insight by examination of the cylindrical distribution function of the MD trajectory (Fig. 8(c)). On-axis arcs centred at h = ±20 Å result from head-to-tail pairing, whereas additional arcs at h ∼ ±14 Å and ±9 Å result from close contacts between the furan ring and carboxylate esters on adjacent molecules. Our interpretation of this data is that the localised electron density that results from the furan-ring enables and favours stronger electrostatic interactions between adjacent molecules, and experimentally this manifest as a large increase in TNF-I. We consider that other related heterocyclic systems (e.g. oxazoles, thiazoles) may allow us to take advantage of these favourable interactions without incurring such a heavy penalty in terms of melting point. This now requires further study.
Conclusions
We report a new route to synthesise RM734 and derivatives which forgoes protecting groups entirely and offers expedient access to this important class of materials. As a demonstration of this, we synthesise new RM734-like materials in which we vary the number and chemical identity of the lateral and terminal methoxy groups. The methyl-terminated material and, surprisingly, the variant with no terminal chain at all are both competent NF materials. We report a family of materials with terminal halogen atoms; the iodo-terminated member exhibits the NF phase whereas others exhibit solely conventional nematic phases. DFT calculations suggest the ability of the iodo-terminated material to form the NF phase results from the presence of a large Sigma hole on this material enabling a weak electrostatic interaction with the highly electronegative nitro group on an adjacent molecule; effectively making head-to-tail pairing more energetically favourable than with other halogens. The lack of hydrogenolysis conditions in our synthesis permits us to synthesise RM734-like materials bearing olefins and unsaturated heterocycles, these would be problematic if not impossible to synthesise with the original synthesis.
Data availability
The data associated with this paper are openly available from the University of Leeds Data Repository at https://doi.org/10.5518/1411.
Conflicts of interest
There are no conflicts to declare.
Acknowledgements
R. J. M. acknowledges funding from UKRI via a Future Leaders Fellowship, grant no. MR/W006391/1, and funding from the University of Leeds via a University Academic Fellowship.
References
- R. J. Mandle, S. J. Cowling and J. W. Goodby, A Nematic to Nematic Transformation Exhibited by a Rod-like Liquid Crystal, Phys. Chem. Chem. Phys., 2017, 19, 11429 RSC
.
- H. Nishikawa, K. Shiroshita, H. Higuchi, Y. Okumura, Y. Haseba, S. I. Yamamoto, K. Sago and H. Kikuchi, A Fluid Liquid-Crystal Material with Highly Polar Order, Adv. Mater., 2017, 29, 1702354 CrossRef PubMed
.
- X. Chen,
et al., First-Principles Experimental Demonstration of Ferroelectricity in a Thermotropic Nematic Liquid Crystal: Polar Domains and Striking Electro-Optics Contributed New Reagents/Analytic Tools; X, Proc. Natl. Acad. Sci. U. S. A., 2002, 117, 14021 CrossRef PubMed
.
- R. J. Mandle, N. Sebastián, J. Martinez-Perdiguero and A. Mertelj, On the Molecular Origins of the Ferroelectric Splay Nematic Phase, Nat. Commun., 2021, 12, 4962 CrossRef CAS PubMed
.
- B. Basnet, M. Rajabi, H. Wang, P. Kumari, K. Thapa, S. Paul, M. O. Lavrentovich and O. D. Lavrentovich, Soliton Walls Paired by Polar Surface Interactions in a Ferroelectric Nematic Liquid Crystal, Nat. Commun., 2022, 13, 3932 CrossRef CAS PubMed
.
- P. Kumari, B. Basnet, H. Wang and O. D. Lavrentovich, Ferroelectric Nematic Liquids with Conics, Nat. Commun., 2023, 14, 748 CrossRef CAS PubMed
.
- O. D. Lavrentovich, Ferroelectric Nematic Liquid Crystal, a Century in Waiting, Proc. Natl. Acad. Sci. U. S. A., 2020, 117, 14629 CrossRef CAS PubMed
.
- X. Chen, E. Korblova, M. A. Glaser, J. E. Maclennan, D. M. Walba and N. A. Clark, Polar In-Plane Surface Orientation of a Ferroelectric Nematic Liquid Crystal: Polar Monodomains and Twisted State Electro-Optics, Proc. Natl. Acad. Sci. U. S. A., 2021, 118, e2104092118 CrossRef CAS
.
- N. Sebastián,
et al., Polarization Patterning in Ferroelectric Nematic Liquids via Flexoelectric Coupling, Nat. Commun., 2023, 14, 3029 CrossRef
.
- H. Nishikawa, K. Sano, S. Kurihara, G. Watanabe, A. Nihonyanagi, B. Dhara and F. Araoka, Nano-Clustering Mediates Phase Transitions in a Diastereomerically-Stabilized Ferroelectric Nematic System, Commun. Mater., 2022, 3, 89 CrossRef CAS
.
- J. Li, Z. Wang, M. Deng, Y. Zhu, X. Zhang, R. Xia, Y. Song, Y. Hisai, S. Aya and M. Huang, General Phase-Structure Relationship in Polar Rod-Shaped Liquid Crystals: Importance of Shape Anisotropy and Dipolar Strength, Giant, 2022, 11, 100109 CrossRef CAS
.
- J. Zhou, R. Xia, M. Huang and S. Aya, Stereoisomer Effect on Ferroelectric Nematics: Stabilization and Phase Behavior Diversification, J. Mater. Chem. C, 2022, 10, 8762 RSC
.
- C. L. Folcia, J. Ortega, R. Vidal, T. Sierra and J. Etxebarria, The Ferroelectric Nematic Phase: An Optimum Liquid Crystal Candidate for Nonlinear Optics, Liq. Cryst., 2022, 49, 899 CrossRef CAS
.
- N. Sebastián, R. J. Mandle, A. Petelin, A. Eremin and A. Mertelj, Electrooptics of Mm-Scale Polar Domains in the Ferroelectric Nematic Phase, Liq. Cryst., 2021, 48, 2055 CrossRef
.
- N. Sebastián, L. Cmok, R. J. Mandle, M. R. De La Fuente, I. Drevenšek Olenik, M. Čopič and A. Mertelj, Ferroelectric-Ferroelastic Phase Transition in a Nematic Liquid Crystal, Phys. Rev. Lett., 2020, 124, 037801 CrossRef PubMed
.
- F. Caimi, G. Nava, R. Barboza, N. A. Clark, E. Korblova, D. M. Walba, T. Bellini and L. Lucchetti, Surface Alignment of Ferroelectric Nematic Liquid Crystals, Soft Matter, 2021, 17, 8130 RSC
.
- H. Nishikawa, K. Sano and F. Araoka, Anisotropic Fluid with Phototunable Dielectric Permittivity, Nat. Commun., 2022, 13, 1142 CrossRef CAS PubMed
.
- S. Nishimura, S. Masuyama, G. Shimizu, C. Chen, T. Ichibayashi and J. Watanabe, Lowering of Electrostatic Actuator Driving Voltage and Increasing Generated Force Using Spontaneous Polarization of Ferroelectric Nematic Liquid Crystals, Adv. Phys. Res., 2022, 1, 2200017 CrossRef
.
- A. Manabe, M. Bremer and M. Kraska, Ferroelectric Nematic Phase at and below Room Temperature, Liq. Cryst., 2021, 48, 1079 CrossRef CAS
.
- S. Brown, E. Cruickshank, J. M. D. Storey, C. T. Imrie, D. Pociecha, M. Majewska, A. Makal and E. Gorecka, Multiple Polar and Non-Polar Nematic Phases, Chem. Phys. Chem., 2021, 22, 2506 CrossRef CAS PubMed
.
- R. J. Mandle, Supramolecular Ferroelectric Nematic Materials, Liq. Cryst., 2022, 49, 2019 CrossRef CAS
.
- R. J. Mandle, A New Order of Liquids: Polar Order in Nematic Liquid Crystals, Soft Matter, 2022, 18, 5014 RSC
.
- R. J. Mandle, S. J. Cowling and J. W. Goodby, Structural Variants of RM734 in the Design of Splay Nematic Materials, Liq. Cryst., 2021, 48, 1780 CrossRef CAS
.
- J. Li, H. Nishikawa, J. Kougo, J. Zhou, S. Dai, W. Tang, X. Zhao, Y. Hisai, M. Huang and S. Aya, Development of Ferroelectric Nematic Fluids with Giant-ε Dielectricity and Nonlinear Optical Properties, Sci. Adv., 2021, 7, 5047 CrossRef
.
- R. J. Mandle, S. J. Cowling and J. W. Goodby, Rational Design of Rod-Like Liquid Crystals Exhibiting Two Nematic Phases, Chem. – Eur. J., 2017, 23, 14554 CrossRef CAS
.
- X. Zhao, J. Zhou, J. Li, J. Kougo, Z. Wan, M. Huang and S. Aya, Spontaneous Helielectric Nematic Liquid Crystals: Electric Analog to Helimagnets, Proc. Natl. Acad. Sci. U. S. A., 2021, 118, e2111101118 CrossRef CAS
.
- N. Tufaha, E. Cruickshank, D. Pociecha, E. Gorecka, J. M. D. Storey and C. T. Imrie, Molecular Shape, Electronic Factors and the Ferroelectric Nematic Phase, Chem. – Eur. J., 2023, 29, e202300073 CrossRef CAS PubMed
.
- E. Cruickshank, R. Walker, J. M. D. Storey and C. T. Imrie, The Effect of a Lateral Alkyloxy Chain on the Ferroelectric Nematic Phase, RSC Adv., 2022, 12, 29482 RSC
.
- D. Pociecha,
et al., Intrinsically Chiral Ferronematic Liquid Crystals: An Inversion of the Helical Twist Sense at the Chiral Nematic – Chiral Ferronematic Phase Transition, J. Mol. Liq., 2022, 361, 119532 CrossRef CAS
.
- M. P. Kumar, J. Karcz, P. Kula, S. Karmakar and S. Dhara, Giant Electroviscous Effects in a Ferroelectric Nematic Liquid Crystal, Phys. Rev. Appl., 2023, 19, 04408 Search PubMed
.
- G. Stepanafas, E. Cruickshank, S. Brown, M. M. Majewska, D. Pociecha, E. Gorecka, J. M. D. Storey and C. T. Imrie, Ferroelectric Nematogens Containing a Methylthio Group, Mater. Adv., 2023 10.1039/D3MA00446E
.
- A. D. Becke, Density-Functional Thermochemistry. III. The Role of Exact Exchange, J. Chem. Phys., 1993, 98, 5648 CrossRef CAS
.
-
M. J. Frisch, G. W. Trucks, H. B. Schlegel, G. E. Scuseria, M. A. Robb, J. R. Cheeseman, G. Scalmani, V. Barone, B. Mennucci, G. A. Petersson, H. Nakatsuji, M. Caricato, X. Li, H. P. Hratchian, A. F. Izmaylov, J. Bloino, G. Zheng, J. L. Sonnenberg, M. Hada, M. Ehara, K. Toyota, R. Fukuda, J. Hasegawa, M. Ishida, T. Nakajima, Y. Honda,O. Kitao, H. Nakai, T. Vreven, J. A. Montgomery Jr., J. E. Peralta, F. Ogliaro, M. J. Bearpark, J. Heyd, E. N. Brothers, K. N. Kudin, V. N. Staroverov, R. Kobayashi, J. Normand, K. Raghavachari, A. P. Rendell, J. C. Burant, S. S. Iyengar, J. Tomasi, M. Cossi, N. Rega, N. J. Millam, M. Klene, J. E. Knox, J. B. Cross, V. Bakken, C. Adamo, J. Jaramillo, R. Gomperts, R. E. Stratmann, O. Yazyev, A. J. Austin, R. Cammi, C. Pomelli, J. W. Ochterski, R. L. Martin, K. Morokuma, V. G. Zakrzewski, G. A. Voth, P. Salvador, J. J. Dannenberg, S. Dapprich, A. D. Daniels, Ö. Farkas, J. B. Foresman, J. V. Ortiz, J. Cioslowski and D. J. Fox, Gaussian 016, Revision E.01, Gaussian, Inc., Wallingford CT, 2016 Search PubMed
.
- S. Grimme, S. Ehrlich and L. Goerigk, Effect of the Damping Function in Dispersion Corrected Density Functional Theory, J. Comput. Chem., 2011, 32, 1456 CrossRef CAS
.
- R. Saha, P. Nepal, C. Feng, M. S. Hossain, M. Fukuto, R. Li, J. T. Gleeson, S. Sprunt, R. J. Twieg and A. Jákli, Multiple Ferroelectric Nematic Phases of a Highly Polar Liquid Crystal Compound, Liq. Cryst., 2022, 49, 1784 CrossRef CAS
.
-
P. G. M. Wuts and T. W. Greene, Protection for Phenols and Catechols, in Greene's Protective Groups in Organic Synthesis, John Wiley & Sons, Ltd, 2006, vol. 4, pp. 367–430 Search PubMed
.
- G. Cevasco, G. Guanti, A. R. Hopkins, S. Thea and A. Williams, A Novel Dissociative Mechanism in Acyl Group Transfer from Aryl 4-Hydroxybenzoates in Aqueous Solventst, J. Org. Chem., 1985, 50, 479 CrossRef CAS
.
- I. H. Um, J. Y. Lee, M. Fujio and Y. Tsuno, Structure-Reactivity Correlations in Nucleophilic Substitution Reactions of Y-Substituted Phenyl X-Substituted Benzoates with Anionic and Neutral Nucleophiles, Org. Biomol. Chem., 2006, 4, 2979 RSC
.
- J. A. Seo, S. I. Kim, Y. J. Hong and I. H. Um, Effect of Alkali Metal Ions on Nucleophilic Substitution Reactions of 4-Nitrophenyl X-Substituted Benzoates with Alkali Metal Ethoxides in Anhydrous Ethanol, Bull. Korean Chem. Soc., 2010, 31, 303 CrossRef CAS
.
- E. Cruickshank, A. Pearson, S. Brown, J. M. D. Storey, C. T. Imrie and R. Walker, The Ferroelectric Nematic Phase: On the Role of Lateral Alkyloxy Chains, Liq. Cryst., 2023 DOI:10.1080/02678292.2023.2221651
.
- J. Li,
et al., How Far Can We Push the Rigid Oligomers/Polymers toward Ferroelectric Nematic Liquid Crystals?, J. Am. Chem. Soc., 2021, 143, 17857 CrossRef CAS PubMed
.
- D. S. Hulme, E. P. Raynes and K. J. Harrison, Eutectic Mixtures of Nematic 4′-Substitued 4-Cyanobiphenyls, J. Chem. Soc., Chem. Commun., 1974, 98–99 RSC
.
- R. J. Mandle, In Silico Interactome of a Room-Temperature Ferroelectric Nematic Material, Crystals, 2023, 13, 857 CrossRef CAS
.
- H. Nishikawa, M. Kuwayama, A. Nihonyanagi, B. Dhara and F. Araoka, Rapid, Solvent-Minimized and Sustainable Access to Various Types of Ferroelectric-Fluid Molecules by Harnessing Mechano-Chemical Technology, J. Mater. Chem. C, 2023, 11, 12525 RSC
.
- J. Y. C. Lim and P. D. Beer, Sigma-Hole Interactions in Anion Recognition, Chem, 2018, 4, 731 CAS
.
- L. C. Roper, C. Präsang, V. N. Kozhevnikov, A. C. Whitwood, P. B. Karadakov and D. W. Bruce, Experimental and Theoretical Study of Halogen-Bonded Complexes of DMAP with Di-and Triiodofluorobenzenes. A Complex with a Very Short N⋯I Halogen Bond, Cryst. Growth Des., 2010, 10, 3710 CrossRef CAS
.
|
This journal is © The Royal Society of Chemistry 2023 |