Insights into size-segregated distribution of benzothiazoles in indoor aerosol from office environments†
Received
13th March 2024
, Accepted 12th April 2024
First published on 18th April 2024
Abstract
Understanding the impact of rubber-derived particles on indoor environmental quality is crucial for effective environmental management. Emissions from both roads and textiles are recognized contributors to pollution across diverse environments, including indoor spaces. Benzothiazoles and their derivatives serve as valuable tracers for identifying pollutant sources. In this study, a total of eight benzothiazoles were determined in indoor size-segregated aerosol samples collected from 6th November to 11th December 2023 in Mestre-Venice. The selected offices host both university personnel and students. The results indicate that SO3H-BTH, SH-BTH and OH-BTH were the most concentrated benzothiazoles in aerosol samples. The inhaled daily intake remained low if compared with previous studies, but higher values were found in fine particles (<0.56 μm). Despite the presence of an advanced filtration system, BTHs have been detected across various dimensional fractions, indicating an internal source. Considering that most of the benzothiazoles were distributed in the finest fraction, the findings raise concerns about their capability to reach alveoli and causing health issues.
Environmental significance
The present study addresses the determination of BTHs in indoor size-segregated particles, employing them as tracers for rubber and textiles. Our findings underscore that the majority of BTHs predominantly inhabit fine particles, yet their sporadic occurrence in the coarse mode is not insignificant. Notably, BTH-SO3H and BTH-SH emerge as the most concentrated benzothiazoles, predominantly found in fine particles. This distribution pattern suggests that BTHs in indoor aerosols may originate from local sources like textiles, shoe abrasion, or building materials. Our results indicate that the EDIinh is higher for ultrafine particles, underscoring the potential health risks associated with exposure to these smaller particulate sizes. This study represents the first documentation of BTHs in size-segregated indoor aerosols, highlighting the need for further investigation into their implications for indoor air quality and human health.
|
1. Introduction
In developed nations, peoples typically allocate approximately 90% of their time indoors.1,2 Within indoor spaces, various materials such as plastics, paints, and construction components can emit pollutants, contributing to the rise in their concentrations.3 Thus, particularly in densely populated urban areas, individuals often find themselves outdoors solely during commuting to and from work or occasional outdoor activities and walks. Consequently, the cumulative exposure to indoor air pollutants is increasingly recognized as a critical factor in understanding and addressing public health concerns.4 Poor ventilation exacerbates this issue, as slow degradation processes indoors can further elevate pollution levels. Elevated temperature and humidity within indoor environments can intensify the concentration of certain pollutants. Additionally, the pursuit of heightened comfort standards in developed regions has increased the demand for biocides, potentially exacerbating indoor air quality concerns. Consequently, inhalation of indoor air emerges as a primary exposure pathway to a multitude of pollutants.5 The recent tightening of building structures leads to decreased air exchange between indoor residential spaces and the external environment. This fact has been linked to a notable rise in the concentration of chemical compounds within confined indoor environments, posing a tangible threat to human health.6
Indoor Air Pollutants (IAPs) can originate from several sources, also generated by materials inside the building itself, or transported from the outside. In this framework, the advancements in synthetic chemistry during the 20th century facilitated the widespread production of pollutants (flame retardants, plasticizers, etc.) on a large scale. This era saw the emergence of various congeners, such as polychlorinated biphenyls (PCBs) and polybrominated biphenyl ethers (PBDEs), among others.7 In addition to the above-mentioned pollutants, rubber-derived particles have attracted increasing concerns in recent years.8 Recently, there has been a noticeable increase in scientific literature addressing the prevalence of emerging high-volume organic compounds, including benzothiazoles (BTHs) in outdoor and indoor aerosols.9–11 The US Environmental Protection Agency has disclosed that the national production volume of BTHs in 2019 amounted to 601 tons. BTHs are utilized in significant quantities across industrial manufacturing and household product sectors.12 They find extensive application as vulcanization accelerators in rubber production, fungicides in paper and leather manufacturing, corrosion inhibitors in antifreeze formulations, ultraviolet light stabilizers in textiles and plastics, and photosensitizers in photography.8,10 BTHs have been found in a wide range of concentrations in influent and effluent,13,14 tap and ground waters,15 surface waters16 and more.8 This extensive detection in many samples suggests potential human exposure. To the best of our knowledge, only a study assessed the concentrations of BTHs in the indoor particulate matter.10 Some studies determined the concentration of some BTHs in indoor and outdoor dust, also fractionating the dust samples for a better risk assessment.10,11,17–19 The concentrations of total BTHs (as the sum of BTH, BTH-OH, NH2-BTH, MeS-BTH) in the particulate and gas phase of indoor offices found by Wan et al.10, (2016) had a median of 17.6 ng m−3 ranging from 6.7 to 99.1 ng m−3.
Due to the widespread use of BTHs in household products, they are expected to be present in indoor air. Although BTHs exhibit low acute toxicities, they have been identified as dermal sensitizers20 and irritants to the respiratory tract.21 Furthermore, studies on laboratory animals have reported that BTHs can inhibit hormone synthesis and induce genotoxicity.22 Thus, determining BTHs in the size-segregated aerosols is of importance due to the recognition that finer particles are deemed the most detrimental to human health. In this study, we conducted a sampling campaign of indoor size-segregated aerosol collected at the Ca' Foscari University of Venice in a corridor of the administrative offices, to investigate the presence of BTHs and to assess the exposures via indoor air inhalation for various age groups. Determining these compounds in aerosol is essential for future evaluations regarding their impacts in the indoor environments, human exposure, and health effects. Furthermore, it helps in assessing their effectiveness as indicators of tyre wear particles resulting from dust resuspension. This is the first study to report the occurrence of a wide group of BTHs in different size fractions of indoor aerosol.
2. Experimental
2.1 Sampling
Aerosol indoor samples were collected in a corridor of the administrative offices of the Scientific Campus of Ca' Foscari University of Venice, located at Mestre-Venezia. The exact location of the sampler is reported in Fig. S1 of the ESI.† The administrative offices are frequented by professors, researchers, students, doctoral candidates, and technical personnel, encompassing an age spectrum ranging from approximately 19 to 70 years. Sampling was performed using a Mini-MOUDI™ 135-6 low-flow cascade impactor (TSI Incorporated, Shoreview, Minnesota, USA). The impactor is equipped with eight stages, with nominal cut points of >18, 10, 5.6, 3.2, 1.8, 1.0, 0.56 and <0.56 μm (Fig. S2†). The flow rate was set at 2 L min−1, with a pressure drop of 1 kPa. The cascade impactor can be affected by sampling artifacts (i.e. the bounding of particles, inter-stage losses, the blow-off of particles and potential losses of semi-volatile compounds) but these are limited only to the back-up filter.23 The low flow rate certainly decreases the temporal sampling resolution, yet it is crucial for keeping the noise of the suction pump below an acceptable limit for personnel in the offices. The sampled volume ranged from 11.6 to 29.2 m3, sampled over a period from 96 to 244 hours. Sample parameters are reported in Table S1.† Particle deposits are collected on 37 mm semicircular substrates made of aluminium for the first 5 stages and on 37 mm circular substrates made of quartz fiber for the back-up stage (both were purchased from TSI Incorporated). Materials and methods are reported in the ESI.†
2.2 Mechanical ventilation system
The general mechanical ventilation system of the building where the aerosol sampling was conducted is managed by five air handling units (Euroclima, model ZHK 2000 S ZL). Each of the units is equipped with the following filtration systems: panel and V-blank filter G2–G4, M5; auto roll filter G3; bag filter G4–F9; compact filter M5–F9; absolute filter H10–H14 and an activated carbon filter. As an example, the average removal efficiency for particles having a diameter of 0.4 μm for the most efficient filters F9 should be ≥95%. A building equipped with filters ranging from G2 to F9 would have varying degrees of particle removal efficiency. The positions of the ventilation units are marked in Fig. S1.†
2.3 Instrumentation and sample treatment
We used benzothiazole-D4 (BTD) as the internal standard purchased from LCG Standards (Teddington, UK). The solid standards were first diluted in MeOH and then in ultrapure water. The instrumental method was developed by Feltracco et al.16, (2023). Briefly, the determination of BTHs was performed using an Ultimate 3000 UHPLC system coupled with a TSQ Altis™ Plus Triple Quadrupole Mass Spectrometer (Thermo Scientific™, ICS-5000, Waltham, USA) using a heated electrospray source (H-ESI). Chromatographic separation was performed using a Betasil™ C18 column (100 × 2.1 I.D. mm, 5 μm) equipped with a guard column Betasil™ Javelin guard C18 10 × 2.1 mm, 5 μm (Thermo Scientific™). Sample treatment and QC/QA were optimized by Favaro et al. (2024, submitted) and Feltracco et al.16 (2023). Briefly, filters were placed in a 5 mL vial (previously decontaminated with MeOH and ultrapure water) with steel tweezers. BTH-D4 was spiked, and 4 mL of 10 μg L−1 ultrapure water was added. The samples were extracted through an ultrasonic bath at 10 °C. The extract was filtered with a 0.45 μm PTFE filter (Biocomma, Guangdong, China) to filter traces or particulates before analysis.
2.4 Human health exposure assessment
Based on the concentrations of BTHs found in indoor air samples, the estimated inhaled daily intake (EDIinh; ng per kg bw per day) of BTHs via inhalation of indoor air was computed according to eqn (1).24 | 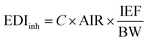 | (1) |
where C is the concentration of BTH air samples (ng m−3), AIR is the air inhalation rate (m3 day−1), IEF is the indoor exposure fraction (the fraction of time spent indoors), and BW is the body weight (kg). We assumed an absorption efficiency of 100% for BTHs from indoor air to systemic blood circulation, as suggested by previous studies.10 Details of the parameters used for estimation of human exposure to through inhalation are reported in Table 1. In the calculation of the EDIinh, only teenagers and adults were considered as individuals attending university are predominantly within that age range.
Table 1 Parameters used for estimation of human exposure to BTHs through inhalation of indoor air.12
Parameters |
Teenagers |
Adults |
Inhalation rate (m3 day−1) (AIR) |
15.8 |
15.7 |
Indoor exposure factor (IEF) |
0.87 |
0.80 |
Body weight (kg) (BW) |
65.0 |
80.0 |
3. Results and discussion
3.1 Concentration of BTHs in the particulate phase and source identification
BTH, BTH-OH, BTH-SH, BTH-Me, BTH-MeS and BTH-SO3H were found in the particulate phase of all indoor air samples, while 2-NH2-BTH and 2-SCNMeS-BTH were found in 22% and 25% of the size-segregated samples, respectively. Considering the total suspended indoor particles, obtained from the sum of all 8 stages sampled, BTH-SO3H was the most abundant compound found (46.4%, average concentration of 2 ± 1 ng m−3), followed by BTH-SH (19.5%, 0.8 ± 0.4 ng m−3), BTH-OH (13.9%, 0.6 ± 0.4 ng m−3) and BTH (12.4%, 0.5 ± 0.3 ng m−3). Other BTHs are below 8%, as shown in Fig. 1 and Table S2.†
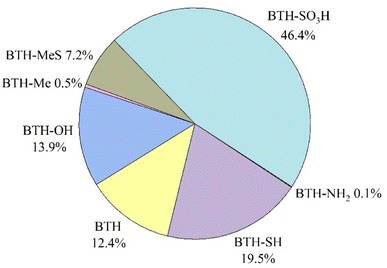 |
| Fig. 1 Relative abundance of benzothiazoles in the total suspended indoor particles. BTH-SCNMeS is not reported because accounted only for 0.02%. | |
To the best of our knowledge, no studies are present on indoor aerosol for the determination of BTH-SO3H and SH-BTH, but they were found in high concentrations in other environmental compartments such as surface waters.16,25 Further investigations are crucial to assess them, as BTH-SO3H appears to be a conversion product of 2-SH-BTH.26 The presence of BTHs in indoor environments is fragmented, making comparisons challenging. Table 2 presents concentrations from studies conducted in similar environments to the office here considered. It is evident that there is significant variability, particularly concerning dust samples. Furthermore, studies often focus on a limited number of benzothiazoles, further complicating comparisons, especially regarding relative abundances.
Table 2 Mean concentration of BTHs in indoor samples. Dust samples are reported in ng g−1 while indoor air samples are reported in ng m−3. BDL = beyond the detection limit
Compound |
Conc. |
Samples |
Site |
Country |
BTH |
360 |
Indoor dust |
Urban homes |
China19 |
BDL |
Indoor dust |
Urban homes |
USA19 |
BDL |
Indoor dust |
Urban homes |
Japan19 |
5.84 |
Indoor dust |
Urban homes |
Korea19 |
60.2 |
Indoor dust |
Bedroom/living rooms |
China11 |
15.6 |
Indoor bulk air |
Offices |
USA10 |
41.6 |
Indoor bulk air |
Homes |
USA10 |
BTH-OH |
472 |
Indoor dust |
Urban homes |
China19 |
1140 |
Indoor dust |
Urban homes |
USA19 |
551 |
Indoor dust |
Urban homes |
Japan19 |
1760 |
Indoor dust |
Urban homes |
Korea19 |
38.5 |
Indoor dust |
Bedroom/living rooms |
China11 |
2.04 |
Indoor bulk air |
Offices |
USA10 |
2.93 |
Indoor bulk air |
Homes |
USA10 |
BTH-NH2 |
BDL |
Indoor dust |
Urban homes |
China19 |
BDL |
Indoor dust |
Urban homes |
USA19 |
BDL |
Indoor dust |
Urban homes |
Japan19 |
BDL |
Indoor dust |
Urban homes |
Korea19 |
1.33 |
Indoor dust |
Bedroom/living rooms |
China11 |
0.07 |
Indoor bulk air |
Offices |
USA10 |
0.08 |
Indoor bulk air |
Homes |
USA10 |
BTH-MeS |
8.43 |
Indoor dust |
Bedroom/living rooms |
China11 |
0.3 |
Indoor bulk air |
Offices |
USA10 |
0.5 |
Indoor bulk air |
Homes |
USA10 |
BTH-MeS |
8.43 |
Indoor dust |
Bedroom/living rooms |
China11 |
0.3 |
Indoor bulk air |
Offices |
USA10 |
0.5 |
Indoor bulk air |
Homes |
USA10 |
BTH-Me |
0.56 |
Indoor dust |
Bedroom/living rooms |
China11 |
BTH-SCNMeS |
2.8 |
Indoor dust |
Urban homes |
China19 |
10.9 |
Indoor dust |
Urban homes |
USA19 |
19.7 |
Indoor dust |
Urban homes |
Japan19 |
15.8 |
Indoor dust |
Urban homes |
Korea19 |
0.57 |
Indoor dust |
Bedroom/living rooms |
China11 |
Mean values and standard deviation of studied BTHs of this study in total suspended particles (TSP), PM10 and PM1 are reported in Table 3. These values are about 1 order of magnitude lower than those found by Wan et al.10 (2016) in the bulk air.
Table 3 Mean concentration (ng m−3) of observed BTHs
|
BT-NH2 |
BTH-SH |
BTH |
BTH-OH |
BTH-Me |
BTH- SCNMeS |
BTH-MeS |
BTH-SO3H |
TSP |
0.004 ± 0.002 |
0.8 ± 0.4 |
0.5 ± 0.3 |
0.6 ± 0.4 |
0.02 ± 0.01 |
0.001 ± 0.001 |
0.3 ± 0.1 |
2 ± 1 |
PM10 |
0.004 ± 0.002 |
0.6 ± 0.3 |
0.3 ± 0.2 |
0.5 ± 0.3 |
0.02 ± 0.01 |
0.001 ± 0.001 |
0.2 ± 0.1 |
2 ± 1 |
PM1 |
0.004 ± 0.002 |
0.5 ± 0.2 |
0.2 ± 0.1 |
0.4 ± 0.2 |
0.01 ± 0.01 |
0.001 ± 0.001 |
0.2 ± 0.1 |
2 ± 1 |
BTHs presented variable concentrations among the fractions. BTH-SO3H was mainly distributed in fine particles (<1 μm) with mean concentration reaching 2 ± 1 ng m−3 in the PM1. The same trend was observed for BTH-SH and BTH-OH, with mean concentrations in PM1 of 0.5 ± 0.2 and 0.4 ± 0.2 ng m−3. BTH followed a different behaviour, with no clear trend among fractions (Fig. 2).
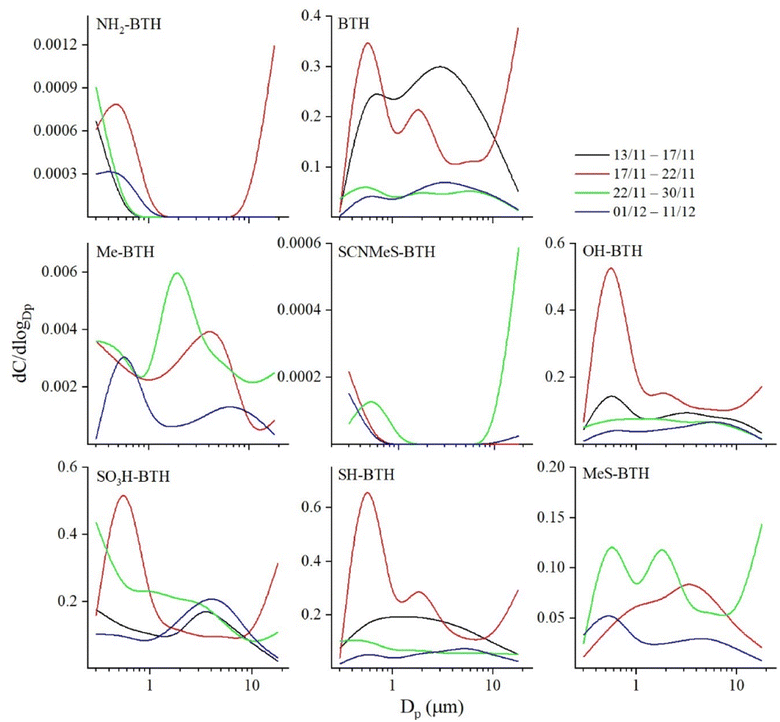 |
| Fig. 2 Size distribution of target compounds. | |
The presence of BTHs in air fresheners, paints, and rubber insulating materials may account for their presence in indoor aerosol.27 BTH, SH-BTH, and MeS-BTH have been discovered not only in the abovementioned material but also in textile clothing. For example, the levels of these compounds in car tires ranged from 0.46 μg g−1 (for MeS-BTH) to 23.5 μg g−1 (for BTH), which were notably higher compared to textile samples, where concentrations ranged from 0.73 to 8.64 μg g−1.28 These findings suggest that wearing clothing may serve as a potential route of human exposure to emerging chemicals. The substantial presence of BTHs in polyester suggests that they are likely utilized in alternative processes or are contaminants from other additives used in production.
Despite a possible source from the outdoor environment, mainly related to windows and door opening, we believe that the tendency of BTHs to be distributed in the fine fractions suggests that the main source is related to the release of small, aged particles from various textile materials. Another plausible explanation for the distribution of BTHs among fractions could be their potential adsorption onto existing small particles such as sand or asphalt fragments. This implies that increased surface area enhances the adsorption and environmental availability of BTHs.16,29 These results were also observed in road dust samples, where the BTHs tended to be distributed in small particles.16
The aging of the particles appears to be explained by the presence of SO3H-BTH and OH-BTH, which are degradation products of SH-BTH and BTH.30 SH-BTH holds significance as a key industrial compound utilized in rubber and rubber product manufacturing, serving as the precursor for various chemical compounds. SH-BTH is the most widely used compound for this purpose.8 Exact production information for SO3H-BTH derivatives is not available and no clear degradation pathway is proposed for SH-BTH that could turn into SO3H-BTH.31 The notably high concentration of SO3H-BTH serves as a clear indication that SH-BTH has undergone degradation, and atmospheric photochemical reactions may facilitate this oxidation process. OH-BT has been recognized as a byproduct of BTH oxidation,32 with laboratory experiments conducted in a previous study19 confirming that elevated temperatures lead to the conversion of BTH into OH-BT within car tire rubber particles. Hence, it is possible that a portion of OH-BTH originates from the oxidation of BTH during friction events.33
In the indoor environment under consideration, it is possible that the presence of vulcanizing agents in rubber could be a primary source, given the widespread use of rubber in shoe soles and the atmospheric emission from their abrasion during walking. Thus, tires and other rubber products are added in amounts over 1% by weight of these additives.10,34 Thus, Lai et al.35 (2017) conducted in a controlled environmental chamber some walking-induced resuspension experiments, in order to investigate the effects of shoe type and shoe groove pattern on particle resuspension. The study interprets the dimensional distribution of the generated aerosol, ranging from 1 to 10 μm. It shows that particles with diameters smaller than 1 micron exhibit a concentration at least 20 times lower compared to larger particles. This finding suggests that the presence of some benzothiazoles in the coarse mode (Fig. 2) may be attributed to resuspension or shoe sole abrasion, whereas finer mode may indicate another source, likely outdoor. To sum up, we identified three major sources: (1) textile materials, likely the most probable source; (2) shoe abrasion; (3) building materials; (4) outdoor sources, due to the presence of fine and ultrafine particles.
3.2 Human exposure
Age, duration of indoor environment exposure, and inhalation rate impact air pollutant exposure levels (see Table 1). Inhalation exposure to BTHs was derived from measured bulk air concentrations. To assess BTH inhalation EDI, we segmented the population into two age brackets: teenagers (12–21 years) and adults (≥21 years), following the US EPA Exposure Factors Handbook24 (Fig. 3). Considering variations in body weight (Table 1), exposure doses of BTHs from indoor TSP air for adults were calculated to be slightly lower for teenagers (0.63 and 0.85 ng per kg bw per day for adults and teenagers, respectively). Fig. 3 shows that estimated inhaled daily intake (EDIinh) is significantly more prominent when considering the finer fraction sampled. This result assumes even greater significance when considering that submicron particles can reach the alveoli, inducing adverse health effects in humans. As expected, BTH-SO3H showed greater values of EDIinh for both categories. The determination of BTH-SO3H is of great significance because it often represents the most concentrated form of BTH in various environmental compartments. This study supports this trend, highlighting its importance in environmental monitoring and assessment. The EDIinh values for total BTHs were about one order of magnitude lower than the exposure doses derived from indoor bulk air of BTHs and its metabolites in USA offices10 and similar to values found for children and adults in the USA and three East Asian Countries.19
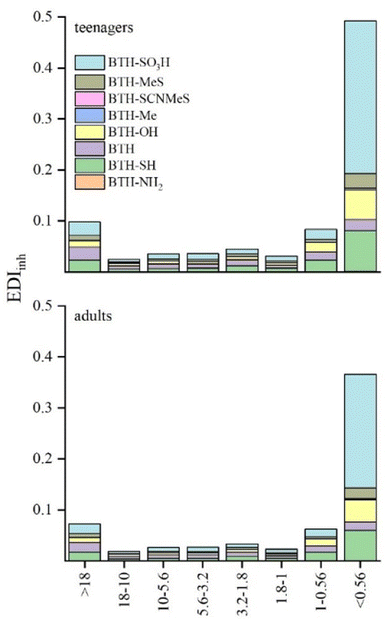 |
| Fig. 3 Estimated daily intake (EDIinh, ng per kg bw per day) of BTHs through inhalation of indoor air for teenagers and adults in the different size fractions. | |
The low values found in this study, both in terms of concentrations and EDIinh, are likely attributed to the efficient filtration system of the building, which was completed in 2015. To the best of our knowledge, this is the first study that describes the presence of BTHs in size-segregated indoor aerosol.
4. Conclusion
Studies on the determination of emerging contaminants in indoor air remain scarce, necessitating a deeper understanding of their potential harm to humans, particularly in environments where individuals spend most of their time, such as workplaces and offices. The present study faces the determination of BTHs in indoor size-segregated particles, using them as rubber and textiles tracers. The findings reveal that most of the BTHs were mainly distributed in the fine particles, but their seldom presence in the coarse mode was not negligible. Specifically, BTH-SO3H and BTH-SH are the most concentrated benzothiazoles, and they have also exhibited a higher presence in fine particles. The distribution suggested that BTHs in indoor aerosol can derive from textiles, shoe scraping or building materials as local sources. An external source cannot be excluded, despite the presence of the filtration system of the building. In fact, considering both concentrations and EDIinh, this study presents lower values than previously published papers. The present manuscript includes a limited number of samples and a low sample volume was sampled. The latter is attributed to the minimal noise generated by the suction pump in a public setting, aimed at minimizing disruption to administrative personnel working nearby. In future studies, efforts are required to increase the sample size by incorporating diverse sampling locations, thus enhancing the obtained information. However, to the best of our knowledge, this is the first study that describes the presence of BTHs in size-segregated indoor aerosol.
Conflicts of interest
There are no conflicts to declare.
Acknowledgements
The authors thank Elga Lab water, High Wycombe UK for supplying the pure water systems used in this study. This work has benefited from the infrastructural support of the Centre for Trace Analysis (CeTrA) of Ca' Foscari University through the project IR0000032 – ITINERIS, Italian Integrated Environmental Research Infrastructures System, funded by EU – Next Generation EU, PNRR – Mission 4 “Education and Research” – Component 2: “From research to business” – Investment 3.1: “Fund for the realisation of an integrated system of research and innovation infrastructures”. The authors thank the Administration, Educational Programmes, and Research Offices of the Department of Environmental Sciences, Informatics, and Statistics of Ca' Foscari University of Venice for their assistance. The study was carried out with the co-financing of the European Union FSE REACT-EU, PON Ricerca e Innovazione 2014–2020.
References
- N. Hamidin, J. Yu, D. T. Phung, D. Connell and C. Chu, Volatile aromatic hydrocarbons (VAHs) in residential indoor air in Brisbane, Australia, Chemosphere, 2013, 92, 1430–1435 Search PubMed.
- J. M. Delgado-Saborit, N. J. Aquilina, C. Meddings, S. Baker and R. M. Harrison, Relationship of personal exposure to volatile organic compounds to home, work and fixed site outdoor concentrations, Sci. Total Environ., 2011, 409, 478–488 CrossRef CAS PubMed.
- L. Mølhave, G. Clausen, B. Berglund, J. De Ceaurriz, A. Kettrup, T. Lindvall, M. Maroni, A. C. Pickering, U. Risse, H. Rothweiler, B. Seifert and M. Younes, Total Volatile Organic Compounds (TVOC) in Indoor Air Quality Investigations, Indoor Air, 1997, 7, 225–240 CrossRef.
-
K. E. Paleologos, M. Y. E. Selim and A.-M. O. Mohamed, in Pollution Assessment for Sustainable Practices in Applied Sciences and Engineering, ed. A.-M. O. Mohamed, E. K. Paleologos and F. M. Howari, Butterworth-Heinemann, 2021, pp. 405–489 Search PubMed.
- C. Garcia-Jares, J. Regueiro, R. Barro, T. Dagnac and M. Llompart, Analysis of industrial contaminants in indoor air. Part 2. Emergent contaminants and pesticides, J. Chromatogr. A, 2009, 1216, 567–597 CrossRef CAS PubMed.
- N. Shinohara, Y. Kai, A. Mizukoshi, M. Fujii, K. Kumagai, Y. Okuizumi, M. Jona and Y. Yanagisawa, On-site passive flux sampler measurement of emission rates of carbonyls and VOCs from multiple indoor sources, Build. Sci., 2009, 44, 859–863 Search PubMed.
- T. Salthammer, Emerging indoor pollutants, Int. J. Hyg. Environ. Health, 2020, 224, 113423 CrossRef CAS PubMed.
- C. Liao, U. J. Kim and K. Kannan, A Review of Environmental Occurrence, Fate, Exposure, and Toxicity of Benzothiazoles, Environ. Sci. Technol., 2018, 52, 5007–5026 CrossRef CAS PubMed.
- A. Maceira, R. M. Marcé and F. Borrull, Occurrence of benzothiazole, benzotriazole and benzenesulfonamide derivates in outdoor air particulate matter samples and human exposure assessment, Chemosphere, 2018, 193, 557–566 CrossRef CAS PubMed.
- Y. Wan, J. Xue and K. Kannan, Benzothiazoles in indoor air from Albany, New York, USA, and its implications for inhalation exposure, J. Hazard. Mater., 2016, 311, 37–42 Search PubMed.
- Q. Zhu, C. Liao and G. Jiang, Occurrence of Human Exposure to Benzothiazoles and Benzotriazoles in Indoor Dust in Suizhou and Beijing, China, Chem. Res. Chin. Univ., 2023, 39, 508–515 Search PubMed.
-
O. US EPA, Chemicals, Pesticides and Toxics Topics, https://www.epa.gov/environmental-topics/chemicals-pesticides-and-toxics-topics, (accessed 28 February 2024).
- A. G. Asimakopoulos, A. Ajibola, K. Kannan and N. S. Thomaidis, Occurrence and removal efficiencies of benzotriazoles and benzothiazoles in a wastewater treatment plant in Greece, Sci. Total Environ., 2013, 452–453, 163–171 CrossRef CAS PubMed.
- D. Salas, F. Borrull, R. M. Marcé and N. Fontanals, Study of the retention of benzotriazoles, benzothiazoles and benzenesulfonamides in mixed-mode solid-phase extraction in environmental samples, J. Chromatogr. A, 2016, 1444, 21–31 CrossRef CAS PubMed.
- A. Speltini, M. Sturini, F. Maraschi, A. Porta and A. Profumo, Fast low-pressurized microwave-assisted extraction of benzotriazole, benzothiazole and benezenesulfonamide compounds from soil samples, Talanta, 2016, 147, 322–327 CrossRef CAS PubMed.
- M. Feltracco, G. Mazzi, E. Barbaro, B. Rosso, F. Sambo, S. Biondi, C. Barbante and A. Gambaro, Occurrence and phase distribution of benzothiazoles in untreated highway stormwater runoff and road dust, Environ. Sci. Pollut. Res., 2023, 30, 107878–107886 CrossRef CAS PubMed.
- C. Deng, J. Huang, Y. Qi, D. Chen and W. Huang, Distribution patterns of rubber tire-related chemicals with particle size in road and indoor parking
lot dust, Sci. Total Environ., 2022, 844, 157144 CrossRef CAS PubMed.
- W. Li, J. Li, M. Deng, Y. Pan and L. Zeng, Benzotriazoles and benzothiazoles prevail in indoor dust from an E-waste dismantling area in South China: Elevated concentrations and implication for human exposure, Sci. Total Environ., 2020, 723, 137979 CrossRef CAS PubMed.
- L. Wang, A. G. Asimakopoulos, H.-B. Moon, H. Nakata and K. Kannan, Benzotriazole, Benzothiazole, and Benzophenone Compounds in Indoor Dust from the United States and East Asian Countries, Environ. Sci. Technol., 2013, 47, 4752–4759 CrossRef CAS PubMed.
- X. Wang and R. R. Suskind, Comparative studies of the sensitization potential of morpholine, 2-mercaptobenzothiazole and 2 of their derivatives in guinea pigs, Contact Dermatitis, 1988, 19, 11–15 CrossRef CAS PubMed.
- G. Ginsberg, B. Toal and T. Kurland, Benzothiazole Toxicity Assessment in Support of Synthetic Turf Field Human Health Risk Assessment, J. Toxicol. Environ. Health, Part A, 2011, 74, 1175–1183 CrossRef CAS PubMed.
- M. W. Hornung, P. A. Kosian, J. T. Haselman, J. J. Korte, K. Challis, C. Macherla, E. Nevalainen and S. J. Degitz,
In Vitro, Ex Vivo, and In Vivo Determination of Thyroid Hormone Modulating Activity of Benzothiazoles, Toxicol. Sci., 2015, 146, 254–264 CrossRef CAS PubMed.
- D. Contini, D. Cesari, A. Genga, M. Siciliano, P. Ielpo, M. R. Guascito and M. Conte, Source apportionment of size-segregated atmospheric particles based on the major water-soluble components in Lecce (Italy), Sci. Total Environ., 2014, 472, 248–261 CrossRef CAS PubMed.
-
U.S. EPA, Exposure Factors Handbook 2011 Edition (Final Report), U.S. Environmental Protection Agency, Washington, DC, 2011, EPA/600/R-09/052F Search PubMed.
- T. Reemtsma, Determination of 2-substituted benzothiazoles of industrial use from water by liquid chromatography/electrospray ionization tandem mass spectrometry, Rapid Commun. Mass Spectrom., 2000, 14, 1612–1618 CrossRef CAS PubMed.
- V. Hinnenkamp, P. Balsaa and T. C. Schmidt, Target, suspect and non-target screening analysis from wastewater treatment plant effluents to drinking water using collision cross section values as additional identification criterion, Anal. Bioanal. Chem., 2022, 414, 425–438 CrossRef CAS PubMed.
- A. Kloepfer, M. Jekel and T. Reemtsma, Occurrence, sources, and fate of benzothiazoles in municipal wastewater treatment plants, Environ. Sci. Technol., 2005, 39, 3792–3798 CrossRef CAS PubMed.
- R. Avagyan, I. Sadiktsis, G. Thorsén, C. Östman and R. Westerholm, Determination of benzothiazole and benzotriazole derivates in tire and clothing textile samples by high performance liquid chromatography–electrospray ionization tandem mass spectrometry, J. Chromatogr. A, 2013, 1307, 119–125 CrossRef CAS PubMed.
- S. Ersahin, H. Gunal, T. Kutlu, B. Yetgin and S. Coban, Estimating specific surface area and cation exchange capacity in soils using fractal dimension of particle-size distribution, Geoderma, 2006, 136, 588–597 CrossRef CAS.
- H. De Wever and H. Verachtert, Biodegradation and toxicity of benzothiazoles, Water Res., 1997, 31, 2673–2684 CrossRef CAS.
- H. De Wever and H. Verachtert, Biodegradation and toxicity of benzothiazoles, Water Res., 1997, 31, 2673–2684 CrossRef CAS.
- N. Haroune, B. Combourieu, P. Besse, M. Sancelme, T. Reemtsma, A. Kloepfer, A. Diab, J. S. Knapp, S. Baumberg and A.-M. Delort, Benzothiazole Degradation by Rhodococcus pyridinovorans Strain PA: Evidence of a Catechol 1,2-Dioxygenase Activity, Appl. Environ. Microbiol., 2002, 68, 6114–6120 CrossRef CAS PubMed.
- J. Zhang, X. Zhang, L. Wu, T. Wang, J. Zhao, Y. Zhang, Z. Men and H. Mao, Occurrence of benzothiazole and its derivates in tire wear, road dust, and roadside soil, Chemosphere, 2018, 201, 310–317 CrossRef CAS PubMed.
- H. G. Ni, F. H. Lu, X. L. Luo, H. Y. Tian and E. Y. Zeng, Occurrence, phase distribution, and mass loadings of benzothiazoles in riverine runoff
of the Pearl River Delta, China, Environ. Sci. Technol., 2008, 42, 1892–1897 CrossRef CAS PubMed.
- A. C. K. Lai, Y. Tian, J. Y. L. Tsoi and A. R. Ferro, Experimental study of the effect of shoes on particle resuspension from indoor flooring materials, Build. Sci., 2017, 118, 251–258 Search PubMed.
|
This journal is © The Royal Society of Chemistry 2024 |
Click here to see how this site uses Cookies. View our privacy policy here.