DOI:
10.1039/D4EA00047A
(Communication)
Environ. Sci.: Atmos., 2024,
4, 1352-1357
Determination of mass concentrations of airborne PET microplastics using liquid chromatography coupled to tandem mass spectrometry (LC-MS/MS)†
Received
18th April 2024
, Accepted 2nd October 2024
First published on 4th October 2024
Abstract
Microplastics (MPs) have recently become a growing environmental pollution concern. MPs are easily transferred and ubiquitously found in ambient air. MPs in the air can act as carriers for several toxic pollutants, and exposure to MPs can lead to pulmonary diseases in humans. Polyethylene terephthalate (PET) is one of the most abundant MPs used in the manufacturing of various fibres and plastics. In this study, we present a method for the determination of mass concentrations of PET MPs in the airborne inhalable fraction of fine particulate matter (PM2.5) using liquid chromatography coupled to tandem mass spectrometry (LC-MS/MS). Teflon and quartz fiber filters were tested for extraction efficiency in measuring the mass concentrations of airborne PET MPs. Teflon filters showed good recovery (80–120%) compared to quartz fiber filters. Using this method, a pilot study was carried out in Delhi, the national capital of India, and Mohali, a suburban city in the northwest Indo-Gangetic Plain (NWIGP), for the determination of mass concentrations of PET MPs present in airborne PM2.5. The observed maximum mass concentrations of PET MPs in airborne PM2.5 in Delhi and Mohali are 135.20 ng m−3 and 157.98 ng m−3, respectively.
Environmental significance
Airborne microplastics (MPs) are a major concern due to their possible health risks and their ability to act as carrier for several toxic air pollutants. PET is the most abundant airborne MP, and in vitro studies reported that exposure to PET MPs can cause pulmonary disorders (>98.6 μg mL−1). Therefore, the determination of mass concentrations of airborne PET MPs is needed to understand the various additional health risks associated with their inhalation exposure. In this study, we reported an optimized LC-MS/MS method for the mass quantification of PET MPs present in the ambient PM2.5 and carried out a pilot study in Delhi and Mohali. We believe that the findings in this study provide a better understanding of the chemical composition of airborne MPs and the associated health risks.
|
1 Introduction
Microplastics (MPs) are plastic debris with sizes ranging from 1 μm to 5 mm.1,2 There are myriad sources of airborne microplastics in the ambient atmosphere. MPs enter the atmosphere from fibres of textiles, erosion of rubber tyres, building materials, solid waste burning, resuspension of emissions from traffic and industries, sewage sludge used as a fertilizer, air blasting media, degradation of larger particles when exposed to UV radiation, and tumble dryer exhaust.3–5 Due to their size and density, MPs are easily transferred to air and transported by wind to longer distances of up to 100 km, causing a potential health risk when inhaled continuously by humans.4,6–8 MPs in the air can act as carriers for heavy metals, microorganisms and carcinogenic polycyclic aromatic hydrocarbons due to their large specific surface area and sorption capacity, and thus, inhalation exposure to MPs increases the potential cancer risk.9–12 Earlier studies reported that PET is the most abundant microplastic present in ambient particulate matter in the urban sites of India and China.13,14 The inhalable fraction of airborne particulate matter, with an aerodynamic equivalent diameter less than or equal to 2.5 μm (PM2.5), can reach deeper into the human body and pose further health risks.15–18 Inhalation of microplastics in airborne PM2.5 can lead to diseases such as asthma, extrinsic allergic alveolitis, chronic pneumonia, inflammation, bronchitis, and pneumothorax.19,20In vitro toxicity studies have shown that PET nanoplastics can result in the formation of reactive oxygenated species, causing cellular oxidative stress.21 PET is also known to cause vascular prostheses due to the suppression of proliferation and migration of endothelial and mesenchymal cells.22 Recent in vitro studies have shown that exposure to PET MPs can cause pulmonary disorders if the concentrations of PET MPs exceed more than 98.6 μg mL−1.21 Also, in vivo studies reported that exposure to PET MPs above 200 ppm can cause hepatoxicity and neurotoxicity in zebrafish and Drosophila melanogaster, respectively.23,24 Hence, it is important to carry out the measurements of mass concentrations of PET MPs in airborne PM2.5 to understand the various additional health risks associated with it.
In most studies, measurements of MPs were carried out extensively using Fourier-transform infrared (FTIR) and Raman spectroscopic techniques. However, these spectroscopic techniques suffer from several artifacts due to interference with the sample matrix, and they also become troublesome for the analysis of MPs when the particle size is below 0.5 μm.25 Other analytical techniques, namely, transmission electron microscopy, scanning electron microscopy, and dynamic light scattering, are also being used in MP studies. Nevertheless, these microscopic techniques can only provide information about particle number, size, and shape but fail to identify the type of polymer present in the given sample.26,27 With the rapid development in various mass spectrometric techniques, pyrolysis gas chromatography coupled to mass spectrometry (py-GC-MS) and thermogravimetry coupled with mass spectrometry techniques (TGA-MS) are being used for the characterization of microplastics present in various sample matrices.28,29 Note that these techniques require bulk sample for analysis, are suitable only for specific polymers, and are semi-quantitative techniques.30 Analysis time with LC-MS/MS is less than the GC-MS/MS technique. In GC-MS/MS analysis, conditioning of oven temperature is time-consuming compared to LC-MS/MS.
In this study, we developed a method for the determination of mass concentrations of PET MPs in the inhalable fraction of ambient PM2.5 using the state-of-the-art instrumentation technique, namely, liquid chromatography coupled to tandem mass spectrometry (LC-MS/MS). To date, no studies have reported the mass concentrations of PET MPs in the inhalable fraction of ambient PM2.5. Using this method, a pilot study was carried out to screen and measure the mass concentrations of PET MPs in the inhalable fraction of ambient PM2.5 in Delhi, the national capital of India, and Mohali, a suburban city in the northwest Indo-Gangetic Plain.
2 Materials and methods
2.1 Chemicals
Polyethylene terephthalate (PET; CAS No.: 25038-59-9, Product No.: 429252-250G) was procured from Sigma Aldrich, Saint Louis, MO 63103, USA. Terephthalic acid (CAS No.: 100-21-0, purity: >99.0%) and pentanol (CAS No.: 71-41-0, purity: >99.0%) were purchased from Tokyo Chemical Industry Co., Ltd, Japan. LC-MS grade formic acid (CAS No.: 64-18-6) and methanol (CAS No.: 67-56-1) were obtained from Fisher Chemical, Belgium. HPLC-grade methanol and potassium hydroxide pellets (1310-58-3) were procured from Merck Life Science Private Limited, Mumbai, India. HPLC-grade trifluoroacetic acid (CAS No.: 76-05-1) was procured from Spectrochem Pvt Ltd, Mumbai, India. High-purity (PicoPure plus grade) hydrochloric acid (CAS No.: 7647-01-0) was purchased from Chem-Lab, Canada. Type 1 Milli Q water (18 MΩ cm) was purified using a Milli Q IQ 7000 ultra-pure water purification system.
2.2 Sample collection
In Mohali, ambient PM2.5 samples were collected on Teflon filters (Whatman membrane filters, Cat No.: 7585-004, pore size: 0.5 μm and diameter: 47 mm) using a U.S. EPA-approved Speciation Aerosol Sampling System (Met One Instruments, Inc., OR, USA) at a flow rate of 6.7 L min−1 for 24 h (04:00 PM to the following day 04:00 PM, IST) between 16/10/2022 to 11/11/2022, which were installed on the rooftop of academic building 2, IISER Mohali (30°39′52′′N 76°43′43′′E). A detailed description of the sampling site is given in our previously reported study.31 A total of seven samples were collected, and their results are presented in this study.
Ambient PM2.5 samples in Delhi were collected from a rooftop in Mausam Bhawan, Indian Meteorological Department (IMD), Lodhi Colony, Delhi (28°35′22′′N 77°13′14′′E) from 16/10/2022 to 04/11/2022. A total of four samples were collected as a part of the pilot study. A custom-designed PM2.5 cyclone (Mars Bioanalytical, India) equipped with a mass flow controller (Bronckhorst, Holland) and low-volume vacuum pump was used for the collection of PM2.5 samples onto Teflon filters (polytetrafluoroethylene (PTFE), Whatman membrane filters, Cat No.: 7585-004, pore size: 0.5 μm and diameter: 47 mm) at a flow rate of 12.6 L min−1 for 24 h (02:00 PM to the following day 02:00 PM, IST).
All the collected samples were stored at 4 °C until the analysis of PET MPs was performed using LC-MS/MS, facilitated at the Sri Sathya Sai Institute of Higher Learning – Central Research Instruments Facility, Puttaparthi, Andhra Pradesh, India. Fig. S1† shows the sampling locations, sampling sites and PM2.5 samplers used for the collection of PM2.5 samples in this study.
2.3 Extraction and depolymerization of airborne polyethylene terephthalate microplastics
In this study, the extraction and depolymerisation of PET microplastics present in the inhalable fraction of particulate matter were carried out using a reported method for sediment samples.32 Filter samples were extracted using 5 mL of HPLC-grade methanol for 15 minutes under ultrasonication. This process was repeated twice, and the methanol layers were pooled into a round-bottomed flask. An aliquot of 200 μL was syringe-filtered (PTFE syringe filter, 0.22 μm) from the pooled methanol layers for determining the free terephthalic acid (terephthalic acid present in the non-depolymerized sample). The remaining methanol fraction was allowed to dry completely under the gentle flow of N2 gas. The obtained residue was then refluxed with 35 mg of KOH and 10 mL of n-pentanol at 135 °C for thirty minutes. After the reflux, the reaction mixture was allowed to cool to room temperature, and extraction was carried out with 10 mL of HPLC-grade water using an orbital shaker (180 rpm; Make: Labnet; Model: Orbit™ 1900 Digital Heavy Duty Shaker) followed by centrifugation (3000 rpm; Rotor radius: 144 mm; Make: Thermo Scientific; Model: Sorvall™ ST 8 Small Benchtop Centrifuge) for ten minutes each. The extracted aqueous layer was adjusted to pH 2–3 using high-purity hydrochloric acid. Further, unwanted compounds were eliminated from the pH-adjusted aqueous layer using Agilent technologies HF Bond Elut – C18 solid phase cartridges (Part No.: 14102052). Note that the SPE cartridges were conditioned using 12 mL of HPLC-grade methanol, followed by 12 mL of HPLC-grade water prior to their use. The extracted aqueous layer containing the depolymerized fraction of TPA was analysed with LC-MS/MS as described in Section 2.4. The remanent fraction of TPA on the SPE cartridge was washed with methanol and analysed with LC-MS/MS. All the samples were syringe-filtered through a 0.22 μm PTFE filter before injecting into the LC-MS/MS. Note that the TPAdeploy used in eqn (1) (Section 2.5) represents the fraction of TPA obtained from both the SPE-treated aqueous and methanol extracts.
2.4 Liquid chromatography – mass spectrometry analysis
In this study, an ultra-high-performance liquid chromatography system (UHPLC; Model Name: Agilent Technologies, 1290 infinity series) coupled to a triple quadrupole mass spectrometer (Model Name: Agilent Technologies, 6490 iFunnel) with electrospray ionization (ESI) source in negative ionization mode was used for the identification and quantification of terephthalic acid present in the non-depolymerized and depolymerized fractions of PM2.5 extracts. The Agilent Zorbax Eclipse Plus Rapid Resolution High-Definition C18 column (internal diameter: 3.0, column length: 100 mm, and particle size: 1.8 μm) was used for the separation of analytes. Chromatographic separation was carried out with a binary mobile phase system in gradient mode. Mobile phases used in this study were 0.01% formic acid in water and 100% methanol as mobile phase A and mobile phase B, respectively. The volume of the sample injected into the LC-MS/MS instrument was 10 μL. The gradient composition of the mobile phase is given in Table S1.† Terephthalic acid was eluted from the column at 5.3 min using the above liquid chromatography parameters. Multiple reaction monitoring (MRM) mode was adopted for the identification of terephthalic acid (TPA) using LC-MS/MS. Source parameters of the mass spectrometer and the MRM conditions used for identification of TPA are given in Table S2.† In this method, 165.0–121.0 and 165.0–77.0 are the transitions used as quantifier and qualifier ions, respectively, for TPA identification. Fig. S2† shows the total ion chromatogram and extracted ion chromatograms for the two targeted transitions of standard terephthalic acid.
2.5 Measurement of mass concentrations of polyethylene terephthalate microplastics
The mass concentrations of PET microplastics in any environmental sample matrix can be measured using eqn (1).32,33 | 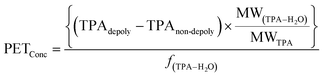 | (1) |
Here, PETConc is the mass concentration of PET microplastics present in ambient air; TPAdepoly is the terephthalic acid (TPA) obtained after depolymerization of airborne PET microplastics; TPAnon-depoly is the free terephthalic acid (actual terephthalic acid present in the ambient fine particulate matter before depolymerization); f(TPA–H2O) and MW(TPA–H2O) represent the mass percentage and molecular weight of (TPA–H2O). MWTPA is the molecular weight of TPA. From the chemical structure of PET polymer, [–C(O)–C6H4–C(O)–O–CH2–CH2–]n, (TPA–H2O) accounts for approximately 77.4% (f(TPA–H2O), % w/w) of PET (Wang et al., 2017).32 TPA is also used as a monomer in the production of polybutylene terephthalate (PBT) and polytrimethylene terephthalate (PTT). Nevertheless, the abundance of these two polymers in ambient particulate matter is relatively much lower compared to the abundance of PET MPs.34 Hence, the contribution of these polymers to the depolymerised fraction of terephthalic acid is insignificant. The reported measurements of PET in this study represent the upper limit for the mass concentrations of the observed PET MPs present in the inhalable fraction of ambient fine particulate matter.
2.6 Quality assurance (QA) and quality control (QC)
No plastic containers were used throughout the study to avoid contamination during the analysis. Calibrations were performed at regular intervals during the analysis time with five different concentrations of TPA ranging from 10 ng mL−1 to 200 ng mL−1. Terephthalic acid standards used in the entire study were prepared in LC-MS-grade methanol. Fig. S3† presents the linearity curve obtained for terephthalic acid. This method had resulted in excellent linearity (r2 > 0.99). The limit of detection (LOD) and limit of quantification (LOQ) of terephthalic acid were determined to be 2.9 ng mL−1 and 9.9 ng mL−1, respectively. The precision error of the method was verified by analyzing the terephthalic acid standard multiple times (n = 5) on the same day. The precision error was observed to be less than 10%, calculated by obtaining the relative standard deviation of the peak area of the same sample that was injected into the LC-MS/MS and analyzed five times. Recovery tests were performed as a part of QA and QC using Teflon and quartz fiber filters, and the details are explained in Section 2.7. The total uncertainty reported in Table 1 was calculated using the root mean square propagation of individual uncertainties, like the 1% accuracy error inherent in the terephthalic acid standard and the instrument precision error determined using the replicates of the samples (n = 5). Further, we extracted and analyzed the blank filters (n = 6) as per the procedure described in Section 2.3. The concentrations of PET MPs from the blank filters are summarized in Table S3.† These concentrations from the blanks were averaged and subtracted from the samples to report the final concentrations.
Table 1 Measured mass concentrations of airborne PET MPs in Delhi and Mohali
Sampling date |
Concentration ± uncertainty (ng m−3) |
Below detection limit.
|
Mohali samples
|
16/10/2022 |
2.8 ± (0.2) |
19/10/2022 |
bdla |
23/10/2022 |
158.0 ± (13.9) |
26/10/2022 |
49.6 ± (4.4) |
31/10/2022 |
bdla |
04/11/2022 |
10.1 ± (0.9) |
05/11/2022 |
13.6 ± (1.2) |
11/11/2022 |
1.9 ± (0.2) |
![[thin space (1/6-em)]](https://www.rsc.org/images/entities/char_2009.gif) |
Delhi samples
|
16/10/2022 |
135.2 ± (11.9) |
22/10/2022 |
52.1 ± (4.6) |
31/10/2022 |
59.8 ± (5.3) |
04/11/2022 |
25.9 ± (2.3) |
2.7 Detection of terephthalic acid from the depolymerization of PET spiked onto blank filters
In this study, we also compared the extraction efficiency of PET MPs with two different extensively used filter substrates for ambient particulate matter collection, namely, Teflon and quartz fiber filters.35 Based on earlier studies reported in the literature using sediment and water samples, we chose the concentrations of 50 ng and 100 ng for recovery testing.28,29,36 To spike the corresponding concentrations of 50 ng and 100 ng of PET microplastics, standard PET pellets were weighed accordingly and dissolved in chloroform. The dissolved PET solution was spiked onto the blank filters and then allowed to dry at room temperature to remove the solvent. Thus, the test concentrations were spiked onto the filters. This test was carried out by spiking two known concentrations (50 ng and 100 ng) of PET onto the blank Teflon and quartz fiber filters. The spiked filters were extracted and analyzed using the procedure described in Sections 2.3 and 2.4. High recovery of PET microplastics was observed with the Teflon spiked filters. The obtained recovery percentages with both quartz fiber filters and Teflon filters are presented in Table S4.† The recovery (80–120%) of PET microplastics using Teflon filters is within the limits set by the U.S. EPA (±20% variability).37 We observed that sonication had destroyed the quartz filter fabric and made it difficult to separate fibres from microplastic particles when filtering the suspensions. Similar observations were reported in earlier studies too.38 Hence, Teflon filters are better suited for the collection and determination of mass concentrations of PET MPs in the inhalable fraction of ambient fine particulate matter.
3 Results and discussion
3.1 Concentrations of polyethylene terephthalate microplastics in the ambient aerosols of Delhi and Mohali
Using the developed and optimised method, a pilot study was performed to screen and measure the mass concentrations of the airborne inhalable fraction of PET MPs samples collected from Delhi and Mohali (refer to Fig. S1†). Considering the logistics, ambient aerosol samples were collected using Teflon filters in October and November 2022 for a few days (refer to Table 1).
All the collected ambient PM2.5 samples were extracted, and mass concentrations of PET MPs were determined as described in Sections 2.3 to 2.5. Fig. 1a–c shows the extracted ion chromatograms of the terephthalic acid standard, free terephthalic acid (TPA) and the depolymerised fraction of terephthalic acid present in the ambient PM2.5 sample collected in Delhi on 31/10/2022 02:00 PM to 01/11/2022 02:00 PM (IST), respectively. The presence of terephthalic acid in the fraction of depolymerized extracts of ambient PM2.5 samples confirms the presence of PET microplastics in the inhalable fraction of ambient particulate matter in Mohali and Delhi. Table 1 shows the mass concentrations of PET MPs in the inhalable fraction of ambient particulate matter from Mohali and Delhi. In Mohali, about 75% of collected samples contained PET MPs in the inhalable fraction of ambient particulate matter. The maximum mass concentration observed in Mohali was about 158.0 ng m−3 on October 23, 2022, which happened to be one of the festive days in India known as Choti Diwali (a day before Diwali), where anthropogenic activity such as burning garbage consisting of household plastic items is known to be very high.39,40 Many households in this region are known to dispose of old household items in the garbage, which are eventually burnt.41,42 During this sampling day, we also observed the enhanced concentration of carbon monoxide (a chemical tracer for inefficient combustion), up to 3.03 ppm. This indicates the co-emission of PET along with inefficient combustion tracers like CO. Hence, pre-Diwali activity could be a plausible reason for the observed peak concentrations. Due to some operational logistic issues, we were not able to carry out the measurements on Diwali day. In Delhi, PET MPs were observed in all the collected ambient PM2.5 samples. Mass concentrations of PET MPs present in the inhalable fraction of ambient PM2.5 samples collected in Delhi ranged from 25.9 to 135.2 ng m−3.
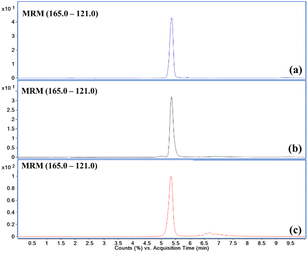 |
| Fig. 1 Extracted ion chromatograms of (a) terephthalic acid standard; (b) free terephthalic acid in the aerosol sample from Delhi collected on 31/10/2022 02:00 PM to 01/11/2022 02:00 PM (IST); (c) depolymerised fraction of terephthalic acid from the inhalable fraction extract of ambient PM2.5 sample collected from Delhi on 31/10/2022 02:00 PM to 01/11/2022 02:00 PM (IST). | |
Recent in vitro studies have shown that exposure to PET MPs can cause pulmonary disorders if the mass concentrations of PET exceed more than 98.6 μg mL−1.21 Hence, it is important to carry out the measurements of mass concentrations of PET MPs in the inhalable fraction of ambient PM2.5 to understand the various additional health risks associated with it. To the best of our knowledge, this is the first-ever study to report the mass concentrations of PET MPs in ambient PM2.5 using LC-MS/MS.
To date, there is no air quality standard for the PET microplastics present in ambient PM2.5. Our study demonstrated the presence of the inhalable fraction of PET MPs in ambient air and developed an optimised method for the determination of mass concentrations of PET MPs using the LC-MS/MS method. This method can be extended to determine the mass concentrations of other types of MPs present in ambient particulate matter. Further long-term regional studies are needed to understand the sources, seasonal variability, and health effects of inhalation exposure to PET MPs.
4 Conclusions
In this study, mass quantification of PET microplastics present in the inhalable fraction of particulate matter was achieved using LC-MS/MS. This would help in understanding the various additional health risks associated with inhalation exposure to the mass concentration of microplastics. Further, after validation of the developed method, a pilot study was conducted to determine the presence of microplastics in the airborne particulate matter, which is also the first-ever study to report the mass concentrations of PET MPs in ambient PM2.5 in New Delhi and Mohali in India using LC-MS/MS.
Data availability
The data supporting this article have been included as part of the ESI.†
Conflicts of interest
There are no conflicts to declare.
Acknowledgements
The authors are grateful and dedicate this work to Bhagawan Sri Sathya Sai Baba, the Founder Chancellor, Sri Sathya Sai Institute of Higher Learning. The authors are thankful to the administration of Sri Sathya Sai Institute of Higher Learning – Central Research Instrument Facility for providing the state-of-the-art instrumentation facility to carry out this scientific study. We thank the IISER Mohali Central Atmospheric Chemistry Facility for the supporting data and the RASAGAM project team of MOES, India, for logistical support.
References
- J. P. G. L. Frias and R. Nash, Microplastics: Finding a consensus on the definition, Mar. Pollut. Bull., 2019, 138, 145–147 CrossRef CAS PubMed.
- Y. Yao, M. Glamoclija, A. Murphy and Y. Gao, Characterization of microplastics in indoor and ambient air in northern New Jersey, Environ. Res., 2022, 207, 112142 CrossRef CAS PubMed.
- S. Dehghani, F. Moore and R. Akhbarizadeh, Microplastic pollution in deposited urban dust, Tehran metropolis, Iran, Environ. Sci. Pollut. Res., 2017, 24, 20360–20371 CrossRef CAS PubMed.
- J. C. Prata, Airborne microplastics: Consequences to human health?, Environ. Pollut., 2018, 234, 115–126 CrossRef CAS PubMed.
- L. E. Revell, P. Kuma, E. C. Le Ru, W. R. C. Somerville and S. Gaw, Direct radiative effects of airborne microplastics, Nature, 2021, 598, 462–467 CrossRef CAS PubMed.
- J. Gasperi, S. L. Wright, R. Dris, F. Collard, C. Mandin and M. Guerrouache,
et al., Microplastics in air: Are we breathing it in?, Curr. Opin. Environ. Sci. Health, 2018, 1, 1–5 CrossRef.
- A. P. Abad López, J. Trilleras, V. A. Arana, L. S. Garcia-Alzate and C. D. Grande-Tovar, Atmospheric microplastics: exposure, toxicity, and detrimental health effects, RSC Adv., 2023, 13, 7468–7489 RSC.
- N. Parashar and S. Hait, Plastic rain—Atmospheric microplastics deposition in urban and peri-urban areas of Patna City, Bihar, India: Distribution, characteristics, transport, and source analysis, J. Hazard. Mater., 2023, 458, 131883 CrossRef CAS PubMed.
- R. Akhbarizadeh, S. Dobaradaran, M. Amouei Torkmahalleh, R. Saeedi, R. Aibaghi and F. Faraji Ghasemi, Suspended fine particulate matter (PM2.5), microplastics (MPs), and polycyclic aromatic hydrocarbons (PAHs) in air: Their possible relationships and health implications, Environ. Res., 2021, 192, 110339 CrossRef CAS PubMed.
- A. A. Koelmans, E. Besseling, A. Wegner and E. M. Foekema, Plastic as a carrier of POPs to aquatic organisms: A model analysis, Environ. Sci. Technol., 2013, 47, 7812–7820 CrossRef CAS PubMed.
- J. Reisser, J. Shaw, G. Hallegraeff, M. Proietti, D. K. A. Barnes and M. Thums,
et al., Millimeter-sized marine plastics: A new pelagic habitat for microorganisms and invertebrates, PLoS One, 2014, 9, e100289, DOI:10.1371/journal.pone.0100289.
- Y. Li, Z. Lu, D. P. Abrahamsson, W. Song, C. Yang and Q. Huang,
et al., Non-targeted analysis for organic components of microplastic leachates, Sci. Total Environ., 2022, 816, 151598 CrossRef CAS PubMed.
- N. Parashar and S. Hait, Plastic rain—Atmospheric microplastics deposition in urban and peri-urban areas of Patna City, Bihar, India: Distribution, characteristics, transport, and source analysis, J. Hazard. Mater., 2023, 458, 131883 CrossRef CAS PubMed.
- K. Liu, X. Wang, N. Wei, Z. Song and D. Li, Accurate quantification and transport estimation of suspended atmospheric microplastics in megacities: Implications for human health, Environ. Int., 2019, 132, 105127 CrossRef CAS PubMed.
- P. G. Gunasekar and L. W. Stanek, Advances in exposure and toxicity assessment of particulate matter: An overview of presentations at the 2009 Toxicology and Risk Assessment Conference, Toxicol. Appl. Pharmacol., 2011, 254, 141–144 CrossRef CAS PubMed.
- J. S. Brown, T. Gordon, O. Price and B. Asgharian, Thoracic and respirable particle definitions for human health risk assessment, Part. Fibre Toxicol., 2013, 10(1), 1–12 CrossRef PubMed.
- Y. Ichikawa, T. Watanabe, Y. Horimoto, K. Ishii and S. Naito, Measurements of 50 non-polar organic compounds including polycyclic aromatic hydrocarbons, n-alkanes and phthalate esters in fine particulate matter (PM2.5) in an industrial area of Chiba Prefecture, Japan, Asian J. Atmos. Environ., 2018, 12, 274–288 CrossRef CAS.
- Z. Li, S. Guo, Y. Wang, Y. Hu and Y. Xing,
et al., PM2.5 associated phenols, phthalates, and water soluble ions from five stationary combustion sources, Aerosol Air Qual. Res., 2020, 20, 61–71 CrossRef CAS.
- J. Cortez Pimentel, R. Avila and A. Galvao Lourenco, Respiratory disease caused by synthetic fibres: a new occupational disease, Thorax, 1975, 30, 204–219 CrossRef PubMed.
-
I. S. B. Eckett, Occupational Respiratory Diseases, 2000 Search PubMed.
- H. Zhang, S. Zhang, Z. Duan and L. Wang, Pulmonary toxicology assessment of polyethylene terephthalate nanoplastic particles in vitro, Environ. Int., 2022, 162, 107177 CrossRef CAS PubMed.
- A. P. Lykov, O. V. Poveshchenko, M. A. Surovtseva, N. A. Bondarenko, I. I. Kim and A. A. Karpenko,
et al., Effect of Polyethylene Terephthalate on Functional Properties of Endothelial and Mesenchymal Cells, Bull. Exp. Biol. Med., 2019, 166, 580–585 CrossRef CAS PubMed.
- N. Bashirova, D. Poppitz, N. Klüver, S. Scholz, J. Matysik and A. Alia, A mechanistic understanding of the effects of polyethylene terephthalate nanoplastics in the zebrafish (Danio rerio) embryo, Sci. Rep., 2023, 13, 1891, DOI:10.1038/s41598-023-28712-y.
- S. Kauts, Y. Mishra, S. Yousuf, R. Bhardwaj, S. K. Singh and F. M. Alshabrmi,
et al., Toxicological Profile of Polyethylene Terephthalate (PET) Microplastic in Ingested Drosophila melanogaster (Oregon R+) and Its Adverse Effect on Behavior and Development, Toxics, 2023, 11, 782, DOI:10.3390/toxics11090782.
- C. Schwaferts, R. Niessner, M. Elsner and N. P. Ivleva, Methods for the analysis of submicrometer- and nanoplastic particles in the environment, TrAC, Trends Anal. Chem., 2019, 112, 52–65 CrossRef CAS.
- T. Rocha-Santos and A. C. Duarte, A critical overview of the analytical approaches to the occurrence, the fate and the behavior of microplastics in the environment, TrAC, Trends Anal. Chem., 2015, 65, 47–53 CrossRef CAS.
- X. Zhu, W. Huang, M. Fang, Z. Liao, Y. Wang and L. Xu,
et al., Airborne Microplastic Concentrations in Five Megacities of Northern and Southeast China, Environ. Sci. Technol., 2021, 55, 12871–12881 CAS.
- E. Duemichen, P. Eisentraut, M. Celina and U. Braun, Automated thermal extraction-desorption gas chromatography mass spectrometry: A multifunctional tool for comprehensive characterization of polymers and their degradation products, J. Chromatogr. A, 2019, 1592, 133–142 CrossRef CAS PubMed.
- A. Ter Halle, L. Jeanneau, M. Martignac, E. Jardé, B. Pedrono and L. Brach,
et al., Nanoplastic in the North Atlantic Subtropical Gyre, Environ. Sci. Technol., 2017, 51, 13689–13697 CrossRef CAS PubMed.
- M. Velimirovic, K. Tirez, S. Verstraelen, E. Frijns, S. Remy and G. Koppen,
et al., Mass spectrometry as a powerful analytical tool for the characterization of indoor airborne microplastics and nanoplastics, J. Anal. At. Spectrom., 2021, 36, 695–705 RSC.
- D. P. Patnana, B. P. Chandra, P. Chaudhary, B. Sinha and V. Sinha, Optimized LC-MS/MS method for simultaneous determination of endocrine disruptors and PAHs bound to PM2.5: Sources and health risk in Indo-Gangetic Plain, Atmos. Environ., 2022, 290, 119363 CrossRef CAS.
- L. Wang, J. Zhang, S. Hou and H. Sun, A Simple Method for Quantifying Polycarbonate and Polyethylene Terephthalate Microplastics in Environmental Samples by Liquid Chromatography-Tandem Mass Spectrometry, Environ. Sci. Technol. Lett., 2017, 4, 530–534 CrossRef CAS.
- J. Zhang, L. Wang and K. Kannan, Polyethylene Terephthalate and Polycarbonate Microplastics in Pet Food and Feces from the United States, Environ. Sci. Technol., 2019, 53, 12035–12042 CrossRef CAS PubMed.
- D. Kau, D. Materić, R. Holzinger, K. Baumann-Stanzer, G. Schauer and A. Kasper-Giebl, Fine micro-and nanoplastics concentrations in particulate matter samples from the high alpine site Sonnblick, Austria, Chemosphere, 2024, 352, 141410 CrossRef CAS PubMed.
- C. Perrino, S. Canepari and M. Catrambone, Comparing the performance of Teflon and quartz membrane filters collecting atmospheric PM: Influence of atmospheric water, Aerosol Air Qual. Res., 2013, 13, 137–147 CrossRef CAS.
- L. Tian, E. Skoczynska, D. Siddhanti, R. J. van Putten, H. A. Leslie and G. J. M. Gruter, Quantification of polyethylene terephthalate microplastics and nanoplastics in sands, indoor dust and sludge using a simplified in-matrix depolymerization method, Mar. Pollut. Bull., 2022, 175, 113403 CrossRef CAS PubMed.
-
US EPA, National Air Toxics Trends Stations Program, 2016 Search PubMed.
- A. Yang, A. Jedynska, B. Hellack, I. Kooter, G. Hoek and B. Brunekreef,
et al., Measurement of the oxidative potential of PM2.5 and its constituents: The effect of extraction solvent and filter type, Atmos. Environ., 2014, 83, 35–42 CrossRef CAS.
- S. Sarkar, P. S. Khillare, D. S. Jyethi, A. Hasan and M. Parween, Chemical speciation of respirable suspended particulate matter during a major firework festival in India, J. Hazard. Mater., 2010, 184, 321–330 CrossRef CAS PubMed.
- D. P. Singh, R. Gadi, T. K. Mandal, C. K. Dixit, K. Singh and T. Saud,
et al., Study of temporal variation in ambient air quality during Diwali festival in India, Environ. Monit. Assess., 2010, 169, 1–13 CrossRef CAS PubMed.
- G. Sharma, B. Sinha, Pallavi, H. Hakkim, B. P. Chandra and A. Kumar,
et al, Gridded Emissions of CO, NOx, SO2, CO2, NH3, HCl, CH4, PM2.5, PM10, BC, and NMVOC from Open Municipal Waste Burning in India, Environ. Sci. Technol., 2019, 53, 4765–4774 CrossRef CAS PubMed.
- S. Garg, B. P. Chandra, V. Sinha, R. Sarda-Esteve, V. Gros and B. Sinha, Limitation of the Use of the Absorption Angstrom Exponent for Source Apportionment of Equivalent Black Carbon: A Case Study from the North West Indo-Gangetic Plain, Environ. Sci. Technol., 2016, 50, 814–824 CrossRef CAS PubMed.
|
This journal is © The Royal Society of Chemistry 2024 |
Click here to see how this site uses Cookies. View our privacy policy here.