More than just sweet: current insights into microplastics in honey products and a case study of Melipona quadrifasciata honey†
Received
9th May 2024
, Accepted 14th July 2024
First published on 29th July 2024
Abstract
Honey, traditionally known as a pure and natural substance, has become an unexpected reservoir for microplastic contamination. This study consisted of an experimental investigation to assess the occurrence of microplastics in honey produced by Melipona quadrifasciata, a native bee species in Brazil. Our investigation covers eight areas (one sample per area), including built and vegetated areas located in São Paulo city, Brazil, to understand the distribution of microplastics in these environments. Honey samples (10 mL) were collected using a syringe and sent to the laboratory for further analysis. Microplastics extracted from honey samples were characterized under a stereomicroscope to determine their size, color, and morphology. Also, the polymer type was determined by FTIR analysis. All honey samples (100%) showed microplastics. The predominant particles displayed a fiber shape with a size below 299 μm and a transparent color and were primarily composed of polypropylene. Their concentrations ranged from 0.1 to 2.6 particles per mL of honey, raising concerns about their potential impact on bee populations and human consumers. This study underscores the need for further research on the sources and implications of microplastic contamination in Melipona quadrifasciata honey, shedding light on the broader issue of environmental plastic pollution and its impact on pollinators.
Environmental significance
Studying the occurrence of microplastics in honey samples from urbanized areas is crucial for comprehending the extent and implications of plastic pollution in densely populated regions. Urban centers are hubs of plastic consumption and disposal; investigating the presence of microplastics in honey serves as a biomonitoring tool, allowing the identification of potential sources and pathways of microplastic pollution within urban settings. Furthermore, honey samples provide a unique perspective on the transfer of plastics along the food chain. Bees play a vital role in ecosystem functioning and food production, making them susceptible to environmental stressors such as pollution. The presence of microplastics in honey raises concerns about their potential impacts on bee health and colony dynamics, with implications for ecosystem stability and biodiversity.
|
1. Introduction
Pollination by animals, particularly bees, is an essential ecosystem service.1–3 In natural ecosystems, bees contribute directly to the conservation of plants through angiosperm reproduction, ensuring the production of fruits and seeds.4,5 By their foraging activities, bees facilitate gene flow and enhance the genetic diversity of plants, ensuring the persistence of these species in natural environments.6–8 This intricate web of interactions extends beyond flora, influencing fauna populations dependent on plants for sustenance. Additionally, in agricultural areas, bees increase the productivity and quality of fruits of many crops dependent on pollination, increasing their commercial values.9–11 Therefore, this ecological role played by bees reinforces the importance of this group for maintaining ecosystems, making them instrumental in sustaining both plant and animal life.1,2,5
In addition to pollination, another notable aspect of bee ecology is honey production, a process integral to the life cycle of some species, including the commercially better-known honeybee, Apis mellifera, and species of stingless bees.12 Bees collect nectar from flowers, which undergoes enzymatic transformation within the hive, resulting in the production of honey.13,14 This substance serves as a primary source for the colony, providing the energy necessary for adult bees to perform essential activities, such as flight, and fostering the development of larvae through both honey and pollen.15 Honey stored in the colony also acts as a reserve for periods when resource collection is challenging or when food sources are scarce.16 In addition to being fundamental for the development and survival of the colony, honey is also consumed by humans and is recognized for its several important medicinal properties and health benefits.5 This substance is used in the treatment of wounds and gastric, hepatic, and pancreatic diseases, in addition to promoting cardiovascular health.17,18 Therefore, honey production represents a symbiotic relationship between bees and humans, with ecological implications extending to agriculture through enhanced pollination services.
In large cities, the high population density and concentration of human activities generate an intense demand for energy, transport, and inputs, causing a significant emission of pollutants.19 In addition to traditionally reported pollutants, microplastics have also been detected in bodies of water, soil, and even the atmosphere of urban areas.20,21 The breeding of stingless bees in cities has become an increasingly common practice, due to the recognition of the importance of these bees and the commercialization of their products.22 Despite being a sustainable practice, this proximity of bees to large cities leads to questions about the food safety of their products, such as honey, and threats to the health of bees due to possible contamination.23
Despite their ecological importance and the benefits of beekeeping items, bees and their products, such as honey, wax, and propolis, are susceptible to the adverse effects of anthropogenic pollutants.2,24,25 In recent decades, several bee species, like Megachile rotundata, Melipona quadrifasciata, Apis mellifera and others, have faced vulnerability due to the presence of heavy metals, pesticides, herbicides, and fungicides.26–29 These chemical products have been responsible for a significant reduction in bee populations, posing a considerable threat to these organisms.9,30 Now, concerns have been raised regarding the interaction between microplastics and bees, a consequence of widespread plastic pollution.31 Microplastics, defined as particles with a size less than 5 mm, encompass a diverse array of synthetic polymers.32 While collecting nectar and pollen bees can inadvertently consume multiple pollutants,33 including microplastics. Regarding honeybees, some studies have reported microplastics in honey worldwide.34–37 Different concentrations were found in honey samples from A. mellifera, ranging from 54 particles per L to 336 particles per kg.34,36,37 However, the studies have neglected the microplastic contamination of native bee species so far.
The present study explored the existing literature on this subject, shedding light on the current state of research. The interplay between bees, microplastics, and honey underscores the need for comprehensive research to elucidate the extent of this issue and its potential long-term consequences. Moreover, we conducted an experimental investigation on the presence of microplastics in honey produced by Melipona quadrifasciata, a native species of the Atlantic Rainforest, in Brazil. This study involved a comparative analysis of hives located in an urban matrix with different levels of urbanization. The examination aimed to discern the distribution and characteristics of microplastics within the honey produced by this native bee species. In addition, we compared hives from built and vegetated areas for an assessment of the potential influence of the surrounding environment on the presence and concentration of microplastics in the honey produced by these bees. Addressing the issue of the contamination of honey is essential to preserving the health of pollinator populations and, consequently, the ecological balance they sustain.
2. Presence and impact of microplastics in bee environments
2.1. Bee species
Estimates suggest that there are over 20
000 bee species worldwide.38 Despite this considerable number, bee diversity has been on the decline due to climate change and anthropogenic impacts.2 While there has been a notable increase in microplastic research in recent years, insects, particularly bees, remain relatively understudied. Few investigations have focused on bees thus far, with A. mellifera being the most extensively researched species to date.21,31,39–43 Beyond A. mellifera, other species explored in the field and/or laboratory include Apis cerana,42Heterotrigona itama,44 and Partamona helleri,45 the latter being a Brazilian stingless species.
We investigated the presence of microplastics in honey from a species native to Brazil. Bees from the genus Melipona, such as Melipona quadrifasciata “mandaçaia”, are widely managed for honey production; in addition, they can be used as environmental quality bioindicators in urban areas, through the analysis of contaminants present, both in their bodies and their honey.46,47 Therefore, Melipona species are excellent models for evaluating potential contamination by microplastics in urban areas.
2.2. Impacts of microplastics on bees
Studies have revealed that the implications of microplastic ingestion by bees extend beyond the immediate physiological effects. Experiments conducted under artificial conditions, where bees were exposed to foodborne microplastics, revealed that contamination occurs across various components of the hive,31 including honey. This process occurs through both ingestion and the adherence of particles to the bees' bodies. Also, these studies reveal that the biological outcomes differ based on the particular polymer to which organisms are exposed. Each plastic is characterized by a distinct polymeric structure, complemented by specific additives tailored for diverse applications. As a result, the toxicity of microplastics is dependent on their composition. Additionally, distinct species demonstrate diverse biological responses based on the specific microplastics to which they are exposed.48,49 Hence, it becomes imperative to investigate the impact of each polymer on bees for a comprehensive understanding of their effects.
Polystyrene (PS) underwent testing in two instances, yielding different outcomes. Deng et al.42 found that it induced mortality in A. mellifera and A. cerana. However, despite impacting the microbiota, gene expression related to immune response, and biochemical changes, it did not reduce the survival of A. mellifera, as observed in the study of Wang et al.21. Al Naggar et al.,39 Alma et al.,31 Balzani et al.,40 Buteler et al.,41 Deng et al.42 Pasquini et al.,43 and Wang et al.21 also did not observe mortality due to exposure to PS; however, they noted changes in feeding behavior. Regarding polyester microfibers, it was found that this polymer did not induce acute mortality in honeybees41 and did not affect the population growth of the species evaluated.31 Pasquini et al.43 investigated the effects of PS and polymethyl methacrylate (PMMA), both in isolated and combined treatments. The results suggested that both polymers could reach the bee's brain after ingestion, influencing learning and memory capabilities. Notably, PS induced more significant impacts than PMMA on A. mellifera.43 On the other hand, polyethylene (PE) exposure led to reduced survival and altered feeding behavior, without impairing associative and non-associative learning or memory.40 Additionally, the impact of PS and polyethylene terephthalate (PET) on the stingless bee Partamona helleri was explored.45 In this case, the survival rate remained unaffected, but individuals exposed to microplastics exhibited altered behavior, increased body weight compared to the control, and changes in the hemocyte count.
2.3. Microplastics in bee-origin products
Bees inadvertently collect nectar and pollen from environments increasingly laden with microplastics.50 These particles can be introduced into colonies and can subsequently find their way into the honey through the foraging, processing, and storage activities of the bees.
Among the types of bee products susceptible to microplastic contamination are honey, beeswax, and propolis. However, only honey has undergone investigation under natural conditions thus far. In all studies available in the literature, the investigated samples showed the presence of synthetic particles. The concentrations of microplastics in honey ranged from as low as 2 fragments per kg of honey to 954 fragments and fibers per L.34,36
Wax samples were investigated under controlled laboratory conditions, revealing that microplastics tend to accumulate in the wax, likely due to the viscosity of this material, thereby limiting their transfer to honey.31 This may suggest that under natural conditions, the concentration of microplastics in wax is likely higher than that found in honey. Further studies with these samples should be encouraged to clarify this matter.
The lack of comprehensive studies has resulted in inconsistent findings. For instance, Mühlschlegel et al.77 detected microplastics in honey; nevertheless, they asserted that the outcomes did not offer substantial evidence of contamination. In contrast, Liebezeit and Liebezeit36,37 highlighted a contrasting perspective, considering the number of particles found to be significant. The study of anthropogenic pollutants in honey is crucial not only for understanding the direct threats to bee populations but also for evaluating the potential implications for human consumers. As honey represents a direct link between the environment and human food supply, comprehending the dynamics of pollutant transfer from bees to honey under realistic conditions underscores the interconnectedness of both ecological and public health.31
3. Materials and methods
3.1. Study area
The study was conducted in São Paulo city, a highly industrialized metropolis situated in southeastern Brazil (23°32′56′′S, 46°38′20′′W). This urban center is marked by significant socio-economic diversity and dense demographic concentrations, with a population exceeding 17 million people in the metropolitan area.51 The intense industrial activity, transportation networks, varied land use patterns, and high demographic concentrations have consequential implications, notably a heightened pollution index, leading to environmental challenges.52,53 Furthermore, this scenario places vegetation areas and fauna at risk. Therefore, São Paulo presents an excellent model for the study of urban environments for investigations seeking to unravel the complex dynamics between these systems and the resulting environmental implications. Eight collection stations were chosen, each one containing an M. quadrifasciata hive, comprising built or “grey areas”, lacking vegetation cover, and areas characterized by extensive vegetation cover (Fig. 1).
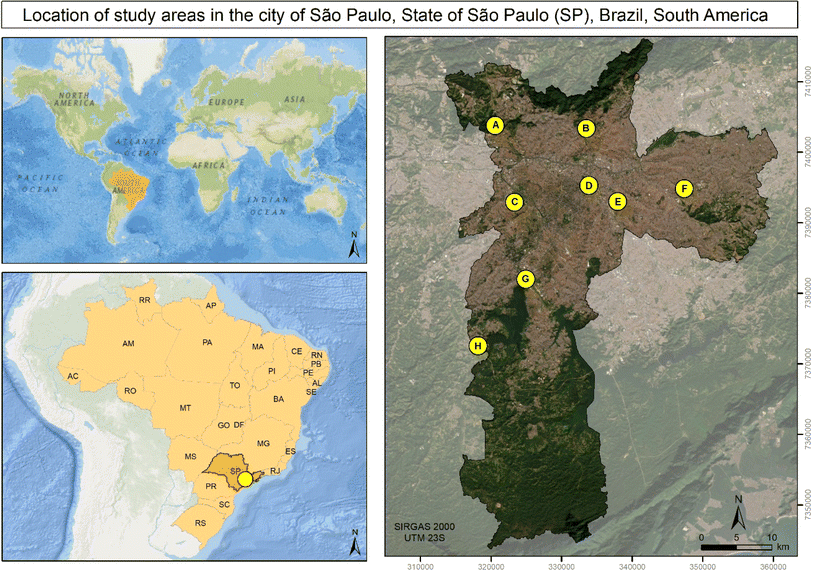 |
| Fig. 1 Study areas in the city of São Paulo, in Brazil. Letters highlighted (A–H) in yellow indicate the sampling stations. Boundaries: IBGE, 2021; aerial images: Google, 2023. | |
3.2. Bee species and sample collection
Melipona quadrifasciata, commonly known as the Mandaçaia bee, is a stingless bee (tribe Meliponini) native to Brazil.54 This species is characterized by continuous yellow stripes,55 reaching from 9 to 10 millimeters in length56 (Fig. 2a). These bees are commonly found in various habitats, ranging from tropical rainforests to urban areas. They are particularly adaptable and have been observed nesting in tree hollows, termite mounds, and even man-made structures like walls and attics.57 The Mandaçaia bee produces honey that is highly valued because of its unique flavor and medicinal properties.12
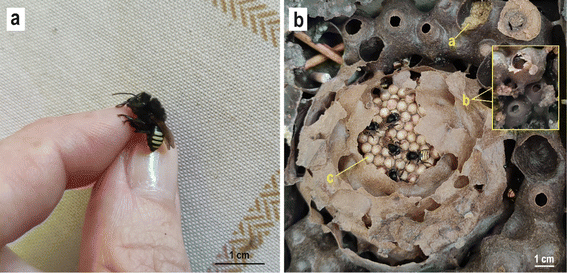 |
| Fig. 2 (a) Worker of Melipona quadrifasciata. (b) Internal view of an M. quadrifasciata hive, showing the food storage on pots ((a): pollen and (b): honey) around the brood area with the combs of sealed brood cells (c) and some workers. | |
Collections were carried out during the summer of 2023. To ensure that the honey produced by bees only presents the characteristics of locally collected resources, artificial food supplementation was suspended three months before the start of the experiment. In each sampling station (ESI, Table S1†), 10 mL of honey were collected from the honey pots using a syringe (Fig. 2b). The honey samples were quickly collected to avoid stress in the beehive and microplastic contamination from the air.
3.3. Extraction of microplastics
For the extraction of microplastics from honey, we used 10 mL of the sample. The samples were subsequently transferred to previously sterilized glass graduated cylinders, where they underwent dilution in filtered (Whatman, 47 mm, 0.7 μm pore/QUIMIS, model 355 B.2) ultrapure water (20 mL) and were then subjected to filtration using a vacuum filtration system with fiberglass filters (Whatman, 47 mm, 0.7 μm pore). The filters were rinsed with an additional 20 mL of water. The filters were stored in glass Petri dishes, previously washed with acetone. The final concentration of the microplastic was given in particles per mL of honey. Due to the composition of honey, which includes sugars, water, and minerals,58 it was unnecessary to proceed with the usual steps of organic matter digestion and/or particle separation by density. The use of raw samples with only dilution reduces processing time and minimizes the introduction of additional solutions, thereby reducing the potential for particle loss during sample transfers between containers and the risk of cross-contamination.
3.4. Microplastic characterization
The examination and identification of extracted microplastics were conducted through visual inspection using a Zeiss Discovery V12 stereomicroscope with a magnification range of 40–100×. The entire filter surface area was carefully examined to locate microplastics, allowing for their quantification and detailed physical characterization, including size, color, and morphology. To ensure precise particle identification and minimize potential human errors in the process, our analysis was specifically limited to particles with a diameter equal to or greater than 50 μm.
Microplastics were categorized based on the following morphological classifications: fiber and filament; film; fragment; beads and spheres; foam. Particle color was divided into five categories: colorless, blue, black, red, and others. The colorless category included grayish particles. Regarding size, microplastics were classified according to five size classes: Class 1 (50–299 μm); Class 2 (300–499 μm); Class 3 (500–699 μm); Class 4 (700–999 μm); Class 5 (≥999 μm).
Random particles were selected for polymer composition identification using micro-Raman spectroscopy. The Raman spectra were recorded using two Raman confocal spectrometers, WITec Alpha 300R and Renishaw inVia-Reflex. The Raman spectra were recorded using 633 and 785 nm excitation lasers and a 10 s exposure time in a range of 600–1650 cm−1.
3.5. Quality control and quality assurance (QA/QC)
The sampling was conducted following some measures to avoid cross-contamination. The researchers used cotton clothes and quickly handled the beehive to avoid microplastic contamination from the air. Shortly thereafter, the samples were immediately placed into cleaned containers and stored until processing under controlled laboratory conditions.
During the experimental procedures, we adhered to a consistent sample-handling protocol. We only use stainless steel and glass tools and containers, which have been rinsed with ultrapure water at least three times each before usage. Subsequently, the materials were cleaned using cotton infused with 90% acetone to eliminate any potential microplastic contaminants and covered with aluminum foil. To mitigate environmental contamination, the water underwent triple filtration through 0.7 μm fiberglass filters (47 mm) before use. All steps of sample preparation and manipulation were executed within a sterile fume hood, maintaining continuous laminar flow. Furthermore, we wear cotton lab coats throughout the experiment. Following the filtration process, filters were placed in Petri dishes. During stereomicroscope analyses, filters were examined within covered Petri dishes, reducing the likelihood of atmospheric deposition of microplastics. This set of measures was employed to ensure the integrity and precision of our experimental outcomes. Despite implementing these rigorous precautions, we further conducted blank/control samples (n = 3) to document the average particle count resulting from potential cross-contamination, be it from atmospheric or water sources. The blank samples comprised filtered ultrapure water in a volume equivalent to that of the honey utilized. These blank samples underwent the same processing stages as the treatment samples. The samples' particle count was adjusted by subtracting the average number of particles identified in the blank samples (ESI, Table S2†).
3.6. Study area classification
The study area surrounding each sampling station was classified as “built areas”, “vegetated areas”, and water. The classification was performed with Sentinel 2A satellite images, using as a temporal filter spanning 1 year (08/30/21 to 22). A reducer was applied to the median of this collection of images. Although the studied species has a maximum flight range of more than two kilometers,59 individuals rarely travel long distances from the nest, preferring forage closer options.60 Therefore, considering the current forage trip that these bees undertake, our analysis considered a radius of 900 meters from the nest of origin as a reference. 22 Having the bee's hives as a central point, 900 m radius buffers were generated to demarcate the coverage regions around each study area. The coverage regions were used as a mask to extract the clippings from the satellite images.
Based on this spatial outline, filters were created based on the Normalized Difference Vegetation Index – NDVI61 and Enhanced Vegetation Index – EVI (MODIS, 2024) to obtain specific responses regarding the presence of vegetation cover. Both indices are used to quantify vegetation greenness, but the NDVI is used to evaluate vegetation density and plant health, while the EVI applies some corrections for atmospheric conditions and canopy background noise, being more sensitive to areas with dense vegetation.62 Supervised classification was used, in which the researchers provide training samples of the spectrally significant classes, that is, manually indicating some pixels that belong to each land classification so that the algorithm can classify the whole image.63 The automatic classification of land uses was performed using the Google Earth Engine (GEE) platform using the “random forest” classifier, and the training was performed 1000 times to verify the best classification. Finally, to confirm the accuracy of the classification, confusion matrices were generated, and we obtained a general accuracy of 0.998.
3.7. Statistical analysis
The quantification of microplastics in honey samples was expressed as the number of microplastics per mL of honey (particles per mL). To assess potential variations in the quantity and characteristics of microplastics between distinct sampling stations (built and vegetated), the Kruskal–Wallis test was employed, given that the data failed to meet the required parametric assumptions. In cases where significant differences were identified, the post hoc paired comparison Dunn test64 was executed to elucidate the specific sources of variation. The data related to the number of particles and sampling station were subjected to a correlation analysis and a Bray–Curtis similarity cluster analysis of the study areas regarding their landscape composition was done for data exploration and description. All statistical analyses were conducted using R software65 with the significance level set at p < 0.05. General linear models were also generated to find correlations between the microplastic quantitative parameters (size and number of particles) and the area (ha) of each land classification (built, vegetated, and water). Analyses were conducted using Jamovi.66
4. Results and discussion
4.1. Microplastics in honey
The blank experiment (n = 3) resulted in an average of 0.8 ± 0.1 particles per mL. This value was subtracted from the total particle count in each sample to obtain the final concentration of microplastics per sample. In total, microplastics were found in 100% of the 8 honey samples examined. 180 microplastics were retrieved from all samples, with an average of 1.45 particles per mL of honey (Fig. 3). Representative images of microplastics found in the samples are shown in Fig. S1.†
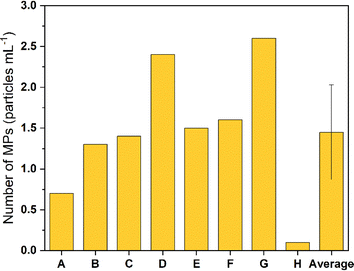 |
| Fig. 3 Amount of microplastics found in honey samples (n = 8) of M. quadrifasciata. The values refer to particles per mL, subtracting the blank result (0.8 particles per mL). | |
A study conducted in Ecuador in 2020 (ref. 34) reported that honey samples showed 54 and 67 particles per L when industrially or handcrafted packaged, respectively. The methodology employed by the authors for microplastic extraction in this study differed from the approach used in our present investigation, which involved the application of high temperatures. The use of elevated temperatures, coupled with the reported presence of wax in the samples, may have contributed to the observed lower concentration when compared to the results obtained in our study. Liebezeit and Liebezeit36 found 2 to 336 particles per kg in 47 analyzed honey samples in Germany. The methodology employed in their study was similar to that used in our article and the results were consistent with the data we recorded. In both studies, the authors used commercial honey, which is supposedly produced by honeybees (Apis mellifera).
As plastic debris is extremely diverse in terms of its physical composition, it is expected that the presence of microplastics with different shapes, colors, and sizes will also be found in environmental samples. Among the shapes in which microplastics can be found, we encountered mostly fibers and fragments, comprising 59.4% and 40%, respectively. According to the chosen size classes for particle classification, 63.3% belonged to Class 1, with a size range between 50 and 299 μm. Regarding color, 82.2% of all recovered microplastics were colorless (Fig. 4).
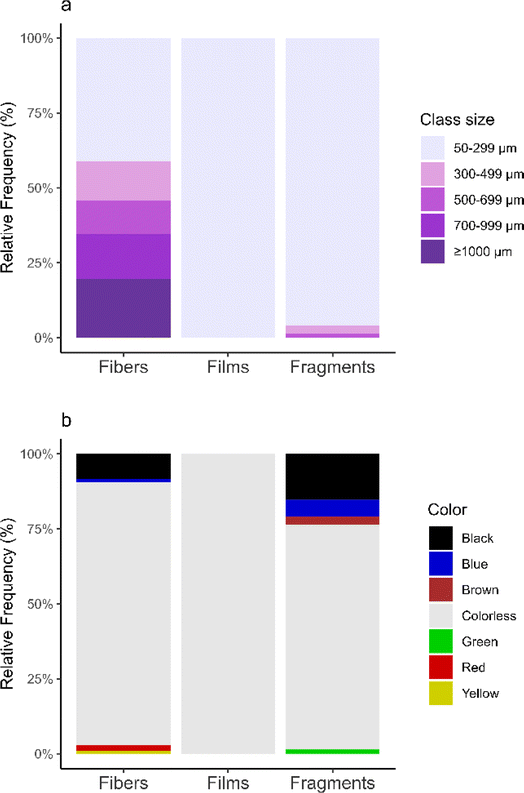 |
| Fig. 4 Types of microplastics found in the honey of M. quadrifasciata according to morphology and split by (a) size and (b) color. | |
Microplastics originate from the physical, chemical, or biological fragmentation processes of large plastic pieces.67 The factors potentially involved in these mechanisms exert their influence continuously and to varying degrees. Therefore, the observed distribution across size classes reflects the tendency for microplastics to constantly reduce their size. The identified predominance of microplastic forms in the studied samples, mainly constituted by fibers, may be attributed to diverse sources and mechanisms. Fibers, commonly sourced from textiles, may be indicative of the widespread release of synthetic fibers during physical abrasion and laundering processes,68,69 ultimately entering the air, terrestrial environments, and aquatic systems. Moreover, the prevalence of colorless microplastics is indicative of degradation. This is because the loss of pigments occurs during the polymer's degradative process,70–72 given that these pigments are additives characterized by relatively weak bonds with the polymer chain.
4.2. Polymer identification
Micro-Raman was used to identify and confirm the polymeric composition of the microplastics found in honey samples. Random particles were selected for analysis (n = 17). Four polymer types were identified, including polypropylene (PP; 52.9%), PET (29.4%), PE (11.8%), and PS (5.9%) (Fig. 5). Of the 17 particles analyzed, 12 were fibers, 4 were fragments, and 1 was a film.
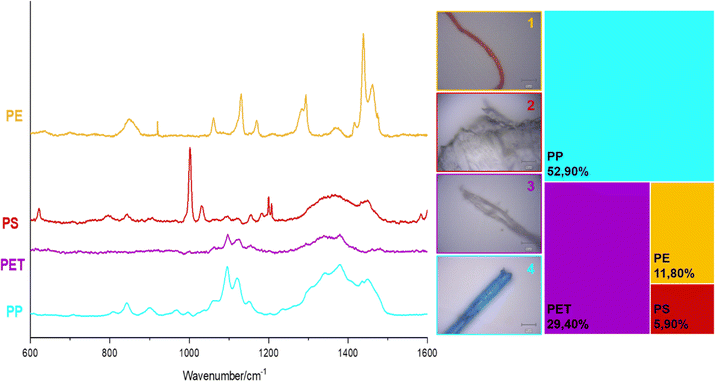 |
| Fig. 5 Composition of synthetic polymers identified by Raman spectroscopy. Representative spectra and images of each type of plastic identified in the honey samples and information on their corresponding proportions and percentages of occurrence. The particles presented were found in the samples: (1) sample A; (2) sample D; (3) sample B; (4) sample H. | |
The identified polymeric types align with the plastics predominantly produced by the global industry.73 Consequently, these extensively manufactured plastics also exhibit the highest occurrence in natural environments, as supported by multiple studies.74–76 Investigations on bee products revealed a prevalence of PET particles,77 while a field study with A. mellifera individuals highlighted the predominant presence of polyester, PE, and polyvinyl chloride.35
As previously described, this is one of the first studies to investigate the presence of microplastics in environmental samples of honey before their packaging for commercial or consumption purposes. For this reason, it is not possible to compare our findings on the polymeric composition of plastics in the honey of M. quadrifasciata with studies from different locations. The literature predominantly consists of studies that analyze samples of commercial honey, often without specific identification of the producing bee species. Consequently, the comparison of our results is restricted to a broad evaluation when aligned with findings from other studies.
Diaz-Basantes et al.34 recorded the presence of microplastics in 14 honey samples sold in supermarkets, whether industrially packaged or handcrafted. The identified polymers in these samples were PP, PE, and polyacrylamide. It is possible to note a similarity between the findings of this study and the current results. However, comparing these results requires caution, as there is a notable likelihood that the particles may have originated from atmospheric contamination during the product processing and packaging process. Furthermore, in this study, a broader range of foods was investigated, and the chemical composition was reported in general for all data rather than specifically for the particles found in honey. In the study of Liebezeit and Liebezeit,36 high concentrations of synthetic particles were reported; however, no chemical analysis was employed.
4.3. Impact of urbanization on microplastic concentrations
The sampling stations can be categorized based on two predominant characteristics: built areas (grey areas, with pavements around them) and areas with the highest proportion of vegetation (green areas). A land cover map is shown in Fig. 6. To conduct a more in-depth analysis, we grouped the points according to their matrixes: points B, D, E, F, and G are located in a built matrix (similarity >75%, SM Fig. 1), whereas points A, C and H are situated in green areas (similarity >80%, SM Fig. 1). Considering the grouped data, we found an average concentration of 0.73 and 1.88 particles per mL in green and grey areas, respectively.
 |
| Fig. 6 Land uses surrounding the study areas (900 m radius). The letters indicate the respective samples from (A) to (H) as shown in Fig. 1. | |
Although a variation in particles was found between the two types of land use, we did not find a significant correlation (p > 0.05) between the quantitative parameters of microplastics with the most vegetated area and the highest proportion of built areas. However, for more definitive conclusions regarding this relationship, it is important to carry out more research with a larger sample size, since we can clearly see a tendency to have more particles in areas with a lower proportion of vegetation (Fig. 7).
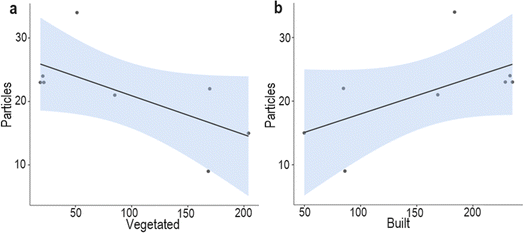 |
| Fig. 7 Scatter plot showing relationships between the number of particles and (a) vegetated and (b) built areas. | |
Urbanized areas exhibit a heightened propensity for elevated concentrations of plastics, primarily attributable to intensified human activity. The heightened urbanization involves increased plastic usage, higher pollution levels, and enhanced potential for environmental contamination compared to less developed and more vegetated areas. Although bees from the Melipona genus can reach more than 2 km from the hive, they typically do not fly more than 700 m during their foraging activities,60 (ESI†), and a radius of 900 m was shown to be efficient to verify the activity of M. quadrifasciata in an urban environment.22 Therefore, it is anticipated that areas with a higher index of plastic pollution within this radius around the hive will lead to increased interaction between bees and these residues. Consequently, it is expected that the concentrations of microplastics in these samples will be more pronounced. Thus, habitat degradation was observed as a contributing factor to the presence of microplastics in bee products.
Despite the already-known presence of microplastics in aquatic and terrestrial environments and their association with population density and urbanization,78 to date, only one study has surveyed the influences of landscape on microplastics and bees. Edo et al.35 found similar concentrations of microplastics attached to the bodies of honeybees in urban and rural areas, since the areas were close (honeybees could easily fly between them), and the particles can be dispersed by wind for long distances. However, they did not differ in the concentrations regarding the land use classes (e.g. vegetated and built areas).
We conclude that the presence of bees in urban areas often brings them into contact with microplastic-laden environments, leading to the incorporation of these particles into the honey produced in urban beehives. The combination of increased anthropogenic influence and the interaction of bees with microplastic-rich surroundings amplifies the likelihood of higher particle concentrations in honey from urban areas compared to more vegetated regions. This increased exposure raises concerns about the potential adverse effects of microplastic on native bees, their pollination services, and the potential human harm from consuming the contaminated honey.50
5. Conclusions
Our study investigated a recently debated challenge faced by bees, revealing the presence of a harmful substance in their habitats. Microplastics were found in the honey samples, highlighting the pervasive nature of these threats across various sampling locations. We reported for the first time the presence of microplastics within honey produced by Melipona quadrifasciata, a native Brazilian bee managed in an urban matrix. The spatial distribution and morphological characteristics of microplastics in São Paulo unveiled a ubiquitous presence across both urbanized (grey areas) and vegetated regions. The predominant features encompassed particles smaller than 299 μm, transparent in color, primarily composed of polypropylene, and exhibiting a fiber shape. The abundance of microplastics varied, ranging from 0.1 to 2.6 particles per mL of honey. Sampling stations situated in grey areas exhibited higher concentrations. This investigation underscores the disconcerting revelation that bees act as vectors, introducing microplastics into their hives, and consequently contaminating bee products. Such contamination raises long-term concerns about the potential harm posed by microplastics in bee-derived consumables. Our results highlight the need for further investigation with other native bee species, other beekeeping products, and other landscapes with greater sampling effort to better understand the effects of urbanization on these bees' honey and, therefore, its implications for the health of the colonies and species conservation in anthropized landscapes.
Data availability
The data supporting this article have been included as part of the ESI.†
Author contributions
Bárbara Rani-Borges: conceptualization, methodology, formal analysis, data curation, investigation, visualization, writing – original draft, and writing – review and editing. Mariana V. N. Arena: sample collection, conceptualization, formal analysis, data curation, writing – original draft, and writing – review and editing. Ingrid Naiara Gomes: data curation, writing – original draft, and writing – review and editing. Luís H. F. C. Lins: formal analysis and writing – review and editing. Livia S. C. Cestaro: formal analysis and writing – review and editing. Marcelo Pompêo: funding acquisition and writing – review and editing. Rômulo A. Ando: funding acquisition and writing – review and editing. Isabel Alves dos Santos: writing – review and editing. Rogério H. Toppa: formal analysis and data curation. Marcos Roberto Martines: formal analysis and data curation. Lucas G. Queiroz: supervision, methodology, formal analysis, data curation, investigation, visualization, writing – original draft, and writing – review and editing.
Conflicts of interest
There are no conflicts to declare.
Acknowledgements
The authors would like to thank the São Paulo State Research Support Foundation (FAPESP) (Processes 2022/15586-0 and 2021/10875-0), The Rufford Foundation (Processes 32839-1, 36332-1, and 37703-1), the Fundo Brasileiro para Biodiversidade-FUNBIO (project #0042021) and the Foundation Coordination for the Improvement of Higher Education Personnel for financial support to the project.
References
- R. G. Porto, R. F. De Almeida, O. Cruz-Neto, M. Tabarelli, B. F. Viana and C. A. Peres,
et al., Pollination ecosystem services: A comprehensive review of economic values, research funding and policy actions, Food Secur., 2020, 12(6), 1425–1442 CrossRef.
- S. G. Potts, J. C. Biesmeijer, C. Kremen, P. Neumann, O. Schweiger and W. E. Kunin, Global pollinator declines: trends, impacts and drivers, Trends Ecol. Evol., 2010, 25(6), 345–353 CrossRef PubMed.
-
R. Winfree, How Does Biodiversity Relate to Ecosystem Functioning in Natural Ecosystems?, in Unsolved Problems in Ecology, ed. Dobson A., Tilman D. and Holt R. D., Princeton University Press, 2020, pp. 338–354, [Internet], [cited 2024 Mar 19], available from: https://www.degruyter.com/document/doi/10.1515/9780691195322-027/html Search PubMed.
- T. L. Ashman, T. M. Knight, J. A. Steets, P. Amarasekare, M. Burd and D. R. Campbell,
et al., Pollen Limitation Of Plant Reproduction: Ecological And Evolutionary Causes And Consequences, Ecology, 2004, 85(9), 2408–2421 CrossRef.
- V. Patel, N. Pauli, E. Biggs, L. Barbour and B. Boruff, Why bees are critical for achieving sustainable development, Ambio, 2021, 50(1), 49–59 CrossRef PubMed.
- J. M. Gómez, M. Abdelaziz, J. Lorite, A. Jesús Muñoz-Pajares and F. Perfectti, Changes in pollinator fauna cause spatial variation in pollen limitation, J. Ecol., 2010, 98(5), 1243–1252 CrossRef.
- J. M. Gómez, J. Bosch, F. Perfectti, J. Fernández and M. Abdelaziz, Pollinator diversity affects plant reproduction and recruitment: the tradeoffs of generalization, Oecologia, 2007, 153(3), 597–605 CrossRef PubMed.
- D. M. Katumo, H. Liang, A. C. Ochola, M. Lv, Q. F. Wang and C. F. Yang, Pollinator diversity benefits natural and agricultural ecosystems, environmental health, and human welfare, Plant Divers., 2022, 44(5), 429–435 CrossRef PubMed.
- T. C. Giannini, S. Boff, G. D. Cordeiro, E. A. Cartolano, A. K. Veiga and V. L. Imperatriz-Fonseca,
et al., Crop pollinators in Brazil: a review of reported interactions, Apidologie, 2015, 46(2), 209–223 CrossRef.
- S. A. M. Khalifa, E. H. Elshafiey, A. A. Shetaia, A. A. A. El-Wahed, A. F. Algethami and S. G. Musharraf,
et al., Overview of Bee Pollination and Its Economic Value for Crop Production, Insects, 2021, 12(8), 688 CrossRef PubMed.
- R. Winfree, The conservation and restoration of wild bees, Ann. N. Y. Acad. Sci., 2010, 1195(1), 169–197 CrossRef PubMed.
- S. Ávila, M. R. Beux, R. H. Ribani and R. C. Zambiazi, Stingless bee honey: Quality parameters, bioactive compounds, health-promotion properties and modification detection strategies, Trends Food Sci. Technol., 2018, 81, 37–50 CrossRef.
- A. Nordin, N. Q. A. V. Sainik, S. R. Chowdhury, A. B. Saim and R. B. H. Idrus, Physicochemical properties of stingless bee honey from around the globe: A comprehensive review, J. Food Compos. Anal., 2018, 73, 91–102 CrossRef CAS.
- M. Sajid, M. Yamin, F. Asad, S. Yaqub, S. Ahmad and M. A. M. S. Mubarik,
et al., Comparative study of physio-chemical analysis of fresh and branded honeys from Pakistan, Saudi J. Biol. Sci., 2020, 27(1), 173–176 CrossRef CAS PubMed.
- J. M. Alvarez-Suarez, S. Tulipani, S. Romandini, E. Bertoli and M. Battino, Contribution of honey in nutrition and human health: a review, Mediterr. J. Nutr. Metab., 2010, 3(1), 15–23 CrossRef.
- C. Maia-Silva, M. Hrncir, C. I. Da Silva and V. L. Imperatriz-Fonseca, Survival strategies of stingless bees (Melipona subnitida) in an unpredictable environment, the Brazilian tropical dry forest, Apidologie, 2015, 46(5), 631–643 CrossRef.
- A. Easton-Calabria, K. C. Demary and N. J. Oner, Beyond Pollination: Honey Bees (Apis mellifera) as Zootherapy Keystone Species, Front. Ecol. Evol., 2019, 6, 161 CrossRef.
- V. R. Pasupuleti, L. Sammugam, N. Ramesh and S. H. Gan, Honey, Propolis, and Royal Jelly: A Comprehensive Review of Their Biological Actions and Health Benefits, Oxid. Med. Cell. Longevity, 2017, 2017, 1–21 CrossRef PubMed.
- M. C. Rodríguez, L. Dupont-Courtade and W. Oueslati, Air pollution and urban structure linkages: Evidence from European cities, Renewable Sustainable Energy Rev., 2016, 53, 1–9 CrossRef.
- R. Dris, J. Gasperi, V. Rocher, M. Saad, N. Renault and B. Tassin, Microplastic contamination in an urban area: a case study in Greater Paris, Environ. Chem., 2015, 12(5), 592 CrossRef CAS.
- K. Wang, J. Li, L. Zhao, X. Mu, C. Wang and M. Wang,
et al., Gut microbiota protects honey bees (Apis mellifera L.) against polystyrene microplastics exposure risks, J. Hazard. Mater., 2021, 402, 123828 CrossRef CAS PubMed.
- M. V. N. Arena, R. H. Toppa, M. Martines and I. Alves-dos-Santos, Release experiments as an indicator of flying activities of stingless bees in urban areas, Front. Sustainable Cities, 2023, 4, 1103835 CrossRef.
- N. H. Salman, L. Mok Sam, K. Ador, B. Binjamin, M. I. J. Johny-Hasbulah and S. Benedick, Linking Measure of the Tropical Stingless Bee (Apidae, Meliponini, and Heterotrigona itama) Honey Quality with Hives Distance to the Source of Heavy Metal Pollution in Urban and Industrial Areas in Sabah, Borneo, J. Toxicol., 2022, 2022, 1–7 CrossRef PubMed.
- S. Bogdanov, Contaminants of bee products, Apidologie, 2006, 37(1), 1–18 CrossRef CAS.
- N. Gallai, J. M. Salles, J. Settele and B. E. Vaissière, Economic valuation of the vulnerability of world agriculture confronted with pollinator decline, Ecol. Econ., 2009, 68(3), 810–821 CrossRef.
- J. M. R. B. V. Aguiar, R. C. F. Nocelli, M. Giurfa and F. S. Nascimento, Neonicotinoid effects on tropical bees: Imidacloprid impairs innate appetitive responsiveness, learning and memory in the stingless bee Melipona quadrifasciata, Sci. Total Environ., 2023, 877, 162859 CrossRef CAS PubMed.
- V. E. Seide, R. C. Bernardes, E. J. G. Pereira and M. A. P. Lima, Glyphosate is lethal and Cry toxins alter the development of the stingless bee Melipona quadrifasciata, Environ. Pollut., 2018, 243, 1854–1860 CrossRef CAS PubMed.
- J. Shaw, C. Cunningham, S. Harper, A. Ragazzon-Smith, P. R. Lythgoe and T. R. Walker, Biomonitoring of honey metal(loid) pollution in Northwest England by citizen scientists, Environ. Adv., 2023, 13, 100406 CrossRef CAS.
- H. V. V. Tomé, W. F. Barbosa, G. F. Martins and R. N. C. Guedes, Spinosad in the native stingless bee Melipona quadrifasciata: Regrettable non-target toxicity of a bioinsecticide, Chemosphere, 2015, 124, 103–109 CrossRef PubMed.
- A. R. Gomes, Í. N. Freitas, T. M. D. Luz, A. T. B. Guimarães, A. P. D. C. Araújo and C. Kamaraj,
et al., Multiple endpoints of polyethylene microplastics toxicity in vascular plants of freshwater ecosystems: A study involving Salvinia auriculata (Salviniaceae), J. Hazard. Mater., 2023, 450, 131069 CrossRef CAS PubMed.
- A. M. Alma, G. S. De Groot and M. Buteler, Microplastics incorporated by honeybees from food are transferred to honey, wax and larvae, Environ. Pollut., 2023, 320, 121078 CrossRef CAS PubMed.
- R. C. Thompson, Y. Olsen, R. P. Mitchell, A. Davis, S. J. Rowland and A. W. G. John,
et al., Lost at Sea: Where Is All the Plastic?, Science, 2004, 304(5672), 838 CrossRef CAS PubMed.
- K. Kerkich, B. Bouargane, A. El Laghdach, B. Souhail and Y. Kadmi, Recent advances in the extraction, purification and analysis of emerging pesticides in honey products: A review, J. Food Compos. Anal., 2023, 105947 Search PubMed.
- M. F. Diaz-Basantes, J. A. Conesa and A. Fullana, Microplastics in Honey, Beer, Milk and Refreshments in Ecuador as Emerging Contaminants, Sustainability, 2020, 12(14), 5514 CrossRef.
- C. Edo, A. R. Fernández-Alba, F. Vejsnæs, J. J. M. Van Der Steen, F. Fernández-Piñas and R. Rosal, Honeybees as active samplers for microplastics, Sci. Total Environ., 2021, 767, 144481 CrossRef CAS PubMed.
- G. Liebezeit and E. Liebezeit, Origin of Synthetic Particles in Honeys, Pol. J. Food Nutr. Sci., 2015, 65(2), 143–147 CrossRef CAS.
- G. Liebezeit and E. Liebezeit, Non-pollen particulates in honey and sugar, Food Addit. Contam.: Part A, 2013, 30(12), 2136–2140 CrossRef CAS PubMed.
-
C. D. Michener, The Bees of the World, Johns Hopkins Univ. Press, Baltimore, Md, 2000, p. 913 Search PubMed.
- Y. Al Naggar, C. M. Sayes, C. Collom, T. Ayorinde, S. Qi and H. R. El-Seedi,
et al., Chronic Exposure to Polystyrene Microplastic Fragments Has No Effect on Honey Bee Survival, but Reduces Feeding Rate and Body Weight, Toxics, 2023, 11(2), 100 CrossRef CAS PubMed.
- P. Balzani, G. Galeotti, S. Scheggi, A. Masoni, G. Santini and D. Baracchi, Acute and chronic ingestion of polyethylene (PE) microplastics has mild effects on honey bee health and cognition, Environ. Pollut., 2022, 305, 119318 CrossRef CAS PubMed.
- M. Buteler, A. M. Alma, T. Stadler, A. C. Gingold, M. C. Manattini and M. Lozada, Acute toxicity of microplastic fibers to honeybees and effects on foraging behavior, Sci. Total Environ., 2022, 822, 153320 CrossRef CAS PubMed.
- Y. Deng, X. Jiang, H. Zhao, S. Yang, J. Gao and Y. Wu,
et al., Microplastic Polystyrene Ingestion Promotes the Susceptibility of Honeybee to Viral Infection, Environ. Sci. Technol., 2021, 55(17), 11680–11692 CrossRef CAS PubMed.
- E. Pasquini, F. Ferrante, L. Passaponti, F. S. Pavone, I. Costantini and D. Baracchi, Microplastics reach the brain and interfere with honey bee cognition, Sci. Total Environ., 2024, 912, 169362 CrossRef CAS PubMed.
-
W. A. Azmi, Y. S. Ibrahim, M. N. Rosazan, M. I. I. Mamat, and S. T. Anuar, Detection of Microplastics in Honey of Stingless Bee (Heterotrigona Itama) and Honey Bee (Apis Mellifera) from Malaysia, [Internet], SSRN, 2023, [cited 2024 Mar 19], available from: https://www.ssrn.com/abstract=4632805 Search PubMed.
- T. A. Viana, L. L. Botina, R. C. Bernardes, W. F. Barbosa, T. K. D. Xavier and M. A. P. Lima,
et al., Ingesting microplastics or nanometals during development harms the tropical pollinator Partamona helleri (Apinae: Meliponini), Sci. Total Environ., 2023, 893, 164790 CrossRef CAS PubMed.
- J. S. Bonsucesso, T. V. Gloaguen, A. S. Do Nascimento, C. A. L. De Carvalho, S. De and F. Dias, Metals in geopropolis from beehive of Melipona scutellaris in urban environments, Sci. Total Environ., 2018, 634, 687–694 CrossRef CAS PubMed.
- C. D. G. M. D. E. Pinheiro, F. A. D. S. Oliveira, S. C. S. Oloris, J. B. A. Da Silva and B. Soto-Blanco, Pesticide Residues in Honey from Stingless Bee Melipona Subnitida (Meliponini, Apidae), J. Apic. Sci., 2020, 64(1), 29–36 CAS.
- R. Qiao, M. Mortimer, J. Richter, B. Rani-Borges, Z. Yu and M. Heinlaan,
et al., Hazard of polystyrene micro-and nanospheres to selected aquatic and terrestrial organisms, Sci. Total Environ., 2022, 853, 158560 CrossRef CAS PubMed.
- B. Rani-Borges, R. Meitern, P. Teesalu, M. Raudna-Kristoffersen, R. Kreitsberg and M. Heinlaan,
et al., Effects of environmentally relevant concentrations of microplastics on amphipods, Chemosphere, 2022, 309, 136599 CrossRef CAS PubMed.
- S. Shah, M. Ilyas, R. Li, J. Yang and F. L. Yang, Microplastics and Nanoplastics Effects on Plant–Pollinator Interaction and Pollination Biology, Environ. Sci. Technol., 2023, 57(16), 6415–6424 CrossRef CAS PubMed.
-
United Nations, The World's Cities in 2018, [Internet], 2018, available from: https://www.un.org/en/development/desa/population/publications/pdf/urbanization/the_worlds_cities_in_2018_data_booklet.pdf Search PubMed.
-
CETESB, Relatório de Qualidade do Ar no Estado de São Paulo-2020, 2021, [Internet], available from: http://cetesb.sp.gov.br/ar/publicacoes-relatorios/ Search PubMed.
- M. Gavidia-Calderón, D. Schuch, A. Vara-Vela, R. Inoue, E. D. Freitas and T. T. D. A. Albuquerque,
et al., Air quality modeling in the metropolitan area of São Paulo, Brazil: A review, Atmos. Environ., 2024, 319, 120301 CrossRef PubMed.
-
J. M. F. Camargo, S. R. M. Pedro, G. A. R. Melo. and L. Meliponini, Catalogue of Bees (Hymenoptera, Apoidea) in the Neotropical Region, 2012, 1836, [Internet], [cited 2023 Dec 29], available from: http://moure.cria.org.br/catalogue?id=117368 Search PubMed.
- G. Moretto and M. C. Arias, Detection of mitochondrial DNA restriction site differences between the subspecies of Melipona quadrifasciata Lepeletier (Hymenoptera: Apidae: Meliponini), Neotrop. Entomol., 2005, 34(3), 381–385 CrossRef CAS.
- L. Nunes, G. Passos, C. Carvalho and E. Araújo, Size and shape in Melipona quadrifasciata anthidioides Lepeletier, 1836 (Hymenoptera; Meliponini), Braz. J. Biol., 2013, 73(4), 887–893 CrossRef CAS PubMed.
-
J. S. Moure, D. Urban, and G. A. R. Melo. Catalogue of Bees (Hymenoptera, Apoidea) in the Neotropical Region, SBE, 2007 Search PubMed.
- L. Wu, B. Du, Y. Vander Heyden, L. Chen, L. Zhao and M. Wang,
et al., Recent advancements in detecting sugar-based adulterants in honey – A challenge, TrAC, Trends Anal. Chem., 2017, 86, 25–38 CrossRef CAS.
-
P. Nogueira Neto, Vida E Criacao De Abelhas Indigenas Sem Ferao. Nogueirapis Edições, 1997 Search PubMed.
- S. S. Greenleaf, N. M. Williams, R. Winfree and C. Kremen, Bee foraging ranges and their relationship to body size, Oecologia, 2007, 153(3), 589–596 CrossRef PubMed.
-
J. W. Rouse, R. H. Haas, J. A. Schell, and D. W. Deering, Monitoring Vegetation Systems in the Great Plains with ERTS (Earth Resources Technology Satellite), in Proceedings of 3rd Earth Resources Technology Satellite Symposium, Greenbelt, 1974. pp. 309–317 Search PubMed.
-
USGS, Landsat Surface Reflectance-Derived Spectral Indices, 2024, [Internet], [cited 2024 Feb 1], available from: https://www.usgs.gov/landsat-missions/landsat-surface-reflectance-derived-spectral-indices Search PubMed.
-
T. G. Florenziano, Iniciação Em Sensoriamento Remoto, Oficina de Textos, 2011 Search PubMed.
- E. A. Sherman, Note on Multiple Comparisons Using Rank Sums, Technometrics, 1965, 7(2), 255–256 CrossRef.
-
R Core Team, R: A language and environment for statistical computing, R Foundation for Statistical Computing, 2022, http://www.r-project.org/index.html, Available from: http://www.r-project.org/index.html Search PubMed.
-
Jamovi, the Jamovi Project (Version 2.5), [Internet], 2024, Available from: https://www.jamovi.org Search PubMed.
- H. Kye, J. Kim, S. Ju, J. Lee, C. Lim and Y. Yoon, Microplastics in water systems: A review of their impacts on the environment and their potential hazards, Heliyon, 2023, 9(3), e14359 CrossRef CAS PubMed.
- S. N. Athey and L. M. Erdle, Are We Underestimating Anthropogenic Microfiber Pollution? A Critical Review of Occurrence, Methods, and Reporting, Environ. Toxicol. Chem., 2022, 41(4), 822–837 CrossRef CAS PubMed.
- R. Ramasamy and R. B. Subramanian, Synthetic textile and microfiber pollution: a review on mitigation strategies, Environ. Sci. Pollut. Res., 2021, 28(31), 41596–41611 CrossRef CAS PubMed.
- H. Luo, Y. Xiang, Y. Li, Y. Zhao and X. Pan, Weathering alters surface characteristic of TiO2-pigmented microplastics and particle size distribution of TiO2 released into water, Sci. Total Environ., 2020, 729, 139083 CrossRef CAS PubMed.
- H. Luo, Y. Zhao, Y. Li, Y. Xiang, D. He and X. Pan, Aging of microplastics affects their surface properties, thermal decomposition, additives leaching and interactions in simulated fluids, Sci. Total Environ., 2020, 714, 136862 CrossRef CAS PubMed.
- X. Zhao, J. Wang, K. M. Yee Leung and F. Wu, Color: An Important but Overlooked Factor for Plastic Photoaging and Microplastic Formation, Environ. Sci. Technol., 2022, 56(13), 9161–9163 CrossRef CAS PubMed.
- R. Geyer, J. R. Jambeck and K. L. Law, Production, use, and fate of all plastics ever made, Sci. Adv., 2017, 3(7), e1700782 CrossRef PubMed.
- L. Huang, S. Zhang, L. Li, S. Zhang, J. Wang and X. Liu,
et al., Research progress on microplastics pollution in polar oceans, Polar Sci., 2023, 36, 100946 CrossRef.
- M. Raju, R. Gandhimathi and P. V. Nidheesh, The cause, fate and effect of microplastics in freshwater ecosystem: Ways to overcome the challenge, J. Water Process Eng., 2023, 55, 104199 CrossRef.
- H. Yang, Y. Yumeng, Y. Yu, H. Yinglin, B. Fu and J. Wang, Distribution, sources, migration, influence and analytical methods of microplastics in soil ecosystems, Ecotoxicol. Environ. Saf., 2022, 243, 114009 CrossRef CAS.
- P. Mühlschlegel, A. Hauk, U. Walter and R. Sieber, Lack of evidence for microplastic contamination in honey, Food Addit. Contam.: Part A, 2017, 34(11), 1982–1989 CrossRef PubMed.
- J. K. H. Wong, K. K. Lee, K. H. D. Tang and P. S. Yap, Microplastics in the freshwater and terrestrial environments: Prevalence, fates, impacts and sustainable solutions, Sci. Total Environ., 2020, 719, 137512 CrossRef CAS PubMed.
|
This journal is © The Royal Society of Chemistry 2024 |
Click here to see how this site uses Cookies. View our privacy policy here.