Finding non-fluorinated alternatives to fluorinated gases used as refrigerants†
Received
23rd July 2024
, Accepted 9th September 2024
First published on 3rd October 2024
Abstract
Hydrofluorocarbons (HFCs) and so-called hydrofluoroolefins (HFOs) are used as refrigerants in air conditioning, refrigeration, chillers, heat pumps and devices for dehumidification and drying. However, many HFCs, including R-134a and R-125, have a high global warming potential and some of the HFCs and HFOs degrade atmospherically and form trifluoroacetic acid (TFA) as a persistent degradation product. Rising levels of TFA around the globe reveal an urgent need to replace fluorinated refrigerants with non-fluorinated working fluids to avoid direct emissions due to leakage, incorrect loading or removal. It is important, however, also to select refrigerants with high efficiencies to avoid increasing indirect CO2 emissions due to higher energy consumption during the use phase. The present study investigates the available non-fluorinated alternatives to fluorinated refrigerants and shows that a transition to non-fluorinated refrigerants, in general, is possible and has happened in many sectors already. Technically, there are only slight barriers to overcome in order to replace fluorinated refrigerants in almost all newly developed systems conforming to existing standards. Additionally, we show that alternatives are available even for some use cases for which derogations have been proposed in the EU PFAS restriction proposal and suggest making these derogations more specific to support a speedy transition to non-fluorinated refrigerants in all sectors.
Environmental significance
Fluorinated gases that contain at least one fully fluorinated methyl (CF3–) or methylene (–CF2–) carbon atom are either persistent themselves or can form persistent degradation products. One of these degradation products is trifluoroacetic acid (TFA). Levels of TFA in the environment are increasing and will continue to increase if sources of TFA are not curbed. Replacing fluorinated gases with non-fluorinated alternatives is therefore of high importance as fluorinated gases are in terms of volume one of the largest group of precursors to TFA. Many of the fluorinated gases have also a lower efficiency than non-fluorinated gases when used as refrigerants, which leads to a higher CO2 footprint of refrigeration systems using those fluorinated gases.
|
1 Introduction
In 1985, the stratospheric ozone layer over Antarctica was found to be partially destroyed,1 and strong regulatory action was taken in the following years to protect the ozone layer. In particular, the most important step was the 1987 Montreal Protocol regulating ozone-depleting substances such as chlorofluorocarbons (CFCs) that were used as refrigerants during this time.
The Montreal Protocol entered into force in January 1989. Following its implementation, CFCs used in refrigeration and air conditioning systems were substituted with hydrochlorofluorocarbons (HCFCs) and hydrofluorocarbons (HFCs).2 HCFCs deplete stratospheric ozone, but to a much lesser extent than CFCs, whereas HFCs have zero ozone depletion potential.3 In 1996, HCFCs were also added as controlled substances to the Montreal Protocol and most companies in the refrigeration and air conditioning sector switched to HFCs.2,4 Alternatives based on hydrocarbons such as propane and butane were also developed. Due to charge limitation (below 150 g), in accordance with the standard EN 378 for unrestricted placement of equipment due to flammability, hydrocarbons were initially only applied in domestic refrigerators in which they are now dominant all over the world. In other sectors, however, because of concerns over their flammability and because they were not manufactured or controlled by a refrigerant manufacturing company, hydrocarbons did not achieve a great market share in the early days after the Montreal Protocol.2,5 The United States (US) health and safety regulations even prevented hydrocarbon refrigerators from entering the market.2,5
While HFCs do not deplete the stratospheric ozone layer, many have a high global warming potential (GWP).6 The parties to the Montreal Protocol agreed, therefore, in 2016 to the Kigali Amendment to gradually reduce the use of substances with high GWP depending on the sector, the refrigerant charge, and year of implementation.7 The regulation of HFCs in the European Union (EU) started even earlier with the European F-gas Regulation. The first version of the F-gas Regulation entered into force in 2006.8 The third (and most current) version was adapted in February 2024.9 The aim of the F-gas Regulation is to replace high-GWP F-gases with alternatives with a low GWP using a quota system based on the total allowed annual sales of F-gases contributing to greenhouse gas emissions, combined with a set of product bans. According to the European Environment Agency, the EU-wide placing on the market of HFCs in 2022 was below the market limit set in the F-gas Regulation and also below the maximum imposed by the Kigali Amendment.10
However, the substances proposed to replace HFCs – so-called hydrofluoroolefins (HFOs) and even the currently applied HFCs with lower GWP – might contribute to another potential environmental problem. Several of the HFOs and HFCs can in part or completely be converted in the atmosphere into trifluoroacetic acid (TFA).11 TFA is a persistent and mobile substance and the terminal breakdown product of many per- and polyfluoroalkyl substances (PFASs). Under the EU's Regulation on classification and labelling, TFA is classified as causing severe skin burns and eye damage (H314), harmful if inhaled (H332), and harmful to aquatic life with long-lasting effects (H412).12 Germany intends to additionally classify TFA as toxic if inhaled (H331) and as a reproductive toxicant.13
TFA has been accumulating in the environment in many parts of the world for decades. For example, TFA levels have increased in: surface water in Beijing, China 17-fold between 2002 and 2012,14 in Arctic ice almost 10-fold between pre-1990 and post-2000,15 in precipitation in Germany 3- to 5-fold between 1996/1997 and 2018/2020,11 in US surface waters 6-fold between 1998 and 2021,16 and in leaves of various tree species in Germany between 1985 and 2022.11,17,18
Claims that the occurrence of TFA in the environment has mainly natural causes have been disproved.19,20 Instead, evidence shows anthropogenic sources of TFA.11,21,22 Samples from older German and Swiss groundwaters23,24 and pre-industrial samples from Greenland and Denmark20 did not contain TFA and therefore support the anthropogenic origin of TFA. Recent data for TFA in rain and snow in Switzerland,24,25 Japan,26 China,27 the US,28,29 Poland,30 the Netherlands,31 and Germany21,32 show concentrations up to the μg L−1-range. Some of these measured concentrations are close to the revised groundwater/drinking water health guidance value of 60 μg L−1 TFA set by Germany in 2020.21,33 The drinking water health guidance value is based on the life-long tolerable daily intake of TFA, at which no harm to human health is to be expected, and was established because of concerns about build-ups of TFA over time. Concentrations close to Germany's health guidance value for TFA in so many places of the world are an alarming signal and show that emissions need to be stopped.
If current sources of TFA are not curbed, concentrations will continue to increase, and the probability of unforeseen effects will also increase.34 Undoing such effects will be very difficult, if possible at all, and will require huge efforts and costs, as e.g., TFA will not degrade in the environment and can also not be removed from (waste)water with common water treatment methods, such as ozonation or activated carbon filtration.11 These risks should be reduced by reducing or avoiding the use of the refrigerants concerned at an early stage. Thus, the way forward has to be to regulate precursors of TFA as soon as possible.
The third version of the European F-gas Regulation intends to tighten the action on fluorinated refrigerants with high and mid-high GWP. HFOs and HFCs with low GWP that can form TFA and other persistent degradation products are not addressed. However, HFOs and HFCs that contain a –CF2– or –CF3 unit (without any H/Cl/Br/I attached to it) are included in the PFAS restriction proposal published by the European Chemicals Agency (ECHA) in February 2023.35 These include R-1234yf, R-1234ze, and R-1233zd (Table 2). The aim of the restriction proposal is a comprehensive ban of the uses of PFASs. Time-limited derogations are foreseen for those uses where alternatives are currently not available. Some derogations are also proposed for fluorinated gases used as refrigerants (Table 1). However, some industry producers are calling for even broader exemptions and derogations based on the argument that fluorinated gases are ‘critical solutions’ and that there are no alternatives ‘that deliver the same high level of performance’.36
Table 1 Proposed derogations in the PFAS restriction proposal submitted to ECHA in January 2023.35 Entries under “Paragraph 5” provide the letter under which the respective derogation is listed in the restriction proposal
Paragraph 5 |
Use |
Proposed derogation perioda |
Years after ‘entry into force’, not including the 18 months transition period and the length of time to adopt the proposed restriction.
|
F |
Refrigerants in low temperature refrigeration below −50 °C |
5 |
G |
Refrigerants in laboratory test and measurement equipment |
12 |
H |
Refrigerants in refrigerated centrifuges |
12 |
I |
Maintenance and refilling of existing HVACR equipment put on the market before 18 months after entry into force and for which no drop-in alternative exists |
12 |
J |
Refrigerants in HVACR equipment in buildings where national safety standards and building codes prohibit use of alternatives |
Unlimited |
P |
Refrigerants in mobile air conditioning systems in combustion engine vehicles with mechanical compressors |
5 |
Q |
Refrigerants in transport refrigeration other than in marine applications |
5 |
The present study explores non-fluorinated alternatives for refrigerants and other heat transfer fluids where fluorinated gases are still commonly used.11,37,38 The focus of the study is on uses in Europe, but many of the alternatives discussed might also be available in other markets. We also discuss the barriers that might exist in the EU for transitioning to non-fluorinated working fluid alternatives. The investigated uses are refrigerants in refrigeration, indoor climate (heat pumps, air conditioning, chillers) and miscellaneous uses. For definitions of the terms see the ESI Section S1.†
2 Fluorinated gases used as refrigerants
Fluorinated gases, here defined as all gases that contain a –CF3 or –CF2– moiety, are used in various applications as refrigerants. Table 2 lists the fluorinated gases mentioned in the present study, along with their 100-year GWP, and whether they are included in Annex I, II, or III of the current F-gas Regulation.9 HFCs in Annex I are included in the quota system whereas the substances in Annex II and III are not. Additionally, Table 3 lists the blends of fluorinated gases mentioned in the present study. The HFCs in the blends (if listed in Annex I) are again part of the quota system. The European F-gas Regulation uses the term ‘fluorinated greenhouse gases’ when referring to the substances listed in Annex I, II or III of the Regulation or mixtures containing any of those substances. We will use this term here when referring to the relevant parts of the F-gas Regulation; otherwise, the term ‘fluorinated gases’ will be used.
Table 2 Fluorinated gases mentioned in the present study. The 100-year GWP was taken from the third version of the European F-gas Regulation,9 the percentages of TFA formation from Behringer et al. (2021).11 The values for the HFCs for the 100-year GWP (GWP-100) in the third version of the European F-gas Regulation are from the fourth assessment report of the Intergovernmental Panel on Climate Change (IPCC) and are lower than the values for the 100 year GWP from the sixth assessment report of the IPCC.45 These outdated (and lower) values were kept here to be in line with the F-gas Regulation and the thresholds set there
CAS RN |
Industrial designation |
Refrigerant designation |
Chemical name |
Chemical formula |
GWP 100 |
Formation of TFA |
PFAS (according to ref. 35) |
Included in Annex I of the F-gas Regulation
|
75-46-7 |
HFC-23 |
R-23 |
Trifluoromethane |
CHF3 |
14 800 |
No |
No |
75-10-5 |
HFC-32 |
R-32 |
Difluoromethane |
CH2F2 |
675 |
No |
No |
354-33-6 |
HFC-125 |
R-125 |
Pentafluoroethane |
CHF2CF3 |
3500 |
No |
Yes |
359-35-3 |
HFC-134 |
R-134 |
1,1,2,2-Tetrafluoroethane |
CHF2CHF2 |
1100 |
No |
No |
811-97-2 |
HFC-134a |
R-134a |
1,1,1,2-Tetrafluoroethane |
CH2FCF3 |
1430 |
7–20% |
Yes |
420-46-2 |
HFC-143a |
R-143a |
1,1,1-Trifluoroethane |
CH3CF3 |
4470 |
Up to 10% |
Yes |
75-37-6 |
HFC-152a |
R-152a |
1,1-Difluoroethane |
CH3CHF2 |
124 |
No |
No |
460-73-1 |
HFC-245fa |
R-245fa |
1,1,1,3,3-Penta-fluoropropane |
CHF2CH2CF3 |
1030 |
No |
Yes |
![[thin space (1/6-em)]](https://www.rsc.org/images/entities/char_2009.gif) |
Included in Annex II of the F-gas Regulation
|
754-12-1 |
HFC-1234yf |
R-1234yf |
2,3,3,3-Tetrafluoropropene |
CF3CF CH2 |
0.501 |
100% |
Yes |
1645-83-6, 29118-25-0 |
HFC-1234ze |
R-1234ze |
1,3,3,3-Tetrafluoropropene |
trans CHF CHCF3 |
1.37 |
Up to 10% |
Yes |
2730-43-0, 102687-65-0 |
HCFO-1233zd |
R-1233zd |
trans-1-Chloro-3,3,3-trifluoroprop-1-ene |
trans CF3CH CHCl |
3.88 |
2% |
Yes |
Table 3 Blends of fluorinated gases mentioned in the present study. The composition of the blends are taken from Behringer11et al. (2021). The GWP 100 was calculated from the GWP values of the individual substances given in Table 2 that refer to the values from the fourth assessment report of the IPCC
Refrigerant designation |
Composition |
GWP 100 |
R-404A |
44% R-125, 4% R-134a, 52% R-143a |
3922 |
R-407C |
23% R-32, 25% R-125, 52% R-134a |
1774 |
R-410A |
50% R-32, 50% R-125 |
2088 |
R-449A |
24.3% R-32, 24.7% R-125, 25.7% R-134a, 25.3% R-1234yf |
1396 |
R-452A |
11% R-32, 59% R-125, 30% R-1234yf |
2139 |
R-454B |
68.9% R-32, 31.1% R-1234yf |
465 |
R-454C |
21.5% R-32, 78.5% R-1234yf |
146 |
R-455A |
21.5% R-32, 75.5% R-1234yf, 3% CO2 |
146 |
R-513A |
44% R-134a, 56% R-1234yf |
629 |
With the substances listed in Tables 2 and 3, we see two main problems. First, some of the fluorinated gases have very high GWPs. R-23, R-125, R-134, R134a, R143a and R-245fa have a GWP that is more than 1000 times higher than that of CO2. R-32 and R-152a have a lower GWP, but they are still more than 100 times higher than that of CO2. The high GWP of these substances is addressed in the F-gas Regulation and a phase-out has been started. So, there is a need to find alternatives here. Second, several of the fluorinated gases form TFA as persistent degradation product. As described before, TFA is accumulating in the environment and cannot be removed from water with common treatment methods. TFA will be addressed in the PFAS restriction proposal but for this it is important to show that non-fluorinated alternatives can be used as refrigerants in the long term.
3 Non-fluorinated gases used as refrigerants
There are different non-fluorinated gases available that can be applied as refrigerants depending on their required temperature levels and the thermal capacities of the systems. Examples include propane (R-290), (iso)butane (R-600a), ammonia (R-717), water (R-718), CO2 (R-744), other hydrocarbons as well as helium and nitrogen. The non-fluorinated refrigerants cover the entire temperature range that is needed in the different applications, although none of them covers the range alone. This is very similar to the fluorinated refrigerants where also not a single refrigerant covers the entire range (Fig. 1). Very important is also the refrigerant-dependent compressor efficiency. It has been shown e.g., that the largest share of the emissions in CO2-equivalents during the life-cycle of a heat pump is due to indirect emissions caused by the electricity consumption during the heat pump use phase.40,41 The direct emissions of the refrigerant in CO2 equivalents during refrigerant production and refrigerant leakage are small compared to emissions during the heat pump use phase. To keep the indirect emissions low, the refrigerant-dependent system energy efficiency (expressed as the seasonal coefficient of performance (SCOP)) needs to be high. The SCOP can vary based on the investigated refrigerant cycle configurations.40 Importantly, based on measured data for air-to-water heat pumps, it has been shown that the non-fluorinated refrigerants have similar if not even higher SCOP values than fluorinated refrigerants. The measured SCOP values reported in Vering et al. (2023)41 were 4.2, 4.19, 3.81 and 4.27 for propane, propene, (iso)-butane and ammonia and 3.71, 3.99 and 3.82 for R-410A, R-32 and R-1234yf, respectively.
 |
| Fig. 1 Application range of some of the fluorinated and some of the non-fluorinated refrigerants. The lower limit is the normal boiling point, the upper limit is the temperature 15 °C below the substance's critical temperature. Exceptions are R-744 and R-718. Graphic is cut at −100 °C and 140 °C. Adapted from IEA [2020].39 | |
Energy efficient utilization of CO2 as working fluid in refrigeration units is possible when hot water is produced enabling a large water temperature lift from below 10 °C to above 80 °C. For central refrigeration systems in commercial stores it has been shown that systems with CO2 can be operated more energy efficiently than direct HFC/CO2 cascade and HFC systems.42–44 According to these results, non-fluorinated refrigerants can be used energy-efficiently for applications over a wide temperature range.
Therefore, when selected carefully for an appropriate application, non-fluorinated refrigerants can replace fluorinated refrigerants in many applications. ESI Section S2† contains a detailed description of the mentioned non-fluorinated refrigerants. Table 4 gives a short overview of the most important non-fluorinated refrigerants, their thermodynamic properties, advantages, and disadvantages.
Table 4 Overview of the non-fluorinated gases that can be used as refrigerants and their advantages and disadvantages. TB is the normal boiling point, TC is the critical temperature
Refrigerant |
Thermodynamic properties |
Advantages |
Disadvantages |
Propane (R-290) |
T
B: −42.1 °C |
GWP of 0.02 (ref. 45), zero ODP, no by-product formation,46 high SCOP |
Flammable with a safety group classification according to standard EN 378-1 of A3 |
T
C: 96.8 °C |
(Iso)butane (R-600a) |
T
B: −11.7 °C |
GWP of 0.006 (ref. 45), zero ODP, no by-product formation46 |
Flammable with a safety group classification according to standard EN 378-1 of A3 |
T
C: 134.7 °C |
Ammonia (R-717) |
T
B: −33.3 °C |
Zero GWP, zero ODP, short atmospheric lifetime, no by-product formation, very high SCOP |
Toxic with a safety-group classification according to standard EN 378-1 of B2L |
T
C: 132.4 °C |
Water (R-718) |
T
B: 100 °C |
Non-flammable, no toxicity, zero GWP, zero ODP, no by-product formation |
Large pressure ratio, large volume flow rate due to low density and high exhaust temperature47,48 |
T
C: 373.9 °C |
CO2 (R-744) |
T
B: −78 °C |
Non-flammable (A1 safety classification), low toxicity, GWP of 1, zero ODP, no by-product formation; large temperature lift enables water heating up to 90 °C; above 5 bar system pressure at −50 °C, enables effective food freezing processes |
Due to low critical temperature often operated in transcritical cycles to enable heat rejection, typically operated at higher pressure levels than fluorocarbons and other refrigerants (but lower pressure ratios, and much smaller tube dimensions due to higher density) |
T
C: 31.1 °C |
Other hydrocarbons |
|
Ethane, ethene, propene, and methane have all a very low GWP, no ODP and do not form by-products |
Flammable with a safety group classification according to standard EN 378-1 of A3, low critical temperature of some of the hydrocarbons |
Helium and nitrogen |
T
B: −268.9 °C (helium) |
Zero GWP, zero ODP, no by-product formation, non-combustible |
Exposure to very high levels of pure nitrogen or helium can lead to suffocation.49,50 |
T
B: −195.8 °C (nitrogen) |
For ease of reading, the chemical names of the non-fluorinated gases are used throughout the text instead of the refrigerant designations.
4 Existing systems with non-fluorinated refrigerants
A simple refrigerant cycle includes the phases of compression, condensation, expansion, and evaporation (ESI Section S3.1†). The selection of the most suitable refrigerant that undergoes these phases is complex because chemical, ecological, and thermodynamic properties must be balanced.41,51 ESI Section S3.2† provides criteria for the selection of refrigerants that address safety, carbon footprint, and some thermodynamic properties. In most cases, more than one refrigerant can be used in a technical application. Below, available products and brands are listed that use non-fluorinated gases as refrigerants. The uses and sub-uses are also summarized in Fig. 2. The intention is to show that alternatives are already available and on the market. However, the listings may not be complete, and more energy-efficient, less expensive systems, or systems with higher capacities may exist or are developed.
 |
| Fig. 2 Refrigerant systems and sub-uses. A technical description of all systems is provided in the ESI Section S4.† Pictures were taken from iStock. | |
4.1 Refrigeration
Refrigeration systems remove heat from a refrigerated cabinet or room via the evaporator by transferring the heat to the ambient air outside through the condenser. Air is used as the secondary fluid for both heat exchangers. Various refrigeration systems are on the market. In analogy to the current F-gas Regulation, they are divided here into domestic refrigeration, commercial refrigeration (stand-alone systems and multipack centralized systems), industrial refrigeration, transport refrigeration of goods, and ultra-low and low-temperature freezers. Table 5 gives an overview of the non-fluorinated refrigerants used in the different sub-uses of refrigeration. A more detailed description is provided in the following sub-sections.
Table 5 Overview of the non-fluorinated refrigerants used in the different sub-uses of refrigeration. Others means here e.g., propene, ethane, ethene, helium and/or nitrogen. x: devices with this refrigerant are on the market. X: the majority of the devices that use non-fluorinated refrigerants use this refrigerant

|
CO2 |
Ammonia |
Propane |
Isobutane |
Others |
Domestic refrigeration |
|
|
|
X |
|
Stand-alone refrigeration systems in commercial stores |
x |
x |
X |
x |
x |
Multipack centralized refrigeration systems in commercial stores |
X |
x |
x |
|
|
Industrial refrigeration |
x |
X |
|
|
|
Transport refrigeration of goods |
x |
|
x |
|
x |
Ultra-low and low temperature freezers |
|
x |
x |
|
x |
4.1.1 Domestic refrigeration.
Currently used refrigerants and upcoming regulations.
After January 2015, new domestic refrigerators and freezers in the EU should not contain HFCs with a GWP ≥150.9 An estimated 95% of all fridges in Europe now use iso-butane; nevertheless, some American-style refrigerators supplied to the EU market still operate with hydrofluorocarbons.11,52 Fluorinated greenhouse gases will not be permitted anymore in new domestic refrigerators and freezers from January 2026 on, except if required to meet safety requirements.9
Non-fluorinated alternatives.
The transition to iso-butane has already happened successfully in the EU, and the entire segment will soon apply only non-fluorinated working fluids.
4.1.2 Stand-alone refrigeration systems in commercial stores.
Currently used refrigerants and upcoming regulations.
Historically, these systems have used R-134a and R-404A. Since January 2022, HFCs with a GWP ≥150 are no longer allowed in the EU in refrigerators and freezers for commercial use.9 From January 2025 on, fluorinated greenhouse gases in general with a GWP ≥150 will not be allowed any more in this application.9
Non-fluorinated alternatives.
A number of different non-fluorinated refrigerants are available including hydrocarbons such as ethane, propane, propene or isobutane, but also ammonia and CO2.42 Propane is used most often in stand-alone refrigeration systems in smaller shops and supermarkets.52 EIA (2021)53 lists specific products that are commercially available.
4.1.3 Central refrigeration systems in commercial stores.
Currently used refrigerants and upcoming regulations.
Since January 2022, fluorinated greenhouse gases with a GWP ≥150 that are listed in Annex I of the current F-gas Regulation9 have not been allowed in the EU in multipack centralized refrigeration systems for commercial use with a rated capacity of more than 40 kW. Exempted are gases for the primary refrigerant circuit of cascade systems, where fluorinated greenhouse gases with a GWP of less than 1500 may be used.9 In 2016, the majority of newly installed commercial refrigeration systems in the EU were still based on R-134a, followed by CO2.54
Non-fluorinated alternatives.
(i) Centralized systems: systems using CO2 as refrigerant in transcritical cycles have become a standard technology across Europe.42 In these systems, CO2 is typically applied for direct evaporation, so no extra water or glycol loops are needed. The number of installed CO2 units globally exceeded 35
000 in 2021, 29
000 of them in Europe.52 The systems available today can achieve high annual energy efficiencies even when located in warmer-climate sites; they can be operated even more energy efficient than direct HFC/CO2 cascade and HFC systems.42–44 Also, the leakage rates of transcritical CO2 systems is on the same order as conventional HFC systems.42 The upfront investment costs for transcritical CO2 systems working in warmer climates were higher than for conventional systems in the past.42 However, this has changed and today the total cost of ownership is lower for well-designed transcritical CO2 systems in these regions.55
(ii) Indirect centralized systems: indirect systems include a number of cascading systems where two or more refrigerant cycles are connected in series so that the absorbed heat is transferred from one cycle to another.42 Indirect systems can therefore combine e.g., the use of CO2, glycol or other heat-transferring fluids inside a store to cool the display cabinets and freezers with refrigerants such as hydrocarbons or ammonia in the outer machine room loop (the “primary refrigerant cycle”).42 Systems with ammonia/CO2 cascades are used in some large stores in warmer climates as they yield good energy efficiencies under these conditions.42 Systems with propane and glycol loops have been installed by a number of retailers across Europe for example, in Germany, UK, Belgium and Switzerland.54 However, glycol loops require a lower evaporation temperature in the refrigeration system, as the heat from the cabinets must be absorbed by the glycol mixture and then removed by the refrigeration system. Also, the glycol mixture must be pumped. Compared to a direct evaporation system of e.g., CO2 in the cabinets, the total energy consumption is higher in systems with glycol loops. The additional required temperature difference (5 K) and the demanded superheat (5–10 K) significantly reduces the suction pressure of the indirect units. However, the temperature requirements inside the display cabinets are different across Europe; in some countries 8 °C is acceptable, while others require at maximum 4 °C. This has a significant impact on the technology to be utilized. Manufacturers that offer those systems in Europe include Carel,56 Epta,57 and Freor.58
4.1.4 Industrial refrigeration.
Currently used refrigerants and upcoming regulation.
Ammonia has been widely used for many years in large industrial refrigeration systems.59 In small industrial systems, R-404A and R-134a were also in use.59 However, since January 2020, HFCs with a GWP ≥2500 are not allowed anymore in refrigeration equipment except equipment intended for application designed to cool products to temperatures below –50 °C. The F-gas Regulation will become stricter again for refrigerant equipment (except chillers) in 2025 and 2030, so that fluorinated greenhouse gases with GWPs above 2500 and 150, respectively, will no longer be permitted. Chillers are regulated separately, see below (Section 4.2.4).
Non-fluorinated alternatives.
Since 1850, ammonia refrigeration systems have been the most energy-efficient systems and are therefore applied in large cooling facilities in the food processing industry e.g., in the fish processing industry, in meat processing plants, slaughterhouses in general, breweries and dairies as well as in the process cooling in the chemical industry.52,60,61 Cascade systems using ammonia and CO2 are also widely used on ships and for warehouses and industrial refrigeration.52,62 With this system configuration the refrigerant charge of the ammonia can be significantly reduced and only the CO2 is distributed within the building or process. In cases of CO2 leakages, the cooled and frozen products can be secured, which is an advantage over systems using ammonia only. Systems applying entirely CO2 have also been developed and have proven to be viable.44,63–65 Additional systems with non-fluorinated gases as refrigerants are listed in EIA (2021).53
4.1.5 Transport refrigeration of goods.
Currently used refrigerants and upcoming regulation.
The majority of trucks and trailers today use R-404A.59 R-452A, R-410A and R-143a are also often used as refrigerants in the transport sector.11,66,67 As transport refrigeration does not fall under any of the sectors in Annex IV of the current F-gas Regulation, no specific GWP limits apply. However, the refrigerants fall under the quota system, so there is still some pressure to move to low-GWP alternatives.
Non-fluorinated alternatives.
(i) For trucks, a roof-mounted refrigeration unit has been developed by the German company ECOOLTEC that applies propene and CO2.68 Propene is used inside the active outdoor refrigeration loop, while the air inside the cargo compartment is either heated or cooled by CO2. The cargo-air/CO2 heat exchanger is operated indirectly by the heat pipe principle, whereby CO2 is utilized as the working fluid between the cargo and the refrigeration unit.
(ii) Refrigeration trailers that use CO2 for the industrial, commercial and public sector are offered by Greencold.69
(iii) The Austrian company PBX has tested a propane-based refrigeration unit, called M24, for light commercial electrical vehicles.70
(iv) Container refrigeration systems applying CO2 as working fluid have been on the market since 2010.71 The NaturaLINE® unit was the world's first natural refrigerant container technology. Additional transport refrigeration systems not based on fluorinated gases are available and summarized in Cooltechnologies (2023)62 and EIA (2021).53
4.1.6 Ultra-low and low temperature freezer (temperatures below −20 °C).
Currently used refrigerants and upcoming regulations.
Fluorinated gases are frequently used in ultra-low temperature freezers or cryogenic storage35,72 as well as in centrifuges and test systems.73,74 There is currently no prohibition for the use of HCFs or other fluorinated gases for equipment intended for application designed to cool products to temperatures below −50 °C. This will change from January 2030 on when fluorinated greenhouse gases with a GWP ≥150 are not allowed anymore in refrigerant equipment, except if required to meet safety requirements at the site of operation.
Non-fluorinated alternatives.
For evaporation temperatures down to −45 °C, propene (R-1270) is preferred.52 Some manufacturers also prefer propene for applications with higher temperature levels as it can be used together with smaller and less expensive compressors.52 For low-temperature applications with freezing temperatures down to about −80 °C, ethane can be utilized. When ethane is used in cascade systems with propane, better energy efficiencies are achieved than with systems using R-23 and other HFCs.52 In special cases where the required temperature needs to be below −80 °C, ethene, helium, nitrogen or methane can be applied.52 Available systems on the market include, e.g., ultra-low freezer (−86 °C to −40 °C) from tecnomara that use a cascade system of propane (first level) and a mixture of propane and ethane (second level),75 and ultra-low temperature freezers with propane from Liebherr.76 Other manufacturers that offer ultra-low temperature freezers with hydrocarbons as refrigerants include Meling,77 VWR,78 and Carlo Erba Reagents.79 Cryogenic ultra-low temperature freezers with hydrocarbons are also on the market, e.g., from PHCBI,80 Haiers Biomedical,81 and Linde.82
The Odense University Hospital in Denmark inaugurated a cold-storage room with −80 °C for storing tissue samples in 2012. The refrigeration system is built as an indirect cascade system using ethane and ammonia.83
4.2 Indoor climate
Indoor climate encompasses here heat pumps in building energy systems, district heating networks, air conditioning, and chillers. Table 6 gives an overview of the non-fluorinated refrigerants used in the different sub-uses of indoor climate. A more detailed description is provided in the following sub-sections.
Table 6 Overview of the non-fluorinated refrigerants used in the different sub-uses of indoor climate. “Others” means here e.g., water, air, and/or propene. x: devices with this refrigerant are on the market. X: the majority of the devices that use non-fluorinated refrigerants use this refrigerant

|
CO2 |
Ammonia |
Propane |
Isobutane |
Others |
Heat pumps in building energy systems |
x |
x |
x |
|
x |
District heating network |
x |
x |
|
|
|
Plug-in room air conditioning |
|
|
X |
|
|
Stationary single and multiple split air conditioning |
x |
|
x |
|
|
Mobile air conditioning in vehicles on the ground |
X |
|
x |
|
x |
Mobile air conditioning in aircraft |
x |
|
|
|
x |
Cooling units for data center |
x |
x |
|
|
x |
Chiller |
x |
x |
x |
x |
x |
4.2.1 Heat pumps in building energy systems.
Currently used refrigerants and upcoming regulations.
(i) Air-source heat pumps: 80% of the air–water heat pumps globally sold in 2019 contained R-410A.84 R-134a was the second-most common refrigerant. The other two main refrigerants in use were R-32 and propane.84
(ii) Ground heat pumps: 35% of the ground heat pumps installations in 2018 in Germany contained R-410A, 20% R-407C and another 20% R-32.84 Ground heat pumps with R-134a are not installed anymore, although they had a small market share before 2018. As of 2018, 7% of the installed ground heat pumps contained propane.84
The current version of the F-gas Regulation includes placing on the market prohibitions for various types of heat-pump equipment. Differences are made between self-contained and split units as well as between large (rated capacity between 12 kW and 50 kW) and small (rated capacity below or equal to 12 kW) heat pumps (Fig. 3).
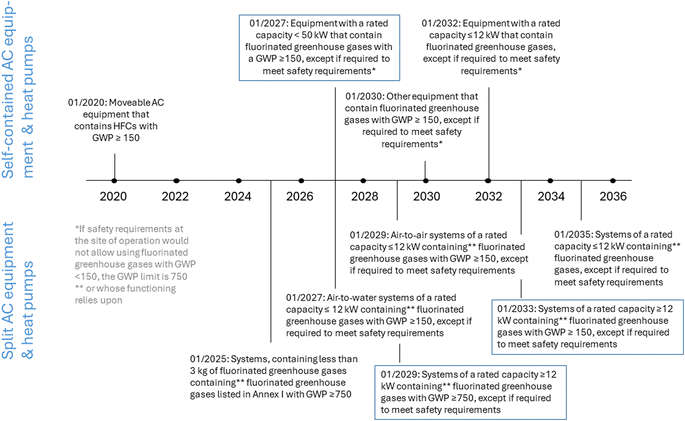 |
| Fig. 3 Placing-on-the-market prohibitions from Annex IV of the F-gas Regulation9 that refer to self-contained air conditioning (AC) equipment and heat pumps, except chillers (upper part), and split AC equipment and heat pumps (lower part). The blue boxes indicate systems with a rated capacity ≥12 kW. | |
The proposed prohibitions will lead – within the next 5 to 10 years – to a decrease of the number of permitted fluorinated refrigerants in the heat pump sector. From the above-mentioned fluorinated refrigerants, only R-1234ze will be permitted if the limit is reduced to a GWP of 150.
Non-fluorinated alternatives.
(i) A variety of air-source heat pumps with non-fluorinated refrigerants are available. Monobloc heat pumps have been developed with propane as refrigerant e.g., by Viessmann, Rhoss, Vaillant, Mitsubishi, Advansor and others.85–89 A split heat pump with a rated capacity of up to 16 kW utilizing propane has just recently been introduced to the market by Haier.90 Larger systems with propane are not (yet) on the market, mainly due to safety concerns as split systems require the refrigerant to be transported inside the building. Suitable measures must be taken to safeguard the technical system. Additionally, when compact heat exchanger technology is employed on the condenser side, the total refrigerant charge can be significantly reduced, and it will also be possible to introduce split heat pumps with higher rated capacities. The Fraunhofer Institute for Solar Energy Systems in Germany is for example already working on developing low-charge propane heat pumps for multi-family housing.91
Split heat pumps have also been developed with CO2 and are widely in use in industrial applications as well as in residential water heaters in countries such as Japan and Australia.92 CO2 has become the new norm for domestic hot-water heat pumps in Japan, where more than 5 million heat pumps with CO2 are already installed to produce hot water for apartments.52 The Swiss army also applies CO2 heat pumps for facility heating and the production of hot tap water in a training hall.93 Stiebel Eltron offers also an air–air heat pump with CO2 as refrigerant.94 In countries where the required hot-water storage temperature is above 70 °C, CO2 heat pumps are the preferred option, as water supply temperatures above 80 °C can be achieved without any auxiliary device.
(ii) Ground-source heat pumps with non-fluorinated refrigerants are also available. Examples are the “ecoGEO 1-6 PRO” heat pump from Ecoforest,95 the sole-water heat pump from HEIM AG or the SNTM3-10 heat pump from Heliotherm,96 which all use propane as refrigerant. Additional providers are HAUTEC,97 or Secon.98 Systems with CO2, ammonia, and water are also available.99,100
(iii) Water-source heat pumps with non-fluorinated refrigerants are also available. For example, the Norwegian heat pump manufacturer Tequs has launched a CO2 water-to-water heat pump for the European market just recently that can provide domestic hot water, space heating and cooling for a variety of applications.101 Enerblue also offers a water-source heat pump with a rated capacity of up to 87 kW with propane as refrigerant.102
4.2.2 District heating network.
Currently used refrigerants and upcoming regulations.
R-134a was present in more than 90% of the installed capacity in the EU28, Norway and Switzerland in 2017.103 Other district heating networks use R-152a, R-245fa, R-1234ze or non-fluorinated refrigerants.93,103
Non-fluorinated alternatives.
Heat pumps with CO2 have been installed in district heating networks in Denmark.52 Those heat pumps are particularly appropriate where the district heating is relatively well developed and also where the district heating temperatures (both supply and return) are relatively low (around 40 °C).52 Systems are offered for example by FENAGY.104 Ammonia is used as working fluid in heat pumps for district heating systems up to a supply temperature of about 90 °C.52,103 Examples are district heating networks in Lemgo in Germany,105 Malmö in Sweden,106 and Lausanne, Aarau, and Rheinfelden Mitte in Switzerland.93
4.2.3 Air conditioning.
Currently only a few percent of European residential and commercial buildings have air conditioning systems installed. However, the annual sales in 2016 were around 12 million units and thus still represent an important market. Due to increasing demands on air quality and thermal comfort, the number of buildings with air conditioning will continue to rise.107
Plug-in room air-conditioning.
Currently used refrigerants and upcoming regulations.
Since January 2020, the EU has allowed only HFCs in movable room air-conditioning equipment if their GWP is below 150.6 Therefore, mainly systems with propane are currently entering the market. From January 2032 onwards, fluorinated greenhouse gases will no longer be allowed in self-contained air-conditioning equipment (Fig. 3).
Non-fluorinated alternatives.
Propane has already been frequently used in moveable air conditioners by Asian and European equipment producers for a number of years and is widely available on the European market.108
Stationary single and multiple split air conditioning.
Currently used refrigerants and upcoming regulations.
In single-split systems with less than 3 kg of refrigerant, R-32 has mostly replaced R-410A.108 R-410A has a GWP of 2088 and will therefore no longer be allowed in the EU in single-split air conditioning systems with less than 3 kg of fluorinated greenhouse gases from January 2025 on, while R-32 will still be allowed.9 However, the use of fluorinated greenhouse gases with GWP ≥150 will be prohibited in systems with a rated capacity up to and including 12 kW from January 2027 (split air-to-water), January 2029 (split air-to-air) and January 2035 on (split systems in general) except if required to meet safety requirements at the site of operation (Fig. 3).9 Thus, R-32 will also not be permitted in those systems in the future.
R-410A is still the dominant refrigerant for larger air conditioning systems placed on the market in the EU today.11,108 However, for split systems with a rated capacity above 12 kW, fluorinated greenhouse gases with a GWP ≥750 will be prohibited from January 2029 on, and fluorinated greenhouse gases with a GWP ≥150 from January 2033 on. A changeover is therefore also necessary in this market segment, which should also extend to the use of alternative systems.
Non-fluorinated alternatives.
Since 2012, propane has been used in commercially-available split air conditioners with cooling capacities up to 7 kW by some Chinese and Indian manufacturers.108,109 China reportedly produced 160
000 split-type room air conditioners with propane until 2019.110 Single-split air conditioners with propane are now also available in Europe, e.g., by ElectrIQ,109 or Midea.111 It has been demonstrated that the energy efficiency and cooling capacity of split units with propane compares favorably to fluorinated refrigerants, including in warm climates.108,112 As refrigerant-carrying pipes have to be routed through the rooms in a building, special safety measures are required that may not be implemented with reasonable technical effort. Alternative solutions that can meet the same requirements must therefore be considered.
A possible replacement for these systems are hydraulic systems that are connected to a refrigeration system. The heat can then be removed via chilled ceilings, cooling sails, fan coils or induction systems.53 Large propane chillers for industrial applications are readily available in Europe although their energy demand is somewhat higher than the demand of air conditioning systems.35 Chillers using ammonia, CO2 or other non-fluorinated refrigerants are also available (see Section 4.2.4). Chillers can also be used for district cooling, as done for example in Copenhagen.113 Across Europe less than 1% of cooling units are district cooling units; however, the market share in Sweden is at about 25%.114
Additional commercial and industrial air conditioning innovations are described in EIA (2021).53
Mobile air conditioning in vehicles on the ground.
Currently used refrigerants and upcoming regulations.
The EU Directive 2006/40/EC on mobile air-conditioning systems (MAC Directive) has prohibited the use of R-134a in new passenger cars and light commercial vehicles since 2017. The main substitute for mobile air conditioning in passenger cars and light commercial vehicles is R-1234yf.
For mobile air-conditioning systems in buses, commercial vehicles of weight classes N3, N2 and partly N1, such as agricultural machinery, ships, rail vehicles, medium-sized aircraft and helicopters, a phase-down of HFCs is not yet required by law. However, the HFCs used in the mobile air-conditioning systems fall under the quota system of the current F-gas Regulation.9 The most common used refrigerants in buses and trains for air conditioning systems are R-134a and R-407C.
Non-fluorinated alternatives.
(i) CO2 is increasingly applied in fully electrified vehicles due to its good performance when used in reversible heat pumps.115,116 One advantage compared to R-1234yf is that CO2 heat pumps supply more heat at temperatures below −5 °C than systems with R-1234yf, where electric heating is needed below −5 °C. CO2 is currently also used by some car manufacturers for cars with combustion engines e.g., by Mercedes and VW.117,118
(ii) CO2 is also used by several bus manufacturers. As an example, Konvekta has supplied CO2 air conditioning systems since 2008.119 Other CO2 air conditioning systems are available from Daimler, Panasonic, Bitzer, Valeo, Bock120 and Solaris.121 A roof heat pump system based on propane is available from HeaVac-Aurora.120
(iii) CO2 has also been tested for long-distance passenger trains and a recent project showed an energy efficiency improvement compared to the conventional system of 30%.122 Elbel (2022)123 demonstrated how to implement a CO2 air conditioning system for high-speed trains. A fully functional prototype CO2 unit was built for demonstration and evaluation. The energy efficiency and cooling capacity were 16% and 14% higher, respectively, compared to the baseline R-407C system.
(iv) Another alternative for passenger trains is air-conditioning with an air-cycle system.124 A recent project conducted in Germany over 2 years on an Intercity Express 3 (ICE3) in standard passenger service provided average savings of 28% in the primary annual energy consumption compared to a systems using R-134a.124 Additionally, the company Wabtec developed and implemented a propane-based air conditioning system including a safety concept for passenger trains.125
Mobile air conditioning in aircraft.
Currently used refrigerants.
Air-cycle air conditioning systems use air as working fluid. The most commonly used refrigerant in vapor-compression cycle systems is R-134a.126,127
Non-fluorinated alternatives.
Efforts have been made to substitute R-134a with CO2 as refrigerant.126 In such systems, CO2 is operated in a transcritical cycle.128
Cooling units for data center.
Currently used refrigerants and upcoming regulation.
HFCs, including R-134a and R-410A, and HFOs are widely used for data center cooling.129
Non-fluorinated alternatives.
CO2-based cooling is becoming more common in data centers.130 An example is a data center in Valencia, Spain, that provides cooling capacity for the servers and the data center's air-conditioning. Waste heat produced by the data center's cooling equipment is recovered and sold to the local district heating system for use in nearby buildings.131 There are also data centers that use ammonia chillers, for example the one in Ostermiething, Austria.132 Chillers that use water as refrigerant have also been developed for data centers by the company Efficient Energy.133 The patents and technology of Efficient Energy are now owned by Vertiv.134
4.2.4 Chillers.
Currently used refrigerants and upcoming regulations.
For low-pressure refrigerant chillers, R-123 was used in the past as well as R-245fa.135 More recently, R-1233zd and R-514A have been used.135 In medium-pressure chillers, R-134a has dominated since it was introduced in the early 1990s. R-513A and R-1234ze are also in use.135 R-410A has been mainly used in the past in high-pressure chillers.135 Alternatives in use are R-454B and R-32. Systems with propane and ammonia are also on the market.
The current and upcoming prohibitions in the F-gas Regulation for chillers are shown in Fig. 4.
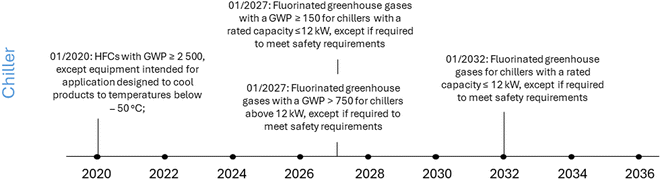 |
| Fig. 4 Placing-on-the-market prohibitions from Annex IV of the F-gas Regulation9 that refer to chillers. | |
Non-fluorinated alternatives.
Enex offers air-cooled and water-cooled CO2 transcritical chillers for the cooling of water and brine mixtures, suitable for process cooling, cold storage, heating, ventilation and air conditioning (HVAC), ice rinks and wineries.136 A large number of manufacturers offer also chillers based on ammonia, isobutane, propane, propene, or water as refrigerants.137,138
4.3 Miscellaneous.
There are some additional refrigerant systems that we discuss here, including heat pumps in industrial energy systems, heat pump tumble dryers, refrigerant air dryers and mobile dehumidifiers, and ice rinks. Table 7 gives an overview of the non-fluorinated refrigerants used in these applications. A more detailed description is provided in the following sub-sections.
Table 7 Overview of the non-fluorinated refrigerants used in the different uses collected as miscellaneous. “Others” means here e.g., butane. x: devices with this refrigerant are on the market. X: the majority of the devices that use non-fluorinated refrigerants use this refrigerant
|
CO2 |
Ammonia |
Propane |
Isobutane |
Others |
Heat pumps in industrial energy systems |
x |
x |
x |
|
x |
Heat pump tumble dryers (HPTD) |
|
|
X |
|
|
Air dryers and mobile dehumidifiers |
|
|
X |
|
|
Ice rinks |
x |
x |
|
|
|
4.3.1 Heat pumps in industrial energy systems.
Currently used refrigerants and upcoming regulations.
Industrial heat pumps in Switzerland use various refrigerants including R-134a, R-1234ze, R-245fa, R-410A, ammonia and CO2.93 R-410A and CO2 were the most often used refrigerants in industrial heat pumps in Japan as of 2020, followed by R-134a and other fluorinated refrigerants.39 For the EU, the prohibitions for placing on the market that have been described above for heat pumps in building energy systems also apply for heat pumps in industrial energy systems. This means that for self-contained heat pumps no fluorinated greenhouse gases will be permitted from January 2030 onwards. For split systems with a rated capacity above 12 kW, fluorinated greenhouse gases with a GWP ≥750 will be prohibited from January 2029 onwards, and fluorinated greenhouse gases with a GWP ≥150 from January 2033 onwards. This means that a system shift is also required here.
Non-fluorinated alternatives.
A report from the Swiss Federal Office of Energy describes the use of four industrial heat pumps that use ammonia as refrigerant and one that uses CO2 as refrigerant.93 All five heat pumps have a capacity between 480 and 1000 kW. Additional examples for the use of ammonia and CO2 are given in IEA (2020)39 and on the IEA website under “Case studies”.139 A recent example is also the use of ammonia as refrigerant in a heat pump to reduce energy consumption from steam generation.140 A high-temperature heat pump unit with propane and butane has been developed and manufactured by a local vendor in Norway. It is implemented in the process plant and the novel heat pump simultaneously produces ice-water and process-heat for a Norwegian dairy.141
4.3.2 Heat pump tumble dryers (HPTD).
Currently used refrigerants and upcoming regulations.
The most commonly used refrigerants in HPTDs globally are R-134a, R-407C, and R-410A. Some transition to propane has happened in EU parties.59 Refrigerants in HPTD are not regulated in the EU so far.
Non-fluorinated alternatives.
Several European manufacturers of HPTD apply propane as the main working fluid in their latest models.142
4.3.3 Refrigerant air dryers and mobile dehumidifiers.
Currently used refrigerants and upcoming regulations.
Many refrigerant air dryers use R-410a, however air dryers with propane are also available. After January 2024, new refrigerant air dryers for laundry quantities of up to 20 kg should not contain refrigerants with a GWP ≥1800 in Switzerland.143 Mobile dehumidifiers with maximum dehumidification rates up to 30 liters in 24 hours should not contain fluorinated refrigerants in Switzerland from January 2024 on.143 There are no product-specific regulations in the EU.
Non-fluorinated alternatives.
A refrigerant air dryer with propane is offered inter alia by Lübra.144 Mobile dehumidifiers with propane are offered by various brands in the EU.145,146
4.3.4 Ice rinks.
Currently used refrigerants and upcoming regulations.
Historically, more than 95% of the ice rinks in Europe have used ammonia; very few rinks used fluorinated refrigerants.147 There are now also 80 rinks that utilize CO2, mostly in Northern Europe and some in Switzerland.147 About three-quarters use indirect CO2 systems where CO2 is used in the machine room along with a secondary refrigerant. The other quarter uses CO2 as primary refrigerant.147 However, half of the ice rinks in the US use a fluorinated gas, mostly R-22.147 Refrigerants in ice rinks are not yet regulated in the EU.
Non-fluorinated alternatives.
Ammonia is one of the most common refrigerants used in ice rinks in North America147 and according to a Canadian study, it shows superior energy performance compared to a R-513A system.147 Transcritical CO2 systems have also been used and have the advantage that surplus heat can be recovered simply and used for heating applications.147,148 Direct expansion of the CO2 underneath the ice is also applied, especially in rinks focusing to achieve the most uniform ice surface temperature at the highest possible evaporation temperature. Such uniform ice temperature is not achievable with secondary loop systems based on sensible heat with a certain temperature difference across the individual loops.149 It has also been stated that CO2 is more energy-efficient than fluorinated gases if the whole year (and not just the hottest hours of the year) is considered.150
5 Discussion
5.1 Importance of the transition to non-fluorinated refrigerants
Refrigeration, air conditioning, heating, and other uses of heat-transfer fluids make up 75% of all uses by mass of F-gases in the EU (Fig. 5a),10 and a significant increase in the demand for refrigerants is expected due to the heat pump boom and increased comfort requirements for air conditioning in the next years.151 The use categories investigated above are therefore important areas for reducing the use of F-gases. This is significant for two reasons: (i) F-gases represented 2.5% of the total EU greenhouse-gas emissions in 2023 (ref. 9) and reducing their direct emissions is an important element in efforts to limit global warming152,153 However, it is also important to note that these emissions are lower than the indirect CO2 emissions that originate from the energy consumption during the operation of the refrigerant systems. Replacing the fluorinated refrigerants with non-fluorinated refrigerants that are at least as efficient as the fluorinated ones is therefore indispensable.
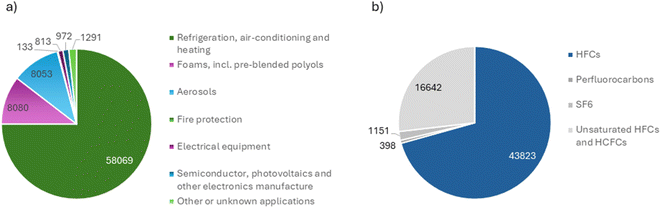 |
| Fig. 5 (a) Intended applications of EU total supply of fluorinated gases in tonnages for 2022. (b) EU bulk supply of fluorinated gases in tonnes for 2022. Data are from European Environment Agency (2020)10 Table 19 and 17, respectively. | |
(ii) Certain fluorinated gases form TFA. 70% of the EU bulk supply of fluorinated gases (Fig. 5b) and 90% of the fluorinated gas emissions in the EU are HFCs10 and many of the HFCs are included in Annex I of the current F-gas Regulation and subject to the quota system. However, unsaturated HFCs and HCFCs such as R-1234yf (GWP 0.5) or R-1234ze (GWP 1.37) are not really affected by the quota system and are used as alternatives to HFCs with a higher GWP. Reducing their emissions is also important in the overall effort to limit sources of TFA.
In addition, atmospheric abundances and emissions of five CFCs (CFC-13, -112a, -113a, -114a and -115) increased between 2010 and 2020.154 These five CFCs are allowed under the Montreal Protocol in the production of other chemicals. The estimated 2020 emissions of these five CFCs are equivalent to 47 ± 5 Tg CO2 equivalent per year, an amount which has the same impact on the climate as the 2020 CO2 emissions of Switzerland.155 Emissions of CFC-113a and CFC-115 have been linked to the production of R-125
156–159 while CFC-113a and CFC-114a are intermediates in a production pathway of R-134a. Replacing R-134a and R-125 with non-fluorinated refrigerants in air conditioners and other refrigerant applications would therefore very likely also reduce these highly problematic CFC emissions.
5.2 Availability of alternatives
Section 4 has shown that non-fluorinated refrigerants are available for all but a few refrigerant applications. Water-based solutions can also be used for the wide range of applications for split appliances. A transition away from fluorinated refrigerants may require some time but is certainly possible. Academic scientists working on heat pump equipment stated in 2023 that a transition time of 3–8 years to use propane for indoor heat pumps (which is currently one of the applications where the use of propane is still challenging) seems to be realistic, depending on the different applications and capacity ranges.160 It is therefore important to make an early announcement of clear and ambitious phase-out dates of fluorinated gases used as refrigerants, and to take different development time spans for different product classes and application areas into account. While safety measures for extended applications of flammable refrigerants are gaining in importance, the development time spans have to be reasonably defined so that products come to the market that ensure less harmful impact on the environment as well as efficient and safe operation.
Looking at the proposed derogation in the PFAS restriction proposal (Table 1), we argue that the proposed derogations for (1) refrigerants in transport refrigeration other than in marine applications and (2) refrigerants in low temperature refrigeration below −50 °C could be made more specific because alternatives for many of the sub-uses are already on the market. Refrigerants for mobile air conditioning systems in combustion-engine vehicles with mechanical compressors are also available. However, since new vehicles with combustion engines will no longer be allowed in the EU from 2035 on (except if they run with e-fuels), a derogation of 6.5 years (or even 13.5 years) seems acceptable (given that the PFAS restriction will likely not enter into force before 2026).
5.3 Economic considerations
Systems are already on the market where the use of non-fluorinated refrigerants is overall more cost-effective than the use of fluorinated refrigerants. For example, it has been shown that the life-cycle costs of an ice rink including initial capital costs, maintenance costs, operating costs, and the asset's residual value at the end of its life are lower when non-fluorinated refrigerants are used compared to fluorinated refrigerants.150
However, other systems that use non-fluorinated refrigerants might be more expensive than systems with fluorinated refrigerants.161 There are two main points that are important to consider here. First, market prices will decrease if the number of produced units increases. So, it can be expected that systems with non-fluorinated refrigerants will become cheaper if their market share increases.162 Secondly, it might be (substantially) cheaper for society to find alternatives to fluorinated gases than to pay the costs of cleaning up the drinking water and dealing with health effects due to the exposure to high levels of persistent fluorinated substances. A study from Minnesota in the United States showed that costs to remove PFASs from waste water are between $14 and $28 billion over 20 years in Minnesota alone.163,164 It is also important that the fluorinated refrigerants will be replaced with non-fluorinated refrigerants that operate at the same or even higher efficiency as the operation costs can easily exceed the investment costs. Also, one environmental problem should not be solved at the expense of another, so having efficient systems will also help to limit global warming. The safety concept for flammable refrigerants should also be critically examined with regard to the areas of application. Specifically, the normative specification of sufficiently safe concepts for different performance classes should be revised.165 If the revision is done in a sensible way, lower costs for these solutions can also be expected.
5.4 Barriers posed by codes and standards
The 2016 report from the European Commission on barriers posed by codes, standards and legislation to using climate-friendly technologies in the refrigeration, air conditioning, heat pump and foam sectors found that standards at the international, European, and national level are an important barrier for the use of hydrocarbons.166 The most relevant European standards for refrigeration, air conditioning, and heat pumps are EN 378, the product standard IEC EN 60335-2-40 for air-conditioning systems, and the product standard IEC EN 60335-2-89 for integral and remote commercial refrigeration applications.167 The product standards take precedence over EN 378 if they are implemented in a national regulation. The revision of EN 378 in 2017 introduced a new flammability category (2L) that allows higher maximum charges for HFCs and HFOs and permits the use of these substances in a larger range of applications and locations.166 However, hydrocarbons do not fall under category 2L and, thus, the charge size below which no additional protective measures are required is still very small, in particular for use in air conditioning and geothermal heat pumps.166 EN 378 is currently again subject to review. The focus of the new revision is the broader use of flammable refrigerants, particularly for equipment not explicitly covered by product standards. However, the process is not expected to be completed before the end of 2024.168
The product standard IEC EN 60335-2-89 was updated in 2019 and the charge limits for flammable (A3) refrigerants such as propane were increased from 150 g to 500 g in self-contained commercial refrigeration cabinets. The charge limits for low-flammable refrigerants (A2 and A2L) were increased to 1200 g.169
Work on the product standard IEC EN 60335-2-40 that is relevant for split air conditioning and heat-pump systems has also been undertaken. The revised standard allows for using a larger charge of flammable refrigerants (up to 988 g of propane in a standard split air conditioning system) in new equipment designed according to certain additional safety requirements to ensure the same high level of safety as equipment using non-flammable refrigerants.170 However, the standard still needs to be adopted in the individual countries.
6 Conclusion
A transition to non-fluorinated refrigerants is possible and has happened in many sectors already. Technically, there are only slight barriers to overcome to replace fluorinated refrigerants in almost all newly developed systems conforming to existing standards. In some cases, trade-offs must be accepted in terms of initial costs, operating pressure, and/or capacity; however, compared to the improved environmental compatibility of the non-fluorinated alternatives, these trade-offs are worth making and have a reasonable payback due to reduced operational expenses. No tradeoffs should be accepted in terms of efficiency, as the efficiency will not only affect the costs but also the contribution of the refrigerant systems to global warming.
Given all the cases where non-fluorinated refrigerants are already in use in refrigeration and heat pumping applications, and given their similar or even higher efficiency, we argue that it is justifiable to have very few (and not more than currently proposed) derogations for fluorinated gases in the EU PFAS restriction.
The transition to non-fluorinated working fluids is also a great opportunity for European companies to be frontrunners; it will create increasing product demand within the heating and cooling sector. Many jobs will remain within the chemical companies, as they will continue to serve and manage the aftermarket for the next decades.
Data availability
No primary research results, software or code are included and no new data were generated or analyzed as part of this review.
Author contributions
JG: conceptualization, methodology, investigation, writing – original draft, AH: investigation, writing – original draft; KB, CV, RL & ITC: conceptualization, investigation, writing – review & editing; DM: writing – review & editing; GG: funding acquisition, writing – review & editing, MS: funding acquisition, supervision, writing – review & editing.
Conflicts of interest
There are no conflicts of interest to declare.
Acknowledgements
We thank Henry Wöhrnschimmel from the Swiss Federal Office for the Environment for his comments on the manuscript. JG acknowledges funding from the Tides Foundation (#TF2101-096992), KB from the German Federal Ministry for Economic Affairs and Climate Action (BMWK) (#03EN1088A) and RL from the US National Institute for Environmental Health Sciences (#P42ES027706). ITC acknowledges funding from the European Union's Horizon 2020 research and innovation program (grant agreement No. 101036756; the ZeroPM project).
References
- J. C. Farman, B. G. Gardiner and J. D. Shanklin, Large Losses of Total Ozone in Antarctica reveal seasonal ClOx/NOx interaction, Nature, 1985, 315, 207–210, DOI:10.1038/315207a0.
-
R. Falkner, The Business of Ozone Layer Protection: Corporate Power in Regime Evolution, in The Business of Global Environmental Governance, 2004, vol. 1999, pp. 105–134, DOI:10.7551/mitpress/1705.003.0010.
- R. G. Prinn, R. F. Weiss, P. J. Fraser, P. G. Simmonds, D. M. Cunnold, F. N. Alyea, S. O'Doherty, P. Salameh, B. R. Miller, J. Huang, R. H. J. Wang, D. E. Hartley, C. Harth, L. P. Steele, G. Sturrock, P. M. Midgley and A. McCulloch, A History of Chemically and Radiatively Important Gases in Air Deduced from ALE/GAGE/AGAGE, J. Geophys. Res.: Atmos., 2000, 105(D14), 17751–17792, DOI:10.1029/2000JD900141.
-
Australian Goverment, Factsheet - Montreal Protocol, 2018, https://www.dcceew.gov.au/sites/default/files/documents/factsheet-montreal-protocol.pdf.
-
DownToEarth, The Gas Game, 2013, https://www.downtoearth.org.in/coverage/the-gas-game-42172 Search PubMed.
-
European Parliament and the Council of the European Union, Regulation (EU) No 517/2014 of the European Parliament and of the Council of 16 April 2014 on Fluorinated Greenhouse Gases and Repealing Regulation (EC) No 842/2006, Off. J. Eur. Union 2014, 2014, vol. 517, L150/195-230 Search PubMed.
-
UNEP, About Montreal Protocol, https://www.unep.org/ozonaction/who-we-are/about-montreal-protocol.
- European Parliament and the Council of the European Union, Regulation (EC) No 842/2006 of the European Parliament and of the Council of 17 May 2006 on Certain Fluorinated Greenhouse Gases, 2006.
-
European Parliament and the Council of the European Union, Regulation (EU) 2024/573 of the European Parliament and of the Council on on Fluorinated Greenhouse Gases, Amending Directive (EU) 2019/1937 and Repealing Regulation (EU) No 517/2014, Off. J. Eur. Union, 2024, vol. 573, pp. 1–67 Search PubMed.
-
European Environment Agency, Fluorinated Greenhouse Gases 2020, 2020, https://www.eea.europa.eu/publications/fluorinated-greenhouse-gases-2020 Search PubMed.
-
Umweltbundesamt, Persistent Degradation Products of Halogenated Refrigerants and Blowing Agents in the Environment: Type, and Fate with Particular Regard to New Halogenated Substitutes with Low Global Warming Potential (73/2021), 2021, https://www.umweltbundesamt.de/publikationen/persistent-degradation-products-of-halogenated Search PubMed.
-
European Chemicals Agency, Summary of Classification and Labelling - Trifluoroacetic acid, https://echa.europa.eu/de/information-on-chemicals/cl-inventory-database/-/discli/details/47316 Search PubMed.
-
Naturalrefrigerants, German Chemicals Office Plans EU Proposal Linking TFA to Reproductive Toxicity. https://naturalrefrigerants.com/german-chemicals-office-plans-eu-proposal-linking-tfa-to-reproductive-toxicity/.
- Z. Zhai, J. Wu, X. Hu, L. Li, J. Guo, B. Zhang, J. Hu and J. Zhang, A 17-Fold Increase of Trifluoroacetic Acid in Landscape Waters of Beijing, China during the Last Decade, Chemosphere, 2015, 129, 110–117, DOI:10.1016/j.chemosphere.2014.09.033.
- H. M. Pickard, A. S. Criscitiello, D. Persaud, C. Spencer, D. C. G. Muir, I. Lehnherr, M. J. Sharp, A. O. De Silva and C. J. Young, Ice Core Record of Persistent Short-Chain Fluorinated Alkyl Acids: Evidence of the Impact From Global Environmental Regulations, Geophys. Res. Lett., 2020, 47(10) DOI:10.1029/2020GL087535.
- T. M. Cahill, Increases in Trifluoroacetate Concentrations in Surface Waters over Two Decades, Environ. Sci. Technol., 2022, 56(13), 9428–9434, DOI:10.1021/acs.est.2c01826.
- F. Freeling, M. Scheurer, J. Koschorreck, G. Hoffmann, T. A. Ternes and K. Nödler, Levels and Temporal Trends of Trifluoroacetate (TFA) in Archived Plants: Evidence for Increasing Emissions of Gaseous TFA Precursors over the Last Decades, Environ. Sci. Technol. Lett., 2022, 9, 400–405, DOI:10.1021/acs.estlett.2c00164.
-
Umweltbundesamt, Langzeittrends für Trifluoressigsäure in Terrestrischen Umweltproben-Untersuchung von Pflanzenproben der Umweltprobenbank des Bundes (UPB) auf Trifluoressigsäure - Teil, 2 (09/2023), 2023, https://www.umweltbundesamt.de/publikationen/langzeittrends-fuer-trifluoressigsaeure-in-0 Search PubMed.
- S. Joudan, A. O. De Silva and C. J. Young, Insufficient Evidence for the Existence of Natural Trifluoroacetic Acid, Environ. Sci.: Processes Impacts, 2021, 23(11), 1641–1649, 10.1039/d1em00306b.
- O. J. Nielsen, B. F. Scott, C. Spencer, T. J. Wallington and J. C. Ball, Trifluoroacetic Acid in Ancient Freshwater, Atmos. Environ., 2001, 35(16), 2799–2801, DOI:10.1016/S1352-2310(01)00148-0.
- M. Scheurer, K. Nödler, F. Freeling, J. Janda, O. Happel, M. Riegel, U. Müller, F. R. Storck, M. Fleig, F. T. Lange, A. Brunsch and H. J. Brauch, Small, Mobile, Persistent: Trifluoroacetate in the Water Cycle – Overlooked Sources, Pathways, and Consequences for Drinking Water Supply, Water Res., 2017, 126, 460–471, DOI:10.1016/j.watres.2017.09.045.
- Z. Wang, Y. Wang, J. Li, S. Henne, B. Zhang, J. Hu and J. Zhang, Impacts of the Degradation of 2,3,3,3-Tetrafluoropropene into Trifluoroacetic Acid from Its Application in Automobile Air Conditioners in China, the United States, and Europe, Environ. Sci. Technol., 2018, 52(5), 2819–2826, DOI:10.1021/acs.est.7b05960.
- A. Jordan and H. Frank, Trifluoroacetate in the Environment. Evidence for Sources Other than HFC/HCFCs, Environ. Sci. Technol., 1999, 33(4), 522–527, DOI:10.1021/es980674y.
- M. Berg, S. R. Müller, J. Mühlemann, A. Wiedmer and R. P. Schwarzenbach, Concentrations and Mass Fluxes of Chloroacetic Acids and Trifluoroacetic Acid in Rain and Natural Waters in Switzerland, Environ. Sci. Technol., 2000, 34(13), 2675–2683, DOI:10.1021/es990855f.
- S. Meier, P. Brodmann, S. Weber, C. Moschet, D. Kull, T. Gelmi, L. William and J. Steinmann, Trinkwasserqualität bezüglich der PFAS-Rückstände, Aqua Gas, 2024, 3, 22–27 Search PubMed.
- S. Taniyasu, K. Kannan, L. W. Y. Yeung, K. Y. Kwok, P. K. S. Lam and N. Yamashita, Analysis of Trifluoroacetic Acid and Other Short-Chain Perfluorinated Acids (C2–C4) in Precipitation by Liquid Chromatography–Tandem Mass Spectrometry: Comparison to Patterns of Long-Chain Perfluorinated Acids (C5–C18), Anal. Chim. Acta, 2008, 619(2), 221–230, DOI:10.1016/j.aca.2008.04.064.
- Q. Wang, X. Wang and X. Ding, Rainwater Trifluoroacetic Acid (TFA) in Guangzhou, South China: Levels, Wet Deposition Fluxes and Source Implication, Sci. Total Environ., 2014, 468–469, 272–279, DOI:10.1016/j.scitotenv.2013.08.055.
- C. E. Wujcik, D. Zehavi and J. N. Seiber, Trifluoroacetic Acid Levels in 1994–1996 Fog, Rain, Snow and Surface Waters from California and Nevada, Chemosphere, 1998, 36(6), 1233–1245, DOI:10.1016/S0045-6535(97)10044-3.
- J. Kazil, S. McKeen, S. W. Kim, R. Ahmadov, G. A. Grell, R. K. Talukdar and A. R. Ravishankara, Deposition and Rainwater Concentrations of Trifluoroacetic Acid in the United States from the Use of Hfo-1234yf, J. Geophys. Res., 2014, 119(22), 14059–14079, DOI:10.1002/2014JD022058.
- L. M. von Sydow, A. B. Grimvall, H. B. Borén, K. Laniewski and A. T. Nielsen, Natural Background Levels of Trifluoroacetate in Rain and Snow, Environ. Sci. Technol., 2000, 34(15), 3115–3118, DOI:10.1021/es9913683.
- M. Sadia, I. Nollen, R. Helmus, T. L. Ter Laak, F. Béen, A. Praetorius and A. P. Van Wezel, Occurrence, Fate, and Related Health Risks of PFAS in Raw and Produced Drinking Water, Environ. Sci. Technol., 2023, 57(8), 3062–3074, DOI:10.1021/acs.est.2c06015.
- F. Freeling, D. Behringer, F. Heydel, M. Scheurer, T. A. Ternes and K. Nödler, Trifluoroacetate in Precipitation: Deriving a Benchmark Data Set, Environ. Sci. Technol., 2020, 54(18), 11210–11219, DOI:10.1021/acs.est.0c02910.
-
Umweltbundesamt, Trifluoressigsäure – Gewässerschutz im Spannungsfeld von Toxikologischem Leitwert, Trinkwasserhygiene und Eintragsminimierung, 2020, https://www.umweltbundesamt.de/sites/default/files/medien/362/dokumente/2020_10_20_uba_einordnung_tfa_leitwert.pdf Search PubMed.
- H. P. H. Arp, A. Gredelj, J. Glüge, M. Scheringer and I. T. Cousins, Preprint: The Global Threat from the Irreversible Accumulation of Trifluoroacetic Acid (TFA), ChemRxiv, 2024 DOI:10.26434/chemrxiv-2024-0djqt-v2.
-
BAuA RIVM, NEA and Danish EPA, Annex XV restriction report - Proposal for a restriction of Per- and polyfluoroalkyl substances (PFASs), https://echa.europa.eu/restrictions-under-consideration/-/substance-rev/72301/term.
-
Chemours, Protecting Fluoropolymers and Fluorinated Gases, the Building Blocks of our Future, https://www.chemours.com/en/pfas-advocacy Search PubMed.
-
UNEP, Refrigerant Management, https://www.unep.org/ozonaction/refrigerant-management-0.
-
UNEP, Promoting Low-GWP Refrigerants for Air-Conditioning Sectors in High-Ambient Temperature Countries (PRAHA), 2016, https://www.unep.org/resources/report/promoting-low-gwp-refrigerants-air-conditioning-sectors-high-ambient-temperature Search PubMed.
-
International Energy Agency (IEA), Annex 48: Industrial Heat Pumps, Second Phase, 2020, https://heatpumpingtechnologies.org/publications/final-report-annex-48-industrial-heat-pumps-second-phase/ Search PubMed.
-
C. Höges, L. Wissing, C. Vering and D. Müller, How to Choose the Best Refrigerant in Heat Pumps from an Ecologic Perspective? Analyzing the Influence of the Evaluation Method, 36th Int. Conf. Effic. Cost, Optim. Simul. Environ. Impact Energy Systems, ECOS, 2023, vol. 2023, pp.725–737, DOI:10.52202/069564-0066.
-
C. Vering, C. Zibunas, F. Tessarek, N. Moschner, K. Breuer, C. Höges, H. Romberg, N. V. D. Aßen and D. Müller, Towards Integral Assessment of Heat Pumps and Refrigerants Using LCA: A Case Study for the German Building Stock, in Heat Pump Conference, 2023, pp. 1–12, https://www.hpc2023.org/wp-content/uploads/gravity_forms/3-7075ba8a16c5f78b321724d090fb2a34/2023/05/<?pdb_no 0311?>0311<?pdb END?>_HPC2023_Full_Paper_Vering_v03.pdf Search PubMed.
-
European Commission, Report from the Commission Assessing the 2022 Requirement to Avoid Highly Global Warming Hydrofluorocarbons in Some Commercial Refrigeration Systems, 2017, https://climate.ec.europa.eu/system/files/2017-08/c_2017_5230_en.pdf Search PubMed.
-
Naturalrefrigerants, ATMO Europe: Integrated HVAC&R CO2 System Cuts Swiss Supermarket's Energy Use by 35%, https://naturalrefrigerants.com/atmo-europe-integrated-hvacr-co2-system-cuts-swiss-supermarkets-energy-use-by-35/.
-
Naturalrefrigerants, ATMO LATAM: Güntner Finds Transcritical CO2 to be up to 18.6% More Efficient Than R404A in Monterrey, Mexico, https://naturalrefrigerants.com/atmo-latam-guntner-finds-transcritical-co2-to-be-up-to-18-6-more-efficient-than-r404a-in-monterrey-mexico/.
-
IPCC, The Earth's Energy Budget, Climate Feedbacks, and Climate Sensitivity Supplementary Material, in Climate Change 2021: the Physical Science Basis. Contribution of Working Group I to the Sixth Assessment Report of the Intergovernmental Panel on Climate Chan, 2021, https://www.ipcc.ch/report/ar6/wg1/downloads/report/IPCC_AR6_WGI_FullReport_small.pdf Search PubMed.
- K. Harby, Hydrocarbons and Their Mixtures as Alternatives to Environmental Unfriendly Halogenated Refrigerants: An Updated Overview, Renewable Sustainable Energy Rev., 2017, 73, 1247–1264, DOI:10.1016/j.rser.2017.02.039.
-
F. F. Lachner, G. F. Nellis and D. T. Reindl, An Investigation into the Feasibility of the Use of Water as a Refrigerant, in International Refrigeration and Air Conditioning Conference, 2004, https://core.ac.uk/download/pdf/4955215.pdf Search PubMed.
- B. Hu, D. Wu and R. Z. Wang, Water Vapor Compression and Its Various Applications, Renewable Sustainable Energy Rev., 2018, 98(September), 92–107, DOI:10.1016/j.rser.2018.08.050.
-
Linde, Safety Data Sheet: Helium, Compressed, 2022, https://produkte.linde-gas.at/sdb_konform/HE_10021690EN.pdf Search PubMed.
-
MineARC, Nitrogen: Hazard in Focus, https://minearc.com/nitrogen-hazard-in-focus/.
-
C. Vering, C. Höges and D. Müller, Who Wants to Be a Millionfold Deployed Refrigerant?, HPT Mag., 2022, vol. 40, iss. 1, https://heatpumpingtechnologies.org/publications/who-wants-to-be-a-millionfold-deployed-refrigerant/ Search PubMed.
-
A. Hafner and R. Ciconkov, Current State and Market Trends in Technologies with Natural Refrigerants, in International Institute of Refrigeration, 2021, pp. 281–289, DOI:10.18462/iir.nh3-co2.2021.0035.
-
Environmental Investigation Agency UK (EIA), Pathway to Net-Zero -Cooling Product List, 2021, https://static.us.eia.org/pdfs/2021-Pathway-to-Net-Zero-Cooling-Product-List-EIA.pdf Search PubMed.
-
Öko-Recherche, Availability of Alternatives to HFCs in Commercial Refrigeration in the EU, 2016, https://climate.ec.europa.eu/system/files/2016-12/20161201_briefing_supermarket_en.pdf.
-
Á. Á. Pardiñas, F. Fabris, L. Contiero, H. Selvnes, K. Banasiak and A. Hafner, Ejector for the World: Simplified Ejector-Supported CO2 Refrigeration Systems for All Climates, in 15th IIR-Gustav Lorentzen Conference on Natural Refrigerants, 2022, https://sintef.brage.unit.no/sintef-xmlui/handle/11250/3029801 Search PubMed.
-
Carel, Heos sistema, https://www.carel.com/heos-sistema.
-
Cooltechnologies, GranVista Integral Waterloop - Epta, https://cooltechnologies.org/zero/granvista-integral-waterloop/.
-
Freor, Hydroloop System, https://freor.com/hydroloop-system/.
-
UNEP, Montreal Protocol on Substances That Deplete the Ozone Layer - Report of the Technology and Economic Assessment Panel - Decision XXVIII/2 TEAP Working Group Report Information on Alternatives to HFCs, 2022, https://ozone.unep.org/system/files/documents/TEAP-Decision-XXVIII-2-HFC-%20Alternatives-report-sept2022.pdf Search PubMed.
-
European Commission, Climate-friendly alternatives to HFCs, https://climate.ec.europa.eu/eu-action/fluorinated-greenhouse-gases/climate-friendly-alternatives-hfcs_en Search PubMed.
-
Naturalrefrigerants, Scantec Provides Centralized Low-Charge Ammonia System for Poultry Distribution Warehouse in Western Australia, https://naturalrefrigerants.com/scantec-provides-centralized-low-charge-ammonia-system-for-poultry-distribution-warehouse-in-western-australia/.
-
Cooltechnologies, Transport Refrigeration, https://cooltechnologies.org/sector/transport-refrigeration/.
-
Emerson, CO2 and Ammonia Cross over into Competing Market Spaces, 2017, https://www.copeland.com/documents/e360-article-%E2%80%93-trading-places-en-4205676.pdf Search PubMed.
-
Naturalrefrigerants, Advansor CO2 Rack Supplies Cooling, Freezing, Air-Conditioning and Heating for Maersk Cold Storage Facility in New Zealand, https://naturalrefrigerants.com/advansor-co2-rack-supplies-cooling-freezing-air-conditioning-and-heating-for-maersk-cold-storage-facility-in-new-zealand/.
- E. Söylemez, K. N. Widell, C. H. Gabrielii, Y. Ladam, T. Lund and A. Hafner, Overview of the Development and Status of Carbon Dioxide (R-744) Refrigeration Systems Onboard Fishing Vessels, Int. J. Refrig., 2022, 140, 198–212, DOI:10.1016/j.ijrefrig.2022.05.007.
-
Ecooltec, Sustainable, Efficient, Safe and Practical: ECOOLTEC Revolutionises Transport Refrigeration, 2022, https://ecooltec.com/media/press_release_natural_refrigerant_en-2.pdf Search PubMed.
-
Tecumseh, Richtlinien zur Verwendung von R452A und R448A/R449A und bestehenden gewerblichen Kälteanlagen, 2018, https://www.tecumseh.com/userfiles/documents/guidelines/tecumseh_richtlinien_zur_verwendung_von_r452a_und_r449a_eu_de.pdf Search PubMed.
-
Ecooltec, Highly efficient roof cooling systems, https://ecooltec.com/en/#technik.
-
Greencold, Custom Refrigerated Trailershttps://greencold.co.uk/custom-refrigerated-trailers/.
-
Atmo, ATMO Europe: R290 Unit Enables ‘First’ Refrigerated Electric Vehicle with Zero Carbon Emissions, https://atmo.org/announcement/r744-com-atmo-europe-r290-unit-enables-first-refrigerated-electric-vehicle-with-zero-carbon-emissions/.
- Carrier, Refrigeration https://www.carrier.com/refrigeration/en/worldwide/transport-refrigeration/.
-
ACS, R472A – The new refrigerant gas with the lowest GWP ever, https://www.acstestchambers.com/en/news-and-events/r472a-new-refrigerant-gas-lowest-gwp-ever/.
- European FluoroCarbons Technical Committee (EFCTC), Centrifugal chillers using HFC & HFO refrigerants designed for optimum performance, https://www.fluorocarbons.org/news/centrifugal-chillers-using-hfc-hfo-refrigerants-designed-for-optimum-performance/.
-
Weisstechnik, Stability Test Systems
Pharma Event, https://www.weiss-technik.com/fileadmin/Redakteur/Mediathek/Broschueren/WeissTechnik/Reinluft_und_Containment/weisstechnik-Stabilitaetspruefsysteme-PharmaEvent-EN.pdf.
-
Tecnomara, -86°C Ultra-Low Temperature Freezers, 2023, https://tecnomara.de/ultratiefkuehlschraenke/.
-
Liebherr, Safe Storage at Ultra Low Temperatures, https://home.liebherr.com/en/mdv/apac/commercial/research-and-laboratory/ultralow-freezers/ultralow-freezers.html.
-
Meling, −86°C Ultra Low Temperature Freezer, https://www.melingbiomedical.com/ultra-low-temperature-freezer_c4.
-
VWR, Ultra low temperature freezer, Classic Eco, −86 °C, PHCbi, https://ch.vwr.com/store/product/de/16611628/ultra-low-temperature-freezer-classic-eco-86-c-phcbi.
-
CarloErba, Ultra-Low-Temperature Freezer Salvum series, https://www.carloerbareagents.de/en/products/ultra-low-temperature-freezer/ult-freezer-comfort-86c/.
-
PHCBI, Cryogenic ULT Freezers (-150°C/-152°C), https://www.phchd.com/apac/biomedical/preservation/ultra-low-freezers/Cryogenic-ULT-freezers.
-
HaierBiomedical, Cryo Freezer, https://www.haiermedical.com/product/Cryo-Freezer.html.
-
Linde, CRYOLINE CF, https://www.linde-gas.com/en/products_and_supply/food_freezing/cryoline_cf.html.
-
Johnson Controls, Biggest -80°C Coldstore in Europe, Odense University Hospital, Denmark, 2015, https://www.youtube.com/watch?v=HeS937tCwPw Search PubMed.
-
ATMOsphere, Presentation on “Market for Heat Pumps Based on Natural Refrigerants – Current Status and Projections” at the TRI-HP Final Event, 2023, https://www.rehva.eu/fileadmin/user_upload/EU_Projects/TRI-HP/TRI-HP_Final_event_slide_deck.pdf.
-
Viessmann. Propan-Wärmepumpen: “grünes” Kältemittel für den Klimaschutzhttps://www.viessmann.ch/de/wissen/technik-und-systeme/waermepumpe/propan.html.
-
B. Santos, Vaillant Showcases Propane Heat Pump for Residential Applications, PV Magazine, 2023, https://www.pv-magazine.com/2023/03/27/vaillant-unveils-propane-heat-pump-for-residential-applications/ Search PubMed.
-
B. Santos, New Propane Heat Pump for Space, Water Heating, PV Magazine, 2023, https://www.pv-magazine.com/2023/03/14/new-propane-heat-pump-for-space-water-heating/ Search PubMed.
-
B. Santos, Mitsubishi Unveils Propane Heat Pump for Residential Applications, 2023, https://www.pv-magazine.com/2023/03/16/mitsubishi-unveils-propane-heat-pump-for-residential-applications/.
-
Naturalrefrigerants, Advansor HeatQuantum Plug-and-Play Heat Pump, https://naturalrefrigerants.com/advansor-releases-industrial-plug-and-play-co2-heat-pump/.
-
Haier, New Air-to-Water Heat Pump Hydro Split R290, https://www.haierhvac.eu/en/product/air-water-treatment/air-water-heat-pump-hydro-split-r290#features.
-
Hydrocarbons21, German Research Institute Looks into Low-Charge R290 Heat Pump Systems, Especially Indoors, for Multi-Family Housing, https://hydrocarbons21.com/german-research-institute-looks-into-low-charge-r290-heat-pump-systems-especially-indoors-for-multi-family-housing/.
-
SmallPlanetSupply, SANCO2™ High-Efficiency Heat Pump Water Heater, https://www.smallplanetsupply.com/sanc02.
-
Swiss Federal Office of Energy (SFOE), Industrial Heat Pumps in Switzerland - Application Potentials and Case Studies, 2020, https://www.aramis.admin.ch/Default?DocumentID=66033&Load=true Search PubMed.
-
Stiebel_Eltron, LWZ 604 air, https://www.stiebel-eltron.de/de/home/produkte-loesungen/erneuerbare_energien/lueftung/zentral/lwz-604/lwz-604-air/technische-daten.html#.
-
Ecoforest, Heat pump «ecoGEO 1-6 PRO, https://ecoforest.com/en/products/heat-pumps/ground-source-heat-pumps/bomba-de-calor-ecogeo-pro-1-6/.
-
Heliotherm, Natural Technology Heat Pump SNTM3-10, https://www.heliotherm.co.uk/products/heat-pumps/natural-technology-heat-pump-new/kategorie/natural-technology-heat-pump/.
-
HAUTEC, Carno HCS Premium, https://hautec.eu/sole-wasser-waermepumpe-r290/.
-
Secon, Wärmepumpen (R290/R600a), https://secon-gmbh.com/produkte/waermepumpen-r290r600a/.
- W. Wu and H. M. Skye, Progress in Ground-Source
Heat Pumps Using Natural Refrigerants, Int. J. Refrig., 2018, 92, 70–85, DOI:10.1016/j.ijrefrig.2018.05.028.
-
Enex, Geoheat, https://www.enex.it/geoheat/.
-
Naturalrefrigerants, Tequs Launches New Commercial Water-to-Water CO2 Heat Pump, https://naturalrefrigerants.com/tequs-launches-new-commercial-water-to-water-co2-heat-pump/.
-
Enerblue, W/W Heat Pump with Natural refrigerant, https://enerblue.it/en/natural-refrigerant/steel.
- A. David, B. V. Mathiesen, H. Averfalk, S. Werner and H. Lund, Heat Roadmap Europe: Large-Scale Electric Heat Pumps in District Heating Systems, Energies, 2017, 10(4), 18, DOI:10.3390/en10040578.
-
Fenagy. Heat pumps, https://www.fenagy.dk/en/heat-pumps-h-range.
-
Naturalrefrigerants, District Heating Plant in Historic German City Uses Ammonia Heat Pumps to Generate Heat from Wastewater, https://naturalrefrigerants.com/district-heating-plant-in-historic-german-city-uses-ammonia-heat-pumps-to-generate-heat-from-wastewater/.
-
GEA, E.ON turns sewage into a valuable resource with GEA heat pump technology, https://www.gea.com/es/news/trade-press/2021/gea-heat-pumps-at-eon-malmoe/.
-
International Energy Agency (IEA), The Future of Cooling - Opportunities for Energy- Efficient Air Conditioning, International Energy Agency, 2018, https://iea.blob.core.windows.net/assets/0bb45525-277f-4c9c-8d0c-9c0cb5e7d525/The_Future_of_Cooling.pdf.
- European Commission, Report from the Commission on the Availability of Refrigerants for New Split Air Conditioning Systems That Can Replace Fluorinated Greenhouse Gases or Result in a Lower Climate Impact, 2020, https://climate.ec.europa.eu/system/files/2020-09/c_2020_6637_en.pdf.
-
Cooltechnologies, Net-Zero Domestic Air Conditioning, https://cooltechnologies.org/sector/domestic-air-conditioning/?pt=zero.
-
Hydrocarbons21, Chinese companies report production of nearly 160,000 propane room ACs August 29, 2019 Air conditioning Asia, https://hydrocarbons21.com/chinese-companies-report-production-of-nearly-160000-propane-room-acs/.
-
Hydrocarbons21, Midea R290 Room ACs Now Available for Sale in Europe, https://hydrocarbons21.com/midea-r290-room-acs-now-available-for-sale-in-europe/.
-
Hydrocarbon21, Propane Outperforms R407C in Test of Rooftop AC Units, https://hydrocarbons21.com/propane-outperforms-r407c-in-test-of-rooftop-ac-units/.
-
Alfalaval, Cooling naturally, https://www.alfalaval.com/media/stories/district-cooling/cooling-naturally/.
- J. Callaghan, Out with Air Conditioners, in with Chilled Water – How Europe Could Slash Its Cooling Footprint, Horizon, 2021 Search PubMed , https://projects.research-and-innovation.ec.europa.eu/en/horizon-magazine/out-air-conditioners-chilled-water-how-europe-could-slash-its-cooling-footprint.
-
Evflux, ID3, Heating and Cooling systems, https://evflux.pro/id3-cooling-and-heating/.
-
Audi-MediaCenter, Battery, thermal management, and charging, https://www.audi-mediacenter.com/en/electric-suvs-in-the-premium-compact-segment-the-audi-q4-e-tron-and-the-q4-sportback-e-tron-13887/battery-thermal-management-and-charging-13902.
-
Mercedes, CO2 as a refrigerant is happening, 2021, https://automotivetechinfo.com/wp-content/uploads/2021/06/CO2-as-a-Refrigerant-is-Happening.pdf.
-
Umweltbundesamt, Mobile air conditioning with climate-friendly refrigerant CO2, https://www.umweltbundesamt.de/en/topics/climate-energy/fluorinated-greenhouse-gases-fully-halogenated-cfcs/application-domains-emission-reduction/moble-air-conditioning-in-cars-buses-railway/mobile-air-conditioning-climate-friendly.
-
Konvekta, Konvekta CO2 heat pump variants for electric buses and rail vehicles, https://www.konvekta.de/en/innovation/konvekta-co2-heat-pump.html.
-
Cooltechnologies. Net-Zero Transport Air Conditioning, https://cooltechnologies.org/sector/transport-air-conditioning/?pt=zero.
-
Naturalrefrigerants, German Cities Opting for Hydrogen Buses with CO2 Heat Pumps, https://naturalrefrigerants.com/german-cities-opting-for-hydrogen-buses-with-co2-heat-pumps/.
-
Naturalrefrigerants, Austrian Passenger Train Shows 30% Energy Saving with CO2 Air Conditioning, https://naturalrefrigerants.com/austrian-passenger-train-shows-30-energy-saving-with-co2-air-conditioning/.
-
Naturalrefrigerants, ATMO Europe: Prototype CO2 High-Speed Rail Air Conditioner Is 16% More Efficient Than HFC Unit, 2024, https://naturalrefrigerants.com/atmo-europe-prototype-co2-high-speed-rail-air-conditioner-is-16-more-efficient-than-hfc-unit/.
-
Umweltbundesamt, Environmentally Friendly Air Conditioning for Trains – Field Data Measurement and Analysis on the ICE 3 Air- Cycle System (120/2019), 2019, https://www.umweltbundesamt.de/publikationen/environmentally-friendly-air-conditioning-for Search PubMed.
-
Wabtec, Wabtec Begins Trial of Transit Rail Industry's First Environmentally Friendly Air Conditioning System, https://www.wabteccorp.com/newsroom/press-releases/wabtec-begins-trial-of-transit-rail-industry-s-first-environmentally-friendly-air-conditioning-system.
- M. Merzvinskas, C. Bringhenti, J. T. Tomita and C. R. De Andrade, Air Conditioning Systems for Aeronautical Applications: A Review, Aeronaut. J., 2020, 124(1274), 499–532, DOI:10.1017/aer.2019.159.
-
Aeronautics-Guide, Aircraft Air Conditioning Systems, https://www.aircraftsystemstech.com/2017/05/aircraft-air-conditioning-systems.html.
- A. Cavallini, A. Mannini, P. Asinari and C. Zilio, Carbon Dioxide as a Working Fluid in Aircraft Air-Conditioning: An Experimental Assessment, Proc. IIR Int. Inst. Refrig. Int. Conf., 2005, 1, 327–334 Search PubMed , https://staff.polito.it/pietro.asinari/proceedings/Asinari_IIR_Vicenza_2005b.PDF.
- European FluoroCarbons Technical Committee (EFCTC), Data centers, https://www.fluorocarbons.org/applications/data-centres/.
-
Naturalrefrigerants, M&M Carnot to Launch Higher Capacity CO2-Based Cooling Units for Data Centers, https://naturalrefrigerants.com/mm-carnot-to-launch-higher-capacity-co2-based-cooling-units-for-data-centers/.
-
Naturalrefrigerants, KIO Networks Says Its New Valencia Data Center Is Spain's First to Use 100% Natural Refrigerants, https://naturalrefrigerants.com/spanish-data-center-is-nations-first-to-use-100-natural-refrigerants-with-co2-cooling-systems-from-advansor/.
-
GEA, The Schwarz IT data center DC 10 combines natural and mechanical cooling, https://www.gea.com/en/customer-cases/natural-cooling-schwarz-it-data-center/.
-
R744, Water-Only Chiller Gaining More Customers, https://archive.r744.com/articles/9277/water_only_chiller_gaining_more_customers.
-
Vertiv, Efficient Energy's Website Now Redirected to Vertiv, https://www.vertiv.com/de-emea/efficient-energy/.
-
CoolingPost, Refrigerant choice in HVAC chillers, https://www.coolingpost.com/blog-posts/refrigerant-choice-in-hvac-chillers/.
-
Enex. Yukon, https://www.enex.it/yukon/.
-
Cooltechnologies, Net-Zero Commercial/Industrial Air Conditioning, https://cooltechnologies.org/sector/commercial-industrial-air-conditioning/?pt=zero.
-
M. Eckert, M. Kauffeld and V. Siegismund, Natural Refrigerants: Applications and Practical Guidelines, VDE Verlag, 2022 Search PubMed.
-
International Energy Agency (IEA), TCP HPT, Annex, 48, https://waermepumpe-izw.de/.
-
Naturalrefrigerants, GEA Ammonia Heat Pump Will Cut Steam Energy Use by 75% at New Nestlé Infant Formula Plant, https://naturalrefrigerants.com/gea-ammonia-heat-pump-will-cut-steam-energy-use-by-75-at-new-nestle-infant-formula-plant/.
-
C. Schlemminger, M. Bantle, S. Jenssen, M. Dallai and M. Dorin, Industrial High Temperature Heat Pump for Simultaneous Production of Ice-Water and Process-Heat, in 15th IIR-Gustav Lorentzen Conference on Natural Refrigerants, 2022, https://sintef.brage.unit.no/sintef-xmlui/handle/11250/3029848 Search PubMed.
-
LPG, Siemens is selling a smart tumble dryer using a propane heat pump, https://lpg-apps.org/mediaroom/80/48/Siemens-is-selling-a-smart-tumble-dryer-using-a-propane-heat-pump.
- Swiss Fedeal Office for the Environment (FOEN), State of the Art Household Applicainces with Heat Pumps, 2022, https://www.bafu.admin.ch/bafu/en/home/topics/chemicals/info-specialists/chemicals--regulations-and-procedures/refrigerants.html.
-
Lübra, Raumluft-Wäschetrockner mit Zirkulations-Kondensation, https://www.luebra.ch/01_produkte/02_waeschetrockner/allgemein_pdf/warendeklaration-secotec.pdf.
-
AirCenter, AirCenter AD432R Luftentfeuchter blau R290, https://shop.aircenter.ch/luftentfeuchter-entfeuchtungsgeraete/AirCenter-AD432R-Luftentfeuchter-blau-R290.aspx.
-
Luft-Entfeuchter, Entfeuchter Dryfix DHP25, https://luft-entfeuchter.ch/media/files/datenblatt-dhp25.pdf.
-
ATMOsphere, North American, Guide to Natural Refrigerants in Ice Arenas, 2022, https://atmosphere.cool/ice-arenas-report-2022/ Search PubMed.
-
International Olympic Committee (IOC), The New Ice Age: Beijing 2022 Winter Olympics debut climate-friendly CO2 cooling system, https://olympics.com/ioc/news/the-new-ice-age-beijing-2022-winter-olympics-debut-climate-friendly-c02-cooling-system.
- J. Rogstam, A. Abdi and S. Sawalha, Carbon Dioxide in Ice Rink Refrigeration, 11th IIR Gustav Lorentzen Conf, Nat. Refrig. Nat. Refrig. Environ. Prot. GL, 2014, 2014, 585–592 Search PubMed , https://www.researchgate.net/publication/287392551_Carbon_dioxide_in_ice_rink_refrigeration.
-
Toromont, Think Natural Refrigerants are an Expensive Investment? Think Again!, https://www.cimcorefrigeration.com/resources/news/think-natural-refrigerants-are-an-expensive-investment-think-again!.
- M. O. McLinden, C. J. Seeton and A. Pearson, New Refrigerants and System Configurations for Vapor-Compression Refrigeration, Science, 2020, 370(6518), 791–796, DOI:10.1126/science.abe3692.
- F. Bai, M. An, J. Wu, X. Fang, P. Jiang, B. Yao, X. Zhao, X. Xiang, Z. Chen and J. Hu, Pathway and Cost-Benefit Analysis to Achieve China’s Zero Hydrofluorocarbon Emissions, Environ. Sci. Technol., 2023, 57, 6474–6484, DOI:10.1021/acs.est.3c00166.
- G. J. M. Velders, D. W. Fahey, J. S. Daniel, S. O. Andersen and M. McFarland, Future Atmospheric Abundances and Climate Forcings from Scenarios of Global and Regional Hydrofluorocarbon (HFC) Emissions, Atmos. Environ., 2015, 123, 200–209, DOI:10.1016/j.atmosenv.2015.10.071.
- L. M. Western, M. K. Vollmer, P. B. Krummel, K. E. Adcock, P. J. Fraser, C. M. Harth, R. L. Langenfelds, S. A. Montzka, J. Mühle, S. O’Doherty, D. E. Oram, S. Reimann, M. Rigby, I. Vimont, R. F. Weiss, D. Young and J. C. Laube, Global Increase of Ozone-Depleting Chlorofluorocarbons from 2010 to 2020, Nat. Geosci., 2023, 16, 309–313, DOI:10.1038/s41561-023-01147-w.
-
PHYSORG, Ozone-depleting CFCs hit record despite ban: Study, https://phys.org/news/2023-04-ozone-depleting-cfcs.html.
- K. E. Adcock, C. E. Reeves, L. J. Gooch, E. Leedham Elvidge, M. J. Ashfold, C. A. M. Brenninkmeijer, C. Chou, P. J. Fraser, R. L. Langenfelds, N. Mohd Hanif, S. O'Doherty, D. E. Oram, C. F. Ou-Yang, S. Moi Phang, A. Abu Samah, T. Röckmann, W. T. Sturges and J. C. Laube, Continued Increase of CFC-113a (CCl3CF3) Mixing Ratios in the Global Atmosphere: Emissions, Occurrence and Potential Sources, Atmos. Chem. Phys., 2018, 18(7), 4737–4751, DOI:10.5194/acp-18-4737-2018.
- M. K. Vollmer, D. Young, C. M. Trudinger, J. Mühle, S. Henne, M. Rigby, S. Park, S. Li, M. Guillevic, B. Mitrevski, C. M. Harth, B. R. Miller, S. Reimann, B. Yao, L. P. Steele, S. A. Wyss, C. R. Lunder, J. Arduini, A. McCulloch, S. Wu, T. S. Rhee, R. H. J. Wang, P. K. Salameh, O. Hermansen, M. Hill, R. L. Langenfelds, D. Ivy, S. O'Doherty, P. B. Krummel, M. Maione, D. M. Etheridge, L. Zhou, P. J. Fraser, R. G. Prinn, R. F. Weiss and P. G. Simmonds, Atmospheric Histories and Emissions of Chlorofluorocarbons CFC-13 (CClF3), ΣCFC-114 (C2Cl2F4), and CFC-115 (C2ClF5), Atmos. Chem. Phys., 2018, 18(2), 979–1002, DOI:10.5194/acp-18-979-2018.
- J. C. Laube, M. J. Newland, C. Hogan, C. A. M. Brenninkmeijer, P. J. Fraser, P. Martinerie, D. E. Oram, C. E. Reeves, T. Röckmann, J. Schwander, E. Witrant and W. T. Sturges, Newly Detected Ozone-Depleting Substances in the Atmosphere, Nat. Geosci., 2014, 7(4), 266–269, DOI:10.1038/ngeo2109.
-
UNEP, Montreal Protocol on Substances That Deplete the Ozone Layer - Report of the Medical and Chemical Technical Options Committee - 2018 Assessment, 2018, https://ozone.unep.org/sites/default/files/2019-04/MCTOC-Assessment-Report-2018.pdf.
-
Scientific Community, A Transition to Sustainable Heat Pumps – A Position Paper from the Scientific, Community, 2023, https://www.energy.kth.se/polopoly_fs/1.1241311.1679563728!/PositionPaper_ReviewFGas.pdf Search PubMed.
- Y. Wang, S. Liu, V. Nian, X. Li and J. Yuan, Life Cycle Cost-Benefit Analysis of Refrigerant Replacement Based on Experience from a Supermarket Project, Energy, 2019, 187, 115918, DOI:10.1016/j.energy.2019.115918.
-
North American Sustainable Refrigerant Council (NASRC), Financial Overview: Natural Refrigerants, 2019, https://static1.squarespace.com/static/55a672f1e4b06d4dd52f83de/t/5d24ecccdb81a40001c34414/1562701008125/NASRC+Financial+Fact+Sheet_053019.pdf Search PubMed.
-
Minnesota Pollution Control Agency (MPCA), Evaluation of Current Alternatives and Estimated Cost Curves for PFAS Removal and Destruction from Municipal Wastewater, Biosolids, Landfill Leachate, and Compost Contact Water, 2023, https://www.pca.state.mn.us/sites/default/files/c-pfc1-26.pdf.
-
Minnesota Pollution Control Agency (MPCA), Groundbreaking study shows unaffordable costs of PFAS cleanup from wastewater, https://www.pca.state.mn.us/news-and-stories/groundbreaking-study-shows-unaffordable-costs-of-pfas-cleanup-from-wastewater.
- C. Vering, H. Kroppa, V. Venzik, R. Streblow and D. Müller, Towards an Integral Decision-Making Process Applied to the Refrigerant Selection in Heat Pumps, Renewable Energy, 2022, 192, 815–827, DOI:10.1016/j.renene.2022.03.103.
- European Commission, EC Report from the Commission on barriers posed by codes, standards and legislation to using climate-friendly technologies in the refrigeration, air conditioning, heat pumps and foam sectors, 2016 Search PubMed , https://eur-lex.europa.eu/legal-content/en/ALL/?uri=CELEX:52016DC0749.
-
B. Gschrey, J. Kleinschmidt and S. Barrault, Briefing Paper: HFCs and HFC Alternatives in Split Air Conditioning Systems, 2020, https://www.oekorecherche.de/sites/default/files/publikationen/briefing_paper_hfc_alternatives_en.pdf Search PubMed.
-
European Commission, Commission Staff Working Document Impact Asessment Report Accompanying the Document “Regulation of the European Parliament and of the Council on Fluorinated Greenhouse Gases”, 2022, https://eur-lex.europa.eu/legal-content/EL/ALL/?uri=CONSIL:ST_8042_2022_ADD_3.
-
Hydrocarbons21, In reversal, IEC approves increase of charge limit for flammable refrigerants, https://hydrocarbons21.com/in-reversal-iec-approves-increase-of-charge-limit-for-flammable-refrigerants/.
-
CoolingPost, Standard revision a “milestone” for propane AC, https://www.coolingpost.com/world-news/standard-revision-a-milestone-for-propane-ac/.
|
This journal is © The Royal Society of Chemistry 2024 |
Click here to see how this site uses Cookies. View our privacy policy here.