Effect of extracellular organic matter (EOM) accumulation on algal proliferation and disinfection by-product precursors during cyclic cultivation†
Received
14th March 2024
, Accepted 23rd September 2024
First published on 23rd September 2024
Abstract
Algal blooms, driven by nutrient enrichment from nitrogen and phosphorus, pose significant challenges to water treatment processes, particularly due to the accumulation of extracellular organic matter (EOM). This study investigates the impact of EOM accumulation on the growth of Chlorella sp. and Microcystis aeruginosa—during a 36 day cyclic cultivation period, focusing on the effects of bound EOM (bEOM) and dissolved EOM (dEOM) on nutrient uptake and disinfection by-product (DBP) formation. The cultivation period was divided into three phases (R1, R2, and R3), with algal cell counts measured every 4 days using a flow cytometer, while changes in bEOM and dEOM were quantified. Nutrient uptake rates for nitrogen (N) and phosphate (P) were also evaluated per cycle, alongside analysis of critical organic precursors for disinfection by-products (DBPs). Results showed that the N and P uptake rates remained relatively stable for both alga types across all cycles. However, Chlorella sp. cell growth decreased to 20% after the third cycle, whereas M. aeruginosa maintained approximately 80% growth. This significant difference in growth inhibition between Chlorella sp. and M. aeruginosa was closely linked to the rate of bEOM accumulation. M. aeruginosa exhibited a three times faster accumulation rate of bEOM per cell compared to Chlorella sp. after the third cycle, which resulted from fewer remaining nutrients and the significant increase in pH during cyclic culturing. Further analysis revealed that DBPs derived from intracellular organic matter (IOM) were consistently higher than those from dEOM regardless of the cultivation phase. However, the formation potential of trihalomethanes (THMs) and haloacetic acids (HAAs) decreased by approximately 62% and 37%, respectively, for M. aeruginosa, while the formation potential of THMs and HAAs showed a minimal variation for Chlorella sp. In conclusion, bEOM accumulation on the algal cell surface following cultivation significantly impacts phosphate uptake and cell proliferation, particularly in Chlorella sp.
Water impact
The accumulation of extracellular organic matter (EOM) in algal blooms presents significant challenges to water treatment processes, particularly by influencing nutrient uptake and promoting the formation of disinfection by-products (DBPs). This study provides a comprehensive examination of the effects of bound EOM (bEOM) and dissolved EOM (dEOM) on the growth of Chlorella sp. and Microcystis aeruginosa during a 36 day cyclic cultivation period. By analyzing key factors such as algae cell count, nutrient uptake, organic changes, and DBP formation potential across multiple cultivation cycles, the research offers critical insights into the varying impacts of EOM accumulation on algal growth and water treatment efficacy. The results indicate that the two types of algae exhibit different growth responses to EOM accumulation. This phenomenon reveals algae-specific behaviors that can be leveraged to optimize water treatment strategies. It may provide a practical guideline for mitigating the adverse effects of EOM on water treatment processes, with implications for improving water quality and treatment efficiency.
|
1. Introduction
Algae blooms occurring in lakes or reservoirs, predominantly caused by nutrient enrichment from nitrogen and phosphorus, can rapidly develop under favorable conditions, leading to significant biological and environmental impacts.1 These nutrients, frequently introduced into aquatic ecosystems by anthropogenic and naturally-occurring activities such as the application of fertilizers, discharge of sewage, and runoff from urban areas, have a detrimental effect on these ecological systems and present environmental and human hazards.2 These hazards include shellfish poisoning, damage to internal organs, and increased risk of cancer.3–6 In drinking water treatment plants, algae-derived biomass and associated algal organic matter (AOM) can cause operational burdens, including filter clogging, higher demand for coagulants, and increased operational costs.7,8 AOM – which includes both extracellular organic matter (EOM) and intracellular organic matter (IOM) – can undergo chemical reactions with chlorinating agents, leading to the formation of disinfection by-products (DBPs) in post-chlorination for drinking water supply.9,10 So far, approaches to minimize the impacts of AOM on drinking water processes have been extensively studied.9,11–13 The reactivity of chlorine with aromatic and protein compounds in EOM, including bound EOM (bEOM) and dissolved EOM (dEOM), is particularly concerning, as it contributes to elevated DBP levels.14 Thus, it is crucial to handle the algae blooming problem using a feasible controlling strategy before algae-laden water enters the drinking water treatment plant.
In algae bloom management, the controlling strategy for algae growth attenuation has been well studied, especially for investigations into some important influencing factors towards algal proliferation, such as ambient temperature, N/P nutrient ratio, and light intensity. The optimal growth conditions for microalgae are sensitive to a temperature range between 20 °C and 30 °C.15,16 It has been proven that the proliferation of green algae and blue-green algae is subject to light intensities ranging from 1000 to 5000 lux and 3000 to 6000 lux, respectively.17 Algae growth rates and biomass productivity are significantly enhanced by an increased nitrogen-to-phosphorus (N/P) ratio where Chlorella vulgaris reaches maximum productivity at a ratio of 55
:
1 and Microcystis aeruginosa achieves an optimal cell yield at an N/P ratio of 16.1.18,19 For optimal algae growth, the nutrient solution of algal cultures should contain a minimum of 0.05 mg L−1 phosphorus and 0.1 mg L−1 nitrate.20 In addition, extracellular polymeric substances (EPSs) also play a significant role in algal growth potential. Previous studies have reported that the accumulation of EPSs in Chlorella sp. suspensions is dominated by hydrophilic groups, particularly hydroxyl and carbonyl functional groups which provide essential binding sites and adsorption properties during cultivation.21,22 In that case, EPSs function as chelators during algae cultivation, by binding essential nutrients such as nitrogen, phosphorus, and cations. This chelation mechanism reduces the availability of these nutrients for algal uptake, hence mitigating the algal growth rate.23 Other studies also have reported that the concentration of nitrogen and phosphorus in the medium can be decreased by up to 50% due to the binding effects of EPSs, leading to a reduction in algal biomass production over the cultivation period.24,25 Meanwhile, a high concentration of EPSs between Microcystis aeruginosa cells can create sticky matrices that trap and immobilize the cells, which eventually reduces cell mobility and suppresses cell proliferation.26
In order to handle algal-impacted water for producing safe drinking water, understanding the origin of EOM and its buildup impact on cell proliferation during algal growth is warranted. The increased EOM concentration may lead to nutrient inhibition and the formation of hazardous organic compounds, which not only inhibit algal growth but also pose concerns for water security by increasing the precursors for DBPs. However, the complexity of EOM including its variations in molecular weight distribution as well as chemical composition could further change its impact on algal growth. For example, the presence of oxygen-containing functional groups, such as carboxylic acids, and nitrogen-containing groups, allows EOM to interact with various inorganic and organic ions, resulting in different degrees of hydrophilicity.27 Additionally, the solubility of EOM is strongly influenced by pH fluctuations, while its production rate is affected by temperature.28,29 Given this complexity and potential to influence the algal growth environment, a thorough examination of the EOM impact on algal growth potential is crucial.28,30 Although several studies have examined the properties of EPSs and their influence on treatment procedures, limited research has paid attention to the behavior of bEOM accumulation on the surface of algae and its impact on the cell growth and corresponding DBP formation potential for drinking water supply.
This study aims to examine the impact of EOM accumulation on the growth of Chlorella sp. and M. aeruginosa cells, along with DBP precursors, over three cultivation cycles. Additionally, the essential uptake rates of nitrate (NO3−) and phosphorus (PO43−) ions are assessed. A detailed analysis of the dissolved organic carbon (DOC) variations in distinct organic substance fractions, specifically IOM, dEOM, and bEOM, is included. The excitation–emission matrix (EEM) fluorescence technique was utilized to study the features of emerging fluorescent organic substances. The possible formation of DBPs was further assessed, given the tendency of their organic precursors to proliferate during algae cultivation. This study proposes a comprehensive model interpreting the relationship between EOM accumulation and algae growth, with potential beneficial implications for water and algae treatment.
2. Materials and methods
2.1 Algae culturing protocol
The two types of algae, Chlorella sp. and M. aeruginosa, were harvested from reservoirs on Matsu Island in Taiwan. Prior to cultivation, the strains were purified. They were then grown in 500 mL Erlenmeyer flasks fortified with nutrients sourced from BG-11 solution media, as per the methodology previously outlined by ref. 12. The initial cell concentration of the algae was set at 2 × 105 cells per mL. The strains were placed under a hood fitted with an automatic flash shaker (OS-D Series, Digisystem Laboratory Instrument Inc., Taiwan) that additionally housed six fluorescent lamps (T5-8W-6500K, Jan Cheng Lighting, China). The lighting followed a 16 hour day and 8 hour night cycle. Algal growth was observed every 4 days using a flow cytometer (Beckman Coulter, USA), which allowed for the assessment of growth dynamics and nutrient uptake rates. A 4 d observation interval is significant as it aligns with the typical growth phases of algae.31,32 Following one cycle (R1), which lasted twelve days, the algal cells were analyzed comprehensively. The cultivation process was repeated for the second and third cycles (R2 and R3), maintaining the same initial algal concentrations, as presented in Fig. 1.
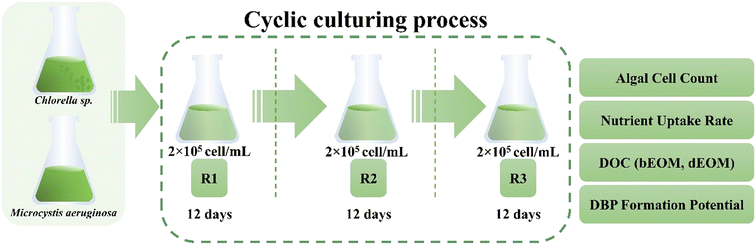 |
| Fig. 1 Algal culturing process with three cycles for Chlorella sp. and M. aeruginosa. | |
2.2 Analytical methods
2.2.1 Algae cell counting.
The number of algal cells was quantified using a flow cytometer from Beckman Coulter, USA. The sample solution was filtered through a 70 μm nylon membrane from Biofil, China, and transferred to microtubes from BioScience, USA. Measurements were performed within 2 min or until the reading cell indicated 10
000 cells.
2.2.2 Nutrient uptake rate measurement.
The residual phosphate (PO43−) and nitrate (NO3−) ions in the mixed solution were quantified to determine the nutrient uptake rate during algal cultivation. The molybdate colorimetric method 365.3 (ref. 33) was used to compute the concentration of PO43− ions. The absorbance was recorded at 880 nm using a UV-visible spectrophotometer (UV-1280, Shimadzu, Japan) within 10 min after reading. Notably, the reagent mixture has a time-sensitivity limitation of 20–25 min. The colorimetric method 352.1 was adopted to determine the NO3− concentration for each test.34 The NO3− ions reacted with brucine sulfate in a 13 N H2SO4 solution at 100 °C, and the absorbance was measured at 410 nm.
2.2.3 AOM extraction.
Before starting the test, algal solutions were filtered using a 0.45 μm cellulose mixed ester membrane from Advantec, Japan. An organic carbon analyzer TOC-L, Shimadzu, Japan, was used to measure the DOC in the samples. Three unique fractions of AOM: IOM, dEOM, and bEOM were further isolated from the algal suspensions. The process began by centrifuging 40 mL of algal suspensions at 4000 rpm for 15 min using a multipurpose centrifuge, DMO606, from DLab, China. Then, the supernatant was filtered through a 0.45 μm membrane to measure the dEOM. The pellets were combined with 60% wt/wt NaCl in a 1
:
1 ratio and heated at a controlled temperature (40–50 °C for M. aeruginosa cells and 50–60 °C for Chlorella sp.) for 20 min. After cooling it to 25 °C, the suspension was centrifuged at 6000 rpm for 25 min. The supernatant was filtered through a 0.45 μm membrane and measured for bEOM. To remove any lingering EOM, the extraction pellet underwent three consecutive rinses with deionized water followed by centrifugation at 6000 rpm for 15 min. The supernatant was discarded, and the pellet was mixed with 10 mL of DI water before being placed in a freezer. The sample was then freeze-dried for 24 h at roughly 50 °C with a pressure of approximately 0 Pa using a freeze dryer, FD-1A50, from Boyikang, Taiwan. Finally, the sample was crushed using a mortar, diluted with DI water, and filtered using a 0.45 μm membrane to acquire the IOM solutions.
2.2.4 DBP formation potential tests.
The potential formation of four types of DBPs – trihalomethanes (THMs), haloacetic acids (HAAs), haloacetonitriles (HANs), and haloketones (HKs) was evaluated after 7 days of chlorination using a solution of NaOCl under darkroom conditions.10 During this analysis, the free chlorine to DOC ratio was preserved at 5
:
1. Modified liquid–liquid extraction from the USEPA 551.1 protocol was used to measure the presence of THMs, HANs, and HKs.35 A 30 mL chlorinated sample mixed with 10 g of Na2SO4 was combined with 3 mL of methyl tert-butyl ether (MTBE) to extract the DBPs. In the case of HAAs, the modified USEPA method 552.3 was employed.36 Here, the chlorinated sample was first acidified with H2SO4 to lower the pH to less than 0.5 before running the extraction. Next, a volume of 40 mL from the sample was mixed with 13.5 mL of Na2SO4, followed by 3 mL of MTBE. The resulting supernatant was combined with an ethanol mixture that contained 10% H2SO4. This mixture was heated at 55 °C for 2 h. After cooling down, the samples were further reacted with 5 mL of Na2SO4 and 2 mL of NaHCO3. The remaining supernatant was then transferred into 1.5 mL vials. The extracted samples were analyzed using a gas chromatography tool furnished with an electron capture detector (Nexis GC-ECD-2030, Shimadzu, Japan). This powerful tool had a column measurement of 30 m × 0.25 mm × 0.25 μm (DB-1701, Agilent, USA). The method detection limit for each DBP compound varied between 0.01 and 0.23 μg L−1.
3. Results and discussion
3.1 Inhibition of algal growth under cyclic algae culturing
The changes in the algal cell count were observed during successive cyclic algal culturing to better understand the inhibitory effects on algal growth (Fig. 2). The observations have revealed that the cell count of Chlorella sp. reaches a peak of about 2.1 × 106 cells per mL after the first round (R1). Subsequently, the count decreases consistently to about 5.5 × 105 cells per mL in the second round (R2) and further decreases to 1.4 × 105 cells per mL in the third round (R3), accounting for a 93.3% decrease in the total cell count. The highest count of M. aeruginosa algal cells is achieved after R1, reaching up to 3.5 × 106 cells per mL. Unlike Chlorella sp., the M. aeruginosa cell counts in R2 and R3 modestly decrease by roughly 8.3% and 22%, respectively. M. aeruginosa cells proliferate stably due to their adaptability under various environmental conditions. A past study has indicated that under conditions of minimal nutrient availability (100 μM for NO3− and 1 μM for PO43−), the cell count could rise significantly by approximately six orders of magnitude.20
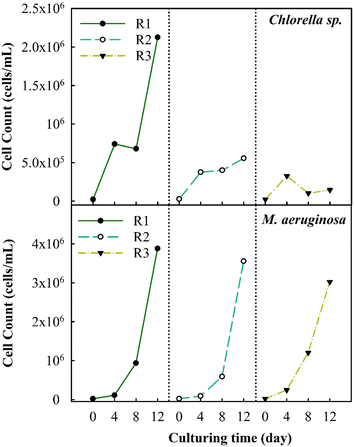 |
| Fig. 2 Cell count of Chlorella sp. and M. aeruginosa during three rounds of cyclic culturing. | |
A previous study has found that a decrease in the levels of PO43− and NO3− ions in the culture solution can negatively impact the growth rate, algal development, and biomass production.37 The substantial drop in cell count in the R2 and R3 stages is observed potentially due to the effect of the remaining nutrients. As shown in Table S1,† the concentration of the remaining nutrients in Chlorella sp. suspensions at 4 days after R3 decreased from 247 mg L−1 to 41.5 mg L−1 in terms of NO3− as well as from 11.3 mg L−1 to 0.04 mg L−1 in terms of PO43−; then, no further reduction in the concentration of nutrients is observed. In contrast, the remaining nutrients in M. aeruginosa suspensions at 4 days after R3 merely decrease from 247 mg L−1 to 173.7 mg L−1 in terms of NO3− as well as from 11.3 mg L−1 to 3.7 mg L−1 in terms of PO43−. This suggests that the decrease of nutrient components would inhibit the algal growth rate. Furthermore, fluctuations in pH levels throughout the cultivation period could have a significant impact on the growth of algae. The pH at the initial of cultivation typically averages 7.5 for Chlorella sp. and M. aeruginosa suspensions and gradually rises to approximately 11.5 after R3, as shown in Fig. S1.† The increase in pH is much more pronounced in the case of Chlorella sp. compared to that in the case of M. aeruginosa. Within the initial 4 day culture, the pH of Chlorella sp. suspensions increases to 10.5–11 from R1 to R3, while it merely increases to 8.7–9.5 from R1 to R3 for M. aeruginosa suspensions. A previous study has reported that a rise in pH during culturing can also inhibit the growth of Chlorella vulgaris. During 8 day cell culturing, the increased pH would facilitate the production of polysaccharides and organic nitrogen, which significantly impacts the growth of Chlorella vulgaris.38 In addition, as the pH increases to exceed 10.5 during algal growth, the form of ionic nutrients (e.g., phosphorous) could be changed into co-precipitates with calcium (Ca3(PO4)2), which could lower the uptake rate of nutrients by algae.39 Therefore, the growth of Chlorella sp. is significantly inhibited by the decreased nutrients and increased pH, which may influence the variations in metabolisms of cells.
3.2 Algal organic variation in cyclic algal culturing
Fig. 3 illustrates the variation of AOM in relation to fluorescent substances as influenced by cyclic algal culturing. The data shows a mild increase in all total fluorescent substances in Chlorella sp. after 36 days of culturing with SMPL and HAL compounds demonstrating higher intensity. In contrast, M. aeruginosa exhibits no substantial changes across four compounds, although SMPL and HAL compounds exhibit an intensity approximately 2.8 times higher than in Chlorella sp. following three cycles of algal culturing.40,41
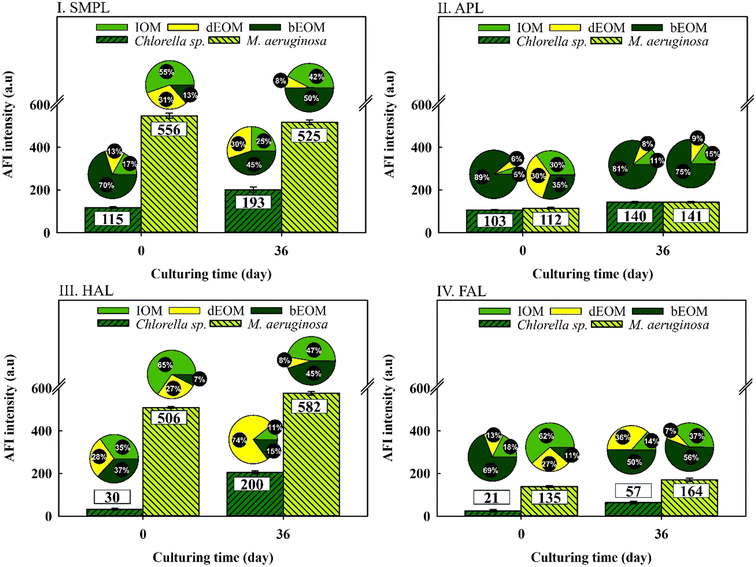 |
| Fig. 3 Variations of AFI reduction in different fluorophore regions from various organic fractions in Chlorella sp. and M. aeruginosa suspensions. | |
Intriguingly, both types of algal cells display significant shifts in organic substance ratios, regardless of average fluorescence intensity (AFI) variations. In Chlorella sp., bEOM-derived fluorescent compounds are reduced between 8 and 30%, while dEOM and IOM-derived fluorescent compounds show a significant rise of around 25–74% following cyclic algal culturing. This increase is most notable in the dEOM fraction. The amplified fluorescence in dEOM-derived compounds following cyclic culturing is attributed to the decay of Chlorella sp. cells. These cells release intracellular substances into the bulk solution in accordance with the data presented in Fig. 2.
On the other hand, M. aeruginosa has shown over 50% increment in bEOM-derived fluorescent compounds following cyclic culturing, indicating significant bEOM accumulation over the culture period. Concurrently, there is a substantial decline in both IOM and dEOM-derived fluorescent compounds – approximately 19% and 22%, respectively – further supporting this accumulation hypothesis. Critical to note is that an increase in bEOM possibly functions as a storage medium for essential nutrients like NO3− and PO43− ions during the algae growth.42 Moreover, a past study by Liu et al. (2017)40 inferred that fluctuations in nutrient availability result in changes in fluorophore substances. Hence, it is evident that fluorescent materials are efficient in detecting bEOM accumulation in M. aeruginosa and organic release in Chlorella sp. Consequently, more research into EOM changes throughout cyclic algal culture would be worthwhile.
The changes in EOM variation were assessed during cyclic algal culturing by examining the differences in dEOM- and bEOM-derived DOC concentrations and EOM concentration per cell in Chlorella sp. and M. aeruginosa (Fig. 4). The DOC level in Chlorella sp. has shown a significant increase to roughly 27 mg L−1 after R2, and then dropped to 12 mg L−1 after R3. The sudden increase of DOC after R2 for Chlorella sp. suspensions could be attributed to suboptimal algal growth where some of the algal cells are damaged, leading to released IOM into the solution at the end of R2. This result is similar to a previous study where the DOC level increases from 1.19 mg L−1 to 7 mg L−1 once cell damage occurs within 8 days of culturing.43 After R3, Chlorella sp. cells significantly undergo decay and limited cell growth occurs in the meantime, as shown in Fig. 2, leading to a rapid decline in excreted DOC from cells. Consequently, this suggests that longer culture periods can create lethal conditions for Chlorella sp., corroborated by a substantial decrease in the total cell count (Fig. 2).
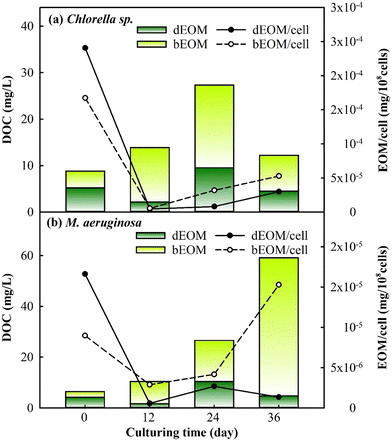 |
| Fig. 4 Variations of dEOM and bEOM concentrations in terms of DOC per cell for (a) Chlorella sp. and (b) M. aeruginosa during cyclic culturing. | |
Conversely, M. aeruginosa has exhibited a substantial increase in cell DOC by approximately 59 mg L−1 post R3, with bEOM-derived DOC contributing about 91% of the total. The significant accumulation of bEOM in M. aeruginosa cells post R3 is likely due to the presence of highly hydrophobic organic compounds, which enhance accumulation rates.44 Previous research has emphasized the rapid accumulation of bEOM in algal cells under nutrient deficiency, primarily with NO3− ions.29,45 Under situations of starvation, M. aeruginosa cells are able to recycle intracellular components, such as lipids and proteins, allowing them to retain their metabolic processes,46 which echoes the increase in bEOM from R1 to R3 culturing. Thus, the cyclic cultivation of M. aeruginosa can significantly contribute to the accumulation of bEOM on the cell surface.
The behavior of EOM accumulation on algae cells was further studied by observing the variation of EOM per cell. It was noticed that both bEOM and dEOM per cell for Chlorella sp. have displayed high levels after cyclic algal culturing, around 2.9–5.2 × 10−5 mg per 108 cells. The increase in dEOM per cell after R3 signifies growth in dEOM substances due to the decay of Chlorella sp. The decay process causes the release of intracellular substances into the bulk solution, becoming dEOM and depositing on algal surfaces as bEOM. In the case of M. aeruginosa cells, there is an increase in bEOM per cell from R2 to R3 by 1.1 × 10−5 mg per 108 cells, while dEOM per cell presents a decrease. The swift increase in bEOM per cell after R3 is linked to the metabolism of living cells. This significant rise is due to the enzymatic activities in M. aeruginosa cells, such as ribulose-1,5-bisphosphate carboxylase/oxygenase (RuBisCO) and fructose-1,6-bisphosphate aldolase (FBA), which are related to photosynthetic activity, especially at optimal temperatures.47 The variations in DOC and EOM during cyclic culturing have indicated that Chlorella sp. suspensions experience a decline in DOC due to cell decay, while M. aeruginosa suspensions undergo an increase in DOC due to the accumulation of EOM induced by cell productivity. These findings have implied that bEOM accumulation during cyclic culturing could further impact the uptake of nutrients that are highly related to the growth of Chlorella sp. and M. aeruginosa.
3.3 Effect of EOM accumulation on the uptake rate
The changes in nutrient absorption rates of NO3− and PO43− ions were evaluated to thoroughly study EOM accumulation impacts during the cultivation of Chlorella sp. and M. aeruginosa cells, as depicted in Fig. 5. The results have revealed a significant increase in the nutrient uptake rates of NO3− and PO43− for Chlorella sp. after 4 days, reaching approximately 80% and 97%, respectively. These uptake rates plateau afterward, particularly for NO3−. This swift absorption within the initial 4 days may be due to the ability of Chlorella sp. to absorb nutrients in the early periods and leverage these nutrients in the following days.48 However, when bEOM accumulates on the cell surface of Chlorella sp., it could potentially impede the nutrient absorption rate.49,50 Minor variances in the PO43− ion uptake rate compared to NO3− ions suggest that Chlorella sp. might rely more on PO43− ions throughout cyclic algal cultivation.
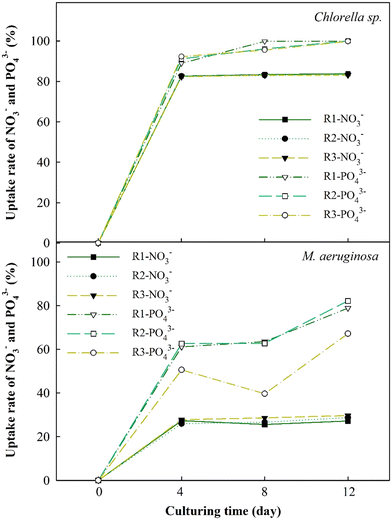 |
| Fig. 5 Uptake rate of NO3− and PO43− for Chlorella sp. and M. aeruginosa during cyclic culturing. | |
The uptake rates for M. aeruginosa cells are lower than those for Chlorella sp., accounting for 30% and 75%, respectively. Nutrient utilization in M. aeruginosa happens gradually, especially with PO43− ions. There is a decrease in PO43− use in the R3 stage, possibly due to accumulation effects from bEOM. Prior research suggested that M. aeruginosa cells react to PO43− starvation by altering growth and metabolism and releasing harmful substances.51 The slight variation in NO3− ion absorption does not necessarily suggest a decrease in M. aeruginosa cell activity. This notion corresponds to earlier research indicating that M. aeruginosa needs only a small concentration of NO3− ions for growth.52 It is inferred that the inhibition of nutrient absorption and cell growth is mainly driven by increased accumulation of bEOM over the culture period.
3.4 Disinfection by-product formation potential under cyclic algae culturing
The DBPFP, derived from bEOM and IOM of the two microcells during cyclic algal culturing, is shown in Fig. 6. It demonstrates a noticeable increase in the total specific DBPFP from bEOM of Chlorella sp., where HANs overwhelmingly lead the formation, followed by THMs and HAAs. This surge in HANs after R3 might result from an increase in protein-like compounds rife with amino acids and nitrogenous organic compounds.53 The shift in bEOM-derived DBPFP generation in Chlorella sp. may correlate with cell decay, which triggers the release of intracellular substances that are then absorbed as bEOM. Additionally, excessive stress conditions in algae can boost the production of stored amino acids, acting as protein reserves. These reserves could be secreted and contribute to the creation of bEOM substances.54 On the other hand, alterations in DBPFP from IOM might be linked to the incorporation of precursors for THMs and HAAs post-R3, such as the substantial presence of HAL substances,55,56 a fact bolstered by the change in fluorophore substances, as indicated in Fig. 3.
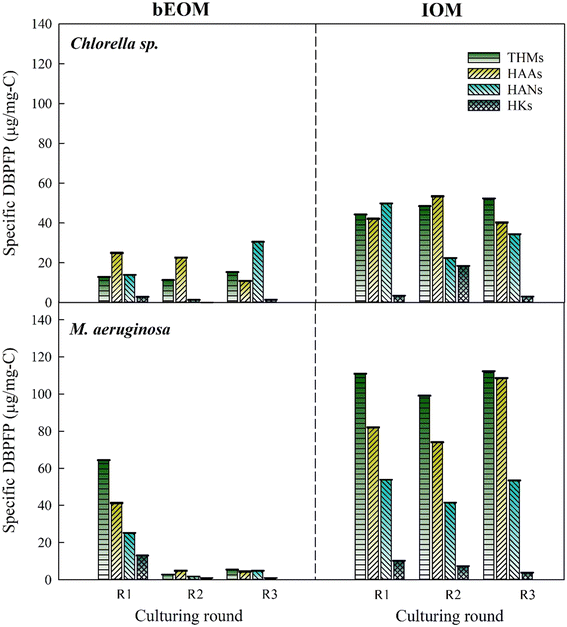 |
| Fig. 6 Variations of specific DBPFP for Chlorella sp. and M. aeruginosa after various rounds during cyclic culturing. | |
A noticeable drop in all DBPFP from bEOM is seen after R2 and R3 in M. aeruginosa cells. This phenomenon occurs when the DOC as bEOM surges higher (see Fig. 4b) compared to the original DBPs, resulting in lower specific DBPFP concentration. This implies that although the formation of DOC as bEOM is high, it does not contribute significantly as a precursor for DBP formation after R2 and R3. This suggests that the accumulation of bEOM can control DBPs at a lower level. The trend of the formation of DBPs in IOM is consistent across all culture cycles, characterized by elevated levels of THMs, HAAs, and HANs. This could be because of higher concentrations of IOM-derived DBP formation, as these compounds could potentially be consumed by M. aeruginosa cells during the algal culture cycle, inhibiting the transport of IOM substances to the bEOM shield. It is fair to conclude that the accumulation of bEOM positively influences the M. aeruginosa cells by enabling them to form DBPs, thereby supporting their intrinsic cellular activities.
3.5 Organic accumulation mechanisms
In the cultivation of Chlorella sp. and M. aeruginosa, the growth rate and metabolic responses of these algae are significantly influenced by bEOM accumulation and nutrient uptake rates. Different bEOM levels trigger a variety of responses in distinct algal species. Fig. 7 reveals the impact of bEOM accumulation levels on nutrient absorption during algal cultivation. Unique interactions with the bEOM layer are showcased by Chlorella sp. due to its cell wall structure. Higher bEOM levels impede the transfer of crucial nutrients into the cell, resulting in a decreased nutrient uptake as Chlorella sp. depends on PO43− ions. The larger molecular size of PO43−, compared to NO3−, poses added difficulty for the formation of cellular barriers. Therefore, even with ample NO3−, restricted PO43− results in growth rate inhibition in Chlorella sp. and a significant decrease in growth after roughly 36 days of continuous algal cultivation. This underlines the key role bEOM plays in modulating nutrient availability and algal growth.
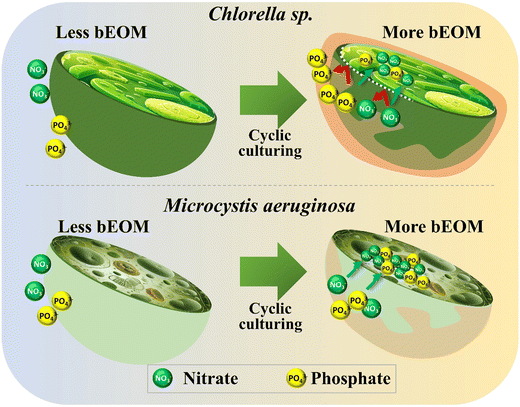 |
| Fig. 7 Impact of bEOM accumulation on nutrient uptake during cyclic culturing for Chlorella sp. and M. aeruginosa. | |
In contrast, M. aeruginosa cells lacking a cell wall demonstrate different interactions between varying levels of bEOM. The lack of a cell wall allows for more efficient nutrient transfer, enabling these cells to utilize available nutrients more effectively. Even at relatively low cell densities, the early stages of M. aeruginosa culture show rapid nutrient uptake.52 As the culture develops, nutrient absorption intensifies, frequently surpassing immediate cellular needs. This “luxury uptake” behavior allows M. aeruginosa cells to store surplus nutrients, notably NO3− and PO43−, for future use under nutrient-poor conditions.4 Moreover, the carboxyl groups found in M. aeruginosa cells amplify their ability to absorb nutrients, giving them a distinct advantage over Chlorella sp. under numerous bEOM circumstances. This sturdiness of M. aeruginosa cells is also demonstrated by their long-term growth. Unlike the decline observed in Chlorella sp., the M. aeruginosa cell density continues to rise. The apparent discrepancy between Chlorella sp. and M. aeruginosa regarding nutrient absorption and utilization under various bEOM states implies that Chlorella sp. fails to efficiently uptake nutrients under most bEOM conditions, leading to stagnated growth. M. aeruginosa cells, on the other hand, show a hearty growth pattern, supported by their proficient nutrient absorption process and the absence of hurdles.
4. Conclusions
This study analyzed the impact of EOM accumulation on algal growth and excretion during cyclic culturing. Findings indicated a consistent growth pattern in M. aeruginosa cells, with a slight decrease noted. In contrast, Chlorella sp. cells were significantly reduced to 1.4 × 105 cells per mL. Both algal types showed significant EOM fluctuations. Chlorella sp. reduced its DOC to 12 mg L−1, while M. aeruginosa increased it to 59 mg L−1 after 36 days of culture. Interestingly, M. aeruginosa had a significantly higher accumulation of bEOM. IOM-derived DBPs exhibit a higher level compared to bEOM, independent of the culture period. During the culture period, significant decreases in precursors for THMs and HAAs for M. aeruginosa were observed, while Chlorella sp. showed minimal variation in THM and HAA formation potential. The build-up of EOM on the cellular surface during culturing forms a physical barrier, impeding nutrient diffusion into the cell and thus restraining cell growth and nutrient consumption. This effect was particularly evident in phosphate absorption by Chlorella sp. Therefore, it is critical to quantitatively determine EOM accumulation during algal culturing to control nutrient consumption and algal growth potential for the minimization of DBP precursors in drinking water supply.
Data availability
The authors confirm that the data supporting the findings of this study are available within the article [and/or its ESI† materials].
Author contributions
Jr-Lin Lin: conceptualization, resources, visualization, writing – review & editing, validation, supervision. Fahrudin Sidik: writing – original draft, writing and editing, methodology, formal analysis.
Conflicts of interest
The authors declare that they have no known competing financial interests or personal relationships that could have appeared to influence the work reported in this paper.
Acknowledgements
The authors would like to extend their appreciation to the National Science and Technology Council (NSTC), Taiwan for the financial assistance of this research (Grant number: 112-2221-E-033-015-MY2).
References
- W. A. Wurtsbaugh, H. W. Paerl and W. K. Dodds, Nutrients, eutrophication and harmful algal blooms along the freshwater to marine continuum, Wiley Interdiscip. Rev.: Water, 2019, 6, e1373 CrossRef.
- R. Wang, J. Zhang, C. Cai and H. Zhang, How to control nitrogen and phosphorus loss during runoff process? – A case study at Fushi Reservoir in Anji County (China), Ecol. Indic., 2023, 155, 111007 CrossRef CAS.
- C. A. Amorim and A. D. N. Moura, Ecological impacts of freshwater algal blooms on water quality, plankton biodiversity, structure, and ecosystem functioning, Sci. Total Environ., 2021, 758, 143605 CrossRef CAS PubMed.
- M. E. S. Bracken, H. Hillebrand, E. T. Borer, E. W. Seabloom, J. Cebrian, E. E. Cleland, J. J. Elser, D. S. Gruner, W. S. Harpole, J. T. Ngai and J. E. Smith, Signatures of nutrient limitation and co-limitation: responses of autotroph internal nutrient concentrations to nitrogen and phosphorus additions, Oikos, 2015, 124, 113–121 CrossRef CAS.
- J. J. Dorantes-Aranda, Harmful Algae Impacting Aquatic Organisms: Recent Field and Laboratory Observations, Toxins, 2023, 15, 339 CrossRef PubMed.
- A. Lad, J. D. Breidenbach, R. C. Su, J. Murray, R. Kuang, A. Mascarenhas, J. Najjar, S. Patel, P. Hegde, M. Youssef, J. Breuler, A. L. Kleinhenz, A. P. Ault, J. A. Westrick, N. N. Modyanov, D. J. Kennedy and S. T. Haller, As We Drink and Breathe: Adverse Health Effects of Microcystins and Other Harmful Algal Bloom Toxins in the Liver, Gut, Lungs and Beyond, Life, 2022, 12, 418 CrossRef CAS PubMed.
- Y. Du, W. Zhou, L. Zhang and X. Liu, Gravity-driven membrane coupled with oxidation technology to modify the surface properties and biofilm formation: Biofouling mitigation, J. Environ. Manage., 2023, 345, 118444 CrossRef CAS PubMed.
- M. Malaguti, A. F. Novoa, F. Ricceri, M. Giagnorio, J. S. Vrouwenvelder, A. Tiraferri and L. Fortunato, Control strategies against algal fouling in membrane processes applied for microalgae biomass harvesting, J. Water Process Eng., 2022, 47, 102787 CrossRef.
- J. Chen, N. Gao, L. Li, M. Zhu, J. Yang, X. Lu and Y. Zhang, Disinfection by-product formation during chlor(am)ination of algal organic matters (AOM) extracted from Microcystis aeruginosa: effect of growth phases, AOM and bromide concentration, Environ. Sci. Pollut. Res., 2017, 24, 8469–8478 CrossRef CAS PubMed.
- J.-L. Lin, F. Sidik and S.-F. Kang, Minimization of halogenated disinfection by-product precursors by Al-based electrocoagulation–flotation (ECF) toward cyanobacteria-laden water, Aqua Water Infrastruct. Ecosyst. Soc., 2022, 71, 1054–1067 Search PubMed.
- X. Tang, H. Zheng, B. Gao, C. Zhao, B. Liu, W. Chen and J. Guo, Interactions of specific extracellular organic matter and polyaluminum chloride and their roles in the algae-polluted water treatment, J. Hazard. Mater., 2017, 332, 1–9 CrossRef CAS PubMed.
- J.-L. Lin, F. Sidik and S.-F. Kang, Harvesting of microcells from cyanobacteria-laden water by energy-efficient electro-flocculation-flotation with aluminum hydrates, J. Water Process Eng., 2023, 52, 103585 CrossRef.
- Y. Wang, H. Xu, H. Yao, B. Liu, M. Ding, T. Lin, T. Mo, L. Gao and L. Zhang, Insights into the role of prechlorination in algae-laden raw water distribution process: Algal organic matter and microcystin-LR release, extracellular polymeric substances (EPS) aggregation, and pipeline biofilm communities, J. Hazard. Mater., 2023, 443, 130306 CrossRef CAS PubMed.
- X. Ruan, Y. Xiang, C. Shang, S. Cheng, J. Liu, Z. Hao and X. Yang, Molecular characterization of transformation and halogenation of natural organic matter during the UV/chlorine AOP using FT-ICR mass spectrometry, J. Environ. Sci., 2021, 102, 24–36 CrossRef CAS PubMed.
- S. P. Singh and P. Singh, Effect of temperature and light on the growth of algae species: A review, Renewable Sustainable Energy Rev., 2015, 50, 431–444 CrossRef CAS.
- C. Butterwick, S. I. Heaney and J. F. Talling, Diversity in the influence of temperature on the growth rates of freshwater algae, and its ecological relevance, Freshwater Biol., 2005, 50, 291–300 CrossRef.
- Y. Meijiao, W. Yindong and H. Xianjiang, Effects of light on the growth rate and chlorophyll content of Chlorella vulgaris and Scenedesmus obliquus, Anhui Agricultural, Sci. Bull., 2007, 13, 27–29 Search PubMed.
- T. Magyar, B. Németh, J. Tamás and P. T. Nagy, Improvement of N and P ratio for enhanced biomass productivity and sustainable cultivation of Chlorella vulgaris microalgae, Heliyon, 2024, 10, e23238 CrossRef CAS PubMed.
- Y. Liu, L. Li and R. Jia, The Optimum Resource Ratio (N:P) for the Growth of Microcystis Aeruginosa with Abundant Nutrients, Procedia Environ. Sci., 2011, 10, 2134–2140 CrossRef CAS.
- H. Kim, B. Y. Jo and H. S. Kim, Effect of different concentrations and ratios of ammonium, nitrate, and phosphate on growth of the blue-green alga (cyanobacterium) Microcystis aeruginosa isolated from the Nakdong River, Korea, Algae, 2017, 32, 275–284 CrossRef CAS.
- J. Lv, F. Zhao, J. Feng, Q. Liu, F. Nan, X. Liu and S. Xie, The impact of particulate and soluble organic matter on physicochemical properties of extracellular polymeric substances in a microalga Neocystis mucosa SX, Algal Res., 2020, 51, 102064 CrossRef.
- P. Gupta and B. Diwan, Bacterial Exopolysaccharide mediated heavy metal removal: A Review on biosynthesis, mechanism and remediation strategies, Biotechnol. Rep., 2017, 13, 58–71 CrossRef PubMed.
- W. Huang, M. Hu, X. Qin, W. Zhou, W. Lv and B. Dong, Fouling of extracellular algal organic matter during ultrafiltration: The influence of iron and the fouling mechanism, Algal Res., 2017, 25, 252–262 CrossRef.
- J. Jaroszuk-Ściseł, A. Nowak, I. Komaniecka, A. Choma, A. Jarosz-Wilkołazka, M. Osińska-Jaroszuk, R. Tyśkiewicz, A. Wiater and J. Rogalski, Differences in Production, Composition, and Antioxidant Activities of Exopolymeric Substances (EPS) Obtained from Cultures of Endophytic Fusarium culmorum Strains with Different Effects on Cereals, Molecules, 2020, 25, 616 CrossRef PubMed.
- Z. Duan, X. Tan, L. Shi, Q. Zeng, I. Ali, R. Zhu, H. Chen and K. Parajuli, Phosphorus Accumulation in Extracellular Polymeric Substances (EPS) of Colony-Forming Cyanobacteria Challenges Imbalanced Nutrient Reduction Strategies in Eutrophic Lakes, Environ. Sci. Technol., 2023, 57, 1600–1612 CrossRef CAS PubMed.
- X. Wang, Y. Zhao, X. Jiang, Y. Wang, H. Li, L. Wang and W. Liang, The growth and physiological activity of Microcystis aeruginosa after flocculation using modified tannin, Int. Biodeterior. Biodegrad., 2018, 133, 180–186 CrossRef CAS.
- H. Chu, H. Yu, X. Tan, Y. Zhang, X. Zhou, L. Yang and D. Li, Extraction procedure optimization and the characteristics of dissolved extracellular organic matter (dEOM) and bound extracellular organic matter (bEOM) from Chlorella pyrenoidosa, Colloids Surf., B, 2015, 125, 238–246 CrossRef CAS PubMed.
- B. Cao, Y. Zhang, Y. Shi, R. Ren, H. Wu, W. Zhang, D. Wang, T. Zhang and J. Xiong, Extracellular organic matter (EOM) distribution characteristic in algae electro-dewatering process, J. Environ. Manage., 2020, 265, 110541 CrossRef CAS PubMed.
- M. Li, W. Zhu, L. Gao and L. Lu, Changes in extracellular polysaccharide content and morphology of Microcystis aeruginosa at different specific growth rates, J. Appl. Phycol., 2013, 25, 1023–1030 CrossRef CAS.
- J. Ma, B. Jia, S. Li, Y. Kong, Y. Nie, H. Zhang, M. Xiao and T. Gao, Enhanced coagulation of covalent composite coagulant with potassium
permanganate oxidation for algae laden water treatment: Algae and extracellular organic matter removal, Chem. Eng. J. Adv., 2023, 13, 100427 CrossRef CAS.
- C. Pinheiro, J. Azevedo, A. Campos, V. Vasconcelos and S. Loureiro, The interactive effects of microcystin-LR and cylindrospermopsin on the growth rate of the freshwater algae Chlorella vulgaris, Ecotoxicology, 2016, 25, 745–758 CrossRef CAS PubMed.
- M. Zhang, F. Kong, P. Xing and X. Tan, Effects of Interspecific Interactions between Microcystis aeruginosa and Chlorella pyrenoidosa on Their Growth and Physiology, Int. Rev. Hydrobiol., 2007, 92, 281–290 CrossRef.
-
USEPA, Standard Method 365.3 Phosphorous, All Forms (Colorimetric, Ascorbic Acid, Two Reagent), Cincinnati, OH, USA, 1978, pp. 3–7 Search PubMed.
-
USEPA, Standard Method 352.1: Nitrogen, Nitrate (Colorimetric, Brucine) by Spectrophotometer, Cincinnati, OH, USA, 1971, pp. 1–5 Search PubMed.
-
USEPA, Standard Method 551.1: Determination of Chlorination Disinfection Byproducts, Chlorinated Solvents, and Halogenated Pesticides/Herbicides in Drinking Water by Liquid-liquid Microextraction, Derivatization, and Gas Chomatography with Electron Capture Detec, Cincinnati, OH, USA, 1995 Search PubMed.
-
USEPA, Standard Method 552.3 Determination of Haloacetic Acids and Dalapon in Drinking Water by Liquid-Liquid Microextraction, Derivatization, and Gas Chromatography with Elctron Capture Detection, Technology Applications, Inc, Cincinnati, OH, USA, 2003 Search PubMed.
- P. Ratomski and M. Hawrot-Paw, Influence of Nutrient-Stress Conditions on Chlorella vulgaris Biomass Production and Lipid Content, Catalysts, 2021, 11, 573 CrossRef CAS.
- R. Jiang, L. Qin, S. Feng, D. Huang, Z. Wang and S. Zhu, The joint effect of ammonium and pH on the growth of Chlorella vulgaris and ammonium removal in artificial liquid digestate, Bioresour. Technol., 2021, 325, 124690 CrossRef CAS PubMed.
- A. Sukenik, W. Schröder, J. Lauer, G. Shelef and C. J. Soeder, Coprecipitation of microalgal biomass with calcium and phosphate ions, Water Res., 1985, 19, 127–129 CrossRef CAS.
- L. Liu, Q. Huang, Y. Zhang, B. Qin and G. Zhu, Excitation-emission matrix fluorescence and parallel factor analyses of the effects of N and P nutrients on the extracellular polymeric substances of Microcystis aeruginosa, Limnologica, 2017, 63, 18–26 CrossRef CAS.
- H. Xu, H. Cai, G. Yu and H. Jiang, Insights into extracellular polymeric substances of cyanobacterium Microcystis aeruginosa using fractionation procedure and parallel factor analysis, Water Res., 2013, 47, 2005–2014 CrossRef CAS PubMed.
- C.-C. Tang, X. Zhang, Z.-W. He, Y. Tian and X. C. Wang, Role of extracellular polymeric substances on nutrients storage and transfer in algal-bacteria symbiosis sludge system treating wastewater, Bioresour. Technol., 2021, 331, 125010 CrossRef CAS PubMed.
- R. Wang, S. Wang, R. Cao, J. Han, T. Huang and G. Wen, The apoptosis of Chlorella vulgaris and the release of intracellular organic matter under metalimnetic oxygen minimum conditions, Sci. Total Environ., 2024, 907, 168001 CrossRef CAS PubMed.
- F. Qu, H. Liang, Z. Wang, H. Wang, H. Yu and G. Li, Ultrafiltration membrane fouling by extracellular organic matters (EOM) of Microcystis aeruginosa in stationary phase: Influences of interfacial characteristics of foulants and fouling mechanisms, Water Res., 2012, 46, 1490–1500 CrossRef CAS PubMed.
- C.-Y. Chen and Y.-H. Chang, Engineering strategies for enhancing C. vulgaris ESP-31 lipid production using effluents of coke-making wastewater, J. Biosci. Bioeng., 2018, 125, 710–716 CrossRef CAS PubMed.
- M. Kajikawa and H. Fukuzawa, Algal Autophagy Is Necessary for the Regulation of Carbon Metabolism Under Nutrient Deficiency, Front. Plant Sci., 2020, 11, 36 CrossRef PubMed.
- Y. Guo, H. Meng, S. Zhao, Z. Wang, L. Zhu, D. Deng, J. Liu, H. He, W. Xie, G. Wang and L. Zhang, How does Microcystis aeruginosa respond to elevated temperature?, Sci. Total Environ., 2023, 889, 164277 CrossRef CAS PubMed.
- T. Hosseinabadi, R. Gharib, S. Salehian and M. Tabarzad, A Study on the Effect of Nitrate and Phosphate Concentrations on the Production of Mycosporine-Like
Amino Acids by Chlorella Vulgaris, Iran. J. Biotechnol., 2022, 20, 66–76 Search PubMed.
- F. Shuhaili, M. Segura-Noguera, Mathumathy, R. Vijayaraghavan, S. Thilagar, U. Lakshmanan, D. Prabaharan and S. Vaidyanathan, Nitrate and phosphate uptake dynamics in two halotolerant strains of Chlorella vulgaris is differentially influenced by carbon, nitrogen and phosphorus supply, Chem. Eng. J., 2023, 458, 141433 CrossRef CAS.
- W. Babiak and I. Krzemińska, Extracellular Polymeric Substances (EPS) as Microalgal Bioproducts: A Review of Factors Affecting EPS Synthesis and Application in Flocculation Processes, Energies, 2021, 14, 4007 CrossRef CAS.
- R. Dai, Z. Li, F. Yan, L. An, W. Du and X. Li, Evaluation of changes in M. aeruginosa growth and microcystin production under phosphorus starvation via transcriptomic surveys, Sci. Total Environ., 2023, 893, 164848 CrossRef CAS PubMed.
- W. Kong, S. Huang, F. Shi, J. Zhou, Y. Feng and Y. Xiao, Study on Microcystis aeruginosa growth in incubator experiments by combination of Logistic and Monod functions, Algal Res., 2018, 35, 602–612 CrossRef.
- E. Fernández-Pascual, B. Droz, J. O'Dwyer, C. O'Driscoll, E. H. Goslan, S. Harrison and J. Weatherill, Fluorescent Dissolved Organic Matter Components as Surrogates for Disinfection Byproduct Formation in Drinking Water: A Critical Review, ACS ES&T Water, 2023, 3, 1997–2008 Search PubMed.
- L. Liang, Z. Wang, Y. Ding, Y. Li and X. Wen, Protein reserves elucidate the growth of microalgae under nitrogen deficiency, Algal Res., 2023, 75, 103269 CrossRef.
- R. Gough, P. J. Holliman, G. M. Cooke and C. Freeman, Characterisation of algogenic organic matter during an algal bloom and its implications for trihalomethane formation, Sustainability Water Qual. Ecol., 2015, 6, 11–19 CrossRef.
- J.-L. Lin and A. R. Ika, Minimization of halogenated DBP precursors by enhanced PACl coagulation: The impact of organic molecule fraction changes on DBP precursors destabilization with Al hydrates, Sci. Total Environ., 2020, 703, 134936 CrossRef CAS PubMed.
|
This journal is © The Royal Society of Chemistry 2024 |
Click here to see how this site uses Cookies. View our privacy policy here.