Dye wastewater treatment and membrane fouling in a moving bed-UV-photocatalytically modified membrane bioreactor†
Received
4th June 2024
, Accepted 24th July 2024
First published on 24th July 2024
Abstract
A moving bed-UV-photocatalytically modified membrane bioreactor (MB-UVPMBR) system effectively removed organic matter, and the removal efficiency of Lanasol blue 3R (LB) reached 85.1%, which was significantly greater than that of a moving bed membrane bioreactor (MBMBR) system. The dye removal efficiency of the system was enhanced as a result of the degradation of LB by a polyvinylidene fluoride (PVDF)/TiO2-modified membrane under UV irradiation. An analysis of the membrane resistance distributions of the two systems revealed that the main cause of membrane fouling was the deposition of a cake layer on the membrane surface. Compared with the membrane in the MBMBR system, the membrane in the MB-UVPMBR system exhibited a 67.5% reduction in total filtration resistance, which was attributed to the hydrophilicity and photocatalytic properties of the PVDF/TiO2-modified material. Overall, the removal efficiency of organic pollutants in the MB-UVPMBR system was better than that in the MBMBR system.
Water impact
The experimental process presented in this journal article is conducted with utmost care and attention to its potential impact on water resources. The focus of our research is to advance scientific understanding in a manner that minimizes the adverse effects on water quality, quantity, and ecosystems.
|
1. Introduction
With increasing economic development and population growth, water pollution has become a major challenge.1,2 Dye wastewater is a type of wastewater containing pollutants such as heavy metal ions, organic matter and biological products.3,4 Dyes (hair and colour groups) are aromatic compounds with highly complex structures and are often highly resistant to photolytic, chemical and biological degradation.5,6 An ideal dye removal method should be effective at removing large amounts of dye in wastewater within a short period without generating secondary pollutants. At present, dye wastewater is treated via three common methods: biological treatment, physical treatment, and chemical treatment.7,8
In biological treatment methods, the metabolic action of microorganisms is utilized to degrade dissolved and colloidal organic pollutants in wastewater.9 The treatment efficiency of biological treatment methods is significantly affected by fluctuations in water quality, and the colour-removal and decomposition efficiencies are usually not high.10,11 Physical methods mainly transfer pollutants from the water to another phase through physical adsorption, without destroying the structure of the pollutants and easily causing secondary pollution.12 The chemical oxidation method uses a catalyst to generate strong oxidizing groups (hydroxyl radicals ·OH and superoxide radicals ·O2−) to oxidize and decompose organic pollutants. Owing to the complex composition, high toxicity, and high organic content of printing and dyeing wastewater, most of the organic compounds contained in wastewater are water soluble, and organic compounds containing chromophores are difficult to degrade. Therefore, in the treatment of wastewater, it is impossible to achieve the desired results by using only one of the methods mentioned above.
Membrane bioreactors (MBRs) combine activated sludge processes and membrane filtration processes and have become a popular research topic in China and other regions for wastewater treatment because of their high solid–liquid separation efficiency, good effluent quality, small footprint, and low level of sludge production. Many researchers have attempted to use this technology to treat printing and dyeing wastewater. However, membrane fouling and the large quantities of harmful pollutants that cannot be biodegraded in printing and dyeing wastewaters have become bottlenecks in the application of membrane bioreactors to treat printing and dyeing wastewaters.13,14
In the 1970s, Porter and Brandon et al.15 used membrane bioreactors to treat dye wastewater, treating 13 acidic and alkaline dye solutions via reverse osmosis technology. Spagni et al.16 used an anaerobic biofilter and a hypoxic aerobic membrane bioreactor to treat active orange 16, and the decolorization in the study was mostly performed under anaerobic conditions. Similarly, Khelifi et al.17 removed 97.3% of a dye under optimal conditions. Although these membrane bioreactors exhibited high treatment efficiency for dye wastewater, long hydraulic residence times were required for the decolorization and degradation of complex dye compounds, and membrane fouling also limited the widespread application of these reactors. Therefore, while conducting research on the application of membrane bioreactors in printing and dyeing wastewater treatment, scholars in China and other regions have also carried out much research on membrane fouling mechanisms and membrane resistance control.18,19
Polyvinylidene fluoride (PVDF) membranes are widely used in MBRs because of their excellent thermal stability, good chemical resistance, and film-forming performance. However, several problems associated with PVDF membranes still exist that limit their promotion and application, especially in aqueous solution purification and separation fields such as drinking water production, wastewater treatment, and biological separation. The key issues are as follows: (1) the surface energy and surface tension of PVDF in aqueous solutions are very low, which leads to poor wettability of PVDF membranes. Therefore, PVDF is strongly hydrophobic, and the pure-water flux is usually low. (2) Membrane fouling tends to occur when aqueous solutions containing natural organic compounds (such as proteins) are treated with hydrophobic PVDF membranes. Proteins tend to adsorb onto the membrane surface or clog membrane pores, reducing permeability, separation performance and membrane lifespan and ultimately increasing operational costs for membrane component replacement and maintenance. Therefore, increasing the hydrophilicity of PVDF membranes, improving the service life of PVDF membrane components, and reducing operating costs are important objectives. In recent years, many researchers have enhanced the hydrophilicity of PVDF membranes by introducing inorganic nanoparticles into them. At present, inorganic nanoparticles introduced into PVDF membranes mainly include SiO2,20 TiO2,21 Al2O3,22 ZnO,23 Mg(OH)2,24 carbon nanotubes, graphene oxide,25etc. Among them, TiO2 has received extensive attention in water or wastewater treatment due to its advantages such as stability, hydrophilicity and catalysis.
In our previous study, PVDF/TiO2-modified membranes were prepared via a layer-by-layer self-assembly method. The modified PVDF/TiO2 membrane showed good applicability in both static and membrane reactor experiments, providing a basis and reference for the practical application of this process in wastewater treatment. In our previous study, we reported that a novel moving bed-UV-photocatalytically modified membrane bioreactor (MB-UVPMBR) constructed with a PVDF/TiO2-modified membrane had good pollutant removal and membrane fouling mitigation performance in the treatment of simulated domestic sewage.26 This system showed excellent performance at the bench scale; the prepared PVDF/TiO2-modified membrane had photocatalytic activity under UV irradiation, could effectively remove refractory organic pollutants in water, and had a high degradation efficiency for Lanasol blue 3R (LB).27 In this study, to address problems associated with poor organic matter removal and poor fouling resistance during wastewater treatment, a PVDF/TiO2-modified membrane was tested in an MB-UVPMBR system, and the system performance and antifouling performance of the PVDF membrane and PVDF/TiO2-modified membrane were compared for treating printing and dyeing wastewater.
2. Experimental
2.1. Description of the setup and operating conditions
On the basis of its superior performance at the bench scale, a pilot study was carried out to provide a scientific basis and reference for the practical application of this process in dye wastewater treatment. The custom-made reactor consisted of 10 L of Plexiglas reactor, as shown in the schematic in Fig. 1. The characteristics of the wastewater were in line with those reported in earlier studies.28 A solution of LB (10 mg L−1) was added to the wastewater, and its concentration was determined via UV-vis spectroscopy at 587 nm. The fresh wastewater was first stabilized in a water storage tank for 24 hours before being transferred into the reactor as the influent via a flow pump. A perforated partition divided the reactor into two sections (2
:
1 v/v): the biocarrier compartment and the membrane module compartment. The biocarrier compartment of the reactor was filled with K1 carriers (30% v/v), and the membrane module compartment was filled with custom-made PVDF flat-sheet-modified membrane modules with an area of 10.0 cm × 15.0 cm. Two UV lamps (Yipin, China) were placed on either side of the modified membrane to irradiate the membrane surface. After 30 days of microbe acclimation, the initial concentration of mixed liquor suspended solids (MLSS) was 3.6 g L−1. The modified membrane was fabricated via a layer-by-layer (LbL) approach by coating TiO2/poly(sodium styrenesulfonate) (PSS) photocatalyst layers. In our previous study, a PVDF/TiO2-modified membrane was prepared via a layer-by-layer self-assembly method.27 The (PVDF/TiO2)7-modified membrane has demonstrated excellent versatility in both static experiments and membrane reactors, providing a scientific foundation and reference for the practical application of this technology in wastewater treatment. Therefore, in this study, a (PVDF/TiO2)7-modified membrane was used to run the MBMBR system, and its actual performance was tested. In the reactor, 1.5 L min−1 air was injected via an air pump, while the permeate was extracted intermittently (8 minutes on/2 minutes off) via suction pumps, maintaining a constant flux of 10 L m−2 h−1. The transmembrane pressure (TMP) indicates the resistance to membrane fouling and was tracked with a pressure gauge placed between the membrane module and peristaltic pump. The membranes were cleaned once the TMP reached 40 kPa.
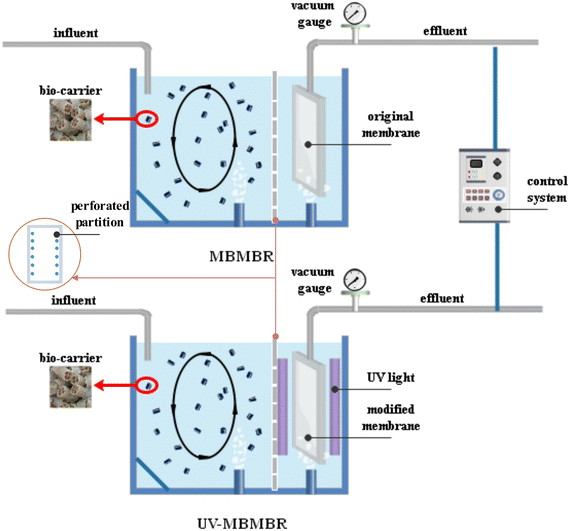 |
| Fig. 1 Schematic of the experimental process. | |
2.2. Experimental procedure and analytical methods
Two reactors, the MBMBR and the MB-UVPMBR, were operated in parallel for approximately 3 months under the same inlet conditions. The experimental operation was divided into two stages. In the first stage (stage I), both reactors were operated under no ultraviolet light irradiation, and the removal efficiency of pollutants and chromaticity, as well as membrane fouling, were compared between the two reactors. In the second stage (stage II), the operating conditions of the MBMBR remained the same, but ultraviolet light was added to the MB-UVPMBR system. The removal efficiency of organic matter and chromaticity, as well as the degree of membrane fouling, was compared between the two reactors. The specific inflow and operating conditions are shown in Table 1.
Table 1 Operational parameters in the experiment
Stage |
Days (d) |
TOCin (mg L−1) |
NH4+-Nin (mg L−1) |
TPin (mg L−1) |
LB (mg L−1) |
I |
1–41 |
139.3 ± 23.5 |
25.2 ± 2.5 |
13.4 ± 2.3 |
10.2 ± 1.4 |
II |
42–81 |
135.2 ± 27.4 |
23.9 ± 2.2 |
13.4 ± 2.9 |
10.3 ± 1.7 |
The compositions of extracellular polymeric substances (EPSs) in the cake layer and soluble microbial products (SMPs) were analysed to determine the dominant contributors to membrane fouling in each operation system. The EPSs in the cake layer were measured via the heating extraction method.29 Generally, proteins and carbohydrates are the main components of EPSs and SMPs. Thus, we used proteins and carbohydrates to characterize the total EPS and total SMP contents in this research. Standard analytical methods were used to measure the concentrations of proteins and carbohydrates.30 The analytical methods for all the parameters evaluated are given in the ESI.†
3. Results and discussion
3.1. Overall performance of the hybrid system
After 20 days of microorganism domestication in the prepared printing and dyeing wastewater, the biological fillers were divided into two reactors to maintain consistent sludge characteristics. Fig. 2a confirms that the MBMBR and MB-UVPMBR systems had high removal efficiencies for total organic carbon (TOC), with an average removal rate of more than 95% for the effluent TOC in both stages. The same results obtained in our previous study of TOC removal from domestic sewage were observed, which showed that adding 10 mg L−1 LB at a fixed concentration with trace elements in the influent did not change the metabolic activity of microorganisms for organic matter. In stage I, the average removal rates of TOC were 95.8% and 96.0%, and the average effluent TOC concentrations were 5.63 mg L−1 and 5.45 mg L−1, respectively. In stage II, the average removal rates of TOC were 95.4% and 97.6%, respectively, and the average effluent TOC concentrations were 5.86 mg L−1 and 3.02 mg L−1, respectively. The PVDF/TiO2-modified membrane in the MB-UVPMBR system slightly improved the removal rate of TOC in the reactor under UV photocatalytic conditions.
 |
| Fig. 2 Pollutants in the influent, supernatant and effluent in the MBMBR and MB-UVPMBR: (a) TOC, (b) NH4+-N, (c) TP, and (d) LB. | |
As shown in Fig. 2b, the NH4+-N concentrations in the influent of the two systems fluctuated between 19 and 28 mg L−1. The effluent quality of both systems was good, and the average removal rate of NH4+-N in both stages was greater than 92%. Owing to the efficient intercepting effect of the membrane module, the microorganisms attached to the surface of the carriers and all the microorganisms were trapped within the reactor; therefore, no sludge discharge was carried out during the operation of the reactor, creating good growth conditions for nitrifying bacteria with long generation times. This provided good conditions for the removal of NH4+-N in both systems; therefore, the removal of NH4+-N by both systems was significant. Similar to the removal efficiency of TOC, the average removal rates of NH4+-N in the MBMBR and MB-UVPMBR systems in stage I were 92.3% and 92.9%, respectively. In stage II, the removal rate of NH4+-N in the MBMBR system remained relatively unchanged compared with that in stage I, whereas the average removal rate of NH4+-N in the MB-UVPMBR system increased to 96.0%. A portion of the NH4+-N underwent degradation due to the photocatalytic activity of the PVDF/TiO2-modified membranes.
Fig. 2c shows the changes in the concentrations of total phosphorus (TP) in the influent and effluent of the two systems during stable operation, as well as the removal efficiency of TP. The average removal rate of TP in both the MBMBR and the MB-UVPMBR systems during the two stages of operation remained at approximately 69%. Similarly, the average removal rate of TP in the MB-UVPMBR system did not significantly differ between the two operating stages (69.3% and 69.1%, respectively), indicating that UV photocatalysis had no effect on TP removal. The TP removal efficiency of the two systems remained stable because of the close relationship between the TP removal efficiency and microbial activity.31 The removal of TP was achieved mainly by discharging sludge on a regular basis. Owing to the use of biofilm carriers in both reactors, the mass transfer resistance of substances increased with increasing biofilm thickness, gradually producing an aerobic layer on the outer surface and an anaerobic/oxygen-deficient layer on the inner surface with increasing biofilm depth.32 The internationally recognized and accepted theory of biological phosphorus removal via polyphosphate-accumulating microorganisms (PAOs) involves phosphorus uptake/release by PAOs and suggests that the biological phosphorus removal process generally includes two processes: anaerobic phosphorus release and aerobic phosphorus absorption.33,34 Therefore, the two processes can be carried out simultaneously on the biofilm, enhancing the overall phosphorus removal ability of the system.
The simulated printing and dyeing wastewater had a certain degree of chromaticity due to the presence of LB. As shown in Fig. 2d, the absorbance of the printing and dyeing wastewater treated with the two systems decreased to a certain extent. Throughout the entire experimental process, both systems exhibited high total LB removal efficiency (both greater than 76%). In stage I, the LB dye removal rates of the MBMBR and MB-UVPMBR systems were 75.6% and 79.1%, respectively. In this stage, owing to the smaller average pore size of the PVDF/TiO2-modified membrane than that of the PVDF membrane, the removal rate of LB dye in the MB-UVPMBR system was slightly greater. When the PVDF/TiO2-modified membrane was irradiated with ultraviolet light (stage II), the removal rate of LB dye in the MB-UVPMBR system increased to 85.1%. These findings indicate that the degradation of LB in both reactors occurred primarily through biological decomposition and that photocatalysis helped increase the rate of LB decomposition. In stage II of the MB-UVPMBR system, LB exhibited a high removal rate in the initial stage, with a maximum removal rate of 89.8%. After 63 days, the removal rate of the LB dye began to gradually decrease. During the period from the 63rd to the 80th days, the average removal rates of LB in the MBMBR and MB-UVPMBR systems were 77.31% and 84.15%, respectively. The MB-UVPMBR system achieved a high LB removal rate of 87.1% during the period from the 40th to the 62nd day. This may have occurred because pollutants gradually covered the membrane surface, resulting in a decrease in the number of exposed TiO2 active sites on the membrane surface and reducing the photocatalytic degradation ability of the PVDF/TiO2-modified membranes for LB dyes. This result indicated that the PVDF/TiO2-modified membrane catalysed the degradation of LB under UV irradiation.
3.2. Colour-removal performance of the hybrid system
Fig. 3a shows the treatment effects of the two systems in terms of effluent quality. Compared with that of the MBMBR system, the effluent colour of the synthetic printing and dyeing wastewater treated with the MB-UVPMBR system had less colour and was almost colourless, indicating that the LB in the influent was largely degraded. As shown in Fig. 3b, the total average removal rates (Rt) of LB during the start-up phase (stage I) of the MBMBR and MB-UVPMBR systems were 75.6% and 79.1%, respectively. The biological removal efficiencies (Re) of the two systems were essentially the same, at 67.9% and 67.0%, respectively. This indicates that approximately 33% LB remained in both systems, indicating that the characteristic pollutants of this type of LB dye are difficult to biodegrade. If strict control of the chromaticity of wastewater is desired, pretreatment or additional treatment procedures should be considered to achieve integrated water pollutant discharge standards. The membrane separation efficiency (Rs) of the MB-UVPMBR system was 36.3%, which was higher than that of the MBMBR system (24.0%). This occurred because the pore size of the modified membrane was smaller than that of the original membrane, which improved the separation efficiency of the modified membrane for macromolecular organic compounds. Moreover, we determined the modified membrane and original membrane pore size via two systems, as detailed in Fig. S2.† Therefore, membrane separation was an important factor in the overall removal of LB in the MB-UVPMBR system compared with that in the MBMBR system with no ultraviolet irradiation. Both systems had high membrane retention.
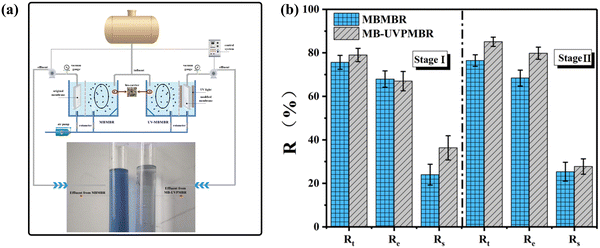 |
| Fig. 3 (a) Images of effluent from the MBMBR and MB-UVPMBR systems. (b) Average total removal efficiency, biodegradation efficiency, and average membrane separation efficiency of the pollutants in the MBMBR and MB-UVPMBR systems. | |
In stage II, ultraviolet light irradiation was applied, and the total average removal rates (Rt) of LB in the MBMBR and MB-UVPMBR systems were 78.8% and 82.2%, respectively. In particular, a slightly greater LB removal efficiency was found in the MB-UVPMBR system than in the traditional MBMBR system because of the influence of the photocatalytic activity of the modified membrane. Compared with those in stage I, the degradation and removal efficiency (Re) of LB in the MB-UVPMBR system increased to 79.8% in the reactor in stage II, indicating a significant decrease in the content of LB in the supernatant due to the photocatalytic effect of the PVDF/TiO2 membrane. The membrane separation efficiencies (Rs) of LB in the MBMBR and MB-UVPMBR systems were 25.4% and 27.7%, respectively. The experimental results confirmed that biodegradation was an important factor in achieving the removal of LB, and photocatalytic degradation could effectively improve the removal efficiency of difficult-to-biodegrade organic compounds.
3.3. Membrane fouling behaviour
3.3.1. Variation in transmembrane pressure.
The most obvious manifestation of membrane fouling during the operation of a membrane bioreactor is an increase in the TMP. To accurately determine the evolution of the membrane fouling process, continuous monitoring of the TMP over time was carried out under specific operating conditions. Fig. 4 shows the variation curves of the difference in transmembrane pressure over time in the MBMBR and MB-UVPMBR systems at different operating stages. For the MBMR system, the difference in TMP gradually increased during stage I. On the 8th day of operation, the TMP plateaued at approximately 29 kPa; that is, 29 kPa was the critical filtration pressure. When the TMP was less than 29 kPa, the difference in membrane filtration pressure gradually increased. When the TMP was greater than 29 kPa, the difference in filtration pressure rapidly increased. This occurred because the reverse diffusion rate of the sludge particles was not enough to offset the penetration rate, and the sludge deposited and accumulated on the surface of the membrane and rapidly formed a filter cake. The filter cake layer became the main barrier to filtration, and the filtration resistance generated by this layer was much greater than that generated by the membrane; therefore, the TMP increased rapidly.28,35 The difference in TMP plateaued on the 26th day of operation in the MB-UVPMBR system at 30 kPa, which meant that the critical filtration pressure of the MB-UVPMBR system was 30 kPa. This indicated that PVDF/TiO2-modified membranes can significantly reduce the membrane fouling rate due to the increased hydrophilicity of the modified membrane surface, which can reduce the deposition of sludge flocs on the membrane surface, resulting in a slow increase in the TMP over time. The rate of TMP increase in the MB-UVPMBR system was relatively low, and on the 35th day, the TMP reached 40 kPa, which was twice as long as that in the MBMBR system. Through chemical cleaning of the PVDF membranes and self-cleaning of the PVDF/TiO2-modified membranes,26 the TMP nearly recovered to the initial level, indicating that membrane pore blockage and cake layer fouling could be effectively reversed by cleaning.
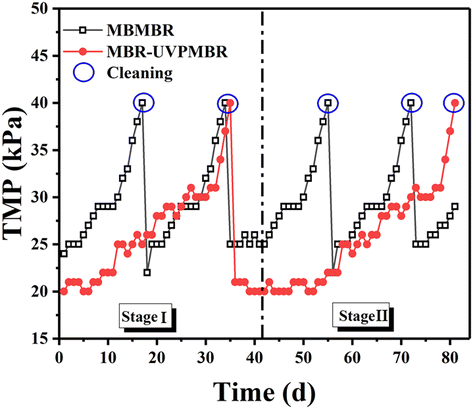 |
| Fig. 4 TMP variations in the MB-UVPMBR and MBMBR systems. | |
In stage II, due to the use of UV irradiation, the MB-UVPMBR system had a slower rate of increase in TMP than it did in stage I. The PVDF/TiO2-modified membrane significantly improved membrane fouling under UV irradiation conditions. The analysis suggested that this difference could be attributed mainly to differences in operating conditions. On the one hand, ultraviolet light degrades pollutants in reactors, resulting in a significant decrease in the membrane fouling rate. On the other hand, the modified membrane is more hydrophilic than the unmodified membrane and reduces the adhesion of pollutants on its surface. Furthermore, the modified membrane has photocatalytic properties and degrades pollutants that attach to the membrane surface, which is also beneficial for reducing membrane fouling.
3.3.2. Membrane characterization.
Fig. 5 shows digital photographs of the MBMBR and MB-UVPMBR systems before and after membrane fouling. The fouled membrane module was removed from the two reactors, and the morphology of the fouling layer (gel layer and cake layer) on the membrane surface was observed. The membrane surfaces of the two systems differed. The colour of the PVDF membrane surface changed from white to blue black, whereas that of the PVDF/TiO2-modified membrane changed to light blue. Compared with the membrane surface of the MB-UVPMBR system, the membrane surface and exposed support plate of the MBMBR were covered with blue viscous sludge, indicating that the membrane surface was covered with a filter cake layer.
 |
| Fig. 5 Images of fresh membranes and fouled membranes in the MBMBR and MB-UVPMBR systems. | |
Scanning electron microscopy was used to observe structural differences in the surface morphology of the fouled membrane. The fouled membrane was dried at room temperature and observed by scanning electron microscopy. During reactor operation, many microorganisms attach to the surface of the separation membrane, and through the adsorption and consumption of organic matter, the microorganisms grow and reproduce, leading to a gradual thickening of the biofilm on the membrane surface and a decrease in membrane flux. Studies have shown that microorganisms can secrete and form a gel layer on membrane surfaces.36 In Fig. 6, the SEM images of the fouled membrane surfaces in the MBMBR and MB-UVPMBR systems show that, compared with those in the new membrane, the surfaces of the fouled membrane were no longer smooth and uniform, and the membrane surface was covered by a filter cake layer. Clear membrane pores were still observed on the surface of the fouled modified membrane in the MB-UVPMBR system. In the MBMBR system, the surface of the PVDF membrane was covered by a filter cake layer. The deposition layer of foulants on the surface of the PVDF membrane was thick and dense at the layer fracture sites. This occurred because the PVDF/TiO2 membrane had high hydrophilicity, while the foulants on the membrane surface were mainly hydrophobic substances. The TiO2 on the surface of the modified membrane interacted with water molecules, forming a hydration layer on the membrane surface that prevented the adsorption of hydrophobic foulants on the membrane surface.37,38
 |
| Fig. 6 SEM images of fouled membranes in the MBMBR and MB-UVPMBR systems. | |
After the MBMBR and MB-UVPMBR systems were used to treat printing and dyeing wastewater, the membrane components were removed, and organic functional groups on the surface of the contaminated membrane were analysed via infrared spectroscopy, as shown in Fig. 7a and b. The peaks attributed to PVDF can be observed at 871, 1070, and 1400 cm−1, which are associated with –CH2 and –CF2.26,39 The curves of the original membrane and modified membrane were well superimposed at higher wavenumbers and deviated at lower wavenumbers. This deviation was attributed to the addition of TiO2.40 A comparison of the spectra of the new membranes in the two systems revealed that the spectrum of the contaminated membrane had a broad absorption peak at 3272 cm−1, which corresponded to the amide A band arising from the coupling of N–H stretching vibrations and O–H stretching vibrations.41 There was an asymmetric stretching vibration peak attributed to CH2 at 2923 cm−1.42 There were two absorption peaks in the spectrum at 1256 cm−1 and 1406 cm−1, corresponding to carbohydrates and lipids, respectively. There were two absorption peaks at 1635 cm−1 and 1542 cm−1, which were attributed to amino groups.29,43,44
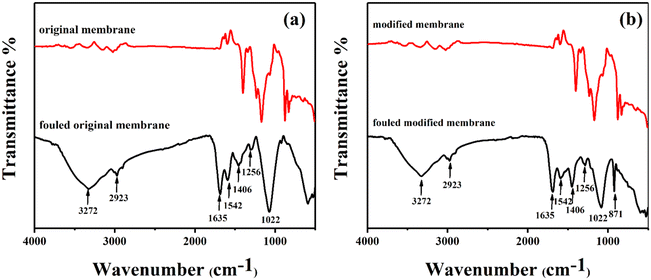 |
| Fig. 7 FTIR spectra of the fresh and fouled membrane surfaces: (a) original membranes in MBMBR, (b) modified membranes in MB-UVPMBR. | |
The presence of proteins, carbohydrates, and lipid substances on the membrane surface indicates severe organic pollution of the membrane. The intensities of the absorption peaks at 3272 cm−1, 2923 cm−1, 1635 cm−1, and 1542 cm−1 increased significantly because the LB structure contains an α-bromoacrylamide group; therefore, the absorption peaks of LB and protein overlapped at these points. Nevertheless, the wastewater samples used in this study did not contain an added F-containing component, and only –CF2 was present on the surface of the membrane. A significant absorption peak corresponding to CF2 was observed at 871 cm−1 only on the surface of the membrane from the MB-UVPMBR system,45 indicating that the PVDF/TiO2-modified membrane surface was not completely covered by foulants. The results confirmed that the PVDF/TiO2-modified membrane placed in the MB-UVPMBR system reduced the contents of proteins, carbohydrates, and LB adsorbed on the membrane surface through UV photocatalysis.
3.4. Membrane filtration resistance distribution
To analyse the main causes of membrane fouling in the MBMBR and MB-UVPMBR systems under different lighting conditions, the filtration resistance of the membranes was calculated. Fig. 8 shows a comparison of the membrane resistances of the two systems at different stages. Notably, under all the operating conditions, the filtration resistances, including the cake layer resistance (Rc), pore blockage resistance (Rp), membrane resistance (Rm), and total resistance (Rt), of the MB-UVPMBR system were lower than those of the MBMBR system. The detailed methods for testing and calculating the membrane filtration resistance are provided in the ESI.† The analysis revealed that in the MBMBR system, the membrane resistance Rm accounted for only a small proportion of the total resistance Rt, with that in stage I accounting for 11.4% and that in stage II accounting for 12.0%. However, the resistance Rc formed by the filter cake layer during the filtration process was much greater than that in stage I and stage II, with resistance contributions of 65.4% and 64.6%, respectively. The main cause of membrane fouling in both systems was the deposition of a filter cake layer.
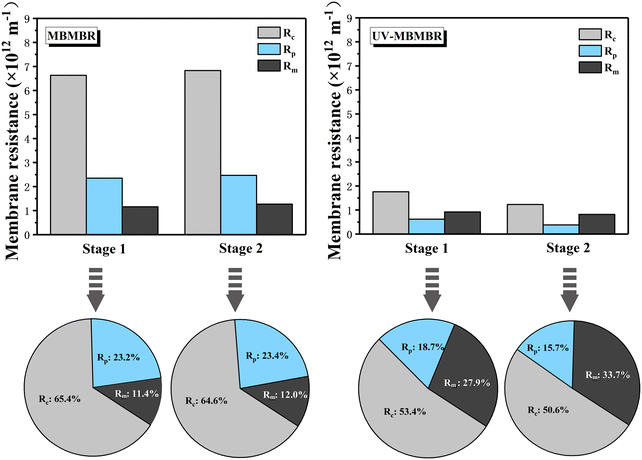 |
| Fig. 8 Comparison of the resistance contributions in the MBMBR and MB-UVPMBR systems. | |
In stage I, the Rm in the MBMBR system was 1.61 × 1012 m−1, accounting for 11.4% of the Rt, and the Rm in the MB-UVPMBR system was 0.92 × 1012 m−1, accounting for 27.9% of the Rt. Although the Rm of the MB-UVPMBR system decreased, its proportion in Rt significantly increased. This difference may have occurred not only because of the decrease in the average pore size of the PVDF/TiO2-modified membrane but also because of the increase in its hydrophilicity, which resulted in a decrease in Rm. Additionally, compared with that of the MBMBR system, the total resistance of Rm increased to 27.9% in the MB-UVPMBR system, which could explain the significant decrease in the total resistance. Compared with those of Rm, the Rc and Rp of the MB-UVPMBR system decreased significantly in stage I. In stage I, the total resistance Rt of the MBMBR system was 10.14 × 1012 m−1, and the Rt of the MB-UVPMBR system was only 3.3 × 1012 m−1. In our previous study, to verify the hydrophobicity of the membrane surface, the water contact angle (WCA) was measured.27 The WCA of the original membrane was 94.96°, and a substantial decrease (to 43.12°) was observed after surface modification of the MB-UVPMBR system. This result indicated that the PVDF/TiO2-modified membrane had a lower total membrane filtration resistance in the MB-UVPMBR system during stage I because of its superhydrophilicity.
In stage II, compared with that in the MBMBR system, the filtration resistance (Rc) of the cake layer in the MB-UVPMBR system was significantly lower. The digital photo (Fig. 5) and SEM image (Fig. 6) clearly reveal that after UV irradiation, only a thin layer of foulants was deposited on the surface of the MB-UVPMBR system. Almost all the membrane surfaces in the MB-UVPMBR system were covered by a dense cake layer. In stage II of the MB-UVPMBR system, both Rc and Rp decreased due to UV photocatalysis. The experimental results further indicated that the superhydrophilicity and photocatalytic activity of the membrane components played important roles in preventing the formation of cake layers and reducing pore blockage on the membrane surface.
3.5. The effect of mixed liquor on membrane fouling
3.5.1. Cake layer.
During the operation of membrane bioreactor, bioactive microorganisms will attach to the membrane and grow to form biofilm, which will lead to biofilm fouling. The EPS secreted by microorganisms of macromolecules (such as proteins, humic acids and carbohydrates) in the mixture will adsorb on the surface of the membrane, forming a gel layer to reduce the permeable flux of the membrane. As shown in Fig. 9, EPSs, which included proteins and carbohydrates, in the filter cake layer were analysed. In stage I, the filter cake layer in the MBMBR system contained a large amount of EPSs, with 17.7 mg m−2 protein and 27.5 mg m−2 carbohydrate, which led to high filtration resistance in the membrane. The protein and carbohydrate concentrations in the MB-UVPMBR system were only 2.8 mg m−2 and 4.9 mg m−2, respectively, which were significantly lower than those in the MBMBR system. Therefore, these results support the conclusion that the MB-UVPMBR system mainly reduced membrane fouling by reducing the formation of filter cake layers. EPSs can cause severe cake layer resistance through the formation of a dense gel layer on the membrane surface. Most proteins and carbohydrates are hydrophobic substances. Owing to the superhydrophilicity of the PVDF/TiO2 membranes, the adsorption of proteins and carbohydrates on the membrane surface was hindered, which resulted in a significant decrease in EPSs on the membrane surface in the MB-UVPMBR system.
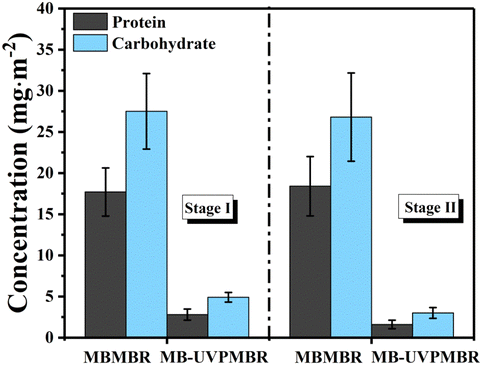 |
| Fig. 9 Comparison of EPSs in cake layer substances in the MBMBR and MB-UVPMBR systems. | |
Compared with stage I, the increased UV irradiation in stage II resulted in a decrease in the protein and sugar contents in the MB-UVPMBR system due to a slight decrease in EPSs. This finding indicates that proteins and carbohydrates attached to the membrane surface were further degraded via photocatalysis, leading to the alleviation of membrane fouling. However, the addition of TiO2 nanoparticles changed the biochemical properties of the mixed solution in the reactor, adding additional complexity to the ability of the PVDF/TiO2-modified membranes to alleviate membrane fouling; therefore, specific analyses were conducted to evaluate this effect in the PVDF/TiO2-modified membranes.
3.5.2. Characteristics of mixed liquids.
Owing to the poor solubility of SMPs, they easily adsorb to membrane pore walls and block membrane pores, leading to internal fouling of the membranes. Yamato et al.46 conducted MBR pilot experiments using urban wastewater to investigate the degree of fouling and the foulants present on two different membranes, one made of polyethylene (PE) and the other made of PVDF. Similar results were reported, indicating that SMPs contributed significantly to the internal resistance of fouled PVDF membranes. As shown in Fig. 10, during stage I, the carbohydrate content in the SMPs in the MBMBR system varied between 0 mg L−1 and 6.9 mg L−1, with an average carbohydrate content of 3.1 mg L−1. In the MB-UVPMBR system, the carbohydrate concentration ranged between 0 mg L−1 and 6.3 mg L−1, with an average value of 3 mg L−1. The protein content in the SMPs varied between 0 mg L−1 and 4.5 mg L−1 in the MBMBR system, with an average value of 1.7 mg L−1. The MB-UVPMBR system yielded values between 0 mg L−1 and 4.8 mg L−1, with an average value of 1.5 mg L−1. The SMP content of the two systems was essentially the same in stage I. In stage II, in the MBMBR system, the average carbohydrate content in the SMPs was 2.8 mg L−1. The average carbohydrate content in the MB-UVPMBR system was 1.9 mg L−1. In the MBMBR system, the average protein content in the SMPs was 1.8 mg L−1. The average protein content in the MB-UVPMBR system was only 0.9 mg L−1. Under irradiation with UV light, the concentrations of carbohydrates and proteins in the SMPs significantly decreased. The PVDF/TiO2-modified membrane in the MB-UVPMBR system degraded some carbohydrates and proteins in the SMPs under UV irradiation, reducing membrane pore blockage and slowing membrane fouling.
 |
| Fig. 10 Comparison of SMP substances in the MBMBR and MB-UVPMBR systems. | |
To confirm that internal fouling of the membrane pores occurred, cross-sections of fouled membranes from the MBMBR and MB-UVPMBR systems used to treat printing and dyeing wastewater were analysed via a scanning electron microscope. As shown in Fig. 11c and d, foulants were attached to the internal framework of the membranes in both systems. Fig. 11c shows a scanning electron microscopy image of a cross-section of a PVDF membrane fouled in the MBMBR system. As shown in the figure, many foulants were deposited in the internal skeleton of the membrane, and most of the membrane pores were blocked. In comparison, as shown in Fig. 11d, the PVDF/TiO2-modified membrane from the MB-UVPMBR system had scattered deposits of foulants inside the pores, and most of the internal structure of the membranes was still clearly visible. This indicated that irradiation with ultraviolet light in the MB-UVPMBR system reduced the degree of internal fouling of the PVDF/TiO2-modified membranes.
 |
| Fig. 11 Cross-sectional views of fouled membranes in the MBMBR and MB-UVPMBR systems. | |
4. Conclusions
An MB-UVPMBR system was used to treat simulated printing and dyeing wastewater, and the average removal rates of total organic carbon (TOC), NH4+-N, and total phosphorus (TP) in the effluent were 97.6%, 96.0%, and 69.1%, respectively. When the PVDF/TiO2-modified membrane was irradiated with ultraviolet light, the removal rate of LB dye in the MB-UVPMBR system increased to 85.1%. The membrane resistance results revealed that membrane fouling in the MB-UVPMBR system occurred mainly via the formation of a filter cake layer. Compared with the MBMBR system, the hydrophilicity and photocatalytic activity of the PVDF/TiO2-modified membranes reduced the total resistance of the MB-UVPMBR system by 67.5%, with significant decreases in the cake layer resistance and membrane pore blockage resistance.
Data availability
The data supporting the findings of this study are available within the article and its ESI.†
Author contributions
Jing Luo: conceptualization, investigation, writing – original draft, project administration. Tingxi Liu: investigation. Weiwei Chen: experiments, dynamic simulation. Caixia Dong: investigation, corresponding analysis. Jianguo Liu: conceptualization, supervision, project administration.
Conflicts of interest
There are no conflicts to declare.
Acknowledgements
The authors thank the Inner Mongolia University of Technology (No. BS2021045) and the Inner Mongolia Natural Science Foundation (No. 2021LHBS02001) for their support.
Notes and references
-
V. K. Chaturvedi, A. Kushwaha, S. Maurya, N. Tabassum, H. Chaurasia and M. P. Singh, Wastewater treatment through nanotechnology: Role and prospects, in Restoration of Wetland Ecosystem: A Trajectory Towards a Sustainable Environment, ed. A. K. Upadhyay, R. Singh and D. P. Singh, Springer Singapore, Singapore, 2020, pp. 227–247 Search PubMed
.
- M. R. Wang, B. L. Bodirsky, R. Rijneveld, F. Beier, M. P. Bak, M. Batool, B. Droppers, A. Popp, M. T. H. van Vliet and M. Strokal, A triple increase in global river basins with water scarcity due to future pollution, Nat. Commun., 2024, 15, 880 CrossRef CAS PubMed
.
- S. Gu, Y. Ma, T. Zhang, Y. Yang, Y. Xu and J. Li, MXene nanosheet tailored bioinspired modification of a nanofiltration membrane for dye/salt separation, ACS ES&T Water, 2023, 3, 1756–1766 Search PubMed
.
- Y. L. Song, L. J. Wang, X. Qiang, W. H. Gu, Z. L. Ma and G. C. Wang, An overview of biological mechanisms and strategies for treating wastewater from printing and dyeing processes, J. Water Process Eng., 2023, 55, 104242 CrossRef
.
- X. Hong, F. Jiao and W. Liu, Review on dyeing wastewater treatment technology, Journal of Central South University of Science and Technology, 2023, 54, 1219–1229 Search PubMed
.
- A. P. Periyasamy, Recent advances in the remediation of textile-dye-containing wastewater: Prioritizing human health and sustainable wastewater treatment, Sustainability, 2024, 16, 495 CrossRef CAS
.
- P. Moradihamedani, Recent advances in dye removal from wastewater by membrane technology: A review, Polym. Bull., 2022, 79, 2603–2631 CrossRef CAS
.
- G. George, A. M. Ealias and M. P. Saravanakumar, Advancements in textile dye removal: A critical review of layered double hydroxides and clay minerals as efficient adsorbents, Environ. Sci. Pollut. Res., 2024, 31, 12748–12779 CrossRef CAS PubMed
.
- L. Chen, Z. Meihan, S. Chengwen, T. Ping, S. Menghan, S. Mihua and W. Tonghua, Enhanced treatment ability of membrane technology by integrating an electric field for dye wastewater treatment: A review, J. AOAC Int., 2018, 101, 1341–1352 CrossRef PubMed
.
- C. Thamaraiselvan, N. Michael and Y. Oren, Selective separation of dyes and brine recovery from textile wastewater by nanofiltration membranes, Chem. Eng. Technol., 2018, 41, 185–293 CrossRef CAS
.
- V. K. Gupta and Suhas, Application of low-cost adsorbents for dye removal – A review, J. Environ. Manage., 2009, 90, 2313–2342 CrossRef CAS PubMed
.
- W. Z. Song, M. Zhang, H. J. Qiu, C. L. Li, T. Chen, L. L. Jiang, M. Yu, S. Ramakrishna, Z. L. Wang and Y. Z. Long, Insulator polymers achieve efficient catalysis under visible light due to contact electrification, Water Res., 2022, 226, 119242 CrossRef CAS PubMed
.
- Y. C. Su, C. Huang, J. R. Pan, W. P. Hsieh and M. C. Chu, Fouling mitigation by composite membrane in membrane bioreactors, J. Environ. Eng., 2012, 138, 344–350 CrossRef CAS
.
- P. Krzeminski, L. Leverette, S. Malamis and E. Katsou, Membrane bioreactors – A review on recent developments in energy reduction, fouling control, novel configurations, LCA and market prospects, J. Membr. Sci., 2017, 527, 207–227 CrossRef CAS
.
- J. J. Porter and C. Brandon, Zero discharge as exemplified by textile dyeing and finishing, Journal of Chemtech, 1976, 6, 402–407 CAS
.
- A. Spagni, S. Grilli, S. Casu and D. Mattioli, Treatment of a simulated textile wastewater containing the azo-dye reactive orange 16 in an anaerobic-biofilm anoxic–aerobic membrane bioreactor, Int. Biodeterior. Biodegrad., 2010, 64, 676–681 CrossRef CAS
.
- E. Khelifi, H. Gannoun, Y. Touhami, H. Bouallagui and M. Hamdi, Aerobic decolourization of the indigo dye-containing textile wastewater using continuous combined bioreactors, J. Hazard. Mater., 2008, 152, 683–689 CrossRef CAS PubMed
.
- Y. Yang, S. Qiao, R. Jin, J. Zhou and X. Quan, A novel aerobic electrochemical membrane bioreactor with CNTs hollow fiber membrane by electrochemical oxidation to improve water quality and mitigate membrane fouling, Water Res., 2019, 151, 54–63 CrossRef CAS PubMed
.
- M. Sun, I. Zucker, D. M. Davenport, X. Zhou, J. Qu and M. Elimelech, Reactive, self-cleaning ultrafiltration membrane functionalized with iron oxychloride nanocatalysts, Environ. Sci. Technol., 2018, 52, 8674–8683 CrossRef CAS PubMed
.
- N. A. Hashim, Y. Liu and K. Li, Preparation of PVDF Hollow Fiber Membranes Using SiO2 Particles: The Effect of Acid and Alkali Treatment on the Membrane Performances, Ind. Eng. Chem. Res., 2011, 50(5), 3035–3040 CrossRef CAS
.
- F. Shi, Y. Ma, J. Ma, P. Wang and W. Sun, Preparation and characterization of PVDF/TiO2 hybrid membranes with different dosage of nano-TiO2, J. Membr. Sci., 2012, 389, 522–531 CrossRef CAS
.
- A. Talavari, B. Ghanavati, A. Azimi and S. Sayyahi, Preparation and characterization of PVDF-filled MWCNT hollow fiber mixed matrix membranes for gas absorption by Al2O3 nanofluid absorbent via gas–liquid membrane contactor, Chem. Eng. Res. Des., 2020, 156, 478–494 CrossRef CAS
.
- B. J. Deka, J. Guo, N. K. Khanzada and A. K. An, Omniphobic re-entrant PVDF membrane with ZnO nanoparticles composite for desalination of low surface tension oily seawater, Water Res., 2019, 165, 114982 CrossRef CAS PubMed
.
- C. Dong, Y. Dai, S. Jiang and G. He, Application of Mg(OH)2 nanoplatelets as pore former to prepare PVDF ultrafiltration membranes, J. Environ. Chem. Eng., 2017, 5(1), 877–883 CrossRef CAS
.
- J.-X. Zhang and W. P. Maddison, Molecular phylogeny, divergence times and biogeography of spiders of the subfamily Euophryinae (Araneae: Salticidae), Mol. Phylogenet. Evol., 2013, 68(1), 81–92 CrossRef PubMed
.
- J. Luo, W. Chen, H. Song and J. Liu, Antifouling behaviour of a photocatalytic modified membrane in a moving bed bioreactor for wastewater treatment, J. Cleaner Prod., 2020, 256, 120381 CrossRef CAS
.
- J. Luo, W. Chen, H. Song and J. Liu, Fabrication of hierarchical layer-by-layer membrane as the photocatalytic degradation of foulants and effective mitigation of membrane fouling for wastewater treatment, Sci. Total Environ., 2020, 699, 134398 CrossRef CAS PubMed
.
- W. Chen, Y. L. Liu and J. R. Liu, Selecting aeration in a PVDF flat-sheet membrane bioreactor for municipal wastewater treatment, Desalin. Water Treat., 2015, 57, 1–9 Search PubMed
.
- S. Comte, G. Guibaud and M. Baudu, Relations between extraction protocols for activated sludge extracellular polymeric substances (EPS) and complexation properties of Pb and Cd with EPS: Part II. Consequences of EPS extraction methods on Pb2+ and Cd2+ complexation, Enzyme Microb. Technol., 2006, 38, 246–252 CrossRef CAS
.
- M. Yao, K. Zhang and L. Cui, Characterization of protein–polysaccharide ratios on membrane fouling, Desalination, 2010, 259, 11–16 CrossRef CAS
.
- Z. Xiong, C. Yinguang and W. Rui, Long-term effects of titanium dioxide nanoparticles on nitrogen and phosphorus removal from wastewater and bacterial community shift in activated sludge, Environ. Sci. Technol., 2011, 45, 7284 CrossRef PubMed
.
- C. Rangarajan and P. Mishra, Simultaneous nitrification and denitrification in biofilters with real time aeration control, Water Sci. Technol., 2001, 43, 269–276 Search PubMed
.
- E. R. Coats, D. L. Watkins and D. C. V. Kranenburg, A comparative environmental life-cycle analysis for removing phosphorus from wastewater: Biological versus physical/chemical processes, Water Environ. Res., 2011, 83, 750–760 CrossRef CAS PubMed
.
- E. R. Coats, F. J. Appel, N. M. Guho, C. K. Brinkman and J. Mellin, Interrogating the performance and microbial ecology of an enhanced biological phosphorus removal/post-anoxic denitrification process at bench and pilot scales, Water Environ. Res., 2023, 95, e10852 CrossRef CAS PubMed
.
- C. H. Wei, X. Huang, R. Ben Aim, K. Yamamoto and G. Amy, Critical flux
and chemical cleaning-in-place during the long-term operation of a pilot-scale submerged membrane bioreactor for municipal wastewater treatment, Water Res., 2011, 45, 863–871 CrossRef CAS PubMed
.
- B. Mahendran, H. J. Lin, B. Q. Liao and S. N. Liss, Surface properties of biofouled membranes from a submerged anaerobic membrane bioreactor after cleaning, J. Environ. Eng., 2011, 137, 504–513 CrossRef CAS
.
- X. Shi, G. Tal, N. P. Hankins and V. Gitis, Fouling and cleaning of ultrafiltration membranes: A review, J. Water Process Eng., 2014, 1, 121–138 CrossRef
.
- U. I. Gaya and A. H. Abdullah, Heterogeneous photocatalytic degradation of organic contaminants over titanium dioxide: A review of fundamentals, progress and problems, J. Photochem. Photobiol., C, 2008, 9, 1–12 CrossRef CAS
.
- S. J. Xu, G. E. Chen and Z. L. Xu, Excellent anti-fouling performance of PVDF polymeric membrane modified by enhanced CaA gel-layer, J. Ind. Eng. Chem., 2017, 58, 179–188 CrossRef
.
- F. Shi, Y. Ma, J. Ma, P. Wang and W. Sun, Preparation and characterization of PVDF/TiO2 hybrid membranes with different dosage of nano-TiO2, J. Membr. Sci., 2012, 389, 522–531 CrossRef CAS
.
- F. Xie, W. Chen, J. Wang and J. Liu, Fouling characteristics and enhancement mechanisms in a submerged flat-sheet membrane bioreactor equipped with micro-channel turbulence promoters with micro-pores, J. Membr. Sci., 2015, 495, 361–371 CrossRef CAS
.
- F. Meng, F. Yang, B. Shi and H. Zhang, A comprehensive study on membrane fouling in submerged membrane bioreactors operated under different aeration intensities, Sep. Purif. Technol., 2008, 59, 91–100 CrossRef CAS
.
- S. Simon, B. Pairo, M. Villain, P. D'Abzac, E. Van Hullebusch, P. Lens and G. Guibaud, Evaluation of size exclusion chromatography (SEC) for the characterization of extracellular polymeric substances (EPS) in anaerobic granular sludges, Bioresour. Technol., 2009, 100, 6258–6268 CrossRef CAS PubMed
.
- K. Nouha, R. S. Kumar, S. Balasubramanian and R. D. Tyagi, Critical review of EPS production, synthesis and composition for sludge flocculation, J. Environ. Sci., 2018, 66, 225–245 CrossRef CAS PubMed
.
- I. H. Shin, S. Hong, S. J. Lim, Y.-S. Son and T.-H. Kim, Surface modification of PVDF membrane by radiation-induced graft polymerization for novel membrane bioreactor, J. Ind. Eng. Chem., 2017, 46, 103–110 CrossRef CAS
.
- N. Yamato, K. Kimura, T. Miyoshi and Y. Watanabe, Difference in membrane fouling in membrane bioreactors (MBRs) caused by membrane polymer materials, J. Membr. Sci., 2006, 280, 911–919 CrossRef CAS
.
|
This journal is © The Royal Society of Chemistry 2024 |
Click here to see how this site uses Cookies. View our privacy policy here.