Evaluation of high-pressure homogenization as a pretreatment for the extraction and drying of 6-gingerol from ginger
Received
19th December 2023
, Accepted 24th March 2024
First published on 8th April 2024
Abstract
6-Gingerol is the major biologically active component found in the ginger rhizome, and this study investigated the effect of high-pressure homogenization pretreatment on the recovery of 6-gingerol. The smallest particle size of ginger suspension was achieved using high-pressure homogenization treatment at 100 MPa for 10 cycles. The total phenolic content (TPC) and antioxidant activity of ginger supernatants were enhanced at higher intensity of high-pressure homogenization pretreatment. At 50 MPa and 100 MPa of 1 cycle of high-pressure homogenization, the recovery of 6-gingerol content was increased by 112.9% and 79.1%, respectively. The high-pressure homogenization-treated and control ginger extracts obtained from ultrasound-assisted extraction and Soxhlet extraction were dried by various methods. All pretreated high-pressure homogenization samples showed a significantly higher 6-gingerol content than control samples. Using Soxhlet and ultrasound-assisted extractions, pretreated freeze-dried high-pressure homogenization ginger extracts demonstrated the highest antioxidant activity. In contrast, pretreated oven-dried high-pressure homogenization ginger extracts exhibited the highest TPC. This study demonstrated that high-pressure homogenization is a potential pretreatment method combined with various extraction and drying techniques for the efficient recovery of 6-gingerol from the rhizome of ginger.
Sustainability spotlight
The rhizome of ginger (Zingiber officinale Roscoe) contains bioactive gingerols with 6-gingerol as the most prominent biologically active component. 6-Gingerol exhibits a wide range of biological activities. This study evaluated the effect of high-pressure homogenization and the optimum extraction and drying combinations for the extraction of 6-gingerol from the rhizome of ginger. High-pressure homogenization increased the extraction of 6-gingerol from the rhizome of ginger. This study revealed the way for sustainable consumption of ginger as a natural source of 6-gingerol by ensuring resource efficiency and healthy food consumption. This study aligns with United Nations' Sustainable Development Goals 12 (responsible consumption and production), 3 (health and well-being) and 9 (industry and innovation).
|
1. Introduction
6-Gingerol [5-hydroxy-1-(4-hydroxy-3methoxyphenyl)decan-3-one] is the most prominent and active type of gingerol found in the rhizome of ginger (Zingiber officinale Roscoe). Ginger is a widely used spice and medicine all over the world.1 6-Gingerol demonstrates a wide range of pharmacological activities, such as antioxidant, antibacterial, antitumor, anti-inflammation, antidiabetic and hypolipidemic activities.2 6-Gingerol shows thermal instability and poor water solubility that limit its recovery during the extraction process.1 Besides traditional extraction methods, including solvent extraction, Soxhlet extraction, and maceration, novel extraction techniques such as supercritical extraction, ultrasound, enzyme, and microwave-assisted extractions have been applied to recover 6-gingerol from ginger rhizomes.3 Most recently, scientists have paid more attention to using green extraction technologies that consume raw materials more effectively, avoiding toxic solvents.4
Several pretreatment methods are applied before extraction processes to maximize the recovery of bioactive compounds from plant materials. Various physical, biological, chemical and physicochemical pretreatment methods are employed to enhance the extractability of bioactive compounds in different manners.5,6 Previous studies discovered that pretreatment techniques including particle size reduction, alkali, acid and thermal methods improve the microstructure of plant materials and increase the yield of the extraction process.7 High-pressure homogenization is a green pretreatment technology applied for multiple purposes in the food industry.8 High-pressure homogenization is an easily scalable continuous flow process that employs intense mechanical stresses induced by shear stress, cavitation, turbulence, and collision.9 High-pressure homogenization technology generates small and uniform particle sizes by cell disruption, inducing the release of intracellular compounds from plant materials.10 Recently, some research studies have investigated the impact of non-thermal and solvent-free high-pressure homogenization treatment on the physical properties of juices or suspensions.11 Accordingly, Preece et al.12 demonstrated reduction of particle sizes in a soybean slurry at 100 MPa after 1 cycle of high-pressure homogenization treatment. Yu et al.9 also indicated that particle sizes of taro pulp decreased with the increasing pressure of high-pressure homogenization. High-pressure homogenization significantly induced the non-selective release of all intracellular compounds, including proteins, carbohydrates, etc., from microalgae.10 Xing et al.13 demonstrated a higher sulforaphane recovery from broccoli seeds after high-pressure homogenization treatment due to cell disruption and an improvement in the mass transfer rate.
The impact of high-pressure homogenization depends on the cell structure, nature of the plant material, intracellular compounds, and high-pressure homogenization treatment conditions. High-pressure homogenization operates at ambient or moderately elevated temperatures with lower energy consumption than traditional thermal processing.14 Therefore, lower energy consumption leads to reduced greenhouse gas emissions and lower environmental impact during the high-pressure homogenization process.14 Furthermore, the enhanced extraction yield by the high-pressure homogenization process could minimize the wastage of raw materials by efficient recovery of intracellular compounds.15 These factors contribute to food sustainability using high-pressure homogenization. Although several studies have explored the potential of applying high-pressure homogenization treatment on several raw materials, reports focusing on the effect of high-pressure homogenization pretreatment on the suspension made from the rhizome of ginger have not been reported. The evaluation of the bioactivity of ginger suspensions pretreated with high-pressure homogenization followed by various extraction and drying combinations emphasizes the novel approach of this study. Therefore, the purpose of this study was to assess the impact of high-pressure homogenization on the particle size distribution and recovery of 6-gingerol from ginger using an aqueous solvent. The influence of high-pressure homogenization pretreatment combined with various extraction methods (ultrasound-assisted and Soxhlet extractions) and drying methods (vacuum drying, oven drying, and freeze drying) on the total phenolic content, 6-gingerol content, and antioxidant activity of ginger extracts was further investigated.
2. Materials and methods
2.1. Materials and chemicals
Fresh ginger rhizomes were acquired from Johor, Malaysia (1° 31′ 39.1764′′ N latitude and 103° 44′ 43.7136′′ E longitude). Methanol (HPLC grade (≥99.9%)) and analytical grade (≥99.8%)) were supplied from Merck (Darmstadt, Germany). Ethanol (analytical grade (99.5%)) was ordered from Fisher Scientific (Loughborough, UK). Ascorbic acid, 2,2-diphenyl-1-picrylhydrazyl (DPPH), ferric chloride, Folin Ciocalteu reagent, gallic acid, potassium ferricyanide, sodium carbonate anhydrous, sodium hydroxide and trichloroacetic acid were obtained from Sigma-Aldrich (St. Louis, USA).
2.2. Sample preparation and pretreatment
Fresh ginger rhizomes were cleaned with running water and peeled and cut into small pieces. After that, water was added to the small slices of ginger to get a final concentration of 10% (w/v), and a commercial blender (Waring MX1100XT11CE, USA) was used for blending for 8 min at low speed. Then, the obtained ginger suspension was sieved with a 200 μm mesh. After the filtering step, half of the ginger suspension was labeled as the control ginger suspension, and the remaining ginger suspension was subjected to high-pressure homogenization pretreatment using a high-pressure homogenizer (Panda Plus, 2000; GEA Niro Soavi, Parma, Italy) according to the method of Vichakshana et al.16 The ginger suspensions were collected after 1, 5, or 10 cycles at various pressures (50 MPa or 100 MPa) of the high-pressure homogenization. These conditions were selected based on the capacity of the equipment and the typical high-pressure homogenization cycle used.
2.3. Determination of the effect of various high-pressure homogenization processing conditions on the physicochemical characteristics of ginger samples
2.3.1. Particle size measurement.
The particle size distribution of ginger suspensions was measured by laser scattering using a Mastersizer 3000 (Malvern Instruments, UK). The particle size distributions were expressed in terms of the characteristic volume mean diameter d (4,3), diameters of particles at which 90% diameter (0.9), 50% diameter (0.5), and 10% diameter (0.1) of particles are by volume.
2.3.2. Scanning electron microscopy.
Scanning electron microscopy (HITACHI SU8010, Japan) was used to determine the surface morphology of ginger samples. The control and high-pressure homogenization-treated ginger suspensions were vacuum-dried, and ginger samples were placed on aluminum stubs, which contained double-sided carbon adhesive tabs. Sputter coating was performed with a gold-palladium thin layer using a sputter coater (Quorum Q150R S, UK). Then, scanning electron microscopic views of the samples were observed.
2.3.3. 6-Gingerol content, antioxidant activity, and total phenolics of aqueous ginger supernatants.
First, 40 mL of each ginger suspension were centrifuged at 15 °C and 2500×g for 10 min using a laboratory centrifuge (5810 R model, Eppendorf, Hamburg, Germany) and then vacuum filtered to obtain aqueous ginger supernatants. Then, a portion of the supernatant was used to analyze the total phenolic content and antioxidant activity according to the methods described in 2.6.3 and 2.6.4 respectively. For high-performance liquid chromatography (HPLC), the remaining portion of the supernatants was dried using a conventional oven (Memmert, UFB 500) at 50 °C and then resuspended with methanol. HPLC analysis was conducted by following the methods described in 2.6.2.
2.4. Extractions from ginger suspensions
2.4.1. Ultrasound-assisted extraction.
The control ginger suspension and pretreated ginger suspension were subjected to ultrasound-assisted extraction according to the method reported by Said et al.17 The high-pressure homogenization treatment conditions were determined based on the particle size measurement of ginger suspensions and analysis of aqueous ginger supernatants. Briefly, 40 mL of each ginger suspension was mixed with 120 mL of absolute ethanol, and ultrasound-assisted extraction was performed in an ultrasonic bath at a frequency of 60 Hz and a maximum power of 230 W (DAIHAN Scientific Ultrasonic Cleaner Set WUC-A03H, Gangwon, Korea) for 30 min at 30 °C. After this, the excess solvents in ginger extracts were removed using a rotary evaporator at 50 °C.
2.4.2. Soxhlet extraction.
The control ginger suspension and the pretreated ginger suspension were subjected to Soxhlet extraction according to the method of Said et al.17 with minor modifications. The high-pressure homogenization treatment conditions were determined considering the particle size measurement of ginger suspensions and analysis of aqueous ginger supernatants. Approximately 40 mL of each ginger suspension was placed in a thimble, and the extraction was performed for 8 h at 78 °C with 120 mL of absolute ethanol. After this, the excess solvents in ginger extracts were removed using a rotary evaporator at 50 °C.
2.5. Drying of ginger extracts acquired from ultrasound-assisted and Soxhlet extractions
Three drying methods were applied to the ginger extracts obtained from ultrasound-assisted and Soxhlet extractions to eliminate the remaining solvents. Oven drying of ginger extracts was conducted in a conventional oven (Memmert, UFB 500) at 50 °C until constant weights were obtained. The ginger extracts were vacuum-dried at 40 °C for 48 h in a vacuum oven (Binder, VD 115). The ginger extracts were freeze-dried to constant weights and stored at −80 °C until further analysis.
2.6. Characterization of ginger extracts obtained from Soxhlet and ultrasound-assisted extractions
2.6.1. Extraction yield.
The following formula was used for the calculation of the extraction yield of ginger extract:
Extraction yield (%) = (Weight of dried ginger extract/Initial weight of ginger) × 100 |
2.6.2. 6-Gingerol content by HPLC analysis.
6-Gingerol content was determined by HPLC analysis following the method of Kamal et al.18 with slight modifications. Samples (5 μL) were injected into a HPLC system (Agilent 1260 Infinity HPLC system, Santa Clara, U.S.A.) through a C-18 column (LiChroCART® Stainless Steel Purospher™ STAR, 5 μm, 4.6 × 150 mm) (Merck, Darmstadt, Germany) at 35 °C. A diode array detector was set at 280 nm, and the flow rate was 1.2 mL min−1. The mobile phase was acetonitrile: 0.05% orthophosphoric acid in water (60
:
40, v/v).
2.6.3. Total phenolic content (TPC).
The TPC of ginger extracts was analyzed according to the Folin-Ciocalteu method as modified by Jeyaraj et al.19 To each ginger extract prepared at 1 mg mL−1, 2.5 mL of Folin-Ciocalteu reagent (10-time dilution) was added and mixed for 3 min. Then 2.5 mL of Na2CO3 (7.5% w/v) was added to the mixture and incubated for 30 min at ambient temperature in a dark place, and the absorbance was read at 760 nm with a UV-VIS spectrophotometer (Norwalk, USA). Gallic acid was used as a standard, and the TPC of each ginger sample was expressed in mg of gallic acid equivalent per g of dried ginger extract (mg GAE/g extract).
2.6.4. Determination of antioxidant activity.
2.6.4.1. DPPH radical scavenging assay.
The DPPH radical scavenging activity of ginger extracts was quantified using the method reported by Vidana Gamage and Choo.20 First, 5.9 mg of DPPH was dissolved in 100 mL of 100% methanol to prepare the stock solution. For different dilutions of each ginger extract, 1 mL of DPPH solution was mixed with 250 μL of the sample. The mixture was then incubated in the dark for 30 min, and absorbance was detected at 517 nm using a UV-VIS spectrophotometer (Norwalk, USA). The following equation was applied to calculate the percentage of DPPH scavenging activity:
Free radical scavenging activity (%) = (AC − As)/ AC × 100 |
where AC = control absorbance and AS = absorbance of the ginger samples.
IC50 was indicated as the concentration of ginger extract that can scavenge 50% of the initial DPPH radicals.
2.6.4.2. Ferric reducing antioxidant power (FRAP) assay.
FRAP analysis of the ginger extracts was performed using the method reported by Vidana Gamage and Choo.21 A quantity of 1 mL potassium ferricyanide (1% w/v) and 1 mL phosphate buffer (0.2 M, pH 6.6) were added to 400 μL of the sample. Then, the mixture was kept at 50 °C for 20 min, and 1 mL of trichloroacetic acid (10% w/v) was mixed. Then, 1 mL of aliquots was separated from the mixture, and the separated aliquots were diluted by adding 1 mL of water. Next, 200 μL of ferric chloride (0.1% w/v) was mixed with the diluted aliquots. The solution was allowed to stand in the darkness for 30 min, and absorbance was recorded at 700 nm. Ascorbic acid (AA) was the standard, and FRAP was indicated as mg of AA per g.
2.7. Statistical analysis
All experiments were performed in independent triplicate. The data were presented with the mean value ± standard deviation. The statistical analysis was conducted by one-way and two-way ANOVA employing the SPSS 26 software (New York, USA). The values with p < 0.05 were identified as statistically significant.
3. Results and discussion
3.1. Effect of high-pressure homogenization pretreatment on the particle size reduction
The particle size distribution (PSD) of ginger samples is a valuable indicator to identify the impact of various high-pressure homogenization conditions (pressure and the number of cycles) on cell disruption and disintegration of complex ginger tissues into small particles. Particle sizes of ginger samples treated with various high-pressure homogenization conditions are presented in Table 1. The PSD of ginger samples subjected to the various high-pressure homogenization conditions is presented in Fig. 1. All ginger samples subjected to the high-pressure homogenization demonstrated a significantly lower volume mean diameter compared to the control ginger sample (Table 1) as a consequence of cell disruption by mechanical forces exerted during the high-pressure homogenization process. The control ginger suspension showed a wider PSD ranging from 1 to 430 μm compared to the other pretreated ginger suspensions (Fig. 1). The significant reduction of particle sizes after the high-pressure homogenization can be further observed in the diameter (0.9) value which indicates the particle diameter at which 90% of particles are by volume. Accordingly, the diameter (0.9) of the control ginger sample was 177.9 ± 13.7 μm. After one cycle of high-pressure homogenization at 50 MPa and 100 MPa, diameter (0.9) values were significantly decreased to 128.5 ± 7.6 μm and 66.0 ± 28.0 μm, respectively (Table 1). A similar influence of high-pressure homogenization on the reduction of particle sizes was reported in the previous literature.9,12,13 Both pressure conditions and the number of cycles through the high-pressure homogenizer cause a prominent decrease in particle sizes.
Table 1 Particle sizes of ginger samples treated under different high-pressure homogenization (HPH) conditionsa
Treatment |
Volume mean diameter (μm) |
Diameter (0.1) (μm) |
Diameter (0.5) (μm) |
Diameter (0.9) (μm) |
Values followed by different superscript letters within a column are significantly different (p < 0.05).
|
Control sample |
54.1 ± 5.2a |
1.5 ± 0.03a |
17.7 ± 0.83a |
177.9 ± 13.7a |
HPH 50 MPa 1 cycle |
39.2 ± 3.1b |
1.5 ± 0.03a |
16.9 ± 0.61ac |
128.5 ± 7.6b |
HPH 50 MPa 5 cycles |
23.1 ± 0.5cd |
1.5 ± 0.04a |
15.6 ± 0.42bc |
40.7 ± 3.9 cd |
HPH 50 MPa 10 cycles |
17.7 ± 2.2df |
1.49 ± 0.04a |
15.2 ± 0.31b |
29.9 ± 2.4d |
HPH 100 MPa 1 cycle |
27.2 ± 2.5c |
1.61 ± 0.10a |
16.1 ± 0.09bc |
66.0 ± 28.0c |
HPH 100 MPa 5 cycles |
15.7 ± 0.2f |
1.47 ± 0.09a |
15.2 ± 0.34b |
27.5 ± 0.2d |
HPH 100 MPa 10 cycles |
10.6 ± 0.2e |
1.34 ± 0.04a |
12.29 ± 0.13b |
21.3 ± 0.5e |
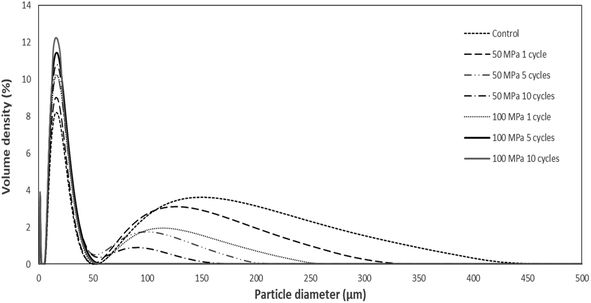 |
| Fig. 1 Particle size distribution of ginger suspension treated under different high-pressure homogenization conditions. | |
High-pressure homogenization pretreatment significantly reduced the size of ginger particles (volume mean diameter) while increasing the pressure from 50 MPa up to 100 MPa under a similar number of cycles (Table 1). Furthermore, the pressure of 100 MPa in high-pressure homogenization demonstrated the most influence on size reduction by directly decreasing the volume mean diameter of ginger samples to 27.2 ± 2.5 μm at 1 cycle from the 54.1 ± 5.2 μm of the control sample (Table 1). Xing et al.13 also observed a similar trend that pressure could have more impact on particle size reduction compared to the number of cycles. However, higher pressure conditions and more cycles did not always result in lower particle sizes.9 Among high-pressure homogenization-treated ginger samples in this study, the pressure of 100 MPa and 10 cycles exhibited the lowest volume mean diameter (Table 1). PSD of ginger samples progressively became narrower with increasing the number of passes and pressure in high-pressure homogenization. Finally, the bimodal distribution of particles was converted to a sharper single peak which shifted to the range of less than 50 μm after applying high-pressure homogenization at a pressure of 100 MPa for 10 cycles (Fig. 1). This observation indicates that maximum cell disruption of ginger suspensions occurred in high-pressure homogenization at 100 MPa for 10 cycles.
3.2. Effect of high-pressure homogenization treatment on the microstructure of ginger samples
SEM was employed to identify the microstructural changes in ginger samples after applying the high-pressure homogenization pretreatment (100 MPa for 10 cycles). SEM images in Fig. 2 demonstrated how high-pressure homogenization affected the breakage and disruption of complex ginger tissues into smaller cell fragments compared to the control ginger sample. As in Fig. 2A, the control ginger sample tissues were tightly packed, and cells remained together with smooth surfaces compared to the ginger sample treated with high-pressure homogenization. Microfragments and disintegrated cells that are observed in Fig. 2B imply that combined forces in high-pressure homogenization, such as shear stress, cavitation, and turbulence could damage the ginger cell structure.
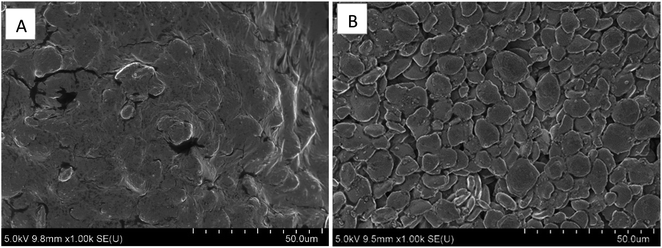 |
| Fig. 2 Scanning electron micrographs of ginger samples, (A) control and (B) treated with high-pressure homogenization under 1000 MPa and 10 cycles (1000× magnification). | |
3.3. Impact of high-pressure homogenization on TPC, 6-gingerol content, and antioxidant activity of ginger supernatants
TPC, 6-gingerol content, and antioxidant activity of ginger supernatants were also analyzed to gain insight into the effect of the various high-pressure homogenization conditions on the cell disruption and the extractability of intracellular compounds in ginger samples. The supernatants of all ginger suspensions treated with high-pressure homogenization had higher 6-gingerol content compared to the control sample (Table 2). The results in Table 2 agree with the particle size measurement in Table 1. The number of cycles and the pressures are the main energy supplies in high-pressure homogenization. Generally, higher intensity of high-pressure homogenization conditions induce more cell rupture and breakage of plant tissue structures and facilitate the liberation of intracellular compounds.22 For example, 6-gingerol content at 100 MPa for 1 cycle increased by 112.9% with respect to the control ginger supernatant, whereas 6-gingerol increased by only 79.1% at 50 MPa for 1 cycle (Table 2). This observation agrees with Jurić et al.,15 indicating that high-pressure conditions (100 MPa) demonstrated the highest recovery of intracellular compounds. Furthermore, when the number of cycles in high-pressure homogenization was expanded from 1 to 10, recovery of 6-gingerol was increased by 33.5% and 20.4% at 50 MPa and 100 MPa, respectively (Table 2). This observation proves that an increase in the number of cycles in high-pressure homogenization also facilitated adequate cell disruption and recovery of 6-gingerol. The results in Table 2 further demonstrate the potential of high-pressure homogenization to enhance the release of poorly water-soluble compounds such as 6-gingerol. This observation agrees with the findings of Jurić et al.,15 in which high-pressure homogenization facilitated the complete recovery of hydrophobic lycopene from tomato peel.
Table 2 Antioxidant activity, TPC, and 6-gingerol content of aqueous ginger supernatants treated by different high-pressure homogenization treatmentsa
Sample |
IC50 of DPPH assay (mg mL−1) |
FRAP assay (mg of ascorbic acid equivalent per mL) |
TPC content (mg of GAE per mL) |
6-Gingerol content (mg mL−1) |
Values followed by different superscript letters within a column are significantly different (p < 0.05).
|
Control |
0.221 ± 0.221a |
0.148 ± 0.002ad |
0.107 ± 0.001a |
37.8 ± 1.5e |
HPH 50 MPa 1 cycle |
0.168 ± 0.168ab |
0.153 ± 0.001ab |
0.110 ± 0.001a |
67.7 ± 4.3d |
HPH 50 MPa 5 cycles |
0.164 ± 0.163ab |
0.160 ± 0.007bd |
0.112 ± 0.001a |
85.9 ± 2.1bc |
HPH 50 MPa 10 cycles |
0.143 ± 0.143bc |
0.165 ± 0.002be |
0.118 ± 0.001bcd |
90.4 ± 1.8ab |
HPH 100 MPa 1 cycle |
0.154 ± 0.153ac |
0.154 ± 0.006ade |
0.121 ± 0.001cd |
80.5 ± 2.9c |
HPH 100 MPa 5 cycles |
0.139 ± 0.139bc |
0.179 ± 0.004c |
0.123 ± 0.001bd |
87.9 ± 4.4bc |
HPH 100 MPa 10 cycles |
0.133 ± 0.132bc |
0.182 ± 0.005c |
0.132 ± 0.003b |
96.9 ± 1.5a |
According to the DPPH assay, the high-pressure homogenization with higher intensity, including 50 MPa for 10 cycles and 100 MPa for 5 and 10 cycles, demonstrated significantly higher antioxidant activity than the control ginger supernatant (Table 2). FRAP assay showed that the control ginger supernatant had significantly lower antioxidant activity than the ginger samples subjected to high-pressure homogenization at a pressure of 50 MPa and 100 MPa for 5 and 10 cycles (Table 2). The recovery of antioxidants increased with an increasing number of cycles and pressures in high-pressure homogenization. This is most likely due to the effect of intense cell rupture. Similarly, Gali et al.23 observed that the antioxidant activity of Ruta chalepensis samples increased only at higher intensities of high-pressure homogenization (8 and 10 cycles). According to the TPC results in Table 2, the high-pressure homogenization at 100 MPa for 5 cycles and 10 cycles increased the TPC by 14.9% and 23.3%, respectively, compared to the control ginger supernatants. However, the TPC of ginger supernatant was not significantly increased under high-pressure homogenization conditions at 50 MPa for 1 cycle and 5 cycles (Table 2). Karacam et al.24 noticed a similar pattern in the TPC value of strawberry juice at which around a 10% TPC increase was observed at 100 MPa in high-pressure homogenization while no significant TPC increase was observed under low-pressure conditions (60 MPa) compared to the control sample. Karacam et al.24 further reported that combining the high-pressure with mild temperature during the high-pressure homogenization could inactivate some enzymes, including peroxidase and polyphenol oxidase, which can degrade polyphenols. Therefore, more polyphenols could be retained in high-pressure homogenization-treated ginger samples than in the control ginger sample. Similarly, the TPC increase in this study at 100 MPa could be due to enzyme inactivation during high-pressure homogenization. Furthermore, another reason for the enhanced antioxidant activity and TPC in the high-pressure homogenization-treated ginger supernatants might be the effect of cell rupturing under the intense high-pressure homogenization conditions, thereby releasing more polyphenols from plant cells. Wellala et al.25 reported that most hydrophilic polyphenols are located in plant cell vacuoles; thus, polyphenols could be readily released to the surrounding liquid phase due to cell rupturing during high-pressure homogenization.
3.4. Impact of high-pressure homogenization pretreatment with various extraction and drying combinations on recovery of 6-gingerol from ginger
3.4.1. Extraction yield.
Fig. 3A shows the extraction yield of ginger samples obtained from ultrasound-assisted and Soxhlet extractions followed by various drying techniques. Considering the results from the particle size measurement and analysis of the ginger supernatant, the high-pressure homogenization conditions with 100 MPa for 10 cycles were selected for pretreatment conditions before extractions and dryings. The high-pressure homogenization-treated ginger sample obtained from ultrasound-assisted extraction followed by vacuum drying showed the highest extraction yield, which was increased by 78.3% compared to that of the control ginger sample (Fig. 3A). Furthermore, both pretreated vacuum-dried and freeze-dried ginger samples demonstrated a higher extraction yield in the ultrasound-assisted extraction compared to the Soxhlet extraction (Fig. 3A). Temperature is crucial in deciding the extraction yield from ginger because thermolabile compounds such as gingerols, total carotenoids, curcumin, and β-carotene could degrade at higher temperatures (>80 °C).26 Soxhlet extraction was carried out at high temperatures compared to ultrasound-assisted extraction. Therefore, a higher extraction yield was achieved from the ultrasound-assisted extraction compared to the Soxhlet extraction. Said et al.17 mentioned that ultrasound-assisted extraction generates cavitation throughout the particles, enhancing the diffusion of extracting solvent; thus, the extraction yield from ultrasound-assisted extraction was 1.75 times higher than that from Soxhlet extraction. Furthermore, Dalsasso et al.26 reported that ultrasound has the potential to disintegrate natural polymers such as pectin, cellulose, and hemicellulose from the ginger matrix into smaller structures with low molecular weights and high solubility, which leads to higher extraction yield.
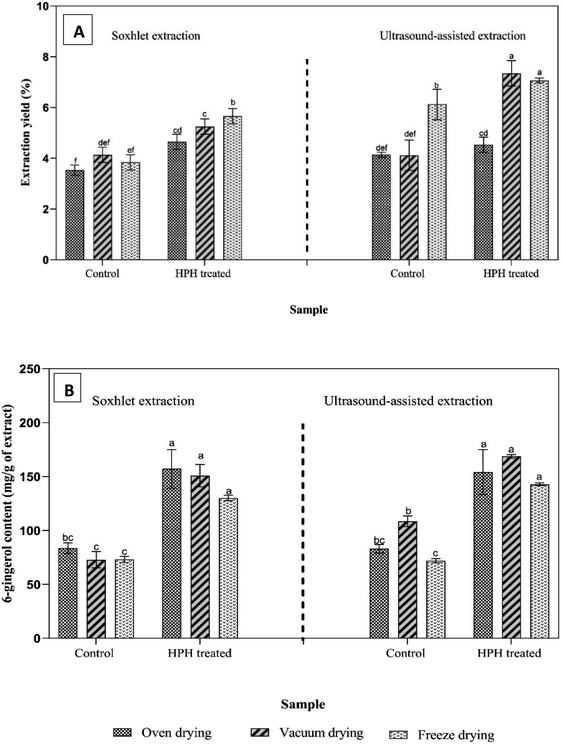 |
| Fig. 3 Extraction yield (%) of ginger extracts (A) and 6-gingerol content (mg of 6-gingerol/g of extract) of ginger extracts (B) obtained from Soxhlet extraction and ultrasound-assisted extraction followed by different drying methods. Values with different lowercase letters were significantly different (p < 0.05) within an extraction method. | |
Considering all extraction and drying combinations, pretreated ginger samples with high-pressure homogenization always resulted in significantly higher extraction yields compared to that of control ginger samples (Fig. 3A). According to the particle size analysis (Table 1), high-pressure homogenization-treated ginger samples resulted in smaller particle sizes compared to the control sample. Reduced particle sizes could increase surface area directly in contact with the solvent facilitating efficient extraction. Makanjuola27 conducted an aqueous extraction of ginger which was dried, ground, and passed through sieves with 0.425 mm, 0.750 mm, and 1.18 mm. The highest extraction yield of ginger extracts was obtained using the ginger powder with the smallest particle size (0.425 mm). Therefore, cell disruption and breakage of plant tissues during high-pressure homogenization could induce efficient extraction resulting in a higher extraction yield than the control samples.28
3.4.2. 6-Gingerol content.
6-Gingerol content of ginger extracts obtained from various combinations of extraction and drying methods is illustrated in Fig. 3B. The pretreated ginger samples with high-pressure homogenization followed by various extraction and drying combinations showed a significantly higher 6-gingerol content compared to control ginger samples (Fig. 3B). This observation agrees with the results in Table 2, in which the 6-gingerol content of pretreated ginger supernatants with high-pressure homogenization was significantly higher than that of the control ginger supernatant.
HPLC analysis showed that the 6-gingerol content was enhanced in the oven-dried ginger extract obtained from Soxhlet extraction and ultrasound-assisted extraction by 87.7% and 85.7%, respectively, in comparison with the control sample (Fig. 3B). The 6-gingerol content of pretreated ginger samples with high-pressure homogenization did not show a significant difference among various drying methods in both Soxhlet and ultrasound-assisted extractions. 6-Gingerol is a thermolabile compound and could degrade to shogaols and zingerone at temperatures above 80 °C.27 In this study, all three drying methods were conducted at temperatures below 50 °C to prevent the conversion of 6-gingerol. Previous studies reported that high-pressure homogenization loosened and ruptured tightly packed plant cells, thereby enhancing the release of bioactive compounds.15,23,28 However, any findings about the influence of high-pressure homogenization as a pretreatment on the extraction from ginger suspensions have not been reported. Therefore, the results obtained from this study could not be directly compared with those from previous studies. The particle sizes and properties of raw materials, intracellular components, extraction methods and conditions, and extracting solvents are important factors in deciding the release of phytochemicals.29
3.4.3. TPC and antioxidant activity.
The total phenolic content of ginger extracts obtained from various extraction and drying combinations is presented in Table 3. The oven-dried pretreated ginger extracts with high-pressure homogenization demonstrated a higher TPC in both Soxhlet and ultrasound-assisted extractions than the freeze-dried and vacuum-dried ginger extracts (Table 3). Ghafoor et al.30 mentioned that the oven drying method has a higher energy strength to enhance the degradation of enzymes such as polyphenol oxidase, which may reduce the TPC of ginger extract. Therefore, the oven-dried ginger extract could exhibit higher TPC. All pretreated ginger extracts with high-pressure homogenization had higher TPC than control ginger extracts under all extraction and drying combinations (Table 3). This observation also proved that high-pressure homogenization could improve the release of polyphenols through cell disruption. DPPH assays showed the antioxidant activity of ginger samples obtained under various combinations of extraction and drying techniques (Table 3). Ginger suspensions pretreated by high-pressure homogenization pretreatment followed by ultrasound-assisted extraction demonstrated higher antioxidant activity than the control suspension (Table 3). For example, the high-pressure homogenization pretreated ginger extracts obtained from ultrasound-assisted extraction exhibited lower IC50 values in all three drying methods than control ginger extracts (Table 3).
Table 3 Antioxidant activity and TPC of ginger samples obtained by different extraction and drying methodsa
Sample |
Oven drying |
Vacuum drying |
Freeze drying |
Values followed by different superscript lowercase letters were significantly different (p < 0.05) between extraction methods. Values followed by different superscript uppercase letters were significantly different (p < 0.05) between drying methods.
|
IC
50
of DPPH asay (mg mL−
1
)
|
Soxhlet extraction |
|
|
|
Control |
0.475 ± 0.05aA |
0.385 ± 0.15cC |
0.416 ± 0.02bB |
HPH treated |
0.437 ± 0.02abA |
0.407 ± 0.02bA |
0.355 ± 0.01cB |
Ultrasound-assisted extraction |
|
|
|
Control |
0.457 ± 0.02aB |
0.422 ± 0.12bA |
0.415 ± 0.16bA |
HPH treated |
0.383 ± 0.03cA |
0.350 ± 0.01cA |
0.338 ± 0.07cA |
![[thin space (1/6-em)]](https://www.rsc.org/images/entities/char_2009.gif) |
FRAP assay (mg of ascorbic acid equivalent per g of extract)
|
Soxhlet extraction |
|
|
|
Control |
55.78 ± 7.3cA |
71.20 ± 12.1bcA |
62.94 ± 5.1cA |
HPH-treated |
68.84 ± 3.2bcB |
80.70 ± 4.1bB |
94.69 ± 3.7aA |
Ultrasound-assisted extraction |
|
|
|
Control |
70.77 ± 4.8bcA |
78.18 ± 19.8bA |
77.82 ± 5.2bA |
HPH-treated |
78.48 ± 7.9bB |
80.72 ± 2.18bB |
97.23 ± 2.0aA |
![[thin space (1/6-em)]](https://www.rsc.org/images/entities/char_2009.gif) |
TPC (mg of GAE per g)
|
Soxhlet extraction |
|
|
|
Control |
8.33 ± 0.3cA |
16.6 ± 3.1abB |
11.3 ± 1.3bA |
HPH-treated |
19.0 ± 7.8aB |
16.9 ± 0.3abB |
14.8 ± 1.5bA |
Ultrasound-assisted extraction |
|
|
|
Control |
12.1 ± 3.3bA |
17.3 ± 6.8abA |
11.7 ± 7.2bA |
HPH-treated |
19.7 ± 2.4aA |
18.2 ± 0.6abA |
15.0 ± 1.2abA |
FRAP assay showed that the antioxidant activity of high-pressure homogenization pretreated freeze-dried ginger extracts was significantly higher in both Soxhlet and ultrasound-assisted extractions than that of ginger extracts obtained from the vacuum and oven drying (Table 3). Vichakshana et al.16 evaluated the influence of high-pressure homogenization pretreatment combined with various extractions and dryings on the antioxidant activity of turmeric extracts. Similarly, Vichakshana et al.16 found that the antioxidant activity of freeze-dried turmeric extracts was higher than that obtained from vacuum drying and oven drying in both Soxhlet and ultrasound-assisted extractions. This observation proves that turmeric and ginger extracts demonstrated a similar trend in antioxidant assays. Dalsasso et al.26 reported that low operating temperature in freeze drying protects the thermolabile compounds in ginger extract and freeze drying could further enhance the antioxidant activity in ginger extracts by the effect of cell disruption during the ice crystal formation in the pre-freezing stage.
4. Conclusions
This study investigated a novel approach of using high-pressure homogenization as a pretreatment combined with various extractions and drying techniques for enhancing the release of intracellular compounds from ginger. While the particle sizes of ginger suspensions decreased at a higher number of cycles (5 or 10) or under high-pressure conditions (100 MPa) in high-pressure homogenization, the antioxidant activity, TPC, and 6-gingerol content in ginger supernatants increased. The pretreated samples showed a significantly higher 6-gingerol content after applying all extraction and drying combinations compared to the control samples. For the pretreated ginger samples in both ultrasound-assisted and Soxhlet extractions, the freeze-dried ginger extracts demonstrated higher antioxidant activity and the oven-dried ginger extracts exhibited a higher TPC than ginger extracts dried by other methods. In conclusion, high-pressure homogenization significantly enhanced the release of 6-gingerol from the rhizome of ginger. Future studies can investigate the effect of time, frequency, solvent type, and solvent volume during extractions of 6-gingerol.
Author contributions
Ginithillawala Arachchilage Dilukshi Vichakshana: investigation, methodology, formal analysis, writing. Su Chern Foo: supervision, writing – review & editing. Wee Sim Choo: supervision, resources, project administration, writing – review & editing.
Conflicts of interest
The authors declare there are no financial interests or personal relationships which may be considered as potential competing interests.
Acknowledgements
The authors are extremely grateful to Monash University Malaysia for providing funds for this research work.
References
- Q. Wang, Q. Wei, Q. Yang, X. Cao, Q. Li, F. Shi, S. S. Tong, C. Feng, Q. Yu, J. Yu and X. Xu, Int. J. Pharm., 2018, 535(1), 308–315 CrossRef CAS.
- Q. Wei, Q. Yang, Q. Wang, C. Sun, Y. Zhu, Y. Niu, J. Yu and X. Xu, AAPS PharmSciTech, 2018, 19(8), 3661–3669 CrossRef CAS PubMed.
- G. A. D. Vichakshana, D. J. Young and W. S. Choo, Qual. Assur. Saf. Crops Foods, 2022, 14(4), 67–83 CrossRef CAS.
- V. Hessel, N. N. Tran, M. R. Asrami, Q. D. Tran, N. V. D. Long, M. E. Gelonch, J. O. Tejada, S. Linke and K. Sundmacher, Green Chem., 2022, 2, 410–437 RSC.
- M. Arshadi, T. M. Attard, R. M. Bogel-Lukasik, M. Brncic, A. M. da Costa Lopes, M. Finell, P. Geladi, L. N. Gerschenson, F. Gogus, M. Herrero, A. J Hunt, E. Ibanez, B. Kamm, I. Mateos-Aparicio Cediel, A. Matias, N. Mavroudis, E. Montoneri, A. R. C. Morais, C. Nilsson, E. H. Papaioannou, A. Richel, P. Ruperez, B. Skrbic, M. Bodroza-Solarov., J. Svarc-Gajic, K. Waldron and F. Yuste, Green Chem., 2016, 18, 6160–6204 RSC.
- A. Patel and A. R. Shah, J. Bioresour. Bioprod., 2021, 6(2), 108–128 CrossRef CAS.
- K. O. Olatunji and D. M. Madyira, Waste Biomass Valorization, 2024, 15, 469–486 CrossRef CAS.
- L. Zhou, Y. Guan, J. Bi, X. Liu, J. Yi, Q. Chen, X. Wu and M. Zhou, LWT--Food Sci. Technol., 2017, 82, 121–130 CrossRef CAS.
- Z. Y. Yu, S. W. Jiang, X. M. Cao, S. T. Jiang and L. T. Pan, J. Food Eng., 2016, 177, 1–8 CrossRef.
- D. Carullo, B. D. Abera, A. A. Casazza, F. Donsì, P. Perego, G. Ferrari and G. Pataro, Algal Res., 2018, 31, 60–69 CrossRef.
- S. X. M. Yong, C. P. Song and W. S. Choo, Front. Sustain. Food Syst., 2021, 4, 593259 CrossRef.
- K. E. Preece, N. Hooshyar, A. J. Krijgsman, P. J. Fryer and N. J. Zuidam, Innovative Food Sci. Emerging Technol., 2017, 41, 47–55 CrossRef CAS.
- J. J. Xing, Y. I. Cheng, P. Chen, L. Shan, R. Ruan, D. Li and L. J. Wang, Powder Technol., 2019, 358, 103–109 CrossRef CAS.
- C. Onumaegbu, J. Mooney, A. Alaswad and A. G. Olabi, Renewable Sustainable Energy Rev., 2018, 93, 16–26 CrossRef CAS.
- S. Jurić, G. Ferrari, K. P. Velikov and F. Donsì, J. Food Eng., 2019, 262, 170–180 CrossRef.
- G. A. D. Vichakshana, S. C. Foo and W. S. Choo, Innovative Food Sci. Emerging Technol., 2023, 83, 103249 CrossRef CAS.
- P. P. Said, O. P. Arya, R. C. Pradhan, R. S. Singh and B. N. Rai, J. Food Process Eng., 2015, 38(2), 107–114 CrossRef CAS.
- Y. K. T. K. Kamal, M. Singh, S. Ahmad, P. Alam and S. Salam, J. Anal. Sci. Technol., 2015, 6(1), 23 CrossRef.
- E. J. Jeyaraj, Y. Y. Lim and W. S Choo, ACS Food Sci. Technol., 2021, 1(9), 1567–1577 CrossRef CAS.
- G. C. Vidana Gamage and W. S. Choo, Food Chem. Adv., 2023, 2, 100209 CrossRef.
- G. C. Vidana Gamage and W. S. Choo, Food Chem. Adv., 2023, 2, 100236 CrossRef.
- S. Plazzotta and L. Manzocco, Innovative Food Sci. Emerging Technol., 2018, 50, 11–19 CrossRef CAS.
- L. Gali, F. Bedjou, K. P. Velikov, G. Ferrari and F. Donsì, J. Food Meas. Charact., 2020, 14(5), 2800–2809 CrossRef.
- C. H. Karacam, S. Sahin and M. H. Oztop, LWT--Food Sci. Technol., 2015, 64(2), 932–937 CrossRef CAS.
- C. K. D. Wellala, J. Bi, X. Liu, J. Liu, J. Lyu, M. Zhou, K. Marszałek and U. Trych, Innovative Food Sci. Emerging Technol., 2020, 60, 102279 CrossRef CAS.
- R. R. Dalsasso, G. A. Valencia and A. R. Monteiro, Food Res. Int., 2022, 154, 111043 CrossRef CAS PubMed.
- S. A. Makanjuola, Food Sci. Nutr., 2017, 5(6), 1179–1185 CrossRef CAS PubMed.
- X. Zhu, Y. Cheng, P. Chen, P. Peng, S. Liu, D. Li and R. Ruan, Innovative Food Sci. Emerging Technol., 2016, 37, 91–97 CrossRef CAS.
- B. Mahayothee, T. Thamsala, P. Khuwijitjaru and S. Janjai, J. Appl. Res. Med. Aromat. Plants, 2020, 18, 100262 Search PubMed.
- K. Ghafoor, F. Al Juhaimi, M. M Özcan, N. Uslu, E. E. Babiker and I. A. Mohamed Ahmed, LWT--Food Sci. Technol., 2020, 126, 109354 CrossRef CAS.
|
This journal is © The Royal Society of Chemistry 2024 |
Click here to see how this site uses Cookies. View our privacy policy here.