Transitioning of petroleum-based plastic food packaging to sustainable bio-based alternatives
Received
28th January 2024
, Accepted 4th March 2024
First published on 4th March 2024
Abstract
Motivated by the urgent need to address environmental concerns associated with traditional food packaging, this review explores the shift towards sustainable, bio-based packaging solutions. It highlights the evolution of food packaging functions, emphasizing not only the basic roles but also the innovative methods developed to enhance food quality. The focus extends to well-researched bio-based plastics, particularly in promoting their mechanical properties. Additionally, the review explores the innovative use of plant-based and animal-based materials in creating sustainable packaging solutions. These materials have shown considerable promise in improving mechanical properties, prolonging shelf life, and providing antimicrobial and antioxidant benefits. A critical aspect of the review is the practical application of bio-based biodegradable materials in the food packaging industry. Despite significant theoretical advancements, there remains a gap in understanding how these materials can be effectively utilized in real-world scenarios.
Sustainability spotlight
Petroleum-based plastic food packaging is leaving a significant impact on the environment. A more sustainable alternative is the use of bio-based plastics. This manuscript reviews the current state of the art in the development of bio-based plastics based on scientific reports and reported intellectual properties. The manuscript also advances this topic by looking at the potential agro-waste sources from which these bio-based plastics can be derived. On these bases, this work aligns with SGD 12 “Responsible Consumption and Production” with more alignment on the production aspect.
|
Introduction
The landscape of food packaging is currently undergoing a significant transformation, driven by the imperative to reconcile the dual objectives of preserving food quality and mitigating environmental impact.1,2 Traditional food packaging solutions, predominantly made from petroleum-based plastics,3 are increasingly recognized as unsustainable due to their contribution to environmental pollution and their reliance on finite fossil fuel resources.4 As these materials accumulate in ecosystems, they pose severe threats to wildlife, human health and ecological balance, persisting for centuries without degradation.5 Despite the fundamental functions that traditional packaging should maintain, bioplastics emerge as a promising solution in this context, offering the advantages of biodegradability and compostability, thus reducing the environmental footprint of packaging waste.6
Agricultural waste has also become a significant environmental concern,7 particularly due to its potential to release greenhouse gases like CO2 into the ecosystem when not disposed of properly.8 Recent studies have shown an increasing trend in the generation of agro-waste, highlighting the urgent need for effective disposal and management systems.9 However, there is a silver lining: this waste can be innovatively repurposed in the production of sustainable food packaging materials, thereby integrating the concept of sustainability into the food packaging production chain. The transformation of agro-waste into bioplastics and other sustainable packaging materials not only mitigates its environmental impact but also contributes to a circular economy.
A critical gap in the field of bio-based biodegradable materials is in the understanding of their real practical applications, particularly in the food packaging industry. Despite the advancement in theoretical knowledge, there's a noticeable lack of comprehensive insight into how these materials can be applied in real-world scenarios. Studies underscore the growing body of research dedicated to exploring alternative materials that align with sustainability goals. However, transitioning from laboratory research to practical, real-world applications presents significant challenges. One of the primary obstacles is scaling up production while maintaining the material properties and cost-effectiveness.10 Another critical aspect is consumer acceptance and market readiness; as investigated by researchers, despite the environmental benefits, consumer preference for bio-based packaging is influenced by factors like appearance, safety, and cost.11,12 These challenges highlight the complexity of implementing bio-based solutions in the food packaging industry, necessitating further research and collaboration between scientists, industry stakeholders, and policymakers.
This review attempts to bridge this gap by juxtaposing theoretical implications with practical applications. It explores how these materials are currently being used and what future applications might entail. Furthermore, the review outlines future research directions and prospects for bio-based materials in food packaging. It pinpoints areas where further research is necessary to overcome existing challenges and enhance the applicability of these materials, offering a forward-looking perspective essential for future research and development in this field.
Packaging function types
The basic functions of food packaging
The roles of food packaging have been defined as “PCCC”, an acronym for “Protection and Preservation, Containment, Communication and Convenience”.13,14 These functions are interconnected and work together to protect food from the moment it is produced to the moment it is brought to the table as safe for consumption. The primary role of food packaging is containment. Clearly, as a packaging, ranging from primary to tertiary, all must have the ability to hold the stuff, ensure the containment of food, and prevent loss or damage during transport and handling.14,15 The most essential function of food packaging is to provide physical, chemical and biological protection that separates the external environment from the food itself. This physical protection is essential to protect food from mechanical damage, including protection from shock and vibration that may be encountered during transportation and distribution.1,16 In addition to physical properties, chemical barriers also prevent undesirable interactions between the product and oxygen, thus further providing a biological barrier against microbial contamination and infestation by insects, rodents, or other pests that could lead to spoilage of the food product or pose a health hazard to the consumer.16
The preservation function is instrumental in extending the shelf life of food products by maintaining the desired conditions within the packaging, thus slowing down the degradation or spoilage processes17 and also maintaining nutritional integrity, like flavour, colour, texture, and overall quality of food throughout its lifespan, ensuring that the food remains palatable and nutritious from the point of packaging to consumption.18 Furthermore, by extending the shelf-life and maintaining the quality of food products, packaging can further minimise food waste in the modern food supply chain.19
Convenience in food packaging is about making the product easy to use, handle, store, and dispose of. This includes aspects like easy opening, resealability, portability, and the ability to use the product in portions. Convenience features are designed to enhance the consumer experience and can often be a deciding factor in the consumer's purchasing decision. Lastly, the communication function of packaging involves conveying essential information about the product to the consumer. This includes labelling that provides details such as nutritional content, ingredients, usage instructions, and expiration dates. Packaging also serves as a key marketing tool, with its design, colour, and branding playing a significant role in attracting consumers and differentiating the product in the market.14,15
What goes beyond the basic functions?
Smart packaging technology refers to innovative packaging technologies that go beyond the conventional functions of containing and protecting products, by providing additional features such as real-time monitoring, extending shelf-life, and improving consumer engagement.20–22 Smart packaging technology can be broadly categorised into active and intelligent packaging.23
Active packaging.
Active packaging is a modern approach that involves the incorporation of certain components into the packaging material or system, which interact with the food or the environment to extend shelf life and ensure the safety or quality of packaged foods (European Regulation No. 450/2009 (ref. 24)). Active packaging mainly includes two pathways to extending food products' shelf-life: releasing agents, which include antimicrobial and antioxidant functions, while the other is scavenging or absorbing that mainly focuses on moisture, odour, and gas elimination.25
Releasing agents.
This specialized packaging prevents microbial growth on food surfaces through either the direct contact of the packaging material with the food or through the release of antimicrobial agents into the food or the packaging environment.21,26 In direct contact antimicrobial packaging, the antimicrobial agents are incorporated into the packaging material, which then interacts directly with the food surface or the microorganisms present on it to inhibit their growth.27 The effectiveness of antimicrobial activity in this mechanism is often dependent on the proximity between the packaging material and the food product, although it's not always necessary for the packaging to be in direct contact with the food for effective antimicrobial action.26 The antimicrobial agents can migrate to the food surface and exhibit their antimicrobial action.
Apart from antimicrobial agents, antioxidant agents can also be incorporated into food packaging systems. These agents help in preventing the oxidation of food products, which can lead to rancidity, discolouration, and the degradation of essential nutrients, further extending the shelf life of food products.28,29 Controlled release packaging (CRP) is a more sophisticated technology where antimicrobial agents are released from the packaging material at controlled rates over time.30 This mechanism ensures a sustained antimicrobial effect, which is particularly beneficial for extending the shelf-life of food products. In CRP, active compounds like antimicrobials and antioxidants are encapsulated or embedded in the packaging material, and their release rates are controlled to provide a continuous protective effect against microbial growth.31 The release of antimicrobials can be triggered or regulated by various factors such as temperature, pH, or the presence of microorganisms. The encapsulation systems in CRP also protect the antimicrobial agents from environmental factors, thereby maintaining their efficacy throughout the shelf life of the food product.32
Scavenging or absorbing functions.
The detrimental effects of oxygen and moisture on food quality and shelf life are well-documented challenges in the food industry. Oxygen, being a highly reactive element, can initiate oxidative reactions in food products, particularly those sensitive to oxygen exposure. These reactions often lead to the degradation of essential food components such as lipids, proteins, and vitamins. The result is a noticeable decline in the quality of the food, characterised by off-flavours, discolouration, and a significant loss of nutritional value. To address this, the integration of oxygen-scavenging functions in active food packaging has become a transformative approach. This strategy, as highlighted in studies by Apriliyani et al.,33 Gaikwad et al.,34 and Vermeiren et al.,35 involves the use of materials within the packaging that can actively absorb or react with oxygen, thereby reducing its concentration around the food product. This reduction in oxygen levels can significantly slow down the rate of oxidative reactions, thus preserving the freshness, taste, and nutritional quality of the food. Similarly, the control of moisture within food packaging is crucial for maintaining food stability and safety. Excessive moisture can create an environment conducive to microbial growth, enzymatic activities, and undesirable textural changes in food products. These changes can compromise both the safety and sensory attributes of the food. Drago et al.36 emphasised the importance of managing moisture levels in food packaging. In response, the development of active food packaging technologies has incorporated moisture-absorbing functions. These functions typically involve the use of desiccants or humidity-regulating materials that can effectively absorb excess moisture or maintain an optimal level of humidity within the packaging. By doing so, they help in preserving the structural integrity and preventing the microbial spoilage of food products. Gaikwad, Singh, and Ajji37 have explored various materials and technologies that can be employed for this purpose, demonstrating how active packaging can be tailored to address specific moisture-related challenges in different types of food products.
Intelligent packaging
The intelligent packaging is designed to continuously monitor the properties of packaged foods. For instance, they might change colour/atmosphere composition/pH or temperature when the food starts to spoil or when it has been exposed to an undesirable environment.38 This real-time feedback is invaluable for both suppliers and consumers, ensuring food safety and reducing waste.38 Intelligent technologies have been classified into three categories: indicators, data carriers and sensors.39
Time–temperature indicators (TTIs) are the most commonly used intelligent packaging technologies.40 They provide visual information about the cumulative temperature history of the packaged food, which is crucial for perishable products.41 They can help to ensure the quality and safety of food products by indicating any temperature abuse during transportation and storage. Gas indicators monitor the levels of certain gases, like oxygen or carbon dioxide, within the packaging. This is particularly important for products packaged in modified atmosphere packaging (MAP) to ensure freshness and prolong shelf life.42 Freshness indicators are integrated into the packaging to provide a visual signal concerning the freshness of the food product.43 They can react with various compounds associated with food spoilage, providing a clear indication of the product's quality over time.
RFID, QR codes and barcodes are examples of data carriers. RFID (Radio Frequency Identification) technology in intelligent packaging allows for real-time tracking and tracing of food products throughout the supply chain,43 with the wireless communication between the tags and readers. QR codes and barcodes can be printed on packaging and scanned by consumers using smartphones to access additional information about the product, such as its origin, ingredients, and any allergen information. It also provides an avenue for brands to engage with consumers directly.
Edible packaging
Edible packaging represents a paradigm shift in sustainable food packaging practices, focusing on materials that are not only environmentally friendly but also safe to consume. Derived primarily from biopolymers, edible packaging materials originate from natural sources, offering a green alternative to conventional packaging materials. One of the most significant advantages of edible packaging is its environmental impact. Edible packaging takes the concept of biodegradability a step further. The properties that make these materials edible also make them hyper-compostable, meaning they break down rapidly and leave no waste behind.44 This zero-waste approach makes edible packaging an attractive solution in the context of increasing concerns about plastic waste and environmental sustainability.
Biopolymers, as the backbone of edible packaging, can be tailored into various forms such as films, coatings, or wraps. These serve as protective barriers for food products, shielding them from external adversities and enhancing their shelf life. Meanwhile both edible films and coatings share similarities in their protective attributes – from acting as a barrier against physical damage, safeguarding food from UV radiation, incorporating bioactives that confer antioxidative and antimicrobial properties, even with living microorganisms to providing health benefits.45,46
Edible films are pre-formed materials that are subsequently applied to food products.47 Their production involves creating a standalone material that is later used to wrap or cover food items. On the other hand, edible coatings are directly applied onto the food product. This can be achieved through various techniques such as coating, spraying, or immersion, ensuring that the food item is enveloped by the protective layer right from the onset.45
The primary constituents for these edible layers encompass a diverse range of materials including polysaccharides, proteins (both animal and vegetable-derived), lipids, resins, and even aloe. Among them, polysaccharide or protein-based films and coatings have been extensively studied due to their abundant availability and versatile properties.48,49 Starch is a common polysaccharide, consisting of multi-glucose units joined by glycosidic bonds, containing two types of glucose polymers: branched amylopectin and linear amylase.50 The starch itself is an excellent sustainable biodegradable material, with huge potential to enhance food preservations.51 The pure starch films are the simplest form of starch-based films, made primarily from starch extracted from sources like corn, potato, or rice. Although scratch-based films tend to have good gas barrier properties, but it come with poor mechanical properties, making them brittle.52 Therefore, further modifications should be done by adding naturally extracted materials to enhance the properties.
Plasticizers like glycerol, sorbitol and others can be added into starch films. Glycerol is one of the most commonly used plasticizers in starch-based films. It is a small molecule that can easily intercalate between starch chains, reducing intermolecular hydrogen bonding and increasing the mobility of the polymer chains.53 This results in films with enhanced flexibility and reduced brittleness.54 The sorbitol extracted from sweet potato starch, rich starch etc., will provide better water solubility, lower the oxygen permeability, increase water-vapour permeability (WVP), enhance flexibility and decrease the moisture content of the film.55
Proteins, which are amino acids linked by peptide bonds, have good mechanical and physical properties and are good raw materials for food coatings.56 Whey protein, gelatin, casein, soy protein, etc. are common proteins from animal and plant sources57 (Zink et al., 2016). One of the prominent sources of protein for edible films and coatings is whey protein. Edible films and coatings derived from whey proteins have been successfully produced and have demonstrated their multifunctional capabilities. Not only do they serve as protective barriers, but they can also offer additional functionalities, such as antimicrobial and antioxidant properties.58 Furthermore, films produced using whey protein isolate (WPI) have shown excellent oxygen and aroma barrier properties. However, they tend to exhibit poor mechanical properties, necessitating the incorporation of other ingredients or modifications to improve their structural integrity.59 Recent advancements in the composition of gelatin-based edible films and coatings have been observed, with researchers exploring the incorporation of nanoparticles to enhance the film's properties. Such modifications aim to improve the film's mechanical strength, barrier properties, and overall functionality. Furthermore, gelatin-based films are being explored not only for their protective capabilities but also for their potential as active and smart edible films. These innovative films can respond to environmental changes, offering dynamic protection to packaged food.60
Casein, a milk protein, has been explored as a base for edible films and coatings due to its biodegradable and biocompatible nature.61 A study by M. W. Apriliyani et al.62 investigated the properties of casein-based edible films/coatings with the addition of chitosan. The study found that the thickness of the casein–chitosan edible film ranged between 0.076 and 0.087 mm. The treatment with 4% chitosan solution resulted in the lowest moisture content (29.24%), swelling degree (5.48%), and opacity (0.078%). The study concluded that the concentration of chitosan solutions influences the moisture content, swelling, and opacity of the edible films/coating. Al-Hashimi et al.63 discussed the potential of protein-based edible films, including those made from casein, collagen, gelatin, corn zein, wheat gluten, soy protein, and mung bean protein. The review highlighted that protein edible films possess significant gas barrier properties compared to films made from polysaccharides and lipids. However, they also mentioned the challenges associated with the water vapour permeability and tensile strength of these films.63
Lipids are natural hydrophobic polymers, and due to their natural property, the lipid-based films and coatings work as effective barriers against moisture loss further extending the shelf-life.64 Neutral lipids, fatty acids, waxes, and resins can be used as edible packaging materials. But also due to their physical properties, lipids have limited ability to form independent and cohesive films. Therefore, lipids should be used as composite films.65
Bio-based degradable plastics for sustainable packaging
As can be alluded to in the preceding section, the edible packaging function lends itself well for utilization and incorporation of sustainable materials. For packaging materials that can provide the other functions highlighted, the bio-based degradable plastics may offer a suitable sustainable avenue.
Types of bio-based biodegradable “plastics”
Unlike conventional plastics, which are derived from non-biodegradable fossil fuels, bio-based biodegradable materials have emerged as a promising tool in reducing the potential carbon footprint associated with the use of petroleum-based plastics.66,67 The development of bio-based biodegradable materials is driven by the goal of achieving green and sustainable development. These bio-based materials are either fully or partially made from biological resources such as starch and sugars, while biodegradability refers to the capacity of a material to be decomposed by natural processes, such as microbial activity, into simpler, non-toxic compounds.68,69 It's crucial to understand that not all bio-based plastics are biodegradable, and conversely, not all biodegradable plastics are necessarily bio-based. However, bio-based biodegradable plastics uniquely combine both these environmentally friendly properties. Among the various types of bio-based biodegradable materials, polyhydroxyalkanoate (PHA) and polylactic acid (PLA) are particularly well-studied.70
Polyhydroxyalkanoates, commonly known as PHAs, are a class of naturally synthesised polyesters. They are produced through the fermentation of a carbon substrate by microorganisms under conditions where nitrogen is limited and carbon is in excess.71 PHAs have gained significant attention as a potential replacement for conventional PVC, especially in food packaging applications. Their properties, such as being water-insoluble, hydrolytic degradation resistant, biodegradable, biocompatible, and non-toxic, make them an attractive alternative.72,73 PHAs can be categorised into three major groups based on the length of their carbon chain: short-chain PHAs consisting of three to five carbon atoms, medium-chain PHAs having six to fourteen carbon atoms, and long-chain PHAs containing more than fifteen carbon atoms.74 Short-chain-length PHAs, scl-PHAs, are known for their stiffness, brittleness, high crystallinity and low melting temperature of around 180 °C, which can be a limitation in some packaging applications.75 Medium-chain-length PHAs, mcl-PHAs, tend to exhibit more flexibility, less crystallinity and low melting temperature compared to scl-PHAs. This makes them more suitable for packaging applications that require a degree of pliability, such as films and bags. Long-chain-PHAs, however, are least studied.76,77
Polylactic acid (PLA) is emerging as a sustainable material, distinguished by its biodegradable nature and derivation from renewable resources like corn starch or sugarcane.78 The production of PLA involves fermentation of these sugars to produce lactic acid, which is then polymerized to form PLA.79 The biodegradability and eco-friendliness of PLA position it as an ideal replacement for PC in food packaging applications.80 Its great applicability is largely due to PLA's mechanical properties and safety profile; moreover, it may be cheaper than commercialised plastics as it even can be extracted from food waste.80,81 In terms of material characteristics, PLA exhibits high tensile strength and rigidity, although it can be somewhat brittle in its unmodified form.82,83 Its thermal behaviour is also noteworthy, with a relatively low melting point compared to traditional plastics.83 This characteristic can be advantageous or limiting, depending on the specific application. These properties have spurred ongoing research aimed at enhancing PLA's toughness and heat resistance, often through blending with other polymers or incorporating additives.
Enhancing the packaging functions of these bioplastics
Recent studies have explored various aspects of polysaccharides in reinforcing materials like PLA. In the realm of polysaccharide enhancements, Nazrin et al.84 explored the potential of sugar palm starch, reinforced with sugar palm crystalline nanocellulose, blended with PLA. This innovative approach aimed to develop materials with superior water barrier and mechanical properties, potentially replacing conventional plastics. The research revealed that adjusting the PLA content in these blends could significantly influence their water vapour permeability and impact strength, highlighting the effectiveness of polysaccharides in enhancing the properties of bioplastics.
Ho et al.85 found that incorporating bamboo charcoal (BC) particles into polylactic acid (PLA) significantly improves the composite's mechanical, thermal, and optical properties, particularly when BC concentration is kept at or below 7.5 wt%. The study highlights that BC/PLA composites outperform pure PLA in various aspects but cautions against excessive BC content (over 7.5 wt%), which can lead to reduced performance due to particle aggregation. Furthermore, these composites show notable UV degradation resistance, making them suitable for UV-exposed applications like food storage.
Lima et al.86 explored environmentally friendly biocomposites for rigid packaging, utilising poly(lactic acid) (PLA) and mango seed waste, a by-product of the agro-industry. The study focused on creating six different biocomposites through extrusion/injection processes, incorporating up to 20% mango seed by-products into the PLA matrix. These biocomposites underwent extensive analysis, including chemical, physical, and mechanical evaluations, and notable enhancements in mechanical and barrier properties were found, especially in the formulation with 20% integument, showing up to 38% increase in the elastic modulus.
De Freitas et al.87 studied how to use active extract and cellulose fibres extracted from rice straw to enhance PLA/starch bilayer films. They used up to 6% rice straw extract coping with the compression technique that showed that although the rice straw addition decreased bilayer film tensile strength with lower oxygen permeability, it enhanced the antioxidant activity. The research done by Mármol et al.88 explored the use of cellulose microfibers (MFs) obtained from kraft paper to reinforce PHA and PLA films. The results show that PHA films reinforced with MF are 23% stronger than neat PHA samples. The addition of MF also increases the crystallinity of PHA, which affects its hygroscopicity and water barrier properties.
The use of agro-waste and its challenges for sustainable food packaging
Agro-waste, a by-product from the food industry, is gaining considerable attention as an eco-friendly alternative to enhance the properties of biodegradable food packaging.89,90 This agro-waste, encompassing a diverse range from straw, stems, and fruit peels to shrimp shells and animal skins, has shown promising potential in augmenting the mechanical and various other material properties of sustainable packaging solutions. These materials can be broadly categorised into two primary sources for extraction: plant-based and animal-based. Plant waste derived from food waste encompasses a wide range of organic materials that are typically discarded before, during and after processing,91 while animal-based materials include skin, feathers and shells.
Plant-based agro-waste
In this subsection, various studies focus on the development of plant-based biodegradable packaging materials, utilising a range of agricultural by-products and natural waste materials. A summary of these innovative packaging materials is graphically presented in Fig. 1. These innovative approaches leverage the inherent properties of plant-derived fibres, starch, and extracts to create environmentally friendly packaging solutions. Fontes-Candia et al.91 demonstrated the innovative utilisation of Arundo donax lignocellulosic waste biomass in the production of highly porous and bioactive aerogels. These aerogels exhibit remarkable water and oil sorption capacities, comparable to those of chemically modified nanocellulose aerogels, thereby highlighting their functional effectiveness. Additionally, the study augmented these aerogels with significant antioxidant properties by incorporating extracts from A. donax biomass, particularly the stem extract generated through heating (S-HW), which is rich in polysaccharides and polyphenols. Furthermore, their ability to reduce colour loss and lipid oxidation in red meat during refrigerated storage positions these aerogels as promising candidates for bioactive food packaging materials.
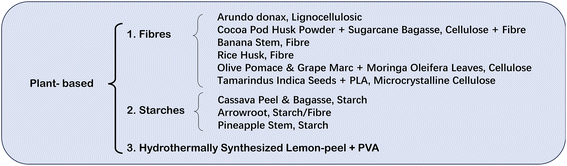 |
| Fig. 1 Plant-based biodegradable packaging materials. | |
Azmin et al.92 developed effective food packaging bioplastics using a blend of cellulose extracted from dried and powdered cocoa pod husk (CPH) and fibre derived from processed sugarcane bagasse, with a cellulose to fibre ratio of 75
:
25. These bioplastics exhibit reduced water absorption and possess good water vapour permeability, aiding in mold prevention and maintaining food freshness by minimising moisture transfer. The addition of a small amount of fibres to the bioplastic mix enhances its resistance to water, making it less brittle and more durable.
Ai et al.93 effectively demonstrated the use of banana stem fibres in creating cellulose films through ionic liquid dissolution, presenting a sustainable alternative to traditional packaging materials. These cellulose films exhibit significantly higher moisture vapour and gas transmission rates compared to commercial polyethylene films. This characteristic is beneficial for ethylene release, thereby aiding in delaying fruit ripening and extending shelf life at room temperature. This study not only offers a potential solution for preserving tropical fruits but also underscores the environmental benefits of these films, evidenced by their high biodegradability, with a mass residual rate of just 7.0% after four weeks in soil.
Srivastava et al.94 utilised rice husk fibres, an agricultural waste, and benzalkonium chloride (BKC), an antimicrobial agent, to enhance corn starch-based biocomposite films. The films, particularly those with 20% rice husk fibre and 0.05% BKC, demonstrated excellent properties for active food packaging, including increased thickness, reduced moisture content, and enhanced water solubility, alongside significant improvements in tensile strength and the elastic modulus. Additionally, the film exhibited effective antimicrobial properties against both Gram-positive and Gram-negative bacteria and was fully biodegradable within 30 days under composting conditions.
Thuppahige et al.95 highlighted the potential of cassava peel and bagasse, byproducts of cassava processing, as viable materials for developing biodegradable food packaging. The study showed that the morphology and thermal properties of the starch granules in these byproducts are comparable to those of commercial cassava starch, with minor variations in the chemical composition and crystalline structure. Notably, the presence of crystalline phases such as α-amylose dihydrate, quartz (SiO2), and whewellite (CaC2O4·H2O) in nanocrystalline forms suggests their suitability as reinforcement agents.
Barzan et al.96 utilised natural extracts from food industry waste (olive pomace and grape marc) and Moringa oleifera leaves in developing innovative cellulose-based biopolymer packaging systems. Employing various extraction methods, the research highlights the superior antioxidant efficacy of methanol and acetone extracts in prolonging the shelf life of food products, particularly in preventing lipid peroxidation in ground beef. Jayakumar et al.97 presented an active food packaging solution by incorporating lemon peel-based carbon quantum dots (LCQDs) into polyvinyl alcohol (PVA) films. The results showed excellent fluorescence, enhanced antioxidant and antibacterial activities against a range of pathogens such as S. aureus and E. coli, and improved mechanical and water vapour barrier properties. Notably, with 3% LCQDs in the PVA films, there was a significant boost in their UV blocking capabilities (UVA, UVB, and UVC) and radical scavenging activity, showcasing their potential as effective UV-blocking, antioxidant, and antibacterial packaging solutions.
Divakaran et al.98 presented an innovative use of agro-waste Tamarindus indica seeds for the extraction and characterization of microcrystalline cellulose (TSMCC) and its application in biofilm formulation. The isolated TSMCC exhibited desirable properties like high crystallinity, moderate density, and thermal stability, making it suitable for lightweight applications. Moreover, with 5% TSMCC applied into a polylactic acid (PLA) matrix, it significantly enhances the mechanical and thermal properties of the biofilms, suggesting an increased crystalline index, tensile strength, and thermal stability.
Tarique et al.99 developed glycerol plasticized thermoplastic arrowroot starch/arrowroot fibre (TPAS/AF) biocomposite films with varying AF loadings (0, 2, 4, 6, 8, and 10%) to investigate the effect on film properties. The results indicated that AF significantly enhanced the thermal stability of the films, with the degradation temperature increasing from 298 to 313 °C. As the concentration of AF increased, there was a notable decrease in both light transmittance and the linear burning rate, with the 8% AF-loaded TPAS/AF film exhibiting the fastest burning rate and shortest burn-out time. Moreover, the biodegradability of the films improved due to the hydrophilic nature of the AF, accelerating the biodegradation process. However, the research also revealed that both TPAS and TPAS/AF films exhibited no significant antibacterial activity against common pathogens such as S. aureus, E. coli, and B. subtilis.
Bumrungnok et al.100 developed water-resistant and biodegradable flexible films using pineapple stem starch (PSS), glycerol, and citric acid. The high amylose content in these films imparts a degree of crystallinity, contributing to their water resistance. A key finding is that the mechanical properties and gas permeability of the films, including oxygen, carbon dioxide, and water vapour transmission, can be effectively modulated by varying the glycerol content. Increased glycerol levels result in softer, more extensible films with higher gas permeability. The practical application of these films was evidenced in their ability to delay the ripening of bananas for over a week when coated with the PSS solution.
Animal-based agro-waste
This subsection focused on the development of bio-based packaging materials, primarily through the extraction of valuable components from animal-based sources and their subsequent incorporation into film-forming materials. This overarching theme involves utilising by-products or waste materials from various animal sources, such as fish, eggshells, shrimp shells, milkfish scales, and chicken feathers to create environmentally friendly and biodegradable packaging solutions. A summary of these innovative packaging materials, based on the different categories of raw materials, is graphically presented in Fig. 2.
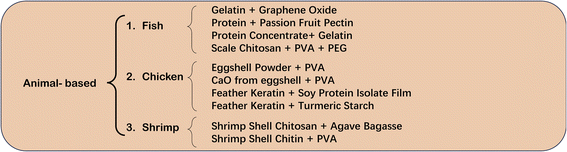 |
| Fig. 2 Animal-based biodegradable packaging materials. | |
Adilah et al.101 explored the potential of enhancing fish gelatin as a bio-packaging material through the incorporation of graphene oxide (GO). By preparing gelatin composite films with different concentrations of GO from 0.5% w/w to 2.0% w/w and applying different sonication times (1.5 and 2.5 hours), they demonstrate a notable improvement in the films' mechanical, optical, and water barrier properties. The result reveals that higher concentrations of GO, particularly at a 2.0% level with extended sonication time, significantly enhance the tensile strength and Young's modulus of the films, making them up to 34% and 76% stronger, respectively. Additionally, these modified films exhibit superior UV and light barrier properties and reduced moisture content compared to control gelatin films.
Florentino et al.102 introduced a sustainable packaging material derived from fish by-product proteins and passion fruit pectin. The research identified that 5% fish by-product protein (FBP) from Serra Spanish Mackerel (Scomberomorus brasiliensis) and 3% pectin from yellow passion fruit (Passiflora edulis flavicarpa) yield the most effective results. The resulting bioplastic demonstrates promising physical, mechanical, and thermal properties, being homogeneous, strong, and flexible, and exhibiting low solubility and water vapour permeability.
Athanasopoulou et al.103 investigated the use of fish waste, a by-product of the fishing industry, to develop biodegradable food packaging materials. This study focused on the upcycling of gilthead seabream discards to extract fish protein concentrate (FPC) and combine it with gelatin to create film-forming solutions. The films exhibit noteworthy properties, including hydrophobicity, as indicated by reduced contact angles, and desirable optical qualities, maintaining transparency and colorlessness, crucial for food packaging. While adding FPC leads to a decrease in tensile strength compared to pure gelatin films, it increases the elongation values.
Wu et al.104 explored the use of eggshell powder (ESP) as a biological filler in poly(vinyl alcohol) (PVA) to create biocomposite films. Their study investigated the integration of ESP into PVA through solution blending and casting, focusing on the effects of varying ESP content (ranging from 0 to 50 wt% dry PVA) on the structure and properties of the resultant films. The results showed that adding 30 wt% ESP into PVA significantly enhances the films' mechanical, thermal, and water vapour barrier properties. At this concentration, the films demonstrate improved tensile strength, elongation at break, and reduced water vapour permeability, attributable to the strong adhesion and well-dispersed ESP particles within the PVA matrix. However, the study also noted that excessive ESP content can lead to particle aggregation, adversely affecting the films' performance.
Another research group conducted similar experiments but used calcium oxide extracted from eggshells. Varadarajan and Nagaraaj's105 research focused on enhancing the physical and chemical properties of PVA packaging materials by converting calcined eggshell waste to calcium oxide (CaO). It was found that at a calcium oxide content of 75 mg, film properties such as thickness, solubility, water content, water absorption, water vapour transmission rate, tensile strength and elongation were improved. The degradation of the films under natural soil conditions was also studied in depth and the results showed that the dissolved matter in the films could be reused for planting purposes.
De Oca-Vásquez et al.106 developed nanocomposite chitosan films, leveraging the antimicrobial properties of agave bagasse and shrimp shells, for potential use in food preservation and packaging. Chitosan, extracted from shrimp shell waste, and cellulose nanofibers, obtained from agave bagasse, form the foundation of these films. The study further enhanced the antimicrobial efficacy by integrating various concentrations of silver nanoparticles (AgNPs). Key findings include a decrease in moisture content and water solubility with the addition of silver nanoparticles and nanofibers, along with an increase in mechanical strength and the elastic modulus due to the nanofibers. Moreover, the reduced transparency and increased opacity of the films, beneficial for limiting UV and visible light transmission, thus potentially reduce food deterioration. However, the inclusion of silver nanoparticles reduces the mechanical properties in proportion to their concentration.
Rasak et al.107 conducted a study on the development of high degradation bioplastics derived from milkfish (Chanos chanos) scale waste, and in their research, chitosan (CS) was extracted from milkfish scales and used to produce a composite bioplastic (CS/PVA/PEG), aiming to evaluate its impact on mechanical properties and degradation time. The study found a notable increase in tensile strength in the bioplastics with higher chitosan content, and the bioplastics demonstrated rapid biodegradation, completely decomposing within 72 hours in soil for the CS-based samples and in seawater for the chitin-based samples. The XRD spectra indicated that chitosan significantly affects the structural properties of the bioplastics, while FTIR analysis revealed the presence of hydrogen bonds between chitosan and other components, impacting tensile strength and elongation break.
Li et al.108 presented an innovative approach in the development of bio-based packaging materials, focusing on enhancing soy protein isolate (SPI) films by incorporating chicken feather keratin (FK). By utilising waste chicken feathers as a source of FK, the study demonstrated the formation of a cross-linked network structure within the SPI matrix through the reaction of disulfide bonds. This method not only substantially improves the mechanical properties of the SPI film, with a 242% increase in tensile strength and a 152% enhancement in toughness, but also boosts its thermal stability and water resistance. Remarkably, the FK-modified SPI can replace up to 40% of the film composition while maintaining 100% biodegradability.
Oluba et al.109 explored the development of biocomposite films using keratin extracted from chicken feather waste and starch derived from turmeric. The research illustrated that the addition of keratin significantly enhanced the tensile strength, elongation at break, and surface smoothness of the starch-based composites while reducing their transparency and solubility. The physicochemical analysis of turmeric starch demonstrates notable characteristics like high moisture content and a moderate amylose content. The films show impressive degradation capabilities, with over 20% mass decay after 12 days of burial in soil, highlighting their environmental friendliness. Furthermore, the keratin-starch biocomposite film demonstrates reduced moisture content and high tensile strength, indicating improved stability.
Qian et al.110 presented an approach to preserve food through the development of bio-based packaging films, utilising liquefied ball-milled shrimp shell chitin (LBSC) and polyvinyl alcohol (PVA) with an incorporation of β-cyclodextrin/cinnamaldehyde (β-CD/CA) inclusion complexes. The research showed that with a 3 wt% β-CD/CA/LBSC/PVA blend film particularly stands out with optimised mechanical properties, including a tensile strength of 41.5 MPa and an elongation at break of 810%, along with improved surface smoothness, water resistance, and UV-blocking capabilities. Importantly, these films demonstrate considerably enhanced antibacterial and antifungal activities, significantly extending the shelf life of cherry tomatoes wrapped in them, compared to films without β-CD.
Bottlenecks in utilising industry food waste to produce food packaging materials
In the quest for sustainable solutions in the packaging industry, the utilisation of food waste as a resource for producing biodegradable packaging materials has garnered significant attention. This innovative approach not only promises environmental benefits by reducing waste and reliance on non-renewable resources but also aligns with the growing global emphasis on circular economy principles. However, transforming this concept into a practical and scalable reality involves navigating a series of complex challenges. These challenges span the entire lifecycle of packaging production, from the initial collection of food waste to the final application of the produced materials. Understanding these hurdles is crucial for assessing the feasibility and potential impact of using food industry waste in packaging applications.
The first step in utilising food leftovers for packaging is the collection of waste. This process can be challenging due to the diverse sources of food waste, ranging from crop processing to household leftovers.111 Efficient collection systems need to be in place to gather sufficient quantities of waste materials that can be used in material production processing. Then, once the waste has been collected, the waste must be separated into its various components. This separation is crucial for extracting valuable materials that can be used in packaging. However, the mixed nature of food waste can make this process complex and labour-intensive. Even though people overcome those challenges, the technologies used to extract useful materials from food waste must be efficient and cost-effective. This includes processes for breaking down organic matter and isolating components that can be used in bioplastic production. The development and optimization of these technologies are ongoing challenges.9
Once the materials have been produced, the next thing to consider is whether these bio-based packaging materials derived from food waste meet the same food safety requirements as conventional packaging materials. This includes ensuring that the packaging does not contain any harmful substances or lead to contamination of the food it contains.112 The quality and performance of packaging materials made from food waste must be comparable to traditional materials. This includes factors like durability, barrier properties, and suitability for various types of food products. Achieving this level of performance can be challenging when working with varied and inconsistent waste streams.113
The last thing that needs to be considered is that the process of converting food waste into packaging materials must be economically viable. This includes considerations of the cost of collection, separation, extraction, and manufacturing processes. The economic feasibility is a significant factor in determining whether such initiatives can be scaled up and widely adopted.114
Challenges in the commercialization of biodegradable food packaging
A survey of relevant intellectual properties
The patents presented in Table 1 showcase an extensive list of recent intellectual properties relevant to innovative biodegradable material technologies for sustainable food packaging solutions. These patents encompass a variety of biodegradable components such as polysaccharides, plant fibres, and synthetic polymers like polylactic acid (PLA), all designed to decompose under specific conditions. The approaches for creating these materials range from using natural fibres and extracts to engineering new polymers, often combining different substances to enhance properties like strength, flexibility, and barrier performance. The intended functions of these materials are tailored towards packaging needs, offering features such as antimicrobial effects, moisture barriers, and food freshness preservation. Some of these patented materials have successfully transitioned to the market, manifesting as commercial products like biodegradable containers and egg cartons. Determining the commercialisation status of a patent can be challenging when a direct partner or market application is not listed in the document. Patents originating from universities, which are often encouraged by government initiatives, might not always immediately transition to commercial products.
Table 1 A survey on intellectual properties relevant to biodegradable and compostable food packaging materials
Patent no. |
Title |
Main material(s) |
Company/current assignee |
Commercialization |
WO2021007579A1 (ref. 115) |
Biodegradable material |
Polysaccharides – alginic acid polymer, hydroxypropyl methylcellulose polymer |
StenCo, LLC |
StenCoat |
CN104321193B116 |
Packaging for foodstuff coating based on Sargassum |
Polysaccharides – Sargassum extract, starch, paper fiber |
Mantrose Haueser Co Inc. |
VerdeCoat® |
CN105646951B117 |
The preparation method of functional solvable edible composite membranes |
Polysaccharides, protides and starch |
Yeyun Biotechnology (Wuhan) Co., Ltd |
|
WO2017163095A1 (ref. 118) |
Natural fiber or fibrous material based completely biodegradable food containers |
Fibers or fibrous materials – natural fibers (jute, coir, banana, and/or bagasse) |
|
|
AU2017340017B2 (ref. 119) |
Biodegradable and compostable food packaging unit from a moulded pulp material, and method for manufacturing such food packaging units |
Pulp – fibrous |
Huhtamaki Mold Fibre Technology B.V. |
Huhtamaki egg cartons |
CN113234307B120 |
Fully degradable antibacterial food packaging film and preparation method thereof |
Starch, biodegradable polyester |
Shandong Agricultural University |
|
CN101935440B121 |
Starch/polylactic acid antibacterial activity packaging material as well as preparation method and application thereof |
Starch, polylactic acid and non-specific antibacterial agent |
South China University of Technology SCUT |
|
US20170049059A1 (ref. 122) |
Method for producing grown materials and products made thereby |
Mycelium |
Ecovative Design LLC |
Mushroom® packaging by Ecovative |
CN101899173A123 |
Edible starch-based food packaging film and preparation method thereof |
Stabilization cross-linking starch |
Shandong Agricultural University |
|
CN101955670B124 |
Gelatin–chitosan composite food packaging film and preparation method thereof |
Gelatin, chitosan, and glycerine |
Fuzhou University |
|
CN109294193B125 |
Biodegradable food packaging material and preparation method thereof |
Plant fibers |
Vanjoin Wuhan New Material Co Ltd |
|
CN110183739A126 |
A kind of degradable scleroglucan natural complex fresh-keeping film and the preparation method and application thereof |
Scleroglucan, potato starch, glycerol |
Tianjin University of Science and Technology |
|
US11708492B2 (ref. 127) |
Biodegradable and edible bioplastic from renewable plant based polymer for packaging and the manufacturing method thereof |
Seaweed, tubers from potato, cassava, and sweet potato, protein isolate from soy and gluten |
Noryawati Mulyono |
Biopac |
JP6955774B2 (ref. 128) |
Hydrophobic thermoplastic starch composite material and its manufacturing method |
Starch |
Green World Biotech Materials Co Ltd |
Green World Biotech Materials Co Ltd – PlasFree® SC Series |
CN110791069A129 |
Full-degradable high-barrier composite material for flexible package and preparation method and application thereof |
Biodegradable matrix resin |
Baixin Fujian New Material Technology Co Ltd |
|
CN113354853B130 |
Biodegradable high-barrier-property antibacterial composite membrane and preparation method thereof |
Polylactic acid, cellulose nano-fiber |
Shandong Century Sunshine Paper Group Co Ltd, Qingdao University of Science and Technology |
|
CN110437590B131 |
Composite nano-material modified starch-based biodegradable food packaging film and preparation method thereof |
Composite bio-based material – the composite bio-based material consists of PLA, PBAT and PCL, wherein the weight ratio of PLA to PBAT to PCL is 1 : 4 : 1; starch |
Huazhong Agricultural University |
|
CN109438937B132 |
Antibacterial PLA-based degradable food packaging material and preparation method thereof |
Nano titanium dioxide loaded active carbon, polylactic acid |
Vanjoin Wuhan New Material Co Ltd |
|
CN111171385B133 |
Starch cross-linked tea polyphenol antibacterial degradable food packaging material and preparation method thereof |
Starch solution and tea polyphenol solution |
Hainan University |
|
KR102372332B1 (ref. 134) |
Moisture barrier biodegradable film, packaging sheet and sticker comprising the film |
(1) Biodegradable film base: polylactic acid (PLA) resin film/polybutylene adipate-co-terephthalate (PBAT) resin film/polycaprolactone (PCL) resin film/polybutylene succinate (PBS) resin film |
|
|
(2) Water-soluble acrylic coating layer |
CN111019170A135 |
Preparation method of completely degradable biomass food packaging film |
Polylactic acid/polyethylene glycol/nano-cellulose |
Dalian Polytechnic University |
|
CN112300542A136 |
Nanoscale plant fiber modified biodegradable composite material and preparation method and application thereof |
Nano-scale plant fiber and PLA |
Individual |
|
CN113583422A137 |
Biodegradable preservative film with antibacterial function and preparation method thereof |
Matrix resin, chitosan |
Individual |
|
KR102404216B1 (ref. 138) |
Biodegradable packaging, preparation method thereof, security pack and security method of mobile devices |
PHA/PLA |
|
|
KR20210103322A139 |
Biodegradable food packaging materials from algae and method for producing the same |
Seaweed extract, agar, gelatin, carrageenan or mixture |
|
|
CN112063135B140 |
Preparation method of antioxidant and antibacterial biodegradable food packaging material |
Cinnamoyl chloride (Cc) modified cellulose nanocrystals (CNCs) |
Zhejiang Sci Tech University ZSTU |
|
CN113698646B141 |
Transparent moisture-preserving antibacterial degradable packaging film and preparation method and application thereof |
(1) Inner active moisturizing layer: biodegradable substrate: PVA |
Jiangnan University |
|
(2) Transparent supporting layer: polylactic acid (PLA) particles |
(3) Outer barrier moisture-retention layer: modified polyvinyl alcohol (PVA), nano-silicon dioxide (SiO2) |
CN113061270B142 |
Biodegradable UVA photoresponse type controlled-release antibacterial food packaging film and preparation method and application thereof |
ZIF-8 micron particles carrying riboflavin |
South China University of Technology SCUT |
|
KR102422234B1 (ref. 143) |
Chitosan membrane for food packaging, its manufacturing method and eco-friendly food packaging materials using the same |
Electro-spun chitosan nanofiber |
|
|
CN113621153A144 |
Plant extract synthesized nano-silver multifunctional degradable food packaging material and preparation method thereof |
Plant extract solution and silver nitrate |
Jimei University |
|
WO2021017030A1 (ref. 145) |
Coffee ground modified biodegradable composite material, and preparation method and application therefor |
Polylactic acid, biodegradable copolyester, nano-coffee grounds |
|
|
CN109265752A146 |
A kind of antibacterial and biodegradable food package box and preparation method thereof |
Starch, cellulose, chitosan, polyhydroxyalkanoate, polylactic acid and polycaprolactone |
Guangxi University of Chinese Medicine |
|
CN110358264B147 |
Bio-based environment-friendly packaging bag and preparation method thereof |
Corn starch, PBAT, PLA |
Qinhuangdao Longjun Environmental Protection Industrial Development Co Ltd, Changzhou Longjun Skypurl Environment Protection Technology Co Ltd |
|
CN111875855A148 |
Eugenol-containing curdlan-based antioxidant film and preparation method thereof |
Polysaccharides |
Taixing Dongsheng Bio Tech Co Ltd, Nanjing Agricultural University |
|
CN110655688A149 |
Hydrophobic food packaging film and preparation method thereof |
Low methoxyl pectin, chitosan, glycerol, acetic acid and lotus leaf cutin |
Minnan Normal University |
|
CN115636961A150 |
Antibacterial and antioxidant gelatin-based composite membrane and preparation method thereof |
Gelatin-based composite membrane with cinnamon essential oil |
Tianjin University of Science and Technology |
|
The gaps between scientific research and intellectual properties
Comparing the biodegradable food packaging materials collated in Table 1 and the discussion earlier in this review, it appears that the types of material in academic research (such as bio-based polymers including PLA and starch-based composites) have made the transition to, and may be of commercial interest. However, many of the more advanced functionalities and properties explored in the scientific communities have yet to be taken up in the intellectual properties space. For example, the bulk of the intellectual properties reviewed focuses mainly on providing a sustainable means to basic PCCC packaging functions (Fig. 3). This is followed by about 36% of the intellectual properties focusing on active packaging which involves the release of active agents. To the best of our knowledge, we did not encounter intellectual properties focusing on active packaging based on active absorption. There were also no reports on enhanced real-time monitoring. Only about 8% of the intellectual properties reviewed focused on sustainable edible films.
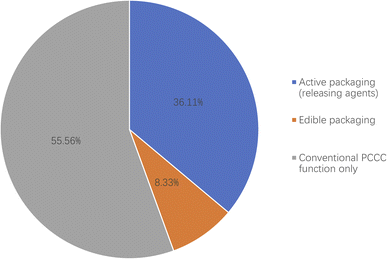 |
| Fig. 3 Snapshot of intellectual properties relevant to biodegradable and compostable food packaging materials. | |
Despite growing awareness and efforts to produce sustainable food packaging materials, the production of biodegradable plastics still accounts for less than 1% of total plastic production, as reported by European Bioplastics in 2023.151 This disparity highlights significant gaps where further research is needed and where the translation of research into practical, commercial applications has been notably slow or challenging. A key area of ongoing research is the development of new bio-based materials for packaging. However, a substantial gap exists in translating these materials into commercially viable products. Challenges include ensuring that these materials meet essential performance standards for food packaging, such as durability, barrier properties, and food safety.152,153 Additionally, for bio-based packaging materials to be market-ready, they need to be not only bio-based and biocompatible but also biodegradable and approved for food contact. Navigating the complex regulatory landscape and obtaining the necessary approvals pose a significant hurdle in moving from research to market.
A notable research gap identified by González-López et al.154 is in scaling up the production of packaging materials based on natural polymers. Technologies that are effective on a laboratory or pilot scale often face challenges when scaled up to industrial levels. For example, as Jeevahan et al.155 pointed out, scaling up edible food packaging technology to industrial levels faces notable difficulties, particularly in replicating the precise and controlled conditions of laboratory settings. Challenges include producing continuous films without interruptions, achieving uniform film thickness, and reducing lengthy drying times that are impractical for high-volume production. Scaling up starch-based biodegradable plastics for industrial production is fraught with challenges.156 These materials inherently possess weaker mechanical and barrier properties compared to traditional plastics. Conventional production techniques like casting are not viable for large-scale manufacturing due to excessive energy requirements and difficulties in achieving consistent product quality. Alternatives such as extrusion and molding, while scalable, involve substantial equipment investment and tend to produce materials with poor qualities compared to traditional plastics. This issue is compounded by the fact that, as shown in Table 1, only a few patents have been spearheaded by commercial entities (commercial success of the University patents listed are, however, not known), despite the original materials being readily accessible and easy to handle in large quantities.
Furthermore, the biodegradable nature of these materials introduces new considerations for end-of-life disposal. End-of-life options for conventional packaging materials include landfill disposal, incineration, recycling, biodegradation, and composting. However, a clear standard for recycling food-waste-based packaging has not yet been established. While aerobic biodegradation appears as a promising solution for these materials, the effectiveness of this degradation method and the consistency of biodegradability enhancement from added materials during experimentation remain variable.157 More research is needed into the practicality and efficiency of these disposal methods to ensure that they are feasible on a large scale and do not create new environmental problems, as highlighted by Cristofoli et al.89 Market acceptance and consumer preferences also play a critical role in the success of bio-based packaging solutions. Even when scientific research leads to innovative products, a gap often exists in market acceptance. Consumer preferences, awareness, and willingness to pay for sustainable packaging options significantly influence the success of these innovations, as observed by Moshood et al.112
Conclusion
This review addressed the transition of food packaging from traditional petroleum-based plastics to sustainable, bio-based alternatives. The imperative driving this shift is the dual objective of preserving food quality while mitigating environmental impact. The review delved into the practical applications of bio-based biodegradable materials in the food packaging industry, juxtaposing theoretical knowledge with real-world scenarios. It explores the basic functions of food packaging, including protection, preservation, containment, communication, and convenience, and extends to innovative approaches like smart packaging technologies. The review highlighted various studies on the development of biocomposite films using agricultural byproducts, demonstrating their enhanced properties like thermal stability, antimicrobial activity, and biodegradability. Furthermore, the review discussed the challenges and prospects of commercializing bio-based materials in food packaging, making comparison with intellectual properties filed in this area. Future research will need to focus on enhancing the mechanical properties and barrier functions of bio-based packaging materials to match or surpass those of traditional plastics. This involves not only scaling up production but also refining the biodegradation process to ensure environmental safety post-use. A particular issue that may require further attention is the sufficiency and consistency of agro-waste sources. Ensuring a reliable and consistent supply of these materials is essential, as their availability is crucial for the commercial viability of bio-based packaging in food applications.
Author contributions
AY: conceptualization, writing – original draft, and writing – review & editing; MWW: conceptualization, supervision, and writing – review & editing.
Conflicts of interest
We declared that there is no conflict of interest.
References
- M. S. Alamri, A. a. A. Qasem, A. A. Mohamed, S. Hussain, M. A. Ibraheem, G. Shamlan, H. a. S. Alqah and A. S. Qasha, Food packaging's materials: A food safety perspective, Saudi J. Biol. Sci., 2021, 28, 4490–4499, DOI:10.1016/j.sjbs.2021.04.047.
- A. Dodero, A. Escher, S. Bertucci, M. Castellano and P. Lova, Intelligent Packaging for Real-Time Monitoring of Food-Quality: Current and Future developments, Appl. Sci., 2021, 11, 3532, DOI:10.3390/app11083532.
- A. Agarwal, B. Shaida, M. Rastogi and N. B. Singh, Food Packaging Materials with Special Reference to Biopolymers-Properties and Applications, Chem. Afr., 2022, 6, 117–144, DOI:10.1007/s42250-022-00446-w.
- L. K. Ncube, A. U. Ude, E. N. Ogunmuyiwa, R. Zulkifli and I. Nongwe, Environmental Impact of Food Packaging Materials: A Review of Contemporary Development from Conventional Plastics to Polylactic Acid Based Materials, Materials, 2020, 13, 4994, DOI:10.3390/ma13214994.
- A. I. Osman, M. Hosny, A. S. Eltaweil, S. Omar, A. M. Elgarahy, M. Farghali, P. Yap, Y. S. Wu, S. Nagandran, K. Batumalaie, S. C. B. Gopinath, O. D. John, M. Sekar, T. Saikia, P. Karunanithi, M. H. M. Hatta and K. A. Akinyede, Microplastic sources, formation, toxicity and remediation: a review, Environ. Chem. Lett., 2023, 21, 2129–2169, DOI:10.1007/s10311-023-01593-3.
- G. Atiwesh, A. Mikhael, C. C. Parrish, J. Banoub and T.-A. T. Le, Environmental impact of bioplastic use: A review, Heliyon, 2021, 7, e07918, DOI:10.1016/j.heliyon.2021.e07918.
-
K. Dhamodharan, T. Konduru, M. Kannan and S. K. Malyan, in Emerging Trends to Approaching Zero Waste, Elsevier, 2022, pp. 243–264, DOI:10.1016/b978-0-323-85403-0.00011-6.
- H. El-Ramady, E. C. Brevik, Y. Bayoumi, T. Shalaby, M. E. El-Mahrouk, N. Taha, H. Elbasiouny, F. Elbehiry, M. Amer, N. Abdalla, J. Prokisch, S. Ø. Sølberg and W. Ling, An overview of Agro-Waste management in light of the Water-Energy-Waste Nexus, Sustainability, 2022, 14, 15717, DOI:10.3390/su142315717.
- S. A. Varghese, H. Pulikkalparambil, K. Promhuad, A. Srisa, Y. Laorenza, L. Jarupan, T. Nampitch, V. Chonhenchob and N. Harnkarnsujarit, Renovation of Agro-Waste for Sustainable Food Packaging: A review, Polymers, 2023, 15, 648, DOI:10.3390/polym15030648.
- S. S. Ali, E. A. Abdelkarim, T. Elsafmahy, R. Al-Tohamy, F. Li, M. Kornaros, A. Zuorro, D. Zhu and J. Sun, Bioplastic production in terms of life cycle assessment: A state-of-the-art review, Environ. Sci. Ecotechnology, 2023, 15, 100254, DOI:10.1016/j.ese.2023.100254.
- C. S. Herrmann, S. Rhein and K. F. Sträter, Consumers' sustainability-related perception of and willingness-to-pay for food packaging alternatives, Resour., Conserv. Recycl., 2022, 181, 106219, DOI:10.1016/j.resconrec.2022.106219.
- D. Taufik, M. J. Reinders, K. Molenveld and M. C. Onwezen, The paradox between the environmental appeal of bio-based plastic packaging for consumers and their disposal behaviour, Sci. Total Environ., 2020, 705, 135820, DOI:10.1016/j.scitotenv.2019.135820.
- A. Aggarwal and H. Langowski, Packaging functions and their role in technical development of food packaging systems: Functional equivalence in yoghurt packaging, Procedia CIRP, 2020, 90, 405–410, DOI:10.1016/j.procir.2020.01.063.
-
F. A. Paine, The Packaging User's Handbook, Blackie Academic & Professional, Glasgow, 1st edn, 1991 Search PubMed.
-
G. L. Robertson, Food Packaging, CRC Press, Boca Raton, 2nd edn, 2005 Search PubMed.
- K. S. Marsh and B. Bugusu, Food Packaging—Roles, materials, and Environmental issues, J. Food Sci., 2007, 72(3) DOI:10.1111/j.1750-3841.2007.00301.x.
- L. M. Júnior, L. Coltro, F. B. H. Dantas and R. P. Vieira, Research on food packaging and storage, Coatings, 2022, 12, 1714, DOI:10.3390/coatings12111714.
- M. A. Pascall, K. DeAngelo, J. Richards and M. B. Arensberg, Role and importance of functional food packaging in specialized products for vulnerable populations: Implications for innovation and policy development for sustainability, Foods, 2022, 11, 3043, DOI:10.3390/foods11193043.
-
K. Verghese, H. Lewis, S. Lockrey and H. Williams, Final Report: The Role of Packaging in Minimising Food Waste in the Supply Chain, RMIT University, Melbourne, VIC, 2013, DOI:10.13140/2.1.4188.5443.
- K. B. Biji, C. N. Ravishankar, C. O. Mohan and T. K. S. Gopal, Smart packaging systems for food applications: a review, J. Food Sci. Technol., 2015, 52, 6125–6135, DOI:10.1007/s13197-015-1766-7.
- C. Vasile and M. Baican, Progresses in Food Packaging, Food Quality, and Safety—Controlled-Release antioxidant and/or Antimicrobial packaging, Molecules, 2021, 26, 1263, DOI:10.3390/molecules26051263.
- M. T. Vasuki, V. Kadirvel and G. P. Narayana, Smart packaging—An overview of concepts and applications in various food industries, Food Bioeng., 2023, 2, 25–41, DOI:10.1002/fbe2.12038.
-
J. P. Kerry, in Advances in Meat, Poultry and Seafood Packaging, Elsevier, 2012, pp. 522–564, DOI:10.1533/9780857095718.4.522.
-
Regulation – 450/2009 – EN – EUR-LEX, https://eur-lex.europa.eu/legal-content/EN/ALL/?uri=CELEX%3A32009R0450, accessed November 30, 2023 Search PubMed.
- C. Vilela, M. Kurek, Z. Hayouka, B. Röcker, S. Yildirim, M. D. C. Antunes, J. Nilsen-Nygaard, M. K. Pettersen and C. S. R. Freire, A concise guide to active agents for active food packaging, Trends Food Sci. Technol., 2018, 80, 212–222, DOI:10.1016/j.tifs.2018.08.006.
- T. Fadiji, M. Rashvand, M. O. Daramola and S. A. Iwarere, A review on antimicrobial packaging for extending the shelf life of food, Processes, 2023, 11, 590, DOI:10.3390/pr11020590.
- B. Malhotra, A. Keshwani and H. Kharkwal, Antimicrobial food packaging: potential and pitfalls, Front. Microbiol., 2015, 6 DOI:10.3389/fmicb.2015.00611.
- M. Pateiro, R. Domínguez, R. Bermúdez, P. E. S. Munekata, W. Zhang, M. Gagaoua and J. M. Lorenzo, Antioxidant active packaging systems to extend the shelf life of sliced cooked ham, Curr. Res. Food Sci., 2019, 1, 24–30, DOI:10.1016/j.crfs.2019.10.002.
- S. Sahraee, J. M. Milani, J. M. Regenstein and H. S. Kafil, Protection of foods against oxidative deterioration using edible films and coatings: A review, Food Biosci., 2019, 32, 100451, DOI:10.1016/j.fbio.2019.100451.
- N. Malekjani, R. Karimi, E. Assadpour and S. M. Jafari, Control of release in active packaging/coating for food products; approaches, mechanisms, profiles, and modeling, Crit. Rev. Food Sci. Nutr., 2023, 1–23, DOI:10.1080/10408398.2023.2228413.
- R. Becerril, C. NerÍN and F. Silva, Encapsulation systems for antimicrobial food packaging components: an update, Molecules, 2020, 25, 1134, DOI:10.3390/molecules25051134.
-
J. L. Koontz, Special delivery: controlled release of active ingredients from food and beverage packaging, Italian Packaging Technology Award (IPTA) Paper Competition, 2006 Search PubMed.
- M. W. Apriliyani, P. Purwadi, A. Manab, M. W. Apriliyanti and A. D. Ikhwan, Characteristics of Moisture Content, Swelling, Opacity and Transparency with Addition Chitosan as Edible Films/Coating Base on Casein, Adv. J. Food Sci. Technol., 2020, 18, 9–14, DOI:10.19026/ajfst.18.6041.
- K. K. Gaikwad, S. Singh and Y. S. Lee, Oxygen scavenging films in food packaging, Environ. Chem. Lett., 2018, 16, 523–538, DOI:10.1007/s10311-018-0705-z.
-
L. Vermeiren, L. Heirlings, F. Devlieghere and J. Debevere, in Novel Food Packaging Techniques, Elsevier eBooks, 2003, pp. 22–49, DOI:10.1533/9781855737020.1.22.
- E. Drago, R. Campardelli, M. Pettinato and P. Perego, Innovations in Smart Packaging Concepts for Food: An Extensive review, Foods, 2020, 9, 1628, DOI:10.3390/foods9111628.
- K. K. Gaikwad, S. Singh and A. Ajji, Moisture absorbers for food packaging applications, Environ. Chem. Lett., 2018, 17, 609–628, DOI:10.1007/s10311-018-0810-z.
- H. Cheng, H. Xu, D. J. McClements, L. Chen, A. Jiao, Y. Tian, M. Miao and Z. Jin, Recent advances in intelligent food packaging materials: Principles, preparation and applications, Food Chem., 2022, 375, 131738, DOI:10.1016/j.foodchem.2021.131738.
- P. A. Muller and M. Schmid, Intelligent Packaging in the food sector: A brief Overview, Foods, 2019, 8, 16, DOI:10.3390/foods8010016.
- T. Gao, Y. Tian, Z. Zhu and D. Sun, Modelling, responses and applications of time-temperature indicators (TTIs) in monitoring fresh food quality, Trends Food Sci. Technol., 2020, 99, 311–322, DOI:10.1016/j.tifs.2020.02.019.
- P. A. Muller and M. Schmid, Intelligent Packaging in the food sector: A brief Overview, Foods, 2019, 8, 16, DOI:10.3390/foods8010016.
- P. R. Salgado, L. Di Giorgio, Y. S. Musso and A. N. Mauri, Recent developments in smart food packaging focused on biobased and biodegradable polymers, Front. Sustain. Food Syst., 2021, 5 DOI:10.3389/fsufs.2021.630393.
- M. Ghaani, C. A. Cozzolino, G. Castelli and S. Farris, An overview of the intelligent packaging technologies in the food sector, Trends Food Sci. Technol., 2016, 51, 1–11, DOI:10.1016/j.tifs.2016.02.008.
- P. Patel, Edible packaging, ACS Cent. Sci., 2019, 5, 1907–1910, DOI:10.1021/acscentsci.9b01251.
- A. C. Guimarães, L. Abrunhosa, L. Pastrana and M. A. Cerqueira, Edible films and coatings as carriers of living microorganisms: A new strategy towards biopreservation and healthier foods, Compr. Rev. Food Sci. Food Saf., 2018, 17, 594–614, DOI:10.1111/1541-4337.12345.
- A. Kumar, M. Hasan, S. Mangaraj, M. Pravitha, D. K. Verma and P. P. Srivastav, Trends in Edible Packaging Films and its Prospective Future in Food: A Review, Appl. Food Res., 2022, 2, 100118, DOI:10.1016/j.afres.2022.100118.
- E. Díaz-Montes and R. Castro-Muñoz, Edible Films and Coatings as Food-Quality Preservers: An Overview, Foods, 2021, 10, 249, DOI:10.3390/foods10020249.
- S. Galus, E. A. A. Kibar, M. Gniewosz and K. Kraśniewska, Novel Materials in the Preparation of Edible Films and Coatings—A review, Coatings, 2020, 10, 674, DOI:10.3390/coatings10070674.
- A. Kumar, M. Hasan, S. Mangaraj, M. Pravitha, D. K. Verma and P. P. Srivastav, Trends in Edible Packaging Films and its Prospective Future in Food: A Review, Appl. Food Res., 2022, 2, 100118, DOI:10.1016/j.afres.2022.100118.
- J. Qu, S. Xu, Z. Zhang, G. Chen, Y. Zhong, L. Liu, R. Zhang, J. Xue and D. Guo, Evolutionary, structural and expression analysis of core genes involved in starch synthesis, Sci. Rep., 2018, 8, 12736, DOI:10.1038/s41598-018-30411-y.
- P. F. Muñoz-Gimena, V. Oliver-Cuenca, L. Peponi and D. López, A review on Reinforcements and Additives in Starch-Based Composites
for food packaging, Polymers, 2023, 15, 2972, DOI:10.3390/polym15132972.
- T. Jiang, Q. Duan, J. Zhu, H. Liu and L. Yu, Starch-based biodegradable materials: Challenges and opportunities, Adv. Ind. Eng. Polym. Res., 2020, 3, 8–18, DOI:10.1016/j.aiepr.2019.11.003.
- J. Tarique, S. M. Sapuan and K. Abdan, Effect of glycerol plasticizer loading on the physical, mechanical, thermal, and barrier properties of arrowroot (Maranta arundinacea) starch biopolymers, Sci. Rep., 2021, 11, 13900, DOI:10.1038/s41598-021-93094-y.
- T. Majeed, A. H. Dar, V. K. Pandey, K. K. Dash, S. Srivastava, R. Shams, G. Jeevarathinam, P. Singh, N. Echegaray and R. Pandiselvam, Role of additives in starch-based edible films and coating: A review with current knowledge, Prog. Org. Coat., 2023, 181, 107597, DOI:10.1016/j.porgcoat.2023.107597.
- M. M. Abe, J. R. Martins, P. B. Sanvezzo, J. V. Macedo, M. C. Branciforti, P. J. Halley, V. R. Botaro and M. Brienzo, Advantages and Disadvantages of Bioplastics Production from Starch and Lignocellulosic Components, Polymers, 2021, 13, 2484, DOI:10.3390/polym13152484.
- B. Cuq, N. Gontard and S. Guilbert, Proteins as agricultural polymers for packaging production, Cereal Chem., 1998, 75, 1–9, DOI:10.1094/CCHEM.1998.75.1.1.
- J. I. Zink, T. Wyrobnik, T. K. Prinz and M. Schmid, Physical, Chemical and Biochemical Modifications of Protein-Based Films and Coatings: An Extensive review, Int. J. Mol. Sci., 2016, 17, 1376, DOI:10.3390/ijms17091376.
- Ó. L. Ramos, J. Fernandes, S. I. Silva, M. Pintado and F. X. Malcata, Edible Films and Coatings from Whey Proteins: A Review on Formulation, and on Mechanical and Bioactive Properties, Crit. Rev. Food Sci. Nutr., 2012, 52, 533–552, DOI:10.1080/10408398.2010.500528.
- S. Kandasamy, J. Yoo, J. Yun, H. S. Kang, K.-H. Seol, H. W. Kim and J.-S. Ham, Application of Whey Protein-Based Edible Films and Coatings in food Industries: An updated overview, Coatings, 2021, 11, 1056, DOI:10.3390/coatings11091056.
- N. S. Said, N. K. Howell and N. M. Sarbon, A review on potential use of gelatin-based film as active and smart biodegradable films for food packaging application, Food Rev. Int., 2021, 39, 1063–1085, DOI:10.1080/87559129.2021.1929298.
-
B. T. O'Kennedy, Caseins, Handbook of Food Proteins, Elsevier eBooks, 2011, pp. 13–29, DOI:10.1533/9780857093639.13.
- M. W. Apriliyani, P. Purwadi, A. Manab, M. W. Apriliyanti and A. D. Ikhwan, Characteristics of Moisture Content, Swelling, Opacity and Transparency with Addition Chitosan as Edible Films/Coating Base on Casein, Adv. J. Food Sci. Technol., 2020, 18, 9–14, DOI:10.19026/ajfst.18.6041.
- A. G. Al-Hashimi, N. H. S. Al-Garory and S. J. A. Abbas, PROTEIN-BASED EDIBLE FILMS A REVIEW, Basrah J. Vet. Res., 2020, 18, 1–15, DOI:10.23975/bjvetr.2020.170623.
- S. Galus and J. Kadzińska, Food applications of emulsion-based edible films and coatings, Trends Food Sci. Technol., 2015, 45, 273–283, DOI:10.1016/j.tifs.2015.07.011.
- B. Yousuf, Y. Sun and S. Wu, Lipid and lipid-containing composite edible coatings and films, Food Rev. Int., 2021, 38, 574–597, DOI:10.1080/87559129.2021.1876084.
- X. Zhao, Y. Wang, X. Chen, X. Yu, W. Li, S. Zhang, X. Meng, Z. Zhao, T. Dong, A. Anderson, A. Aiyedun, Y. Li, E. Webb, Z. Wu, V. Kunc, A. J. Ragauskas, S. Ozcan and H. Zhu, Sustainable bioplastics derived from renewable natural resources for food packaging, Matter, 2023, 6, 97–127, DOI:10.1016/j.matt.2022.11.006.
- J. Zheng and S. Suh, Strategies to reduce the global carbon footprint of plastics, Nat. Clim. Change, 2019, 9, 374–378, DOI:10.1038/s41558-019-0459-z.
-
O. Goudouri, P. Balasubramanian and A. R. Boccaccını, Characterizing the degradation behavior of bioceramic scaffolds, Characterisation and Design of Tissue Scaffolds, Elsevier eBooks, 2016, pp. 127–147, DOI:10.1016/b978-1-78242-087-3.00006-7.
-
J. P. Greene, Bio-Based and Biodegradable Plastics, Automotive Plastics and Composites, Elsevier eBooks, 2021, pp. 149–174, DOI:10.1016/b978-0-12-818008-2.00020-9.
- K. Sudesh and T. Iwata, Sustainability of biobased and biodegradable plastics, Clean: Soil, Air, Water, 2008, 36, 433–442, DOI:10.1002/clen.200700183.
-
P. Basnett, S. Ravi and I. Roy, Natural bacterial biodegradable medical polymers: Polyhydroxyalkanoates, Science and Principles of Biodegradable and Bioresorbable Medical Polymers, Elsevier eBooks, 2017, pp. 257–277, DOI:10.1016/b978-0-08-100372-5.00008-8.
- Ł. Kaniuk and U. Stachewicz, Development and advantages of biodegradable PHA polymers based on electrospun PHBV fibers for tissue engineering and other biomedical applications, ACS Biomater. Sci. Eng., 2021, 7, 5339–5362, DOI:10.1021/acsbiomaterials.1c00757.
- J. R. Westlake, M. W. Tran, Y. Jiang, X. Zhang, A. D. Burrows and M. Xie, Biodegradable biopolymers for active packaging: demand, development and directions, Sustainable Food Technol., 2023, 1, 50–72, 10.1039/d2fb00004k.
- J. R. Xavier, S. T. Babusha, J. George and K. V. Ramana, Material Properties and Antimicrobial Activity of Polyhydroxybutyrate (PHB) Films Incorporated with Vanillin, Appl. Biochem. Biotechnol., 2015, 176, 1498–1510, DOI:10.1007/s12010-015-1660-9.
-
M. E. Gomes, H. S. Azevedo, P. B. Malafaya, S. Silva, J. M. Oliveira, G. Silva, R. S. J. Mano and R. L. Reis, Handbook of Biopolymers and Biodegradable Plastics, Elsevier eBooks, 2013, pp. 385–425, DOI:10.1016/b978-1-4557-2834-3.00016-1.
- A. B. Akinmulewo and O. Nwinyi, Polyhydroxyalkanoate: a biodegradable polymer (a mini review), J. Phys.: Conf. Ser., 2019, 1378, 042007, DOI:10.1088/1742-6596/1378/4/042007.
- B. Lukasiewicz, P. Basnett, R. Nigmatullin, M. Edirisinghe, J. C. Knowles and I. Roy, Binary polyhydroxyalkanoate systems for soft tissue engineering, Acta Biomater., 2018, 71, 225–234, DOI:10.1016/j.actbio.2018.02.027.
- E. Balla, V. Daniilidis, G. Karlioti, T. Kalamas, M. Stefanidou, N. D. Bikiaris, A. Vlachopoulos, I. Koumentakou and D. N. Bikiaris, Poly(lactic Acid): A Versatile Biobased Polymer for the Future with Multifunctional Properties—From Monomer Synthesis, Polymerization Techniques and Molecular Weight Increase to PLA Applications, Polymers, 2021, 13, 1822, DOI:10.3390/polym13111822.
- S. Riaz, N. Fatima, A. Rasheed, M. Riaz, F. Anwar and Y. Khatoon, Metabolic Engineered Biocatalyst: a solution for PLA based problems, Int. J. Biomater., 2018, 2018, 1–9, DOI:10.1155/2018/1963024.
- A. Z. Naser, I. Deiab and B. M. Darras, Poly(lactic acid) (PLA) and polyhydroxyalkanoates (PHAs), green alternatives to petroleum-based plastics: a review, RSC Adv., 2021, 11, 17151–17196, 10.1039/d1ra02390j.
- I. S. Fahim, H. Chbib and H. M. Mahmoud, The synthesis, production & economic feasibility of manufacturing PLA from agricultural waste, Sustainable Chem. Pharm., 2019, 12, 100142, DOI:10.1016/j.scp.2019.100142.
- H. Liu and J. Zhang, Research progress in toughening modification of poly(lactic acid), J. Polym. Sci., Part B: Polym. Phys., 2011, 49, 1051–1083, DOI:10.1002/polb.22283.
- A. Z. Naser, I. Deiab, F. M. Defersha and S. Yang, Expanding Poly(lactic acid) (PLA) and Polyhydroxyalkanoates (PHAs) Applications: A Review on Modifications and Effects, Polymers, 2021, 13, 4271, DOI:10.3390/polym13234271.
- A. Nazrin, S. M. Sapuan, M. Y. M. Zuhri, I. S. M. A. Tawakkal and R. A. Ilyas, Water barrier and mechanical properties of sugar palm crystalline nanocellulose reinforced thermoplastic sugar palm starch (TPS)/poly(lactic acid) (PLA) blend bionanocomposites, Nanotechnol. Rev., 2021, 10, 431–442, DOI:10.1515/ntrev-2021-0033.
- M. Ho, K. Lau, H. Wang and D. Hui, Improvement on the properties of polylactic acid (PLA) using bamboo charcoal particles, Composites, Part B, 2015, 81, 14–25, DOI:10.1016/j.compositesb.2015.05.048.
- E. M. B. Lima, A. Middea, R. Neumann, R. M. Da Silva Moreira Thiré, J. F. Pereira, S. C. De Freitas, M. S. Penteado, A. M. Lima, A. P. Da Silva Minguita, M. Da Costa Mattos, A. Da Silva Teixeira, I. C. S. Pereira, N. R. R. D. Santos, J. M. Marconcini, R. N. Oliveira and A. C. Corrêa, Biocomposites of PLA and Mango Seed Waste: Potential Material for Food Packaging and a Technological Alternative to Reduce Environmental Impact, Starch, 2021, 73(5–6) DOI:10.1002/star.202000118.
- P. A. V. De Freitas, A. Chiralt and A. Chiralt, Using rice straw fractions to develop reinforced, active PLA-starch bilayers for meat preservation, Food Chem., 2023, 405, 134990, DOI:10.1016/j.foodchem.2022.134990.
- G. Mármol, C. Gauss and R. Fangueiro, Potential of cellulose microfibers for PHA and PLA biopolymers reinforcement, Molecules, 2020, 25, 4653, DOI:10.3390/molecules25204653.
- N. L. Cristofoli, A. R. Lima, R. D. Tchonkouang, A. C. Quintino and M. C. Vieira, Advances in the Food Packaging Production from Agri-Food Waste and By-Products: Market Trends for a Sustainable Development, Sustainability, 2023, 15, 6153, DOI:10.3390/su15076153.
- Q. Jin, L. Yang, N. Poe and H. Huang, Integrated processing of plant-derived waste to produce value-added products based on the biorefinery concept, Trends Food Sci. Technol., 2018, 74, 119–131, DOI:10.1016/j.tifs.2018.02.014.
- C. Fontes-Candia, E. Erboz, A. MartÍnez-Abad, A. López-Rubio and M. Martínez-Sanz, Superabsorbent food packaging bioactive cellulose-based aerogels from Arundo donax waste biomass, Food Hydrocolloids, 2019, 96, 151–160, DOI:10.1016/j.foodhyd.2019.05.011.
- S. N. H. M. Azmin, N. A. B. M. Hayat and M. S. M. Nor, Development and characterization of food packaging bioplastic film from cocoa pod husk cellulose incorporated with sugarcane bagasse fibre, J. Bioresour. Bioprod., 2020, 5, 248–255, DOI:10.1016/j.jobab.2020.10.003.
- B. Ai, L. Zheng, W. Li, X. Zheng, Y. Yang, D. Xiao, J. Shi and Z. Sheng, Biodegradable cellulose film prepared from banana Pseudo-Stem using an ionic liquid for mango preservation, Front. Plant Sci., 2021, 12 DOI:10.3389/fpls.2021.625878.
- V. Srivastava, S. Singh and D. Das, Rice husk fiber-reinforced starch antimicrobial biocomposite film for active food packaging, J. Cleaner Prod., 2023, 421, 138525, DOI:10.1016/j.jclepro.2023.138525.
- V. T. W. Thuppahige, L. Moghaddam, Z. Welsh, T. Wang and A. Karim, Investigation of critical properties of Cassava (Manihot esculenta) peel and bagasse as starch-rich fibrous agro-industrial wastes for biodegradable food packaging, Food Chem., 2023, 422, 136200, DOI:10.1016/j.foodchem.2023.136200.
- G. Barzan, A. Sacco, A. M. Giovannozzi, C. Portesi, C. Schiavone, J. Salafranca, M. Wrona, C. Nerín and A. Rossi, Development of innovative antioxidant food packaging systems based on natural extracts from food industry waste and Moringa oleifera leaves, Food Chem., 2024, 432, 137088, DOI:10.1016/j.foodchem.2023.137088.
- A. Jayakumar, S. Radoor, G. H. Shin and J. T. Kim, Lemon peel-based fluorescent carbon quantum dots as a functional filler in polyvinyl alcohol-based films for active packaging applications, Ind. Crops Prod., 2024, 209, 117968, DOI:10.1016/j.indcrop.2023.117968.
- D. Divakaran, I. Suyambulingam, M. R. Sanjay, R. Vijay, V. Ayyappan and S. Siengchin, Isolation and characterization of microcrystalline cellulose from an agro-waste tamarind (Tamarindus indica) seeds and its suitability investigation for biofilm formulation, Int. J. Biol. Macromol., 2024, 254, 127687, DOI:10.1016/j.ijbiomac.2023.127687.
- J. Tarique, S. M. Sapuan, A. Khalina, R. A. Ilyas and E. S. Zainudin, Thermal, flammability, and antimicrobial properties of arrowroot (Maranta arundinacea) fiber reinforced arrowroot starch biopolymer composites for food packaging applications, Int. J. Biol. Macromol., 2022, 213, 1–10, DOI:10.1016/j.ijbiomac.2022.05.104.
- K. Bumrungnok, P. Threepopnatkul, T. Amornsakchai, C. H. Chia, R. Wongsagonsup and S. M. Smith, Toward a circular bioeconomy: Exploring pineapple stem starch film as protective coating for fruits and vegetables, Polymers, 2023, 15, 2493, DOI:10.3390/polym15112493.
- A. N. Adilah, G. H. Chong and Z. A. N. Hanani, Incorporation of graphene oxide to enhance fish gelatin as bio-packaging material, Food Packag. Shelf Life, 2021, 28, 100679, DOI:10.1016/j.fpsl.2021.100679.
- G. I. Florentino, D. A. S. Lima, M. M. F. Santos, V. C. Da Silva Ferreira, C. V. B. Grisi, M. S. Madruga and F. A. P. Da Silva, Characterization of a new food packaging material based on fish by-product proteins and passion fruit pectin, Food Packag. Shelf Life, 2022, 33, 100920, DOI:10.1016/j.fpsl.2022.100920.
- E. Athanasopoulou, A. Michailidi, D. Ladakis, K. I. Kalliampakou, E. Flemetakis, A. Koutinas and T. Tsironi, Extraction of Fish Protein Concentrates from Discards and Combined Application with Gelatin for the Development of Biodegradable Food Packaging, Sustainability, 2023, 15, 12062, DOI:10.3390/su151512062.
- H. Wu, D. Xiao, J. Lu, T. Li, C. Jiao, S. Li, P. Lü and Z. Zhang, Preparation and Properties of Biocomposite Films Based on Poly(vinyl alcohol) Incorporated with Eggshell Powder as a Biological Filler, J. Polym. Environ., 2020, 28, 2020–2028, DOI:10.1007/s10924-020-01747-2.
- G. S. Varadarajan and P. Nagaraaj, Enhancement of PVA packaging properties using calcined eggshell waste as filler and nanonutrient, Mater. Chem. Phys., 2022, 291, 126611, DOI:10.1016/j.matchemphys.2022.126611.
- G. M. De Oca-Vásquez, M. E. Alfaro, J. R. Vega-Baudrit, G. Jiménez-Villalta, V. H. R. Arellano and B. Sulbarán-Rangel, Development of nanocomposite chitosan films with antimicrobial activity from agave bagasse and shrimp shells, J. Agric. Food Res., 2023, 14, 100759, DOI:10.1016/j.jafr.2023.100759.
- A. N. M. Rasak, H. Heryanto and D. Tahir, High degradation bioplastics chitosan-based from scale waste of milkfish (Chanos chanos), Int. J. Biol. Macromol., 2024, 256, 128074, DOI:10.1016/j.ijbiomac.2023.128074.
- X. Li, Y. Wei, S. Jiang, Y. Zhou, J. Li, L. Kuang, S. Q. Shi and J. Li, Full Bio-Based soy protein isolate film enhanced by chicken feather keratin, Macromol. Mater. Eng., 2021, 306(5) DOI:10.1002/mame.202100004.
- O. M. Oluba, E. Osayame and A. J. Shoyombo, Production and characterization of keratin-starch bio-composite film from chicken feather waste and turmeric starch, Biocatal. Agric. Biotechnol., 2021, 33, 101996, DOI:10.1016/j.bcab.2021.101996.
- Z. Qian, J. Zhang, W.-R. Xu and Y. Zhang, Development of active packaging films based on liquefied shrimp shell chitin and polyvinyl alcohol containing β-cyclodextrin/cinnamaldehyde inclusion, Int. J. Biol. Macromol., 2022, 214, 67–76, DOI:10.1016/j.ijbiomac.2022.06.052.
- A. Sahoo, A. Dwivedi, P. Madheshiya, U. Kumar, R. Sharma and S. Tiwari, Insights into the management of food waste in developing countries: with special reference to India, Environ. Sci. Pollut. Res., 2023, 31, 17887–17913, DOI:10.1007/s11356-023-27901-6.
- T. D. Moshood, G. Nawanir, F. Mahmud, F. Mohamad, M. H. Ahmad and A. AbdulGhani, Sustainability of biodegradable plastics: New problem or solution to solve the global plastic pollution?, Curr. Res. Green Sustainable Chem., 2022, 5, 100273, DOI:10.1016/j.crgsc.2022.100273.
- E. Boccalon and G. Gorrasi, Functional bioplastics from food residual: Potentiality and safety issues, Compr. Rev. Food Sci. Food Saf., 2022, 21, 3177–3204, DOI:10.1111/1541-4337.12986.
- M. Asgher, S. A. Qamar, M. Bilal and H. M. N. Iqbal, Bio-based active food packaging materials: Sustainable alternative to conventional petrochemical-based packaging materials, Food Res. Int., 2020, 137, 109625, DOI:10.1016/j.foodres.2020.109625.
-
J. G. Brown, Biodegradable material, WO2021007579A1, 2021.
-
S. A. Santos, Packaging for foodstuff coating based on Sargassum, Chinese Pat., CN104321193B, 2016.
-
S. Zou, Y. Wu, Y. He, T. Zhang, J. Tang and Z. Zheng, The preparation method of functional solvable edible composite membrane, Chinese Pat., CN105646951B, 2018.
-
R. Narendra, K. Venkatesh, M. Santosh, S. Balaji and J. Kishore, Natural fiber or fibrous material based completely biodegradable food containers, WO2017163095A1, 2017.
-
H. Kuiper, Biodegradable and compostable food packaging unit from a moulded pulp material, and method for manufacturing such food packaging unit, AU2017340017B2, 2021.
-
H. X. Hou, S. Gao and W. T. Wang, Full-degradable antibacterial food packaging film and preparation method thereof, Chinese Pat., CN113234307B, 2022.
-
X. X. Li, L. Chen, J. Liu, P. Liu, L. Li and J. Y. Su, Starch/polylactic acid antibacterial activity packaging material as well as preparation method and application thereof, Chinese Pat., CN101935440B, 2012.
-
E. Bayer and G. Mclntyre, Method for Producing Grown Materials and Products Made Thereby, US Pat., US20170049059A1, 2020.
-
H. X. Hou, H. Z. Dong, Y. Yang and H. Zhang, Edible starch-based food packaging film and preparation method thereof, Chinese Pat., CN101899173A, 2012.
-
H. B. Zhan and W. P. Chen, Gelatin-chitosan composite food packaging film and preparation method thereof, Chinese Pat., CN101955670B, 2012.
-
Q. Deng and Z. J. Yan, Biodegradable food packaging material and preparation method thereof, Chinese Pat., CN109294193B, 2020.
-
J. Song, M. Wang, Y. X. Jia, Y. Zheng, J. H. Zhou, L. N. Tu, T. Xia, and J. M. Luo, A kind of degradable scleroglucan natural complex fresh-keeping film and the preparation method and application thereof, Chinese Pat., CN110183739A, 2021.
-
N. Mulyono, Biodegradable and edible bioplastic from renewable plant based polymer for packaging and the manufacturing method thereof, US Pat., US11708492B2, 2023.
-
S. H. Chen, Hydrophobic thermoplastic starch composite material and its manufacturing method, JP6955774B2, 2021.
-
X. H. Lin, Full-degradable high-barrier composite material for flexible package and preparation method and application thereof, Chinese Pat., CN110791069A, 2021.
-
Z. Ji, X. F. Jiang, H. M. Xu, F. S. Chen and Y. Meng Yao, Biodegradable high-barrier-property antibacterial composite membrane and preparation method thereof, Chinese Pat., CN113354853B, 2022.
-
H. G. Xiong, Z. Y. Xiong, P. K. Wang, L. Chen, S. Y. Ding and Y. Li, Composite nano material modified starch-based biodegradable food packaging film and preparation method thereof, Chinese Pat., CN110437590B, 2020.
-
Q. Deng and Z. J. Yan, Antibacterial PLA-based degradable food packaging material and preparation method thereof, Chinese Pat., CN109438937B, 2020.
-
Y. C. Zhang, Q. Liu, J. Y. Guo, Z. J. Qian, J. Cheng and S. Y. Li, Starch cross-linked tea polyphenol antibacterial degradable food packaging material and preparation method thereof, Chinese Pat., CN111171385B, 2021.
-
Y. Cho, S. Yoon, J. Min, S. Kim, M. Jin and S. Yoon, Moisture barrier biodegradable film, packaging sheet and sticker comprising the film, KR102372332B1, 2022.
-
G. Q. Mou, F. F. Zhang, F. Qian, G. X. Zhang, Y. Xia, X. J. Lin, H. M. Zhou, Q. Yang and G. Z. Li, Preparation method of completely degradable biomass food packaging film, Chinese Pat., CN111019170A, 2020.
-
H. M. Luo, C. Q. Wu and Y. J. Luo, Nanoscale plant fiber modified biodegradable composite material and preparation method and application thereof, Chinese Pat., CN112300542A, 2021.
-
X. P. Lan, Biodegradable preservative film with antibacterial function and preparation method thereof, Chinese Pat., CN113583422A, 2023.
-
S. Moon, K. Yoon, J. Park, J. Su and S. Sin, Biodegradable packaging, preparation method thereof, security pack and security method of mobile device, KR102404216B1, 2022.
-
H. Jo, H. Ryu, H. Lee and W. Moon, Biodegradable food packaging materials using algae and method for producing the same, KR20210103322A, 2021.
-
H. Y. Yu, F. Li, Y. Z. Li, Y. Guan and Y. Zhou, Preparation method of antioxidant and antibacterial biodegradable food packaging material, Chinese Pat., CN112063135B, 2022.
-
X. L. Qiu, X. F. Gong, S. J. W. Gao, K. H. Wang, X. C. Zhou, X. H. Li and Y. Wang, Transparent moisture-preserving antibacterial degradable packaging film and preparation method and application thereof, Chinese Pat., CN113698646B, 2022.
-
J. Y. Su, Y. Cai and L. M. Fang, Biodegradable UVA photoresponse type controlled-release antibacterial food packaging film and preparation method and application thereof, Chinese Pat., CN113061270B, 2021.
-
H. Jeong, H. Kim, L. Kwak, J. Kim, D. T. Tran, B. S. Soram and E. Kim, Chitosan membrane for food packaging, its manufacturing method and eco-friendly food packaging materials using the same, KR102422234B1, 2022.
-
Y. C. Zhang, J. Cheng and Q. Liu, Plant extract synthesized nano-silver multifunctional degradable food packaging material and preparation method thereof, Chinese Pat., CN113621153A, 2021.
-
H. M. Luo, C. Q. Wu, Y. J. Luo and L. Jiayu, Coffee grounds modified biodegradable composite material, and preparation method and application therefor, WO2021017030A1, 2021.
-
L. C. Zhao and Y. C. Zhao, A kind of antibacterial and biodegradable food package box and preparation method thereof, Chinese Pat., CN109265752A, 2019.
-
C. H. Zhi, D. Jiang and Q. W. Jiao, Bio-based environment-friendly packaging bag and preparation method thereof, Chinese Pat., CN110358264B, 2022.
-
C. Zhang, L. Pan, L. Han, K. Tu, J. Peng and G. J. Bu, Eugenol-containing curdlan-based antioxidant film and preparation method thereof, Chinese Pat., CN111875855A, 2021.
-
P. Fei, Hydrophobic food packaging film and preparation method thereof, Chinese Pat., CN110655688A, 2020.
-
Y. Y. Xie, L. Li, X. T. Qin and C. Zhong, Antibacterial and antioxidant gelatin-based composite membrane and preparation method thereof, Chinese Pat., CN115636961A, 2023.
-
150EUBIO_Admin, Market, https://www.european-bioplastics.org/market/, accessed December 31, 2023 Search PubMed.
- C. L. Reichert, E. Bugnicourt, M. Coltelli, P. Cinelli, A. Lazzeri, I. Canesi, F. Braca, B. M. Martínez, R. Alonso, L. Agostinis, S. Verstichel, L. Six, S. De Mets, E. C. Gómez, C. Ißbrücker, R. Geerinck, D. Nettleton, I. Campos, E. Sauter, P. Pieczyk and M. Schmid, Bio-Based packaging: materials, modifications, industrial applications and sustainability, Polymers, 2020, 12, 1558, DOI:10.3390/polym12071558.
- X. Zhao, K. Cornish and Y. Vodovotz, Narrowing the gap for bioplastic use in food packaging: An update, Environ. Sci. Technol., 2020, 54, 4712–4732, DOI:10.1021/acs.est.9b03755.
- M. E. González-López, S. De Jesús Calva-Estrada, M. S. Gradilla-Hernández and P. Barajas-Álvarez, Current trends in biopolymers for food packaging: a review, Front. Sustain. Food Syst., 2023, 7 DOI:10.3389/fsufs.2023.1225371.
- J. Jeevahan, M. Chandrasekaran, S. P. Venkatesan, V. Sriram, G. B. Joseph, G. Mageshwaran and R. B. Durairaj, Scaling up difficulties and commercial aspects of edible films for food packaging: A review, Trends Food Sci. Technol., 2020, 100, 210–222, DOI:10.1016/j.tifs.2020.04.014.
- L. D. V. Siqueira, C. I. La Fuente Arias, B. C. Maniglia and C. C. Tadini, Starch-based biodegradable plastics: methods of production, challenges and future perspectives, Curr. Opin. Food Sci., 2021, 38, 122–130, DOI:10.1016/j.cofs.2020.10.020.
- H. T. Duguma, P. Khule, A. McArdle, K. Fennell and E. Almenar, Turning agricultural waste into packages for food: A literature review from origin to end-of-life, Food Packag. Shelf Life, 2023, 40, 101166, DOI:10.1016/j.fpsl.2023.101166.
|
This journal is © The Royal Society of Chemistry 2024 |
Click here to see how this site uses Cookies. View our privacy policy here.