Plant phytochemicals as bio-responsive compounds in smart packaging for food spoilage detection: a comprehensive review
Received
3rd March 2024
, Accepted 2nd April 2024
First published on 2nd April 2024
Abstract
With growing consumer concern for eating fresh and nutritious food, there arises a demand for freshness indicators to build consumer trust and brand value. An active and intelligent packaging system helps extend the shelf life of a product and facilitates visual communication of the dynamic shelf life, respectively. These methods thereby may improve food safety and reduce food wastage at household and retail level by eliminating the confusing use-by and best-before labels printed on packaging. This review on smart packaging has more emphasis on freshness indicators, a segment of intelligent packaging. In the past few decades, the trend of using natural coloring compounds, flavonoids, as pH indicators has been booming. The complex process of food spoilage and its association with color change of flavonoids is elaborated. These compounds can be extracted efficiently from fruit and vegetable peels and flowers using several techniques. They can be employed as pH indicators after immobilizing them in a base matrix, usually biopolymers owing to their biodegradability.
 Konala Akhila | Konala Akhila received a BTech degree in chemical engineering (2017) from NIT Warangal, India and MTech degree in packaging technology (2020) from IIT Roorkee, India. She is pursuing her PhD under the supervision of Prof. Kirtiraj K. Gaikwad, at Indian Institute of Technology, Roorkee, India. She was awarded the Indo-German Science and Technology (IGSTC-Germany) Fellowship-2022. Her research is focused on smart packaging techniques to monitor dynamic shelf life for food safety. Her area of interest also includes biopolymers, encapsulation of bioactive compounds and active packaging. |
 Dakuri Ramakanth | Dakuri Ramakanth received his BTech degree in chemical engineering (2014) from J.N.T.U., India and MTech degree in packaging technology (2018) from IIT Roorkee, India. He is pursuing his PhD under the joint supervision of Prof. Kirtiraj K. Gaikwad and Prof. Pradip K. Maji, at Indian Institute of Technology, Roorkee, India. He received the most prestigious Commonwealth Split-site Fellowship (UK) and Indo-German Science and Technology (IGSTC-Germany) Fellowship-2022, and DAAD Scholarship-TU Dresden, Germany in 2017. His research is focused on sustainable packaging materials for food quality and alternative materials to single use plastics, active and intelligent packaging. |
 Lingala Lakshmana Rao | Lingala Lakshman Rao obtained a BTech in chemical engineering from Andhra University College of Engineering (Visakhapatnam, Andhra Pradesh, India) in 2022. Currently, he is enrolled in MTech in packaging technology in the Department of Paper Technology, Indian Institute of Technology Roorkee (Roorkee, Uttarakhand, India) under the supervision of Prof. Kirtiraj K. Gaikwad. He was awarded the Ansys Fellowship Award for his master research proposal in the area of sustainable food packaging. His studies concentrate on intelligent indicators for packaging applications, biodegradable and UV blocking packaging films, milk adulteration indicators and sustainable food packaging. |
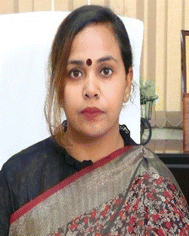 Suman Singh | Prof. Suman Singh earned her PhD from GB Pant University, Uttarakhand, India. She worked as a post-doctoral fellow in Yonsei University, Seoul, South Korea. She is currently working as Assistant Professor in Veer Chandra Singh Garhwali Uttarakhand University of Horticulture & Forestry. Her area of interests includes antimicrobial packaging, food processing and preservation techniques, and waste valorization. She was named as one of the most influential women of the year – 2024 in India by Printweek and awarded with packaging person of the year title. |
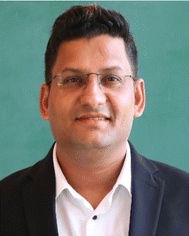 Kirtiraj K. Gaikwad | Prof. Kirtiraj K. Gaikwad earned his MS (packaging technology) from Michigan State University, USA, and his PhD (packaging technology) from Yonsei University, Seoul, South Korea. After his PhD, he worked as a postdoctoral fellow at Polytechnique Montréal, Canada. He is currently working as an Associate Professor in the Department of Paper Technology at the Indian Institute of Technology Roorkee, India. His research is aimed at active food packaging and waste valorization. He has published more than 100 peer-reviewed papers and 2 international and 4 Indian patents. He was listed in Stanford's list of top 2% scientists of the world – 2023. |
Sustainability spotlight
In the wake of global challenges posed by climate change and escalating concerns regarding environmental degradation, the pursuit of sustainable practices across all sectors is imperative. The forthcoming review paper titled “Plant phytochemicals as bio-responsive compounds in smart packaging for food spoilage detection: a comprehensive review” endeavors to shed light on an innovative approach towards enhancing food packaging sustainability while addressing the critical issue of food spoilage. Acknowledging the profound impact of traditional packaging materials on our ecosystem, this review paper explores the potential of integrating plant phytochemicals as bio-responsive compounds in smart packaging systems. By harnessing the inherent properties of these natural compounds, such as their antimicrobial and antioxidant characteristics, smart packaging holds promise in not only extending the shelf life of food products but also minimizing waste generation along the supply chain. The utilization of plant-derived compounds aligns seamlessly with the principles of sustainability, offering a renewable and biodegradable alternative to conventional packaging materials derived from fossil fuels. Moreover, by employing smart packaging technology embedded with phytochemical sensors, real-time monitoring of food freshness can be achieved, facilitating timely interventions to prevent spoilage and reduce food wastage. Furthermore, this comprehensive review aims to elucidate the multifaceted benefits of integrating plant phytochemicals into smart packaging systems, ranging from enhanced food safety and preservation to reduced environmental footprint. Through a meticulous examination of existing research findings and technological advancements, this paper endeavors to provide valuable insights into the feasibility, efficacy, and potential challenges associated with implementing such innovative solutions on a broader scale. In essence, the exploration of plant phytochemicals as bio-responsive compounds in smart packaging represents a significant stride towards fostering sustainability within the food industry. By embracing this paradigm shift towards eco-friendly packaging solutions, we can not only mitigate the adverse impacts of conventional packaging practices but also contribute towards building a more resilient and sustainable future for generations to come.
|
1. Introduction
From its rudimentary origins, packaging has served its purpose to provide protection and convenience to consumers in terms of storage and handling of any commodity. Industrialization and globalization have meant that packaging is used not just for functional purposes but also for creating brand image and differentiating products. In day-to-day life of a modern human being, comfort and convenience are prioritized to an extreme extent. One of the major sectors to which packaging contributes immensely is food and beverages. It is a known fact that anything consumed by humans needs to be protected from extrinsic and intrinsic factors to extend its shelf life without compromising on taste, appearance, and nutritional benefits. Packaging for food prevents contamination, delays spoilage, communicates about the product to the consumer and most significantly minimizes food loss and wastage.1
Despite the efforts made by packaging to reduce food loss and wastage, over 30% of produced food is lost or wasted at various stages of supply chain and distribution annually.2 Evolution of packaging in terms of functionality and performance is necessary to meet societal expectations. Some of the factors that contribute to the evolution of packaging are changes in consumer demand for mildly preserved safe and nutritious food, improved consumer lifestyle preferring convenience over cost, longer shelf life with same nutritional benefits, increasing ways of digital interactions and consumer awareness of environmental effects.3 Hence, packaging has undergone a major transformation with technological advancements and led to a new age of smart packaging. The term smart packaging is self-explanatory: suggesting that the packaging necessarily involves and interacts with a product's atmosphere to dynamically preserve the food by retaining its safety and quality until it reaches the end consumer.4
Smart packaging is such a technique that increases efficiency, ensures authenticity and traceability, and restricts fraud and theft. Smart packaging is broadly categorized into two systems based on the action it performs on the product or product atmosphere: active packaging and intelligent packaging.5 Active packaging is deeply associated with altering a product's atmosphere to slow down the deterioration process and extend its shelf life while intelligent packaging does not disturb the head space gases but only senses them to communicate the product's state.6 Whilst the concept of smart packaging sounds fascinating and innovative, it has been quite a challenge for full-scale adoption and application because of lack of extensive scientific knowledge on most of the techniques.7 Extensive research has been conducted in the last few years to advance this technology and put it into commercial use.
Further classification of smart packaging and types of techniques that come under these two systems are represented in Fig. 1. The importance of these packaging systems is very well perceived in the case of food packaging as food is vulnerable to the slightest changes in storage conditions leading to microbial growth, lipid and protein degradation. To date, active packaging techniques like the use of oxygen scavengers, moisture absorbers, carbon dioxide emitters and antimicrobials are the most popular and commercialized techniques not just for food packaging but also for electronics, household goods etc. Some of the well-known examples of active packaging systems are tabulated in Table 1.
 |
| Fig. 1 Broad categorization of smart packaging into active and intelligent packaging and further types of systems involved in smart packaging based on working principles. | |
Table 1 Commercial examples of active packaging techniques8
Active packaging technique |
Name of product |
Manufacturer |
Oxygen scavengers |
Ageless® |
Mitsubishi Gas Chemical |
ATCO® |
Laboratories STANDA |
Cryovac® |
OS1000, Sealed Air Co |
Shelflus™ |
CIBA |
Carbon dioxide emitters/absorbers |
CO2® fresh |
CO2 Technologies |
Freshlock |
Multisorb Technologies Inc. |
UltraZap® |
Paper Pak Industries |
Ethylene scavengers |
Crisper SL |
Ohe Chemicals |
Ethysorb® |
Molecular Products Limited |
Evert-fresh |
Evert Fresh Corp. Ltd |
Antimicrobials |
Agion® |
Agion Technologies |
Bactiblock® |
Nanobio Maters |
Ethicap™ |
Freund |
Antioxidant packaging |
ATOX® |
Artibal SA |
Odor emitters |
Aroma-Can® |
— |
2. Market for intelligent packaging
Unlike active packaging techniques, intelligent packaging techniques are complicated to understand and implement in the existing packaging line as they involve deeper knowledge of product and head space. Intelligent packaging brings in the concept of representing dynamic shelf life of a packed product by interacting with the product's head space. In simpler words, IP allows the customer to know whether the product was stored, handled, and transported in appropriate conditions.9 According to the European Food Safety Authority (EFSA), intelligent packaging is defined as materials and articles that monitor the condition of packaged food or the environment surrounding the food and can communicate the condition of the product by not interacting with the product directly.10
A recent report on growth of the smart packaging market revealed that in 2021 it had a market potential of USD19.33 billion and is expected to grow at a CAGR of 6.2% to reach USD29.45 billion by 2028. With Asia Pacific being the fastest growing region, IP is expected to lead the smart packaging market especially in North America and Europe. Although these smart packaging techniques were first commercialized in Japan, in the present-day scenario the United States contributes a whopping USD3.6 billion to the smart packaging market followed by Japan ($2.36B), Australia, the United Kingdom, and Germany. Some of the leading companies that are huge players in this sector include Sealed Air® Corporation, Amcor Plc (Australia), Multisorb Technologies (USA), Ball Corporation (USA), Sysco Corporation (USA), Timestrip PLC (UK), Paksense Incorporated (USA), and M&G USA Corporation. Some more examples of commercialized intelligent packaging systems are summarized in Table 2.
Table 2 Examples of commercial intelligent packaging systems along with their manufacturers
Intelligent packaging system |
Trade name |
Manufacturer |
Freshness indicators |
SensorQ® |
DSM NV FQSI Inc. |
RipeSense |
Ripesense Ltd |
Toxin Guard |
Toxin Alert Inc. |
Fresh Tag® |
COX Technologies |
Raflatac |
VTT and UPM Raflatac |
Food Sentinel System |
SIRA Technologies |
Time–temperature indicators |
VITSAB® |
VITSAB International AB |
OnVu™/Coolvu™ |
Freshpoint Inc./BASF |
Best-by® |
FreshPoint Lab |
TopCryo® |
Cryolog |
Traceo® & eO® |
|
Keep-it® |
Keep-it Technologies |
Novas® |
Insignia Technologies Ltd |
CheckPoint® |
VITSAB |
3M™ Monitor Mark® |
3M Company |
FreshCode® |
Varcode Ltd |
Timestrip® |
Timestrip Plc |
PLUS |
Timestrip Plc |
Tempix® |
Temptix AB |
TempDot® |
Delta Trak |
Thermax® |
Thermografic Measurements Ltd |
WarmMark® |
Delta Track |
SmartDot |
EVIGENCE SENSORS |
Insignia Deli Intelligent Labels™ |
Insignia Technologies |
Fresh-Check® |
Temptime Corp |
RFID Tags |
Intelligo |
Schiassi Diamo Scaltole Alle Tue Idee |
easy2log® |
CAEN RFID |
CS8304 |
Convergence Systems Ltd |
Intelligent fish box |
Craemer Group GmbH |
Intelligent box |
Mondi Plc |
TempTRIP |
TempTRIP LLC |
Sensors |
OxyDot-O2xyDot |
OxySense |
Food Sentinel System |
SIRA Technologies Inc. |
Integrity indicators |
Ageless Eye® |
Mitsubishi Gas Chemical Co. |
Tufflex GS |
Sealed Air Ltd |
Shelf Life Gaurd |
UPM |
Tell-Tab |
IMPAK |
3. Association of food spoilage with intelligent packaging
Food safety is a pivotal issue in the present day. Food losses and food wastage contribute significantly towards food safety. Therefore, minimizing loss and wastage are the need of the hour. But how do we comprehend that food is spoiled way before it develops a visible mold growth or discoloration? Or how do we know that despite looking withered, food is still nutritious? Spoilage is characterized by any unacceptable change in food's sensory properties.11 The process of food spoilage technically involves various factors like composition of food, its water activity, its sensitivity to temperature and sunlight,12,13 but microbial growth is the top contributor of health concerns.14 Combinations of any trigger points of spoilage lead to growth of microbes and they release chemical substances into head space called analytes which can be toxins, mycotoxins, chemical residues, and gases. Common gases released during microbial spoilage are CO2 and volatile organic compounds like alcohols, esters, acids, and biogenic amines.15,16Fig. 2 presents the factors affecting food spoilage.
 |
| Fig. 2 Critical factors affecting food spoilage during storage and transportation. | |
Up until recent times, laboratory techniques like microbial counting, chemical methods like TVBN and lipid oxidation tests, sensory tests, and GC-MS tests were performed to find the freshness. But these techniques are confined to the laboratory level, time-consuming and not accessible to the general population.17 Hence the requirement of rapid testing systems that are cheap, reliable, non-destructive, portable, and available to every consumer has risen. Any intelligent sensor or indicator can detect and track emitted gases by reacting with them. Since all the analytes released during food spoilage tend to change the pH of the product, freshness colorimetric indicators that detect released gases and thus pH of the food are popular intelligent packaging systems.
3.1. Emphasis on freshness indicators
As already discussed, consumers nowadays focus on quality information of food. They are conscious about the safety of food. Fresh and minimally processed food is catchy for branding of any particular food product on the market. Therefore, freshness indicators (FI) are an attractive option to integrate into packaging. FI as the name suggests will represent the freshness of a product and scientifically prove it to the consumer. Freshness indictors are simple and rapid that confirm the presence or absence of target analyte with minimum or no quantitative data.6
Addition of color to this indication will make this reading visually accessible and hence they are also called colorimetric indicators. This representation can be done by monitoring head space gases, microbial metabolites, or storage conditions. Based on detection principle, time–temperature indicators, pH indicators, and gas/integrity indicators are considered as FI.18
3.2. Time–temperature indicators
TTIs are a useful tool to record freshness and quality of a food product. They indicate quality changes and remaining shelf life of a product by monitoring the collaborative effect of exposed temperature with time.19 These come under the category of external indicators as they monitor the external temperature of packaging and are applied on the outer side of the package. They contribute to the direct improvement of qualitative analysis of food and so far are the most popular indicating systems.20 From activation to termination, the duration of time taken will indirectly give the remaining shelf life usually by changing the color of the TTI. Mechanisms for activation of TTIs are mainly based on enzymatic, microbial, electrochemical, and chemical reactions. Accurate prediction of spoilage time is achieved by mathematical and kinetic modelling of one or more parameters of food. TTIs are engineered in a way to respond precisely to these mathematical parameters and reflect parallelly which can then be translated into qualitative deterioration of food.21
3.3. Gas indicators
Gas profiling of a package's head space plays an important role in keeping food fresh. Also, timely monitoring of these gases is necessary to ensure the safety of food. During the process of aging or spoiling, certain gases are released into the head space. Permeation of gases through the packaging can also change the concentrations leading to unwanted food spoilage. Pinholes in the package, improper sealing, improper barrier properties, counterfeiting, and mishandling will also lead to gas accumulation in the headspace.22 Among various gases, oxygen, carbon dioxide, and biogenic amines are the common culprits to disturb the integrity of packaging. Hence indicators that monitor these gases in a product's head space are also called integrity indicators. Increase in concentration of amines or CO2 indicates the presence of microbial metabolites. Increased percentage of oxygen in headspace leads to lipid oxidation, accelerating respiration and growth of aerobic microbes which indirectly changes color, flavor and odor of food. These indicators are in-packet indicators that are colorimetric in nature.23
Gas indicators are developed in the form of labels, printable inks, tablets, and films. Most of the oxygen indicators use redox chemical compounds as reactants to undergo instantaneous oxidation and change color. Dyes like anthraquinones, methylene blue and 2,6-dichloroindophenol and reducing agents like sugars and alkaline compounds are the typical constituents of oxygen indicators. CO2 indicator strips were developed with lysine, ε-polylysine and natural colorants. Remaining gases like H2S are analyzed typically using electrochemical sensors.24 Usage of integrity/gas indicators are dominantly found in vacuum packaging and modified atmospheric packaging. These indicators ensure that a product is protected from even negligible concentrations of undesired gas during its shelf life.25 Of concern for some oxygen indicators is the use of harmful chemicals that can migrate to food very easily. A lot of interest was piqued in replacing these chemical dyes with natural colorants that are sensitive to oxygen.
3.4. pH indicators
Changes or fluctuations in pH of a food product are an indirect quality determination factor. Growth of microbes, release of undesired metabolites, organic acids and gases result in irreversible changes in color, flavor, odor, and pH of food.26 Regardless of the reason for spoilage, pH is a common factor that fluctuates with the freshness of food and can be easily identified without quantification. Monitoring the gases, metabolites and organic acids in package head space will give the pH of the product thus helping to understand the freshness of the product. Statistical data collected from Web of Science on total publications to date on pH indicators and gas indicators are presented in Fig. 3. A pH indicator generates a trustworthy color response to quality changes and is cost effective. It is undemandingly simple to comprehend the quality just by looking at it with the naked eye, making such indicators a fascinating technology to develop.
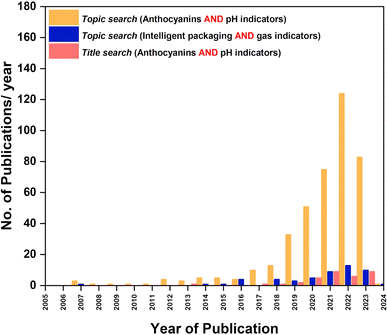 |
| Fig. 3 Statistical data on total papers published since 2005 on using anthocyanins as pH indicators (topic search and title search) and total publications on gas indicators applied as intelligent packaging systems. Data were collected from “Web of Science” database on 7th November 2023. | |
4. Phytochemicals as pH-sensitive pigments
All the commercially developed pH-sensitive freshness indicators incorporate chemical or synthetic pH-sensitive dyes. Some commonly known and widely used synthetic pH-sensitive dyes are bromophenol blue, bromocresol green and purple, chlorophenol red, bromothymol blue, phenolphthalein, and xylenol.27 Unfortunately, the toxicity of these synthetic dyes has overshadowed their competent pH sensitivity. Short exposure to these dyes can raise minor issues like allergies, irritability, and hyperactivity. Prolonged exposure may lead to severe health concerns.28 By means of exposure, they migrate into the product and can be ingested by consumers. Strict regulations were set by several countries on the usage of these colorants in food packaging. These circumstances have led to the exploration of non-toxic pH-sensitive dyes. Color is an integral part of our vast nature. These colors are sourced from plants, minerals, microbes, and animals. Among them, plants and their parts are abundant sources of colors. From leaves to roots, flowers to fruits, vegetables to peels have coloring compounds in them. All the colorants that are found in plants come under the category of phytochemicals which contribute to the color, antioxidant, anti-inflammatory, anti-diabetic, and anti-cancerous properties of plants. Simply, phytochemicals are biologically active natural chemical compounds that protect plants from external adverse factors. To date, exact classification of phytochemicals has not been done owing to their diverse structures.
The known categorization and subcategorization of phytochemicals is represented in Fig. 4. Among them, phenolics are abundantly available in plants (∼45%). Classified as coloring compounds in phytochemicals are anthocyanins (flavonoid derivative), carotenoids (isoprenoid derivative/terpenoids), chlorophyll II (pyrrole derivative), and betalains (nitrogen heterocyclic derivative/alkaloids).29 All these coloring compounds are capable of imparting color on addition and are pH sensitive because of their chemical structure. Materials from the group of phenolic acids and flavonoids, especially anthocyanins, are studied as pH-sensitive compounds. Their potential in indicating the freshness of a food product was determined.
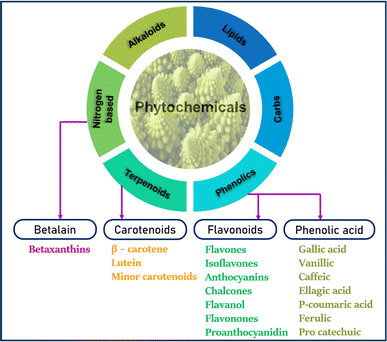 |
| Fig. 4 Known classification of phytochemicals in plants and their subclassification of coloring and bioactive compounds.30–32 | |
4.1. Phenolic acids
Phenolic acids are simple phenols with carboxylic acid groups with two structures, hydroxycinnamic and hydroxybenzoic structures. A list of phenolic acids is presented in Fig. 4 and their structures are shown in Fig. 5. Plant phenolics share several similarities with alcohols possessing aliphatic structures, but the inclusion of an aromatic ring and the presence of a hydrogen atom in the phenolic hydroxyl group render them relatively weak acids. These compounds are recognized for their diverse range of functions, which encompass roles in plant growth, development, and defense, while also yielding positive effects for humanity.33 Phenolic compounds are known for their widespread biological applications like therapeutics, cosmetics, and food add-ons. Phenolic acids are the major antioxidant supplements in human diet.34 Many of them absorb light in the UV region and provide protection from solar radiation. Some of them are soluble in water, some in organic solvents but large molecules like lignin are insoluble.35 Commonly found and explored benzoic acids are gallic acid, salicylic acid, and p-hydroxybenzoic acid, and cinnamic acids are ferulic acid, caffeic acid and p-coumaric acid. All these phenolic acids are identified in root exudates, leaf leachates and residues of decomposing plant in either bound or free form.36 Phenolic acids are most known and used for their antioxidant properties, free radical scavenging, breaking radical chains and chelating metals.37 The property of scavenging free radicals was taken advantage of in food packaging applications and they were employed as natural non-toxic oxygen scavengers to replace metal scavengers.38–45 However, studies on their pH sensitivity and their application in indicating systems are barely reported, leaving it a potential option to explore.
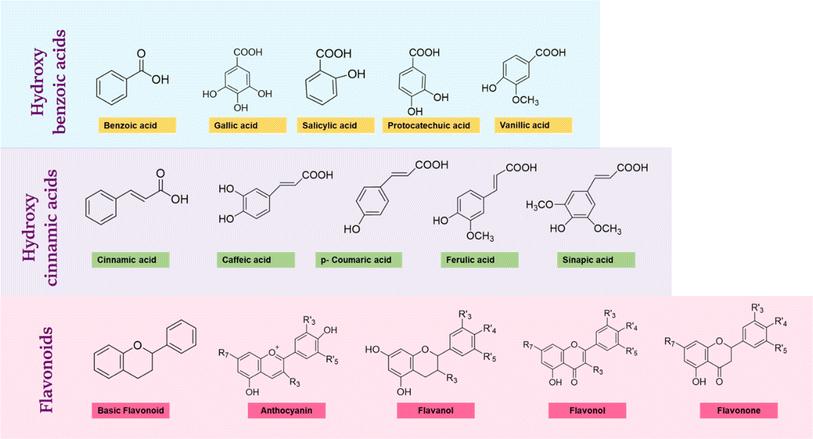 |
| Fig. 5 Chemical structural representation of the common phenolic acids (hydroxybenzoic acids, hydroxycinnamic acids) and a few flavonoids. | |
4.2. Flavonoids
Flavonoids are the secondary metabolites of a plant that participate in biological and non-biological responses of plants like developing color and protecting from bacteria or fungi and UV radiation. They also participate in plant growth, energy transfer, photosynthesis, and morphogenesis. The skeletal structure of flavonoids includes a minimum of 2 or 3 aromatic rings bearing one or more hydroxyl groups. The chemical variations of these flavonoids are characterized using degree of methoxylation or other substances in A, B, and C rings. Flavonoids contain a characteristic C6–C3–C6 structure, with free hydroxyl groups attached to aromatic rings as represented in Fig. 6. There are nearly 9000 flavonoid compounds identified so far.46 Some of the important pharmacological uses of flavonoids include antioxidant, antimutagenic, anti-inflammatory, chemoprotective and photoresponsive.47
 |
| Fig. 6 Six different types of anthocyanins found commonly in nature. Depending on R3, the color and absorbing wavelength of the anthocyanin change. | |
Anthocyanins, a subclass of pigmented flavonoids, are the largest group of water-soluble pigments of the plant kingdom that impart color to flowers, fruits, and roots ranging from orange-red to purple-blue hues.48 Anthocyanins can be both electrophilic and nucleophilic depending on the reacting medium. The skeletal structure of anthocyanins comprises di- or tri-hydroxyl groups attached to B-ring structure of a flavylium cationic flavonoid. The complex conjugated double bond structure of anthocyanins allows them to absorb light in the visible region, particularly between 520 and 570 nm which is far from the range of other polyphenolic compounds.49 A wide range of colors are displayed by anthocyanins depending on the degree of hydroxylation and substituted groups in their structure, usually red, blue, and purple.50 There are six different types of anthocyanins that are predominantly found in nature: delphinidin, petunidin, malvidin, cyanidin, pelargonidin, and peonidin, which have the same basic skeletal structure but differ in functional groups attached.51 These compounds are non-toxic and considered to have health benefits as they exhibit excellent physiological, anti-inflammatory, and antioxidant properties making them a potential part of human diet.52 These compounds are water-soluble and, unlike chlorophyll, they can be extracted effortlessly.53 With wide variations in color, anthocyanins are used as natural colorants for the food and cosmetics industries as well. Anthocyanins are highly sensitive to pH changes. Color change occurs due to the electron delocalization of anthocyanins when exposed to a high concentration of hydroxyl, i.e., at a high pH. These compounds' sensitivity to pH was turned into a useful mechanism that can react with acid or basic vapors released from spoiling food thus changing their structure and hence indirectly determining the pH of food. Structural changes of anthocyanins at different pH are schematically presented in Fig. 7 (de Freitas & Mateus, 2006; Houghton et al., 2021).54,55 A major setback faced by these compounds in applying them as pH indicators is their inherent instability to heat, oxygen and light.56
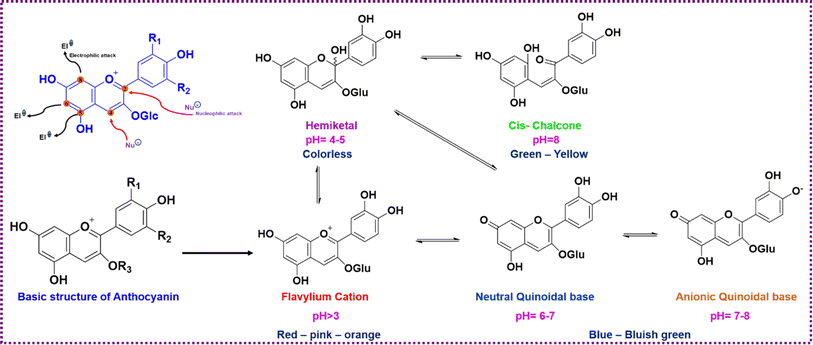 |
| Fig. 7 Structural changes of anthocyanin-3-glucoside when subjected to electrophilic and nucleophilic attack and exhibited color with respect to pH. | |
4.3. Extraction methods for phytochemicals
In the literature, there are several methods proposed and developed for the extraction of plant phytochemicals. Most phytochemicals are extractable and exist in the outer layer of cell walls which is easy to break with simple solvents, temperature, and mechanical damage. Polyphenols are generally more hydrophilic than lipophilic owing to their phenolic nature. Therefore, free polyphenols can be facilely extracted by simple solvents such as water, methanol, ethanol, acetonitrile and acetone, or by their mixtures. Extracts with higher amounts of coloring compounds like anthocyanins can be obtained by using acidified organic solvents.57 Some key parameters that affect the yield of extraction are extraction time and temperature, solvent to source ratio, choice of solvent, number of repetitions, pretreatments, and targeted polyphenol. The main purpose of optimizing these parameters is to minimize degradation of polyphenols and increase yield.58 Selection of solvents and their ratios can significantly affect the extraction yield depending on the polarity of the solvent. Polar solvents like water, ethanol, methanol, and acetic acid are used for extraction of polar compounds like flavonoids and non-polar solvents like hexane, benzene, toluene etc. for non-polar compounds like terpenoids.59 Soxhlet extraction, maceration and percolation are the conventional extraction techniques that have been used for more than a century to isolate polyphenols. New techniques like supercritical fluid extraction, microwave-assisted extraction, ultrasound-assisted extraction, pressurized liquid extraction, and deep eutectic solvent extraction have been studied for the last few decades.60 When a suitable solvent is selected, higher extraction yield depends on the increased mass transfer rate, larger diffusivity coefficient, smaller particle size, increased temperature, decreased viscosity of solvent, and larger surface area.61 Some advantages and disadvantages of all these methods are summarized in Table 3.
Table 3 Advantages and disadvantages of techniques employed in extraction of polyphenols from plants
Techniquea |
Advantages |
Drawbacks |
DESE: deep eutectic solvent extraction; SCE: supercritical fluid extraction; UAE: ultrasonication-assisted extraction; MAE: microwave-assisted extraction; PLE: pressurized liquid extraction.
|
Maceration and SE |
• Can use any type of solvent for extraction |
• Prolonged extraction time |
• Minimal damage to chemical compounds |
• Destruction of thermolabile compounds |
• Both batch and continuous process |
• Might cause oxidative stress on phenols |
DESE |
• Eutectic mixture of two or more solvents to give low-volatility, high-solubility and low-cost materials |
• High viscosity compared to organic solvents |
• Non-flammable and non-volatile extraction process |
• Can also dissolve lipophilic substances |
• Efficient when combined with UAE, MAE |
• Reacts with target compound using hydrogen bonding altering its properties |
SCFE |
• Minimal usage of organic solvents |
• Lower diffusion rate |
• Reduced extraction time |
• Requires higher pressure of SC fluids |
• Maximum degree of separation |
• CO2 cannot always be the solvent |
• Continuous process |
• Inconsistency in reproducibility |
• Easy recovery of solvent |
|
• Cost-effective handling |
|
UAE |
• Easy to use |
• High-frequency waves can degrade polyphenols |
• Rapid extraction rate |
• Rapid increase in temperature can cause volatile solvent losses |
• Least time-consuming |
|
• Economically viable |
|
• Lowered wastes |
|
• Increased mass transfer due to deeper penetration of sound waves |
|
MAE |
• Reduced time of extraction |
• Expensive setup compared with UAE |
• Lower solvent consumption |
• Usage of organic solvents like methanol |
• High-quality yield with high rate |
• Extraction efficiency is lower in the case of non-polar solute and higher viscosity solvent |
• Decreased by-product pollution |
• Unsuitable for thermally liable compound extraction |
• Accelerated extraction due to impact of microwave radiation on cell walls |
|
PLE |
• Faster extraction |
• Incomplete extraction in static mode |
• Enhanced penetration of solvent into deeper cell wall matrix |
• Lower recovery of polyphenols at higher temperatures |
• Increased solute diffusion due to decreased viscosity |
• Need sophisticated and automated equipment (expensive) |
Although new advanced technologies were proven to involve minimal extraction time, the damage that can occur to phytochemicals due to strong radiation, sound waves, pressure and temperatures is inevitable in the case of poor optimization. These techniques do not necessarily involve organic solvents for extraction but are relatively expensive in terms of energy consumption, equipment costs and handling. Whilst conventional methods involve longer time for extraction, the extract will have highest yield with undamaged phytochemicals and they are also economically reasonable. Hence, maceration is used in this contribution for extracting flavonoids. Also, in order to reduce the usage of organic solvent, water was preferred as solvent and also a mixture of ethanol and water was also studied.
4.4. Colorimetric pH indicators using phytochemicals for smart food packaging
Consequently, freshness indicators based on pH-sensitive dyes are a practical solution. Embedding these pH-sensitive dyes into a solid support matrix and applying as either a label or a film is widely adopted in current researches.62 Innovative progress has been made by researchers in the last two decades in developing pH indicators using anthocyanins (flavonoids). Some of the most recent publications based on anthocyanins as freshness indicators (pH and gas indicators) are discussed in the following text. Common sources of anthocyanins were purple sweet potato, black rice, blackberries, mulberries, black plum, red cabbage, purple tomato, purple corn, black carrot, litchi shells63etc. When applied to monitor the freshness of poultry meat, red meat, and seafood, the color changes observed for these anthocyanins are of diverse hues and relate to the slightest change in food pH.
Black rice extract was employed to develop a pH indicator by using a pectin and chitosan copolymerized matrix that changed its color from red to blue to yellow from acidic to basic medium.64 Amylose sourced from barley was used to trap red cabbage anthocyanins and studied for its light stability. The color changed from red to purple to dark green within a minute of exposure to pH conditions.65 The same anthocyanins were added to sodium alginate hydrogel matrix and a cryogel was developed to act as promising pH indicator for chicken fillet freshness monitoring.66 Black soyabean seed coat anthocyanins were extracted and incorporated into sodium alginate film matrix and applied as a pH indicator to monitor meat freshness.67 Another efficient source, plum peel extract, was added to sodium alginate/gelatin composite and studied as a pH indicator label for chicken freshness.68 A combination of two different flavonoids, anthocyanins from Hibiscus sabderiffa L. and curcumin, were loaded into a gelatin matrix. The label changed its color from reddish brown to dark brown indicating the spoilage of shrimp.69 A bilayer film was fabricated with several biopolymers by Huang et al.123 The innermost layer was added with mulberry anthocyanins for freshness detection of shrimps and remaining layers give an additional protection to shrimps and to anthocyanins from oxygen. This film changed its color from pink to yellow in response to pH and volatile gases in the head space.70 Betacyanins also proved to be excellent pH indicators in combination with anthocyanins for pork freshness monitoring. This combination was added to polyvinyl alcohol and sodium carboxy methyl cellulose matrix that changed its color form pink to green when the pH of pork increased to 6.05 from 5.99 within 8 hours.71 Red cabbage anthocyanins were also employed as time–temperature freshness indicators in a cellulose matrix.72 A fluorescent pH indicator was developed using Lycium ruthencium anthocyanins and carbon dots embedded in a cellulose nanofiber/polyvinyl alcohol matrix which changed its color from pink to blue to green to yellow when applied to monitor the freshness of shrimps.73
Incorporating anthocyanins into a film matrix is not the only option for employing them as pH indicators. Different forms of pH indicating systems have been reported in the literature like gels, membranes, and nanofiber mats. A gelatin matrix was also used to fabricate a nanofibrous membrane incorporated with red radish anthocyanin extract that changed its color from pink to yellow with the spoilage of meat. A bacterial cellulose nanofibrous mat entrapped with saffron anthocyanins and curcumin was fabricated to detect the freshness of fish stored at 4 °C for 4 days. The nanofiber mat changed its color when pH increased from 6.38 to 7.39.74 A comparative study between nanofiber mats and solvent-cast films made out of PVA and κ-carrageenan loaded with corn flower anthocyanins was performed to monitor shrimp freshness. The fibrous mat changed its color from pink to white and mint green through shrimp spoilage whereas the film's color change was from heather violet to brown to olive yellow.75 Red grape anthocyanin extract was added to a cellulose/salep copolymer and an irreversible pH-sensitive aerogel was developed to monitor the freshness of meat for 6 days. The color change was very prominent from purple to blue-gray to dark brown through the spoilage period.76 Chitin nanofibers and gelatin composite with barberry and saffron petal anthocyanins was developed for fish freshness monitoring.77 All the literature discussed so far has sourced the anthocyanins from edible sources or parts of edible fruits, vegetables, or grains. Less focus has been given to flower anthocyanins despite their outstanding coloring properties. A few research publications where flowers were used to extract anthocyanins and studied for pH indicating systems are tabulated in Table 4.
Table 4 Some recently published articles on anthocyanins as pH indicating systems embedded in biopolymer matrices and used in various food packaging applications
Source of anthocyanins |
Total anthocyanin content |
Polymer matrix |
pH range |
Change in color with pH medium |
Food application |
Ref. |
Grape skins |
11.38 ± 1.78 mg Cy3gl |
— |
3.2 to 3.5 |
Pink through red and violet to dark blue |
— |
92
|
Purple sweet potato |
— |
Potato starch |
2 to 10 |
Red, pink, purple, and blue |
Pork spoilage |
93
|
Black carrot |
10.5 mg Cy3gl/100 mL |
Cellulose and chitosan |
2 to 11 |
Pink to khaki |
Milk spoilage |
94
|
Red cabbage and rose |
— |
Agarose and filter paper |
4 to 8 |
Red, purple and green |
Fish spoilage |
95
|
Red barberry |
0.7 mg mL−1 Cy3gl |
Carbohydrate based |
1 to 14 |
Reddish to yellowish |
Fish fillet freshness |
96
|
Purple sweet potato |
— |
Cellulose nanofiber |
2 to 12 |
Pink to yellow |
Food application |
97
|
Blueberry peel |
— |
Corn starch |
2 to 10 |
Pink, red, purple and green |
Tilapia fillet freshness |
98
|
Purple tomato |
— |
Chitosan |
3 to 11 |
Purple, green and yellow |
Milk and fish |
99
|
Black chokeberry |
631 ± 13 mg L−1 Cy3gl |
Chitosan |
1 to 10 |
Pink, brownish, green |
Natural pH indicator |
100
|
Black bean seed coat |
1.12 ± 0.05 μg g−1 Cy3gl |
Corn starch |
1 to 10 |
Pink to grey green |
Shrimp spoilage |
101
|
Eggplant |
1.5 g/100 g Cy3gl |
Chitosan and starch |
2 to 10 |
Pink, green and violet |
— |
102
|
Hibiscus sabdariffa L. |
— |
Chitosan |
2 to 12 |
Reddish to pink to olive to yellow |
Fish spoilage indication |
103
|
Cornflower (Centaurea arvensis) |
3.35 ± 0.06 mg Cy3G/100 mL CAE |
PVA/κ-carrageenan |
2 to 12 |
Pink, red, brown, green, yellow |
Shrimp spoilage indication |
104
|
Butterfly pea flower |
40.99 mg Cy3G/L |
N-Glutaryl chitosan |
1 to 13 |
Pink, blue, yellow, green, yellow |
Chicken breast freshness indicator |
105
|
Red poppy (Papaver rhoeas L.) |
— |
Chitosan |
2 to 12 |
Brown/light yellow to dark brown |
Shrimp freshness |
106
|
Roselle (Hibiscus sabdariffa L.) |
225.01 ± 3.99 mg L−1 |
Sodium carboxymethyl cellulose/starch/nisin |
2 to 13 |
Dark pink to yellow-green |
Chicken breast |
107
|
Ipomoea coccinea
|
|
Polyvinyl alcohol and guar gum |
2 to 13 |
Red to yellow |
Chicken freshness |
108
|
Coleus scutellarioides
|
39.27 ± 5.78 mg L−1 |
Fish gelatin |
4 to 9 |
Pink to colorless |
Freshness monitoring of rainbow fish |
109
|
Saffron (Crocus sativus L.) |
1600 mg mL−1 Cy3G g−1 |
Bacterial cellulose nano paper |
5 to 11 |
Pinkish purple, blue, brown and green |
Fish spoilage |
110
|
Saffron (Crocus sativus L.) |
103.4 mg/100 g Cy3G g−1 |
Salep mucilage edible film |
3 to 11 |
Red to yellow |
Fish freshness |
111
|
Orychophragmus violaceus
|
— |
Liquid spray |
7 to 11 |
Pink, cyan, green, and yellow |
Carbonation detection indicator in concrete |
112
|
Butterfly pea flower (Clitoria ternatea L.) |
136.37 ± 3.86 mg Cy3G g−1 |
Sodium alginate |
1 to 12 |
Reddish purple to yellow green |
Antimicrobial and freshness indicator for milk, pork, and shrimp |
113
|
Butterfly pea flower (Clitoria ternatea L.) |
16.99 mg Cy3G eq. L−1 |
— |
2 to 12 |
Reddish-orange, red, purple, blue, green, and brownish yellow |
Natural pH indicator |
114
|
Butterfly pea flower (Clitoria ternatea L.) |
20.66 mg Cy3G eq. L−1 |
Starch/carbon nano dots |
1 to 12 |
Red, purple, blue, green, and yellow |
Monitoring pork meat spoilage |
115
|
Carissa carandas
|
0.612 mg mL−1 |
Chitosan and polyvinyl alcohol |
1 to 13 |
Red, colorless, and yellow |
Chicken meat storage |
116
|
Anthocyanins can also be applied as pH indicators after encapsulating them in several biopolymeric materials. The process of encapsulation is theorized to increase the thermal and oxidative stability of anthocyanins during storage and transportation. But encapsulated anthocyanins have seldom been applied as pH indicators in food systems in the literature.78 Most of the studies were on analyzing the storage stability and on in vitro gastric release kinetics.79–81 Alginate or alginate in combination with other polysaccharides, reinforced with nanoparticles were the abundantly found shell materials for encapsulation in published research.81–84 Emulsification techniques were also used for micro- and nano-encapsulation of anthocyanins but were not really developed for freshness indicating purposes.85–88 Encapsulation was preferably done with biopolymers to serve the purpose of disintegration for in vitro studies. These kinds of soft-shell materials cannot be used in real-time food systems as pH indicators, as they do not possess any mechanical strength and can easily be destroyed during packing. Also, hydrophilic polymeric beads cannot be applied in high-water-activity food as moisture may disintegrate them and lead to leakage of anthocyanins into food systems without serving their purpose. Hence there is a need to explore potential biopolymers that can encapsulate anthocyanins and retain their structure in any condition, also retaining the pH sensitivity of the anthocyanins.
4.5. Biopolymers in pH-responsive indicators
Any freshness indicator comprises a supporting matrix and a pH-sensitive dye. Along with improvements, packaging has also been criticized based on environmental concerns. So, using conventional polymers to make pH-sensitive labels is not recommended at this point of time. Also, when trying to replace synthetic pigments with natural colors, it is to be noted that phytochemicals are thermolabile and hence cannot withstand the operating temperatures of conventional plastics. Another important factor that demands the usage of biopolymers to entrap natural pigments is that the majority of these natural compounds are water soluble. New ways to employ a combination of natural pigments and biodegradable polymers represent potential research opportunities. A typical chart of classification with regards to biopolymers is shown in Fig. 8. Any polymer that is derived from living matter is considered as a biopolymer. Biosynthesized polymers are those that are produced by microbial activity and biobased polymers have monomers that originate from bio-sources and are chemically synthesized at some stage. Biomass-derived polymers are obtained from the concentrate of biomass like plants, animal, and their waste.89
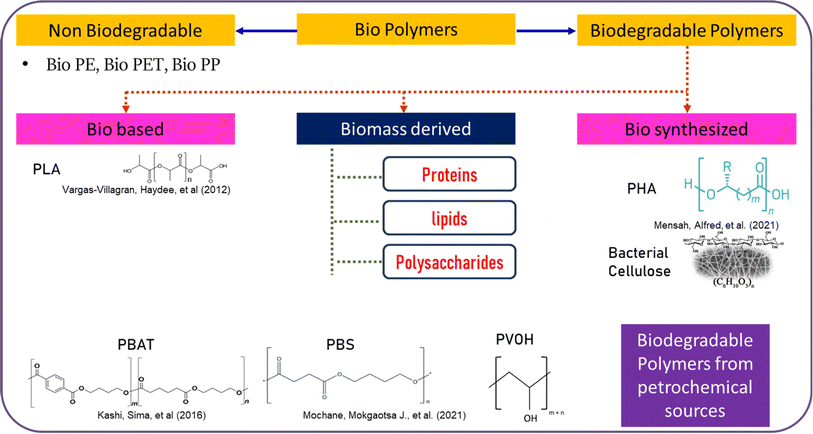 |
| Fig. 8 Detailed classification of biopolymers based on biodegradability with some examples and other biodegradable polymers that are sourced from petrochemicals with structural representations. | |
Cellulose and chitosan are the two prime abundantly available polysaccharides in nature. Starch, pectin, alginate, carrageenan, gums and their derivatives follow them. Protein-based polymers like corn zein, collagen, wheat gluten, and gelatin are also frequently used for packaging applications.90 Since polysaccharides are the most plentifully available biopolymers, they bring a huge scope to engineer them to replace non-biodegradable plastics. They have an excellent film-forming capability ranging from opaque to transparent. The downsides of these polymers are their high hydrophilicity, weak mechanical strength and poor barrier properties.91 Biodegradable synthesized polymers (PVA, PLA, PBAT, PBS, PCL) are used in combination with polysaccharides or proteins to enhance their mechanical and barrier properties. In this contribution, a few polysaccharides and their derivatives, combinations of polysaccharides or with synthesized polymers were explored as potential carriers of anthocyanins to act as pH indicator systems. A detailed description of polysaccharides and combinations chosen, their pros and cons were given in each individual chapter in the introduction section.
5. Challenges during application as pH indicators
The versatile hues and attractive color-changing ability have marked anthocyanins as excellent pH indicators. What concerns their application in real food systems is their stability to storage conditions. Whilst their instability to pH is the key for indicators, they also are unstable to factors like temperature, light and oxygen whose variations are usually the case for packed food throughout its shelf life.117 Structural changes due to pH are reversible whereas exposure to temperature, light and oxygen destroys the structure leading to degradation, changing the colour to brown or yellow. Anthocyanins extracted from different sources have different degradation temperatures usually ranging from 30 °C to 50 °C.118
Thermal and oxidative stabilities of anthocyanins are most studied in the literature. It is reported that thermal degradation of anthocyanins is dependent on certain factors like pH, co-pigmentation and raw material. Cyanidin-3-glycoside and pelargonidin-3-glycoside are highly vulnerable to heat owing to their substitution at
positions.119 Usually, they are stabilized to temperature by the process of methylation or acetylation. Acylation of anthocyanins with organic acids makes them resistant to heat and inhibits degradation.120 Higher degree of acylation in delphinidin makes blue anthocyanins called ternatins very stable to temperature. Co-pigmented anthocyanins are most used in pH indicating systems with increased thermal stability. Phenolic acids like gallic, rosmarinic,121 caffeic,122 tannic, catechin, p-coumaric and chlorogenic acids have been reported widely in the literature.123 Oxalic acid124 was used with roselle anthocyanins and applied as a freshness indicator for shrimps, and maleic acid125 and chondroitin sulphate126 were combined with blueberry anthocyanins and used for detection of biogenic amines in shrimp spoilage.
Addition of metal cations was also examined to improve the thermal stability of anthocyanins. For instance, Fe3+ was mixed with goji berry anthocyanins and analysed for its efficiency as a CO2 indicator for chicken spoilage detection. The color of the indicator changed from dark green to greyish blue.127 To monitor the freshness of chicken fillet, Cu2+ was used for metal complexation with red cabbage anthocyanins by changing its colour from purple to blue.128 Encapsulation of anthocyanins is also a popular technique to increase their storage stability, but has not been explored for pH indicators in real food systems.129 An increased thermal stability was claimed by Li et al., 2023, where zein and anthocyanin nanoparticles were formed in media of different pH and analysed for their stability at 60–90 °C.129 Improving the photostability of anthocyanins for pH indicators remains least explored. It is understood from the literature that using anthocyanins in freshness indicators requires care during handling and storage.
6. Conclusion and future scope
Flavonoids are abundantly available in nature and held mainly responsible for imparting color to plant parts. Several extraction techniques have been used recently for efficient separation of these compounds to use them as natural food colorants and as pH indicators. Despite the advantages they still lack upgradation as pH indicators owing to their poor stability to thermal, oxidative and photo stresses. Many research articles have only shown the positive side of these indicators, but some gaps need to be addressed in future research. Apart from complex flavonoids, simple polyphenolic acids are expected to have an excellent potential to be pH-sensitive compounds but remain unexplored for this property. Sources of polyphenols extracted and used in developing pH-sensitive indicators are mainly from flesh and peels of fruits and vegetables. Usage of perfectly edible parts of a plant is not encouraged as they are a part of human nutrition. Polyphenol-rich waste flower sources remain unmapped due to lower extraction yield and thermal and oxidative instability despite their brilliant hues. The disadvantages of using biopolymer matrices to embed polyphenols must be overcome as the shelf life of pH-sensitive labels cannot be predicted owing to the inferior properties of biopolymers in terms of moisture resistance and water solubility. Degradation of natural colorants due to thermal and oxidative stresses must be dealt with by stabilizing them in their raw form and also after their employment in pH indicating films.
Author contributions
Konala Akhila: manuscript writing – original draft, visualization, data curation. Dakuri Ramakanth: formal analysis, figures, and review. Lingala Lakshman Rao: formal analysis, figures, and tables review. Suman Singh: visualization, data curation, and editing. Kirtiraj K. Gaikwad: conceptualization, manuscript editing and review, supervision, project administration, funding acquisition.
Conflicts of interest
The authors declare that they have no known competing financial interests or personal relationships that could have appeared to influence the work reported in this paper.
Acknowledgements
Author Konala Akhila would like to thank the Ministry of Education (MoE), Government of India, and Indian Institute of Technology Roorkee for providing resources. Author K.K. Gaikwad would like to thank the Indian Institute of Technology Roorkee.
References
- C. M. Fernandez, J. Alves, P. D. Gaspar, T. M. Lima and P. D. Silva, J. Sci. Food Agric., 2023, 103, 986–1003 CrossRef CAS PubMed.
- K. Ganeson, G. K. Mouriya, K. Bhubalan, M. R. Razifah, R. Jasmine, S. Sowmiya, A. A. A. Amirul, S. Vigneswari and S. Ramakrishna, Food Packag. Shelf Life, 2023, 36, 101044 CrossRef.
-
J. H. Han, Innovations in Food Packaging, 2nd edn, 2014, 3–12 Search PubMed.
- E. Drago, R. Campardelli, M. Pettinato and P. Perego, Foods, 2020, 9, 1628 CrossRef CAS PubMed.
- J. Zhang, S. Liu, C. Xie, C. Wang, Y. Zhong and K. Fan, Crit. Rev. Food Sci. Nutr., 2023, 1–20 Search PubMed.
- K. A. M. O’ Callaghan and J. P. Kerry, Food Packag. Shelf Life, 2016, 9, 1–9 CrossRef.
- J. Alves, P. D. Gaspar, T. M. Lima and P. D. Silva, J. Sci. Food Agric., 2023, 103, 1004–1020 CrossRef CAS PubMed.
- H. Yousefi, H. M. Su, S. M. Imani, K. Alkhaldi, C. D. Filipe and T. F. Didar, ACS Sens., 2019, 4, 808–821 CrossRef CAS PubMed.
- P. Müller and M. Schmid, Foods, 2019, 8(1), 16 CrossRef PubMed.
- L. Gram, L. Ravn, M. Rasch, J. B. Bruhn, A. B. Christensen and M. Givskov, Int. J. Food Microbiol., 2002, 78, 79–97 CrossRef PubMed.
- K. Majer-Baranyi, A. Székács and N. Adányi, Biosensors, 2023, 13, 456 CrossRef CAS PubMed.
- E. Sonwani, U. Bansal, R. Alroobaea, A. M. Baqasah and M. Hedabou, Front. Public Health, 2022, 9, 816226 CrossRef PubMed.
- M. E. Genovese, J. Zia and D. Fragouli, Sustainable Food Packag. Technol., 2021, 369–393 CAS.
- S. K. Kannan, B. Ambrose, S. Sudalaimani, M. Pandiaraj, K. Giribabu and M. Kathiresan, Anal. Methods, 2020, 12, 3438–3453 RSC.
-
S. M. Abdel-Aziz, M. M. S. Asker, A. A. Keera and M. G. Mahmoud, Microbes in Food and Health, 2016, pp. 239–264 Search PubMed.
- B. Kuswandi, M. Moradi and P. Ezati, Packag. Technol. Sci., 2022, 35, 847–862 CrossRef.
- M. Soltani Firouz, K. Mohi-Alden and M. Omid, Food Res. Int., 2021, 141, 110113 CrossRef CAS PubMed.
- N. Alves, C. Moura, J. Coutinho, L. Leite, L. Nobre, I. Boticas, M. Navarro, J. Bessa, F. Cunha, P. Neves and R. Fangueiro, Mater. Proc., 2022, 8, 130 Search PubMed.
- T. Tsironi, P. Ronnow, M. Giannoglou and P. Taoukis, Food Control, 2017, 73, 51–56 CrossRef.
- T. Gao, Y. Tian, Z. Zhu and D. W. Sun, Trends Food Sci. Technol., 2020, 99, 311–322 CrossRef CAS.
- Y. Ma, W. Yang, Y. Xia, W. Xue, H. Wu, Z. Li, F. Zhang, B. Qiu and C. Fu, Membranes, 2022, 12, 477 CrossRef CAS PubMed.
- A. Mirza Alizadeh, M. Masoomian, M. Shakooie, M. Zabihzadeh Khajavi and M. Farhoodi, Crit. Rev. Food Sci. Nutr., 2021, 62, 383–397 CrossRef PubMed.
- E. Mohammadian, M. Alizadeh-Sani and S. M. Jafari, Compr. Rev. Food Sci. Food Saf., 2020, 19, 2885–2931 CrossRef PubMed.
- C. T. Tracey, A. L. Predeina, E. F. Krivoshapkina and E. Kumacheva, Trends Food Sci. Technol., 2022, 127, 87–98 CrossRef CAS.
- Q. Luo, A. Hossen, D. E. Sameen, S. Ahmed, J. Dai, S. Li, W. Qin and Y. Liu, Crit. Rev. Food Sci. Nutr., 2023, 63, 1102–1118 CrossRef CAS PubMed.
- W. Yin, C. Qiu, H. Ji, X. Li, S. Sang, D. J. McClements, A. Jiao, J. Wang and Z. Jin, Food Biosci., 2023, 52, 102378 CrossRef CAS.
- S. Roy and J. W. Rhim, Crit. Rev. Food Sci. Nutr., 2021, 61, 2297–2325 CrossRef CAS PubMed.
- N. Echegaray, N. Guzel, M. Kumar, M. Guzel, A. Hassoun and J. M. Lorenzo, Food Chem., 2023, 404, 134453 CrossRef CAS PubMed.
-
H. I. Mohamed, H. S. El-Beltagi, S. M. Jain and J. M. Al-Khayri, Phytomedicine: A Treasure of Pharmacologically Active Products from Plants, 2021, pp. 483–531 Search PubMed.
-
M. Iyer, K. Pal and V. Upadhye, Recent Frontiers of Phytochemicals: Applications in Food, Pharmacy, Cosmetics, and Biotechnology, 2023, pp. 295–308 Search PubMed.
- I. G. Rafał, B. J. Króliczewski, I. Górniak, R. Bartoszewski and Á. J. Króliczewski, Phytochem. Rev., 2018, 18(1), 241–272 Search PubMed.
- N. Kumar and N. Goel, Biotechnol. Rep., 2019, 24, e00370 CrossRef PubMed.
- K. Akhila, D. Ramakanth and K. K. Gaikwad, J. Coat. Technol. Res., 2022, 19, 1493–1506 CrossRef CAS.
- R. Marchiosi, W. D. dos Santos, R. P. Constantin, R. B. de Lima, A. R. Soares, A. Finger-Teixeira, T. R. Mota, D. M. de Oliveira, M. de P. Foletto-Felipe, J. Abrahão and O. Ferrarese-Filho, Phytochem. Rev., 2020, 19(4), 865–906 CrossRef CAS.
- M. Abotaleb, A. Liskova, P. Kubatka and D. Büsselberg, Biomolecules, 2020, 10, 221 CrossRef CAS PubMed.
- O. Kaisoon, S. Siriamornpun, N. Weerapreeyakul and N. Meeso, J. Funct. Foods, 2011, 3, 88–99 CrossRef CAS.
- B. J. Ahn, K. K. Gaikwad and Y. S. Lee, J. Appl. Polym. Sci., 2016, 133, 44138 CrossRef.
- G. Singh, S. Singh, B. Kumar and K. K. Gaikwad, J. Food Meas. Charact., 2021, 15, 585–593 CrossRef.
- V. Tulyathan, R. B. Boulton and V. L. Singleton, J. Agric. Food Chem., 1989, 37(4), 844–849 CrossRef CAS.
- A. F. Pant, S. Sangerlaub and K. Muller, Materials, 2017, 10(5), 489 CrossRef PubMed.
- A. Aydogdu, G. Sumnu and S. Sahin, Carbohydr. Polym., 2019, 208, 241–250 CrossRef CAS PubMed.
- F. Acevedo, J. Hermosilla, C. Sanhueza, B. Mora-Lagos, I. Fuentes, M. Rubilar, A. Concheiro and C. Alvarez-Lorenzo, Eur. J. Pharm. Sci., 2018, 119, 49–61 CrossRef CAS PubMed.
- A. Aydogdu Emir, E. Yildiz, Y. Aydogdu, G. Sumnu and S. Sahin, Legume Sci., 2021, 3(2), e90 CrossRef CAS.
- Y. Zhao and M. D. A. Saldaña, J. Supercrit. Fluids, 2019, 143, 97–106 CrossRef CAS.
- E. Middleton Jr, Int. J. Pharmacogn., 1996, 34(5), 344–348 Search PubMed.
- E. Z. Ma and A. Khachemoune, Arch. Dermatol. Res., 2023, 315, 321–331 CrossRef CAS PubMed.
- L. Cruz, N. Basílio, N. Mateus, V. De Freitas and F. Pina, Chem. Rev., 2022, 122, 1416–1481 CrossRef CAS PubMed.
- G. Mazza, J. E. Cacace and C. D. Kay, J. AOAC Int., 2004, 87, 129–145 CrossRef CAS PubMed.
- H. Liu, Z. Liu, Y. Wu, L. Zheng and G. Zhang, Int. J. Mol. Sci., 2021, 22, 8441 CrossRef CAS PubMed.
- A. N. Nunes, A. Borges, A. A. Matias, M. R. Bronze and J. Oliveira, Molecules, 2022, 27, 368 CrossRef CAS PubMed.
- R. Brouillard, Anthocyanins Food Colors, 1982, 1–40 CAS.
- J. P. Palta, Remote sensing reviews, 1990, 5(1), 207–213 CrossRef.
- N. Oladzadabbasabadi, A. Mohammadi Nafchi, M. Ghasemlou, F. Ariffin, Z. Singh and A. A. Al-Hassan, Food Packag. Shelf Life, 2022, 33, 100872 CrossRef CAS.
- V. de Freitas and N. Mateus, Environ. Chem. Lett., 2006, 4(3), 175–183 CrossRef CAS.
- A. Houghton, I. Appelhagen and C. Martin, Plants, 2021, 10(4), 726 CrossRef CAS PubMed.
- E. Brglez Mojzer, M. Knez Hrnčič, M. Škerget, Ž. Knez and U. Bren, Molecules, 2016, 21, 901 CrossRef PubMed.
- W. Routray and V. Orsat, Food Bioprocess Technol., 2011, 5(2), 409–424 CrossRef.
- P. Panja, Curr. Opin. Food Sci., 2018, 23, 173–182 CrossRef.
- K. Ameer, H. M. Shahbaz and J. H. Kwon, Compr. Rev. Food Sci. Food Saf., 2017, 16, 295–315 CrossRef PubMed.
- J. Shi, H. Nawaz, J. Pohorly, G. Mittal, Y. Kakuda and Y. Jiang, Food Rev. Int., 2005, 21, 139–166 CrossRef CAS.
- R. Becerril, C. Nerín and F. Silva, Trends Food Sci. Technol., 2021, 111, 495–505 CrossRef CAS.
- H. Guo, C. Shao, Y. Ma, Y. Zhang and P. Lu, Int. J. Biol. Macromol., 2023, 226, 77–89 CrossRef CAS PubMed.
- F. Zeng, Y. Ye, J. Liu and P. Fei, Food Chem.: X, 2023, 17, 100531 CAS.
- M. Faisal, M. Bevilacqua, R. Bro, H. N. Bordallo, J. J. K. Kirkensgaard, K. H. Hebelstrup and A. Blennow, Int. J. Biol. Macromol., 2023, 250, 126250 CrossRef CAS PubMed.
- D. N. Dikmetas, E. Uysal, F. Karbancioglu-Guler and S. Gurmen, Int. J. Biol. Macromol., 2023, 231, 123304 CrossRef CAS PubMed.
- S. Shi, X. Xu, J. Feng, Y. Ren, X. Bai and X. Xia, Food Packag. Shelf Life, 2023, 35, 100994 CrossRef CAS.
- K. Chen, J. Li, L. Li, Y. Wang, Y. Qin and H. Chen, Food Biosci., 2023, 53, 102584 CrossRef CAS.
- F. S. Mohseni-Shahri and F. Moeinpour, Food Sci. Nutr., 2023, 11, 3898–3910 CrossRef CAS PubMed.
- X. Huang, L. Du, Z. Li, J. Xue, J. Shi, H. E. Tahir, X. Zhai, J. Zhang, N. Zhang, W. Sun and X. Zou, Food Chem., 2023, 411, 135497 CrossRef CAS PubMed.
- D. Liu, C. Zhang, Y. Pu, S. Chen, H. Li and Y. Zhong, Food Chem., 2023, 404, 134426 CrossRef CAS PubMed.
- K. Halász, Z. Kóczán and E. Joóbné Preklet, J. Food Meas. Charact., 2023, 17, 2555–2565 CrossRef.
- X. Cheng, Q. Zhao, J. Kang, X. Zhao, X. He and J. Li, ACS Appl. Polym. Mater., 2023, 5, 6307–6317 CrossRef CAS.
- F. Mohseni-Shahri, A. Mehrzad, Z. Khoshbin, M. Sarabi-Jamab, F. Khanmohamadi and A. Verdian, Int. J. Biol. Macromol., 2023, 224, 1174–1182 CrossRef CAS PubMed.
- S. Forghani, F. Zeynali, H. Almasi and H. Hamishehkar, J. Polym. Environ., 2023, 31, 4258–4273 CrossRef CAS.
- S. S. Mirmoeini, M. Moradi, H. Tajik, H. Almasi and F. M. Gama, Food Chem., 2023, 425, 136493 CrossRef CAS PubMed.
- A. Khezerlou, M. Alizadeh Sani, M. Tavassoli, R. Abedi-Firoozjah, A. Ehsani and D. J. McClements, J. Compos. Sci., 2023, 7, 143 CrossRef CAS.
- I. Fierri, L. De Marchi, R. Chignola, G. Rossin, M. Bellumori, A. Perbellini, I. Mancini, A. Romeo, G. Ischia, A. Saorin, F. Mainente and G. Zoccatelli, Antioxidants, 2023, 12, 1757 CrossRef CAS PubMed.
- M. Fidan-Yardimci, S. Akay, F. Sharifi, C. Sevimli-Gur, G. Ongen and O. Yesil-Celiktas, Food Chem., 2019, 293, 57–65 CrossRef CAS PubMed.
- M. Salah, M. Mansour, D. Zogona and X. Xu, Food Res. Int., 2020, 137, 109635 CrossRef CAS PubMed.
- J. Martinović, J. Lukinac, M. Jukić, R. Ambrus, M. Planinić, G. Šelo, A. M. Klarić, G. Perković and A. Bucić-Kojić, Molecules, 2023, 28, 5285 CrossRef PubMed.
- S. Mohammadalinejhad, A. Almonaitytė, I. J. Jensen, M. Kurek and J. Lerfall, Int. J. Biol. Macromol., 2023, 246, 125684 CrossRef CAS PubMed.
- V. G. da Silva, G. F. Nogueira, C. T. Soares and R. A. de Oliveira, Polysaccharides, 2023, 4, 33–50 CrossRef.
- D. Pedrali, A. Scarafoni, A. Giorgi and V. Lavelli, Antioxidants, 2023, 12, 1192 CrossRef CAS PubMed.
- J. Yi, M. Qiu, N. Liu, L. Tian, X. Zhu, E. A. Decker and D. J. McClements, J. Agric. Food Chem., 2020, 68, 10149–10156 CrossRef CAS PubMed.
- R. Shaddel, J. Hesari, S. Azadmard-Damirchi, H. Hamishehkar, B. Fathi-Achachlouei and Q. Huang, Food Hydrocolloids, 2018, 77, 803–816 CrossRef CAS.
- J. Teixé-Roig, G. Oms-Oliu, G. R. Velderrain-Rodríguez, I. Odriozola-Serrano and O. Martín-Belloso, Food Bioprocess Technol., 2018, 11, 2229–2241 CrossRef.
- J. Liu, Y. Tan, H. Zhou, J. L. Muriel Mundo and D. J. McClements, J. Food Eng., 2019, 254, 1–9 CrossRef CAS.
-
R. Grujić, D. Vujadinović and D. Savanović, Advances in Applications of Industrial Biomaterials, 2017, pp. 139–160 Search PubMed.
-
J. Rydz, M. Musiol, B. Zawidlak-Wegrzyńska and W. Sikorska, Biopolymers for Food Design, 2018, pp. 431–467 Search PubMed.
- P. A. Dillon and K. V Aleksanyan, Polymers, 2023, 15, 451 CrossRef PubMed.
- M. Corrales, A. F. García, P. Butz and B. Tauscher, J. Food Eng., 2009, 90, 415–421 CrossRef CAS.
- I. Choi, J. Y. Lee, M. Lacroix and J. Han, Food Chem., 2017, 218, 122–128 CrossRef CAS PubMed.
- F. Ebrahimi Tirtashi, M. Moradi, H. Tajik, M. Forough, P. Ezati and B. Kuswandi, Int. J. Biol. Macromol., 2019, 136, 920–926 CrossRef CAS PubMed.
- V. Shukla, G. Kandeepan, M. R. Vishnuraj and A. Soni, Agric. Res., 2016, 5, 205–209 CrossRef CAS.
- M. A. Sani, M. Tavassoli, H. Hamishehkar and D. J. McClements, Carbohydr. Polym., 2021, 255, 117488 CrossRef CAS PubMed.
- S. Chen, M. Wu, P. Lu, L. Gao, S. Yan and S. Wang, Int. J. Biol. Macromol., 2020, 149, 271–280 CrossRef CAS PubMed.
- C. Shi, J. Zhang, Z. Jia, X. Yang and Z. Zhou, J. Sci. Food Agric., 2021, 101, 1800–1811 CrossRef CAS PubMed.
- Y. Li, K. Wu, B. Wang and X. Li, Int. J. Biol. Macromol., 2021, 174, 370–376 CrossRef CAS PubMed.
- K. Halász and L. Csóka, Food Packag. Shelf Life, 2018, 16, 185–193 CrossRef.
- L. Prietto, T. C. Mirapalhete, V. Z. Pinto, J. F. Hoffmann, N. L. Vanier, L. T. Lim, A. R. Guerra Dias and E. da Rosa Zavareze, LWT-Ed., 2017, 80, 492–500 CrossRef CAS.
- S. Bilgiç, E. Söğüt and A. C. Seydim, Turkish Journal of Agriculture - Food Science and Technology, 2019, 7, 61–66 CrossRef.
- A. Khezerlou, M. Tavassoli, M. Alizadeh-Sani, A. Ehsani and D. J. Mcclements, J. Compos. Sci., 2023, 7(10), 404 CrossRef CAS.
- S. Forghani, F. Zeynali, H. Almasi and H. Hamishehkar, J. Polym. Environ., 2023, 1–16 Search PubMed.
- D. S. B. Anugrah, G. Delarosa, P. Wangker, R. Pramitasari and D. Subali, Packag. Technol. Sci., 2023, 36, 681–697 CrossRef CAS.
- M. Tavassoli, A. Khezerlou, S. Firoozy, A. Ehsani and S. Punia Bangar, Int. J. Food Sci. Technol., 2023, 58, 3050–3057 CrossRef CAS.
- Y. Wu and C. Li, Int. J. Biol. Macromol., 2023, 232, 123464 CrossRef CAS PubMed.
- K. Akhila, A. Sultana, D. Ramakanth and K. K. Gaikwad, Food Biosci., 2023, 52, 102397 CrossRef CAS.
- F. Hematian, H. Baghaei, A. Mohammadi Nafchi and M. Bolandi, Food Sci. Nutr., 2023, 11, 379–389 CrossRef CAS PubMed.
- F. Mohseni-Shahri, A. Mehrzad, Z. Khoshbin, M. Sarabi-Jamab, F. Khanmohamadi and A. Verdian, Int. J. Biol. Macromol., 2023, 224, 1174–1182 CrossRef CAS PubMed.
- M. Ekrami, N. Roshani-Dehlaghi, A. Ekrami, M. Shakouri and Z. Emam-Djomeh, Chemistry, 2022, 4, 1360–1381 CrossRef CAS.
- D. Cui, W. Liu, J. Wang, J. Hu, D. Shan, Y. Wan, Q. Wang and J. Wang, Cem. Concr. Compos., 2022, 134, 104804 CrossRef CAS.
- L. G. Santos, G. F. Alves-Silva and V. G. Martins, Int. J. Biol. Macromol., 2022, 220, 866–877 CrossRef CAS PubMed.
- S. Mulyani, Y. Pratama, M. W. Wijaya, V. Priyo Bintoro and N. A. Hidayati, Int. J. Adv. Sci. Eng. Inf. Technol., 2021, 11(5), 1928–1934 CrossRef.
- R. R. Koshy, J. T. Koshy, S. K. Mary, S. Sadanandan, S. Jisha and L. A. Pothan, Food Control, 2021, 126, 108039 CrossRef CAS.
- Y. Amaregouda, K. Kamanna and A. Kamath, ACS Food Sci. Technol., 2023, 3, 1411–1422 CrossRef CAS.
- K. Akhila, A. Sultana, D. Ramakanth and K. K. Gaikwad, Food Biosci., 2023, 52, 102397 CrossRef CAS.
- A. Castañeda-Ovando, M. de L. Pacheco-Hernández, M. E. Páez-Hernández, J. A. Rodríguez and C. A. Galán-Vidal, Food Chem., 2009, 113, 859–871 CrossRef.
- B. Enaru, G. Drețcanu, T. D. Pop, A. Stǎnilǎ and Z. Diaconeasa, Antioxidants, 2021, 10, 1967 CrossRef CAS PubMed.
- S. Oancea, Antioxidants, 2021, 10, 1337 CrossRef CAS PubMed.
- M. Lu, Y. Cai, X. Chen, Y. Wang and G. Yuan, J. Sci. Food Agric., 2024, 104, 2641–2650 CrossRef CAS PubMed.
- H. He, Y. Song, M. Li, H. Zhang, J. Li, H. Huang and Y. Li, Anal. Methods, 2023, 15, 228–239 RSC.
- D. Klisurova, I. Petrova, M. Ognyanov, Y. Georgiev, M. Kratchanova and P. Denev, Food Chem., 2019, 279, 162–170 CrossRef CAS PubMed.
- J. Huang, Z. Hu, G. Li, Y. Chin, Z. Pei, Q. Yao, D. Li and Y. Hu, Food Res. Int., 2023, 173, 113416 CrossRef CAS PubMed.
- P. Fei, F. Zeng, S. Zheng, Q. Chen, Y. Hu and J. Cai, Dyes Pigm., 2021, 184, 108852 CrossRef CAS.
- Y. Bao, H. Cui, J. Tian, Y. Ding, Q. Tian, W. Zhang, M. Wang, Z. Zang, X. Sun, D. Li, X. Si and B. Li, Food Control, 2022, 131, 108441 CrossRef CAS.
- I. Choi, H. Choi, J. S. Lee and J. Han, Food Chem., 2023, 404, 134534 CrossRef CAS PubMed.
- D. N. Dikmetas, E. Uysal, F. Karbancioglu-Guler and S. Gurmen, Int. J. Biol. Macromol., 2023, 231, 123304 CrossRef CAS PubMed.
- Y. Jang and E. Koh, Food Chem., 2023, 405, 135002 CrossRef CAS PubMed.
- S. Li, X. Wang, X. Zhang, H. Zhang, S. Li, J. Zhou and L. Fan, Food Res. Int., 2023, 166, 112552 CrossRef CAS PubMed.
|
This journal is © The Royal Society of Chemistry 2024 |
Click here to see how this site uses Cookies. View our privacy policy here.