DOI:
10.1039/D3FO02407E
(Review Article)
Food Funct., 2024,
15, 12-36
Evidential support for garlic supplements against diabetic kidney disease: a preclinical meta-analysis and systematic review†
Received
15th June 2023
, Accepted 13th November 2023
First published on 15th November 2023
Abstract
Garlic (Allium sativum L.) is a popular spice that is widely used for food and medicinal purposes and has shown potential effects on diabetic kidney disease (DKD). Nevertheless, systematic preclinical studies are still lacking. In this meta-analysis and systematic review, we evaluated the role and potential mechanisms of action of garlic and its derived components in animal models of DKD. We searched eight databases for relevant studies from the establishment of the databases to December 2022 and updated in April 2023 before the completion of this review. A total of 24 trials were included in the meta-analysis. It provided preliminary evidence that supplementing with garlic could improve the indicators of renal function (BUN, Scr, 24 h urine volume, proteinuria, and KI) and metabolic disorders (BG, insulin, and body weight). Meanwhile, the beneficial effects of garlic and its components in DKD could be related to alleviating oxidative stress, suppressing inflammatory reactions, delaying renal fibrosis, and improving glucose metabolism. Furthermore, time–dose interval analysis exhibited relatively greater effectiveness when garlic products were supplied at doses of 500 mg kg−1 with interventions lasting 8–10 weeks, and garlic components were administered at doses of 45–150 mg kg−1 with interventions lasting 4–10 weeks. This meta-analysis and systematic review highlights for the first time the therapeutic potential of garlic supplementation in animal models of DKD and offers a more thorough evaluation of its effects and mechanisms to establish an evidence-based basis for designing future clinical trials.
1. Introduction
Diabetic kidney disease (DKD) is one of the most prevalent and serious microvascular complications of diabetes mellitus and the leading cause of end-stage renal disease (ESRD).1–4 DKD may occur in about 50% of persons with type 2 diabetes mellitus (T2DM) and 30% of those with type 1 diabetes mellitus (T1DM).5–9 With the increasing number of diabetic patients globally, the incidence of DKD has also risen.9,10 It is estimated that over the next 24 years, the number of patients with DKD will climb by more than 50%, from 537 to 783 million,11 which will significantly impact economic costs and daily life. The clinical manifestation of DKD is characterized by albuminuria, a decline in glomerular filtration rate, and hypertension, eventually leading to ESRD. There are a variety of pathological characteristics of DKD, such as nodular or diffuse glomerulosclerosis, tubulointerstitial fibrosis, tubular atrophy, and renal arteriolar hyalinosis.12,13 Metabolic abnormalities, hemodynamic dysfunction, inflammation, oxidative stress, fibrosis, and their interactions drive the development and progression of DKD toward ESRD.14–18
Substantial evidence suggests that keeping blood pressure and glucose under control is very beneficial for preventing albuminuria in T2DM.19–21 But even with more aggressive glycemic control, many individuals with diabetes still suffer DKD.22 The renin–angiotensin–aldosterone system (RAAS) inhibitors and sodium–glucose cotransporter 2 inhibitors (SGLT-2i) are the main therapeutic methods for hemodynamic disorders.23 The RAAS blocker, which has antihypertensive and antialbuminuric effects, has been the only therapy licensed for renoprotection in DKD over the previous two decades. Angiotensin-converting enzyme inhibitors (ACEI) and angiotensin II receptor blockers (ARBs), which may delay the progression of renal failure, are the most used treatment medications. But in addition to failing to reverse kidney damage, these medications also carry the risk of irreversible acute renal injury, hypotension,24 and hyperkalemia.25 By selectively inhibiting the SGLT2 transporter, which is located in the S1 area of the proximal renal tubule, SGLT-2i produces its hypoglycemic effect via preventing the renal tubule from reabsorbing glucose.26–28 According to a meta-analysis by Kaze et al., SGLT-2i was linked to a lower risk of death and unfavorable kidney outcomes in adults with DKD.29,30 They did note that there are only a few available data points regarding the relationship between SGLT-2i and safety outcomes. Unfortunately, it has been reported that SGLT-2i could cause a sharp drop in blood glucose (BG) levels, and encourages the release of hormones that work against BG regulation, like glucagon, which is linked to the onset of glucose ketoacidosis.31–33 Therefore, the current treatment of DKD is considered unsatisfactory.34 Considering the significant global impact of DKD, exploring more potent and secure natural medicines for DKD is urgently needed.35
Food supplements have their unique, popularly acceptable, and low side effect properties, many of which are beneficial for DKD.36 Among natural resources, dietary supplements have additional advantages accepted by patients. For thousands of years, garlic (Allium sativum L.) has been widely used for food consumption and medicinal purposes, particularly in Arabic,37 Egyptian,38 Chinese,39,40 Persian,41 and Indian42 traditional medicinal systems. In addition, garlic has been shown to exert its beneficial effects against a broad spectrum of diseases, including cancer,43 hypertension,44 hyperlipidemia,45 obesity,46 diabetes,47 bacterial infections,48 fungal infections,49 viral infections,50 parasitic diseases,51 asthma,52 immunological disorders53 and cardiovascular diseases.54 These therapeutic properties are attributed to its several bioactive components, such as allitride, allicin, diallyl trisulfide, allyl methyl sulfide, S-ethyl cysteine, S-allyl cysteine, S-allyl mercapto cysteine, and S-methyl L-cysteine.55,56 With the deepening of research on the anti-diabetes and microcirculation-protective effects of garlic, more and more literature has reported that garlic products and garlic components could significantly improve renal function, reduce albuminuria, and relieve renal microstructure abnormalities.57 However, we still find some contradictory results in a comprehensive literature review. For example, most studies have shown that garlic products and ingredients significantly reduce the 24 h urinary albumin excretion rate (UAER), yet different results were obtained in the experiment by Yu et al.58 Similar inconsistencies were also found in serum creatinine (Scr) outcomes. The teams of Arellano Buendía59,60 and Venkataiah61 discovered that comparing the garlic group with the model group, the Scr levels did not statistically significantly decrease, and Ziamajidi et al.62 discovered that garlic even marginally increased Scr levels (P > 0.05). Besides, considering the small sample size of these in vivo studies, the statistical ability is limited. Based on these rich and controversial experimental results, as well as the limitations of small sample sizes and poor statistical power in the design of individual animal experiments, the introduction of meta-analysis and systematic review was necessary to guide the application of garlic supplements and provide evidential support for the further design of dietary strategies and conducting of large-scale clinical trials.
Meta-analysis and systematic review are widely recognized as high-level evidence for clinical trials. In recent years, this method has been advocated for preclinical animal experiment quality evaluation and result analysis, thus reducing the risk of translating preclinical studies to clinical studies and the waste of animal experiment resources. The meta-analysis and systematic review of preclinical animal experiments makes a unique contribution to the exploration of pathological mechanisms, which is also crucial for the re-evaluation of nutrient effectiveness and the exploration of the mechanism of therapeutic action of natural products. To thoroughly investigate the effects of garlic, preclinical studies using animal DKD models were subjected to a quality evaluation and meta-analysis in this systematic review. In-depth discussion is also given to the ideal dosage and duration for applying garlic, as well as to the underlying mechanisms and existing obstacles.
2. Methods
Preferred Reporting Items for Systematic Reviews and Meta-Analyses (PRISMA)63 was used to formulate and conduct this meta-analysis and systematic review. This research was registered on the PROSPERO platform (registration number: CRD42022384309).
2.1 Search strategy
Searches were conducted independently by two authors and screened independently according to the inclusion–exclusion criteria, and any disagreements were resolved through discussion or consultation with the corresponding author. Publications from eight databases were included in our search: PubMed, Cochrane Library, EMBASE, Web of Science, China National Knowledge Internet, China Biology Medicine Disc, Chinese VIP Database, and Wanfang database from the establishment of the database until December 2022. We also conducted a forward search of included studies until April 2023. There were no language limitations on the search. Utilizing a combination of MeSH and free-text terms, diseases and interventions were identified: (“Diabetic Nephropathies”, “Nephropathies, Diabetic”, “Nephropathy, Diabetic”, “Diabetic Nephropathy”, “Diabetic Kidney Disease”, “Diabetic Kidney Diseases”, “Kidney Disease, Diabetic”, “Kidney Diseases, Diabetic”, “Diabetic Glomerulosclerosis”, “Glomerulosclerosis, Diabetic”, “Intracapillary Glomerulosclerosis”, “Nodular Glomerulosclerosis”, “Glomerulosclerosis, Nodular”, “Kimmelstiel–Wilson Syndrome”, “Kimmelstiel Wilson Syndrome”, “Syndrome, Kimmelstiel–Wilson”, “Kimmelstiel–Wilson Disease”, “Kimmelstiel Wilson Disease”), and (“Allium sativum”, “garlic”, “Alliin”, “S allylcysteine sulfoxide”, “PCSO”, “isoalliin”, “Allicin”, “allylthiosulphinic acid allyl ester”, “diallyl disulfide oxide”, “allylthiosulfinate”, “allimin”, “S allyl cysteine”, “diallyl trisulfide”, “allyl trisulfide”, “allitridin”, “allitridum”, “allitridi”, “Dasuansu”, “allyl sulfide”, “diallylsulfide”, “diallyl sulfide”, “garlic oil”, “ajoene”, “allyl mercaptan”, “S methyl L cysteine”, “allyl methyl sulfide”, “methyl propenyl sulfide”, “allylmethylsulfide”, “diallyl disulfide”, “allyl disulfide”, “diallyl disulphide”, “allyll disulfide”, “allitin”, “garlicin”). Additionally, some search terms were appropriately modified to match other databases. ESI Table 1† displays the whole electronic search technique for each database.
2.2 Eligibility criteria
The following predetermined inclusion criteria applied: (1) participants: DKD model animals; (2) intervention: garlic or its derived components; (3) the control group either received no therapy, or an equivalent dose of a non-active sterile liquid (such as saline or carboxymethyl cellulose); (4) outcomes: the primary outcomes were blood urea nitrogen (BUN), Scr, 24 h urine volume, proteinuria (24 h UP, 24 h UAER, and albumin/creatinine ratio), Kidney Index (KI), and BG. For a more thorough evaluation of renal function, at least one of BUN, Scr, 24 h urine volume, proteinuria, and KI must also be available. The secondary outcomes were insulin, body weight, and the mechanisms (e.g. oxidative stress index, inflammatory factors) of garlic against diabetic kidney damage.
The following predetermined exclusion criteria were applied: (1) an abstract, review, case study, in vitro, human, or in silico was the type of paper that was published; (2) literature that fulfilled the requirements for inclusion but was not accessible from the source text through reasonable access; (3) no control group or the test group without garlic and its derived compounds or concomitant treatment with oral hypoglycemic agents, insulin or other interventions; (4) duplicate publications; (5) non-DKD models; (6) there is no information on the type of article data, such as standard deviation (SD) or standard error (SE), or there is no predetermined primary outcome index.
2.3. Data extraction
The retrieved material was managed with EndNote (Version X9) software. Following deduplication, the retrieved studies were reviewed by two independent authors according to the inclusion and exclusion criteria. In order to exclude items that were not relevant, the titles and abstracts were first checked. To determine if the remaining articles were appropriate for meta-analysis, the entire texts of the remaining publications were then examined. The following information from each article was taken: (1) article title, the first author's name, and publication year; (2) basic characteristics of animals (species, sex, age, sample size, and body weight); (3) method of modeling (the dosage of a medication needed for model establishment, the delivery method, and the model success judgment index); (4) interventions (the method of delivery, dosage, and duration); and (5) outcome indicators and differences between groups.
Data that were manually extracted from the included literature were compiled into a database of tables. The data for the graphically represented outcome indicators were extracted using the GetData Graph Digitizer software (version 2.26). We only collected the data from the highest dose for the findings obtained at various doses for the meta-analysis. Furthermore, if the trial investigated the effects of garlic at several time points, only the data from the latest point were collected for meta-analysis. The conversion formula
was used to convert the data from the text to SD if it displayed the SE.64 Urea and BUN are actually the same indexes. According to their conversion relationship, 1 mg dL−1 urea = 2.14 mg dl−1 BUN, and they were gathered and then displayed as BUN in the following.
2.4. Risk of bias in individual studies
Each study's risk of bias was evaluated independently by two authors using the Systematic Review Center for Laboratory Animal Experimentation (SYRCLE) risk of bias tool (RoBT) 10-item scale.64 One point (10 total points) was awarded for each item. Negotiation or third-party arbitration were used to settle the disagreements resulting from the evaluation.
2.5. Statistical analyses
For statistical analysis, RevMan software (version 5.3) and Stata software (version 15.0) were both used. A standard mean difference (SMD) and 95% confidence interval (95% CI) were employed to show the effect size for predetermined outcomes, which were considered as continuous variables. The meta-analysis, which took into account the variations between investigations, the weights of each study, and the accuracy of individual studies, was carried out using a random-effects model. Heterogeneity was expressed using the (I2) index and I2 > 50% suggested substantial heterogeneity. The robustness of the results to ambiguous judgments or data assumptions was evaluated using sensitivity analysis in Stata 15.0. Since the majority of the included studies reported on BUN, Scr, 24 h urine volume, 24 h UAER, and BG, we provide the following six subgroups to assess the impact of the variables or study characteristics on the predicted effect size: (1) animal species (mice and rats); (2) model categories (T1DM and T2DM); (3) garlic categories (garlic products and garlic components); (4) garlic dose (<100, 100–400, and >400 mg kg−1); (5) duration (<10 weeks and ≥10 weeks); and (6) route of administration (by intragastric, by intraperitoneal injection, and by free access with meals). Furthermore, subgroup analysis was frequently utilized to identify the origins of heterogeneity. Additional meta-regression analysis was carried out using Stata if the predetermined six subgroups (characteristics) were unable to identify the source of heterogeneity and the research remained extremely heterogeneous (I2 > 50%). The criteria for selecting characteristics for the meta-regression analysis included following the recommendations in the Cochrane Handbook for Systematic Reviews of Interventions65 and avoiding selecting characteristics that have been predetermined in the subgroup analysis. Meta-regression analysis was performed to analyze ≥10 primary outcomes. Statistical significance was defined as P < 0.05. Only when at least ten studies were included in the meta-analysis were the Egger's test and the Trim-and-fill method utilized to evaluate publication bias. We utilized time–dose scatter plots with garlic extracts and garlic components that were distinguished by sphere size to better illustrate the effect of garlic type, dose, and duration on outcome indicators like 24 h urine volume, 24 h UAER, and BG.
3. Results
3.1. Study selection
A total of 250 articles (28 from PubMed, 25 from Embase, 24 from Web of Science, 13 from Cochrane Library, 54 from CNKI, 85 from VIP, 8 from CBM, and 13 from Wanfang) were retrieved from online databases. Of these, 74 were duplicates. When the remaining 176 articles were evaluated by browsing the titles and abstracts, 136 of them were eliminated because they were reviews, comments, case reports, non-DKD models, not treated with garlic and its components, or non-animal studies. The following were the primary reasons for removing 16 studies after carefully reading the entire text: (1) absence of primary outcomes that have been predetermined; (2) meeting abstract; (3) duplicate literature data; (4) combination with other agents; (5) no model success criteria. Eventually, this systematic review and meta-analysis included 24 publications.58–62,66–84 Over 70% of the literature was published within the previous ten years (i.e., 2012–2022), and the first of these included studies appeared in 2007, indicating that the great potential of garlic in the treatment of DKD is of increasing recent interest. Fig. 1 illustrates the comprehensive selection procedure.
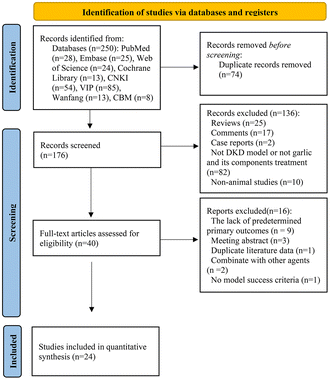 |
| Fig. 1 Flowchart of selection for studies’ inclusion. | |
3.2. Study characteristics
The 24 studies that were included enrolled a total of 472 diabetic model animals: 228 model groups and 244 treatment groups. Rats or mice served as the basis for the animal models used in the experiments; 11 studies used Wistar rats (n = 170), 8 studies used Sprague-Dawley rats (n = 170), 2 studies used Balb/cA mice (n = 90), and one study used C57BL/6 mice (n = 10). Without mentioning specific species, one study merely mentioned albino rats (n = 12), and another only mentioned rats (n = 20). Two studies utilized animal models without sex information; one employed a female model; one used animals of either gender; and the remaining 20 studies used male animals. There were 11 articles that stated the age of the experimental animals, which ranged from three weeks to three months. One study did not specify the weight of the animal, while the other 23 did. In terms of animal modeling methods, 15 studies used a single intraperitoneal injection of high-dose streptozotocin (STZ), and two studies used a continuous intraperitoneal injection of small doses of STZ. Additionally, two investigations built models using alloxan. Furthermore, STZ was combined with a high-fat diet in four studies, while STZ and nicotinamide were employed in just one experiment. In 15 studies, BG levels (fasting blood glucose (FBG) > 11.1 mmol L−1 and higher or random BG > 16.7 mmol L−1 and above) were used as an independent indicator to assess modeling performance, and in 9 additional studies, abnormal BG and aberrant urine as a criterion for successful modeling. The minimum and maximum dosing periods were 4 and 20 weeks, respectively. The garlic products included raw garlic extracts, aqueous garlic extract, garlic juice, aged garlic extract, garlic essential oil capsule, garlic oil, and nano-emulsified garlic oil, while the garlic components included allicin, allitride, S-allyl cysteine, S-propyl cysteine, diaceto-dipropyl disulphide, S-methyl cysteine, S-ethyl cysteine, and S-allyl mercapto cysteine (Fig. 2). The dosage for garlic products ranged from 500 mg kg−1 day−1 for the lowest dose to 8000 mg kg−1 day−1 for the highest dose. In addition, the lowest and maximum daily doses for the components of garlic were 15 mg kg−1 and 150 mg kg−1, respectively. Since three research teams—Yuvashree, Mong, and Yin—designed two different garlic intervention groups, each regarded as an independent study (Yuvashree 2020(A),77 Yuvashree 2020(B),77 Mong 2012(A),76 Mong 2012(B),76 Yin 2007(A),83 and Yin 2007(B))83 in the meta-analysis. Table 1 lists the specific characteristics of the listed studies.
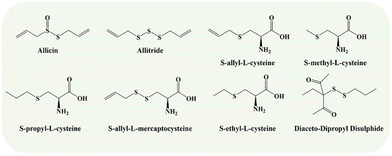 |
| Fig. 2 The chemical structure of garlic components. | |
Table 1 Basic characteristics of the included studies
Study |
Species (sex; n = treatment/control group), age; weight |
Establish |
Modeling standard |
Experimental group |
Control group |
Administration/duration |
Outcomes |
SIJ, single intraperitoneal injection; CIJ, consecutive intraperitoneal injection; NS, normal saline; NM: not mentioned; a. BUN, b. Scr, c. 24 h urine volume, d. 24 h UAER, e. 24 h UP, f. albumin/creatinine, g. KI, h. BG, i. serum insulin, j. body weight, k. MDA, l. SOD, m. GPx, n. GSH, o. TBARS, p. ROS, q. nitrite, r. TNFα, s. IL-6, t. NF-κB, u. IL-1β, v. TGF-β1, w. collagen, x. PKC;↑: significantly increased compared to the diabetic control, ↓: significantly decreased compared to the diabetic control, /: no significant difference compared with the diabetic control or not mentioned. |
Al-Qattan (2008)67 |
SD rats (male; 10/10), NM; 180–200 g |
SIJ STZ (60 mg kg−1) |
BG > 19.4 mmol L−1 |
Raw garlic extracts; 500 mg kg−1 day−1 |
The same volume of NS |
By intraperitoneal injection/7 weeks |
e↓, h↓ |
Arellano Buendía (2018)59 |
Wistar rats (male; 6/6), NM; 280–310 g |
SIJ STZ (50 mg kg−1) |
FBG > 13.9 mg dL−1 |
Allicin; 16 mg kg−1 day−1 |
The same volume of vehicle solution |
By intragastric/30 days |
b/, c↓, e/, h↓, j/, s↓, t↓, u↓, v↓ |
Arellano Buendía (2020)60 |
Wistar rats (male; 7/7), NM; 280–310 g |
SIJ STZ (50 mg kg−1) |
FBG > 13.9 mg dL−1 |
Allicin;16 mg kg−1 day−1 |
The same volume of vehicle solution |
By intragastric/30 days |
a/, b/, c↓, h↓, i/, v↓ |
Hfaiedh (2013)70 |
Wistar rats (male; 6/6), 3 month; about 160 g |
SIJ alloxan (120 mg kg−1) |
BG > 11.1 mmol L−1; Glycosuria |
Aqueous garlic extract; 300 mg kg−1 day−1 |
NM |
By intraperitoneal injection/4 weeks |
a↓, b↓, c↓, e↓, j↑, l↑, m↑, o↓ |
Huang (2016)71 |
SD rats (male; 12, 12, 12/12), NM; 200–220 g |
SIJ STZ (60 mg kg−1) |
FBG ≥ 16.7 mmol L−1;24 h UV &24 h urine albumin > 50% before modeling |
Allitride; 15, 30, 45 mg kg−1 day−1 |
The same volume of NS |
By intragastric/12 weeks |
a↓, b↓, d↓, g↓, h↓, j/, v↓, w↓ |
Huang (2017)72 |
SD rats (NM; 12/12), 8 weeks; NM |
SIJ STZ (60 mg kg−1) |
BG > 16.7 mmol L−1 |
Allitride; 15, 30, 45 mg kg−1 day−1 |
The same volume of NS |
By intragastric/12 weeks. |
a↓, b↓, c/, d↓, g↓, h↓, j/ |
Jiang (2019)98 |
SD rats (male; 11/11), 8 weeks; 200–250 g |
SIJ STZ (60 mg kg−1) |
BG > 16.7 mmol L−1; urine sugar is strongly positive |
Allitride; 150 mg kg−1 day−1 |
The same volume of PBS |
By intragastric/4 weeks |
a↓, b↓, d↓, h/, k↓, l↑ |
Kemmak (2011)78 |
Wistar rats (male; 8/8), NM; 230–270 g |
SIJ STZ (60 mg kg−1) |
BG > 16.7 mmol L−1 |
Garlic juice; 10 ml kg−1 day−1 |
Regular diet |
By intragastric/6 weeks |
c↓, d↓, f↓, h↓ |
Luan (2017)(A)68 |
Wistar rats (NM; 15/15), NM; 180–220 g |
HFD + SIJ STZ (25 mg kg−1) |
FBG ≥ 16.8 mmol L−1; urine sugar (+++–++++) |
Allitride; 60 mg kg−1 day−1 |
The same volume of NS |
By intraperitoneal injection/12 weeks |
a↓, b↓, d↓, h↓ |
Luan (2017)(B)69 |
Wistar rats (male and female; 10/10), NM; 180–220 g |
HFD + SIJ STZ (25 mg kg−1) |
FBG ≥ 16.8 mmol L−1; urine sugar (+++–++++) |
Allitride; 20, 40, 60 mg kg−1 day−1 |
The same volume of NS |
By intraperitoneal injection/12 weeks |
a↓, b↓, d↓, h↓, w↓, x↓ |
Mariee (2009)75 |
SD rats (male; 10/10), NM; 180–200 g |
SIJ STZ (60 mg kg−1) |
FBG > 13.9 mg dl−1 |
Fresh garlic homogenate; 200, 400 mg kg−1 day−1 |
The same volume of NS |
By intragastric/30 days |
f↓, h↓, i↑, j↑, n↑, o↓, q↑ |
Mong (2012)(A)76 |
Balb/cA mice (male, 10/10), 3–4 weeks; 4.3 ± 0.7 g |
CJ STZ (40 mg kg−1) for 5 days |
FBG ≥ 14.0 mmol L−1 |
S-Allyl cysteine; 0.5, 1 g L−1 added to drinking water |
Regular diet |
By free access with meal/10 weeks |
a↓, c↓, g↓, h↓, i↑, j↑, p↓, r↓, s↓, t↓, x↓ |
Mong (2012)(B)76 |
Balb/cA mice (male, 10/10), 3–4 weeks; 4.3 ± 0.7 g |
CIJ STZ (40 mg kg−1) for 5 days |
FBG ≥ 14.0 mmol L−1 |
S-Propyl cysteine; 0.5, 1 g L−1 added to drinking water |
Regular diet |
By free access with meal/10 weeks. |
a↓, c↓, g↓, h↓, i↑, j↑, p↓, r↓, s↓, t↓, x↓ |
Nasiri (2017)66 |
Wistar rats (male; 6/6), 6–8 weeks; 250–300 g |
SIJ STZ (65 mg kg−1) |
FBG > 16.7 mmol L−1 |
Aqueous garlic extract; 2000 mg kg−1 day−1 |
NM |
By intragastric/30 days |
a↓, b/, h↓, j↑, k↓, q↓, r↓ |
Shen (2022)73 |
Rats (female; 10, 10, 10/10), NM; 180–200 g |
HFD + SIJ STZ (35 mg kg−1) |
FBG ≥ 16.7 mmol L−1; 24 h UP quantitative >30 mg |
Allitride;10, 20, 40 mg kg−1 day−1 |
The same volume of NS |
By intragastric/8 weeks |
e↓, p↓, u↓ |
Shiju (2013)(A)80 |
Wistar rats (male; 6/6), 8 weeks; 200–250 g |
SIJ STZ (45 mg kg−1) |
BG > 16.7 mmol L−1 |
Aqueous garlic extract; 500 mg kg−1 day−1 |
NM |
By intragastric/10 weeks |
a↓, b↓, c↓, d↓, h↓, j↑ |
Shiju (2013)(B)79 |
Wistar rats (male; 6/6), 8 weeks; 200–250 g |
SIJ STZ (45 mg kg−1) |
BG > 16.7 mmol L−1 |
Aged garlic extract; 500 mg kg−1 day−1 |
NM |
By intragastric/10 weeks |
a↓, b↓, c↓, f↓, h/, j/ |
Thomson (2013)81 |
SD rats (male; 10/10), 2 monthS; 150–200 g |
SIJ STZ (60 mg kg−1) |
BG > 16.5 mmol L−1 |
Aqueous garlic extract; 500 mg kg−1 day−1 |
The same volume of NS |
By intraperitoneal injection/8 weeks |
b↓, c↓, d↓, e↓, f↓, h↓, i↑, j↑ |
Venkataiah (2016)61 |
Albino rats (male; 6/6), NM; 200–250 g |
SIJ alloxan (150 mg kg−1) |
BG > 13.9 mmol L−1 |
Diaceto-dipropyl disulphide; 100 mg kg−1 day−1 |
The same volume of NS |
By intragastric/60 days |
b/, h/, o↓ |
Yin (2007)(A)83 |
Balb/cA mice (male, 15, 15, 15, 15/15), 3–4 weeks; 22.1 ± 0.5 g |
CIJ STZ (40 mg kg−1) for 5 days |
FBG ≥ 14.0 mmol L−1 |
S-Methyl cysteine; 0.5, 1, 1.5, 2 g L−1 |
Regular diet |
By free access with meal/6 weeks |
a↓, c↓, e↓, h↓, i↑, j↑, k↓, m↑, n↑, r↓, s↓, v↓, w↓, x↓ |
Yin (2007)(B)83 |
Balb/cA mice (male, 15, 15, 15, 15/15), 3–4 weeks; 22.1 ± 0.5 g |
CIJ STZ (40 mg kg−1) for 5 days |
FBG ≥ 14.0 mmol L−1 |
S-Ethyl cysteine; 0.5, 1, 1.5, 2 g L−1 |
Regular diet |
By free access with meal/6 weeks |
a↓, c↓, e↓, h↓, i↑, j↑, k↓, m↑, n↑, r↓, s↓, v↓, w↓, x↓ |
Yu (2008)82 |
SD rats (male; 10/10), NM; 180–200 g |
SIJ STZ (55 mg kg−1) |
BG ≥ 16.7 mmol L−1 |
Garlic essential oil capsules; 2000, 8000 mg kg−1 day−1 |
The same volume of distilled water |
By intragastric/8 weeks |
d↓, g↓, h/, k↓, l↑, v↓, w↓ |
Yu (2010)58 |
SD rats (male; 10/10), NM; 180–200 g |
SIJ STZ (NM) |
BG ≥ 16.7 mmol L−1 |
Garlic essential oil capsules; 2000, 8000 mg kg−1 day−1 |
The same volume of distilled water |
By intragastric/8 weeks |
d↓, v↓, w↓ |
Yuvashree (2020)(A)77 |
Wistar rats (male; 6/6), NM; 150–180 g |
HFD + SIJ STZ (35 mg kg−1) |
BG > 16.7 mmol L−1 |
Nanoemulsified garlic oil blend; 20 mg kg−1 day−1 |
NM |
By intragastric/5 months |
g↑, h↓, f↓ |
Yuvashree (2020)(B)77 |
Wistar rats (male; 6/6), NM; 150–180 g |
HFD + SIJ STZ (35 mg kg−1) |
BG > 16.7 mmol L−1 |
Garlic oil blend; 20 mg kg−1 day−1 |
NM |
By intragastric/5 months |
g↑, h/, f↓ |
Zhou (2021)84 |
C57BL/6 mice (male; 5/5), 6–8weeks; 18 ± 2 g |
SIJ STZ (130 mg kg−1) |
FBG ≥ 11.1 mmol L−1 |
S-Allyl mercapto cysteine cyclodextrin inclusion compound; 50, 100 mg kg−1 day−1 |
The same volume of distilled water |
By intragastric/8 weeks |
a↓, b↓, d↓, g↓, h/, j/, s↓, t↓, u↓, w/ |
Ziamajidi (2017)62 |
Wistar rats (male; 6/6), 6–8 weeks; 200–250 g |
SIJ STZ (65 mg kg−1) + Nicotinamide (110 mg kg−1) |
FBG > 13.9 mmol L−1 |
Aqueous garlic extract; 2000 mg kg−1 day−1 |
NM |
By intragastric/33 days |
a↓, b/, h↓, j↑, k↓, q↓, r↓ |
3.3. Risk of bias and quality of included studies
All included studies were evaluated for quality. The scores for included studies ranged from 2 to 6. Only one study got 2 points (4.17%),74 4 studies got 3 points (20.83%),58,60,68,69,81 14 studies got 4 points (58.33%),59,61,66,67,70,72,73,75,77–80,82,84 3 studies got 5 points (12.5%),62,76,83 and 1 study got 6 points (4.17%).71 Only one study divided experimental animals into groups using a random number table,71 while one study was assigned according to the results of body weight;73 the information concerning this procedure was not mentioned in the remaining research. Comparable baseline traits were reported in six investigations.62,71,72,76,82,83 No studies claimed that the allocation to the various groups was sufficiently hidden. Since the housing conditions for animals were stated in 18 studies in all as being the same, we assumed that the animals were randomly housed during the experiment.59–62,66,67,70,71,73,75–81,83,84 Two studies60,81 performed random outcome assessment, but the specific randomized procedures were not reported. In terms of blinding of intervention measures and outcome evaluation, every study was unable to determine the precise risk. Of the total 24 studies, 2 reports had incomplete data,60,74 in 1 study81 we were unable to determine whether data loss had occurred, due to a lack of information in the paper, and the remaining 21 included all animals in the final analysis. There were no additional sources of bias in any of the trials that were considered, and all pre-reported results were published. The methodological quality assessment of the included studies is presented in Fig. 3.
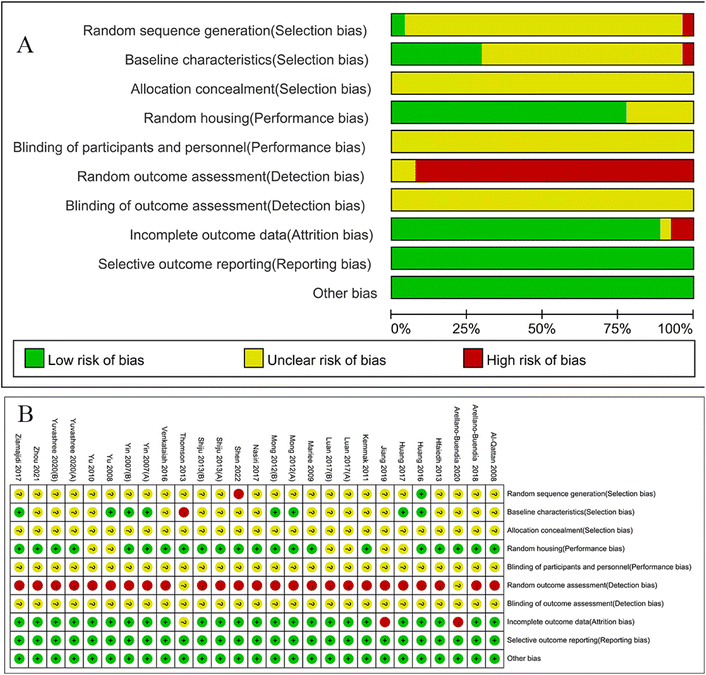 |
| Fig. 3 Risk of (A) bias graph and (B) bias summary. | |
3.4. Effects of intervention
3.4.1. Primary outcome.
3.4.1.1. Effect of garlic and its components on BUN.
Eleven of the 16 trials (which involved 284 animals) provided information on the effectiveness of garlic and its derived components on BUN levels in comparison with the model group.60,62,66,68–72,74,76,79,80,83,84 Overall analysis findings showed that as compared with the model group, garlic and its derivatives could dramatically reduce BUN levels (SMD: −3.86 [95% CI: −5.04, −2.69], P < 0.00001; heterogeneity: I2 = 88%, P < 0.00001) (Fig. 4(a)).
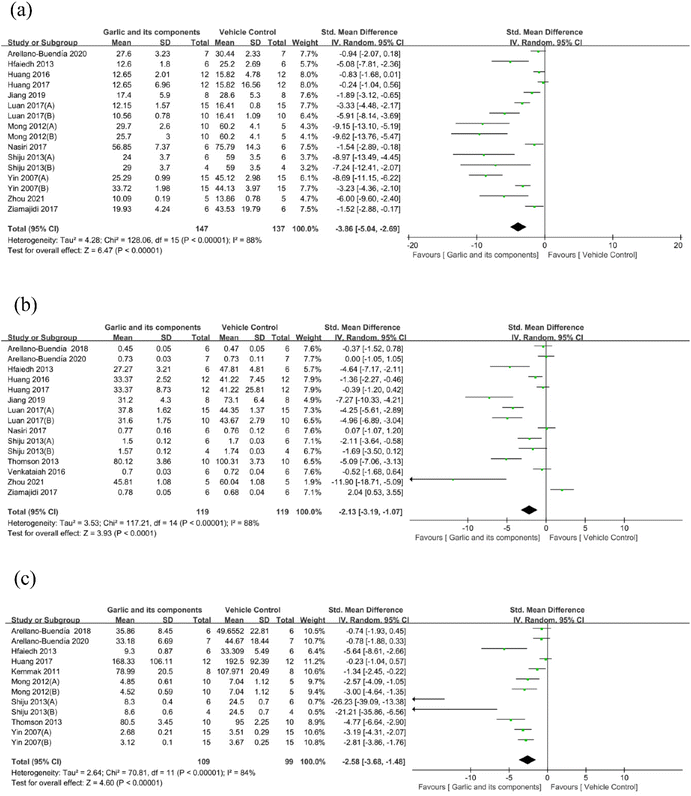 |
| Fig. 4 Standard mean differences estimates for the effect of garlic or its derivatives on (a) BUN, (b) Scr, and (c) 24 h urine volume. | |
3.4.1.2. Effects of garlic and its components on Scr.
The Scr level was examined in 15 studies (including 238 animals). Overall study findings revealed that as compared with the model group, garlic and its components could lower Scr levels (SMD: −2.13 [95% CI: −3.19, −1.07], P < 0.0001; heterogeneity: I2 = 88%, P < 0.00001)59–62,66,68–72,74,79–81,84 (Fig. 4(b)).
3.4.1.3. Effect of garlic and its components on 24 h urine volume.
Analysis of 12 studies59,60,70,72,76,78–81,83 showed that the garlic group had a markedly reduced 24 h urine volume in DKD models compared with the control group (n = 208; SMD: −2.58 [95% CI: −3.68, −1.48], P < 0.00001; heterogeneity: I2 = 84%, P < 0.00001) (Fig. 4(c)).
3.4.1.4. Effect of garlic and its components on proteinuria.
Data on the effectiveness of garlic and its components on 24 h UAER levels in comparison with the model group were supplied in 11 studies (containing 212 animals).58,68,69,71,72,74,78,80–82,84 It is reported that 24 h UAER was significantly decreased after garlic treatment (SMD: −3.03 [95% CI: −4.43, −1.64], P < 0.0001; heterogeneity: I2 = 91%, P < 0.00001) (Fig. 5(a)). With a total of 72 treatment rats and 72 control rats, 7 studies reported measuring the 24 h UP.59,67,70,73,81,83 Garlic and its derived components were able to dramatically lower the content of 24 h UP as compared with the control group (SMD: −3.60 [95% CI: −5.19, −2.02], P < 0.00001; heterogeneity: I2 = 87%, P < 0.00001) (Fig. 5(b)). The urinary albumin–creatinine ratio is a sensitive marker of kidney injury.85 When compared with the model group, garlic products and their derivatives could also reduce the albumin/creatinine ratio (SMD: −4.96 [95% CI: −8.60, −1.32], P = 0.008; heterogeneity: I2 = 90%, P < 0.00001) (Fig. 5(c)). Two studies also revealed that the urine albumin level was considerably lower in the garlic group compared with the model group.78,79
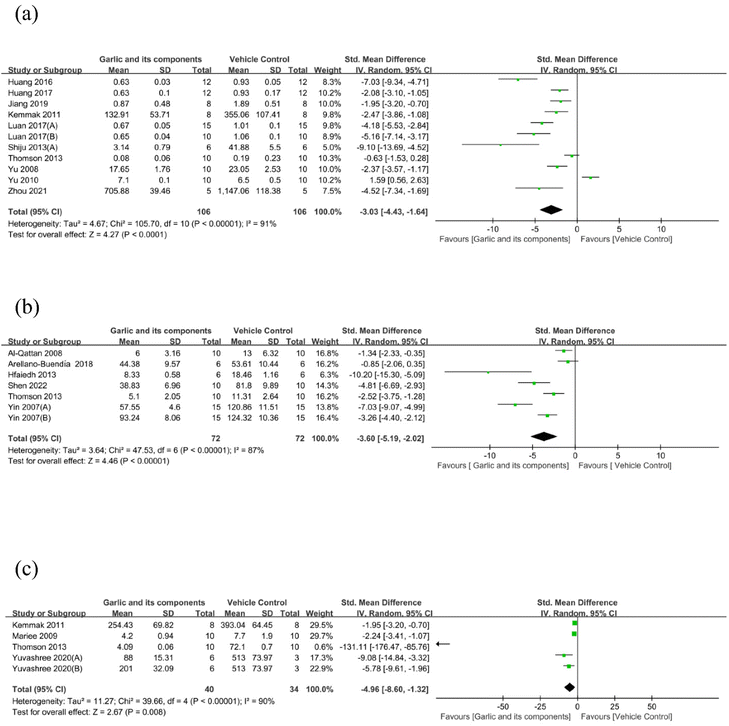 |
| Fig. 5 Standard mean differences estimates for the effect of garlic or its derivatives on (a) 24 h UAER, (b) 24 h UP, and (c) albumin/creatinine ratio. | |
3.4.1.5. Effect of garlic and its components on KI.
The kidney index, or KI, is the kidney weight to body weight ratio. Eight studies reported the measurement of KI.71,72,76,77,82,84 Garlic and its components could also decrease KI compared with the model group (SMD: −2.43 [95% CI: −4.03, −0.83], P = 0.003; heterogeneity: I2 = 87%, P < 0.00001) (Fig. 6(a)).
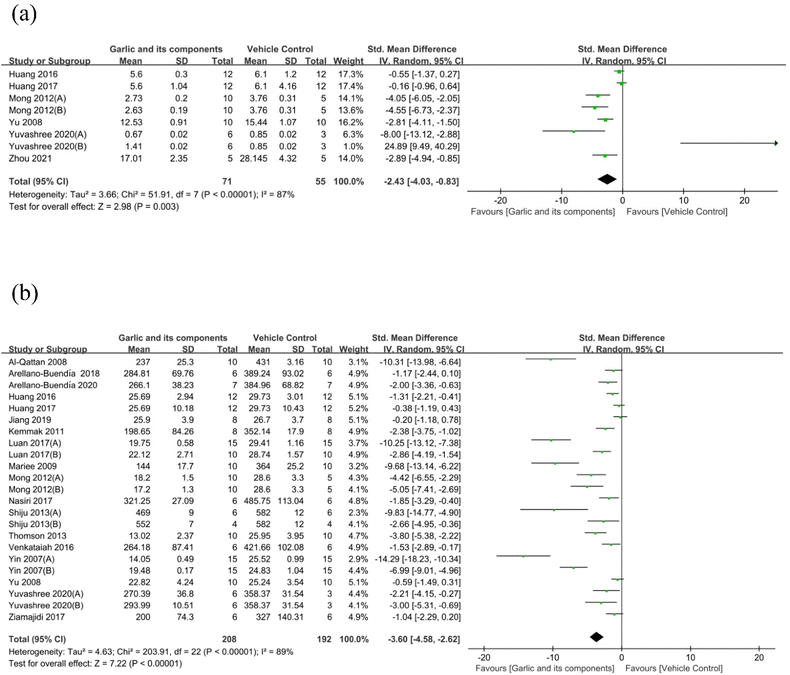 |
| Fig. 6 Standard mean differences estimates for the effect of garlic or its derivatives on (a) KI and (b) BG. | |
3.4.1.6. Effect of garlic and its components on BG.
Twenty-four studies59–62,66–69,71,72,74–84 evaluated BG among these studies (I2 = 89%, P < 0.00001). Due to the missing SD value, the data from one study cannot be used for meta-analysis.84 The results of the meta-analysis showed that the garlic group and the model group differed statistically significantly (n = 400; SMD: −3.60 [95% CI: −4.58, −2.62], P < 0.00001) (Fig. 6(b)).
3.4.2. Secondary outcomes.
3.4.2.1. Effect of garlic and its components on serum insulin.
The serum insulin data were available in eight studies.60,75,76,81,83,84 Data from two of these studies were unavailable for the meta-analysis since they lacked SD values.81,84 The findings showed that when compared with the model group, the garlic group was able to dramatically raise insulin levels in the DKD model (n = 124; SMD: 6.33 [95% CI: 3.53, 9.14], P < 0.00001; heterogeneity: I2 = 92%, P < 0.00001) (Fig. 7(a)).
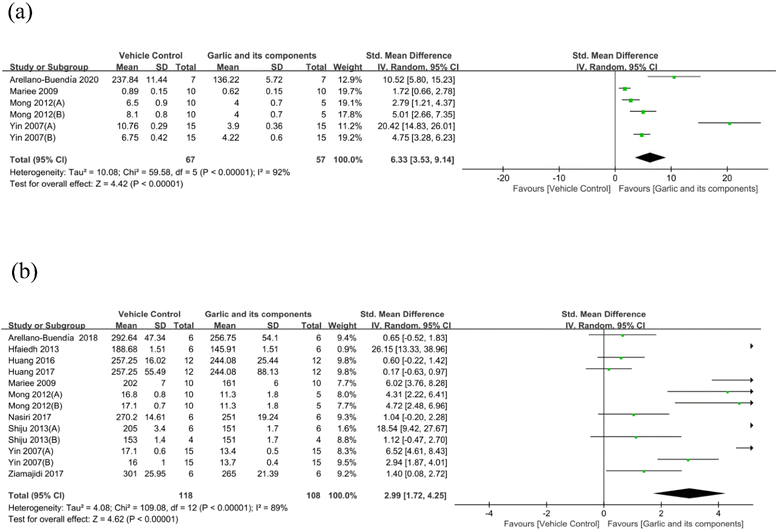 |
| Fig. 7 Standard mean differences estimates for the effect of garlic or its derivatives on (a) serum insulin and (b) body weight. | |
3.4.2.2. Effect of garlic and its components on body weight.
Thirteen trials provided information on the effects of garlic and its constituents on body weight levels when compared with the model group,59,62,66,70–72,75,76,79,80,83 including 226 animals. We excluded the study by Thomson because it only showed data on weight changes.81 Analysis of these studies revealed that the intervention with garlic and its components resulted in higher body weight levels than the control groups (SMD: 2.99 [95% CI: 1.72, 4.25], P < 0.00001; heterogeneity: I2 = 89%, P < 0.00001) (Fig. 7(b)).
3.4.2.3. Oxidative stress index changes.
Malondialdehyde (MDA) was used as an outcome measure in six studies.62,66,74,82,83 It revealed that when compared with the control group, the MDA levels in the garlic group were much lower (n = 120, SMD: −2.78 [95% CI: −3.79, −1.77], P < 0.00001; heterogeneity: I2 = 70%, P = 0.005) (Fig. 8(a)). Three studies have reported the effect of garlic and its components on superoxidase dismutase (SOD) protein levels.70,74,82 The SOD levels in the intervention group were higher than those in the control group, according to the results of the SOD meta-analysis (n = 48, SMD: 3.67 [95% CI: 2.65, 4.69], P < 0.00001; heterogeneity: I2 = 0%, P = 0.9) (Fig. 8(b)). According to the three studies, the glutathione peroxidase (GPx) was downregulated in the DKD model,70,83 whereas garlic and its components could upregulate the GPx (n = 72, SMD: 2.81 [95% CI: 0.74, 4.88], P = 0.008; heterogeneity: I2 = 87%, P = 0.0005) (Fig. 8(c)). Three studies75,83 measured glutathione (GSH) and found that garlic significantly enhanced GSH compared with the control group (n = 80, SMD: 6.68 [95% CI: 3.76, 9.59], P < 0.00001; heterogeneity: I2 = 83%, P = 0.003) (Fig. 9(a)). In addition, three studies provided quantitative data on the thiobarbituric acid-reacting substances (TBARS).61,70,75 The pooled data from three studies suggested that garlic has the potential to drastically lower TBARS in DKD models. (n = 44, SMD: −4.18 [95% CI: −5.81, −2.55], P < 0.00001; heterogeneity: I2 = 44%, P = 0.17) (Fig. 9(b)). For the assessment of reactive oxygen species (ROS), data from three animal experiments were pooled.73,76 A significant reduction of ROS was observed (n = 50, SMD: −8.05 [95% CI: −14.03, −2.07], P = 0.008; heterogeneity: I2 = 88%, P = 0.0002) (Fig. 9(c)). Three studies reported nitrite as an outcome index.62,66,75 One of them reported that garlic could significantly elevate nitrite levels.75 However, two other studies have reached the opposite conclusion.62,66 When all data were combined, the SMD for the treatment effect of garlic and its derived components on nitrite was not statistically significant (n = 44, SMD: −0.30 [95% CI: −4.57, 3.97], P = 0.89; heterogeneity: I2 = 95%, P < 0.00001) (Fig. 9(d)). Two studies60,66 reported total antioxidant capacity, one of which indicated no obvious difference between the treatment and model groups.60 Two studies62,66 showed a significant decrease in total oxidant status. Only two studies used nitric oxide (NO) and nitric oxide synthase (NOS) as results, which was markedly inhibited by garlic component treatments.76 One study74 showed a significantly lower level of the subunit p22phox of reduced nicotinamide adenine dinucleotide phosphate (NADPH) oxidase levels. Besides, only one study reported that the inhibited activities of catalase (CAT) were largely corrected in animals treated with garlic extract compared with model values.70
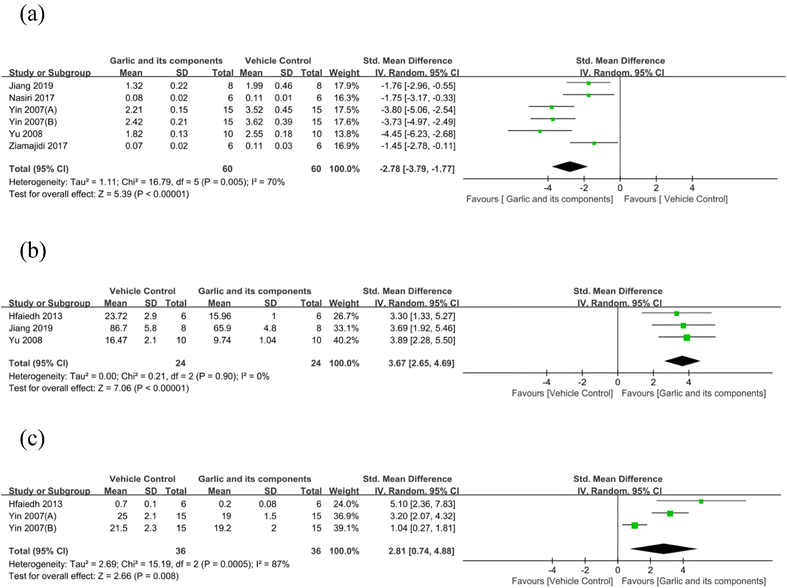 |
| Fig. 8 Standard mean differences estimates for the effect of garlic or its derivatives on (a) MDA, (b) SOD, and (c) GPx. | |
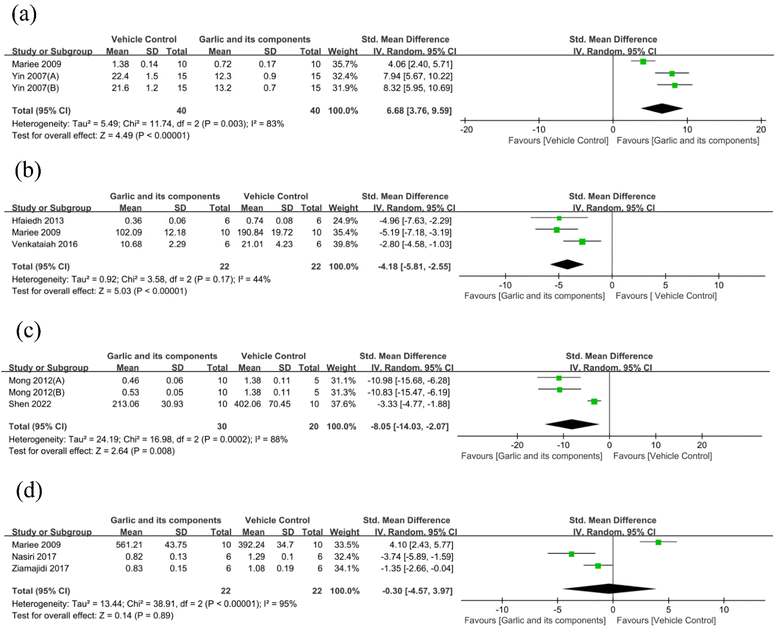 |
| Fig. 9 Standard mean differences estimates for the effect of garlic or its derivatives on (a) GSH, (b) TBARS, (c) ROS, and (d) nitrite. | |
3.4.2.4. Biomarkers of inflammation.
According to the TNFα analysis results,62,66,76,83 the garlic group was able to reduce TNFα expression in DKD animals (n = 114, SMD: −4.11 [95% CI: −5.93, −2.29], P < 0.00001; heterogeneity: I2 = 85%, P < 0.00001) (Fig. 10(a)). Six studies used interleukin (IL)-6 as outcome index.59,76,83,84 Overall study findings revealed that when compared with the model group, garlic and its components could lower the level of IL-6 (n = 108, SMD: −7.23 [95% CI: −9.38, −5.08], P < 0.00001; heterogeneity: I2 = 65%, P = 0.01) (Fig. 10(b)). Four reported NF-κB levels.59,76,84 The model group showed a significant increase in NF-κB level, while garlic and its components could decrease NF-κB level (n = 48, SMD: −5.51 [95% CI: −8.93, −2.08], P = 0.002; heterogeneity: I2 = 80%, P = 0.002) (Fig. 10(c)). Three studies evaluated IL-1β, and the results of the total study revealed a notable decrease in IL-1β levels following the garlic administration (n = 38, SMD: −2.75 [95% CI: −4.36, −1.15], P = 0.0008; heterogeneity: I2 = 38%, P = 0.2) (Fig. 10(d)). Only one study referred to changes in IL-4 and IL-10. According to Yin et al., IL-4 and IL-10 levels were considerably elevated by s-methyl cysteine (P < 0.05) compared with the model group, but not by S-ethyl cysteine.83
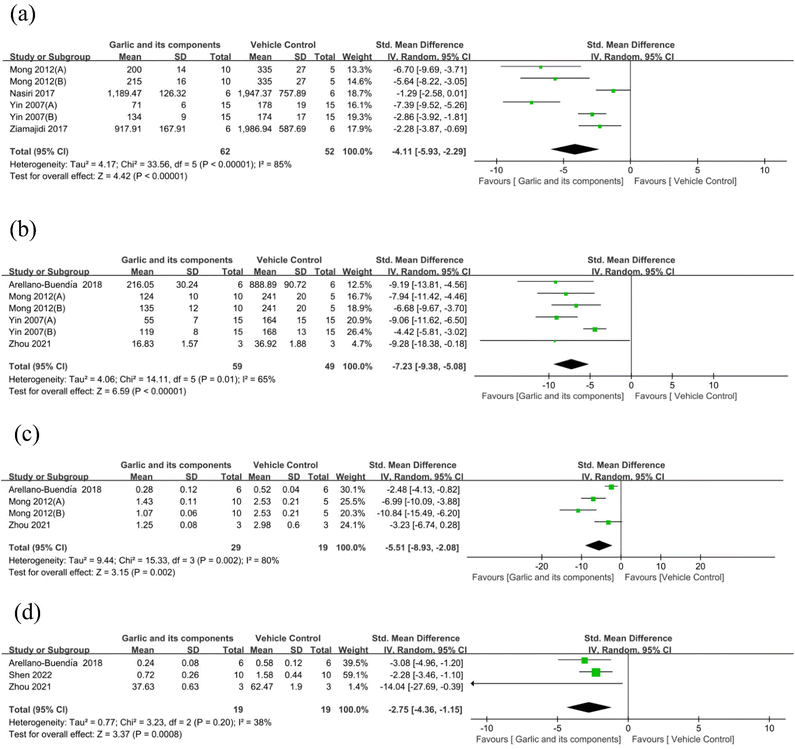 |
| Fig. 10 Standard mean differences estimates for the effect of garlic or its derivatives on (a) TNFα, (b) IL-6, (c) NF-κB, and (d) IL-1β. | |
3.4.2.5. Delaying renal fibrosis.
In eight studies, transforming growth factor-β1 (TGF-β1) was used as the outcome measure.58–60,71,72,82,83 Data from two of the studies were unavailable for meta-analysis because they lacked SD information.58,82 According to the results of the TGF-β1 analysis, the garlic group was able to suppress the expression level of TGF-β1 protein in comparison with the model group (n = 126, SMD: −7.73 [95% CI: −10.64, −4.82], P < 0.00001; heterogeneity: I2 = 86%, P < 0.00001) (Fig. 11(a)). Collagen I was used as an outcome measure in four studies,69,71,72,84 and the results of the overall analysis revealed that the levels of collagen I significantly decreased following garlic administration (n = 74, SMD: −6.43 [95% CI: −10.99, −1.87], P = 0.006; heterogeneity: I2 = 94%, P < 0.00001) (Fig. 11(b)). Four studies used collagen IV as an outcome parameter,58,82,83 but the data from two of the studies were inadequate for meta-analysis. Collagen IV is only described as follows. Yin et al. showed that S-ethyl cysteine and S-methyl cysteine significantly lowered collagen IV levels (P < 0.05).83 Besides, both studies reported lower levels of α-smooth muscle actin (α-SMA),60,68 while one of them also reported lower levels of vimentin, and the other reported lower levels of connective tissue growth factor (CTGF). Fibronectin was reported as an outcome index in three studies.58,82,84 However, there was not enough information in two of the studies for meta-analysis. Thus, Zhou et al.84 reported that S-allyl mercaptocysteine cyclodextrin inclusion compound could lower the level of fibronectin, while also decreasing the expression of vimentin and increasing the E-cadherin level.
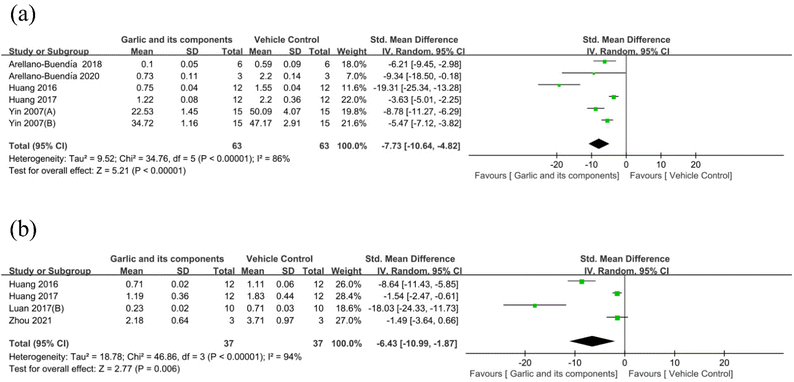 |
| Fig. 11 Standard mean differences estimates for the effect of garlic or its derivatives on (a) TGF-β1 and (b) collagen I. | |
3.4.2.6. Other renoprotective mechanisms.
The included studies also suggested potential therapeutic effects of garlic products and their derivatives on DKD perhaps also through other renoprotective mechanisms. Four studies reported phosphorylated extracellular signal-regulated kinase (p-ERK) 1/2 measurement;71,72,76 the data of two studies were not available for meta-analysis.72,76 Compared with the model group, p-ERK1/2 could be significantly decreased by garlic and its components. PKC activity data were available in five studies.69,76,83 The results of the meta-analysis demonstrated that garlic derivatives could dramatically lower PKC levels (n = 110, SMD: −8.20 [95% CI: −11.34, −5.06], P < 0.00001; heterogeneity: I2 = 84%, P < 0.0001) (Fig. 12). Only one study reported that after diallyl trisulfide treatment, the level of NOD-like receptor family 3 (NLRP3), apoptosis-associated spot-like protein, caspase-1, and IL-18 decreased. Two studies reported that the level of hypoxia-inducible factor-1α (HIF-1α) could be decreased by garlic and its components.60,84 Two studies demonstrated a decreased level of vascular endothelial growth factor (VEGF) after garlic treatment.60,80 Only one study found no significant difference in erythropoietin (Epo) and erythropoietin receptor (Epo-R) levels between the intervention group and control group.60 Only one study demonstrated the improved expression of Wnt 3a, β-catenin, and low-density lipoprotein receptor-related protein 5/6 (LRP5/6), which is a well-recognized receptor for Wnt signaling activation.84 Decreased NFE2-related factor 2 (Nrf2) and increased Kelch-like erythroid cell-derived protein with CNC homology (ECH)-associated protein 1 (Keap1) were observed in DKD models, and all the above indexes were reversed by allicin.60 In addition, there are also key proteins including glucose transporter (GLUT) 4, GLUT1, insulin receptor substrate (IRS)-1, IRS-2, peroxisome proliferator-activated receptor (PPAR)-α, and PPAR-γ that are also regulated by garlic and related components, which reverses the abnormal state of DKD.69,76
 |
| Fig. 12 Standard mean differences estimates for the effect of garlic or its derivatives on PKC. | |
3.5. Sensitivity analysis
For BUN, Scr, 24 hours urine volume, BG, 24 hours UP, 24 hours UAER, and KI, we conducted a sensitivity analysis. After we progressively eliminated each trial from the meta-analysis, there was no significant difference between the pre- and post-sensitivity pooled effects for BUN, Scr, 24 h urine volume, BG, 24 h UP, 24 h UAER, and KI. The lowest and highest pooled effects of BUN after excluding the data from the investigations of “Yin (2007)(A)”83 and “Huang (2016)”71 were −4.20 (95% CI: −5.61, −2.78) and −5.18 (95% CI: −6.86, −3.49), respectively. The lowest and greatest pooled effects of Scr after excluding data from the investigations by “Jiang (2019)”74 and “Ziamajidi (2017)”62 were −2.15 (95% CI: −3.34, −0.96) and −2.84 (95% CI: −4.04, −1.64). The lowest and highest pooled effects of 24 h urine volume following the exclusion of experimental data from the studies “Shiju (2013)(A)”80 and “Huang (2017)”72 were −2.55 (95% CI: −3.62, −1.49) and −3.12 (95% CI: −4.34, −1.90). In terms of the pooled effects of BG, the lowest and highest values were −3.45 (95% CI: −4.40, −2.51) and −4.06 (95% CI: −5.13, −3.00) when data from the studies by “Yin (2007)(A)”83 and “Huang (2017)”72 were excluded. After excluding data from the studies “Yin (2007)(A)”83 and “Arellano Buendía (2018)”,59 the results showed that the lowest and highest pooled effects of 24 h UP were −3.11 (95% CI: −4.58, −1.64) and −4.39 (95% CI: −6.22, −2.56) respectively. When data from the studies by “Shiju (2013)(A)”80 and “Yu (2010)”58 were eliminated, the lowest and highest 24 h UAER pooled effects were −2.86 (95% CI: −4.29, −1.42) and −3.65 (95% CI: −4.89, −2.42). After data from the research carried out by “Yuvashree (2020)(A)”77 and “Huang (2017)”72 were disregarded, the lowest and highest KI pooled effects were −2.13 (95% CI: −3.84, −0.43) and −3.06 (95% CI: −5.23, −0.90).
3.6. Subgroup analysis and meta-regression
Due to the significant heterogeneity of the research, we evaluated the BUN, Scr, 24 h urine volume, 24 h UAER, and BG concerning the species of animals (rats/mice), modeling category (T1DM/T2DM), duration of treatment (<10/≥10 weeks), garlic category (garlic products/garlic components), doses of garlic (<100/100–400/>400 mg kg−1 day−1), and route of administration (by intragastric/by intraperitoneal injection/by free access with meals). Since Kemmak et al.78 used 10 ml kg−1 d−1 of garlic juice and did not elaborate on the preparation of the garlic juice, this literature was not included in the dose analysis. In addition, the teams of Mong76 and Yin83 used “1 g L−1” or “2 g L−1” of garlic components added to drinking water, so we performed a rough calculation based on the mean values of drinking water and body weight defined in the literature. We converted them into a uniform “mg kg−1 day−1” unit for further analysis and comparison. Also, since Yu et al. failed to provide the STZ dosage, we were unable to identify model categories. We disqualified this study from the pertinent subgroup analysis.58 Surprisingly, the Scr index did not show any statistically significant changes in the T2DM subgroup, garlic products subgroup, or high-dosage subgroup (>400 mg kg−1 d−1) between the treatment group and the model group (P > 0.05). The low-dose subgroup (100 mg kg−1 d−1) and the oral administration route subgroup showed no differences between the treatment group and the control group under the 24 h urine volume index. The 24 h UAER index did not reveal any statistically significant differences between the treatment group and the model group for the garlic products subgroup or high-dose subgroup (>400 mg kg−1 d−1) (P > 0.05). The remaining results for BUN, Scr, 24 h urine volume, 24 h UAER and BG for all subgroups remained significantly different between the experimental and model groups, implying that garlic and its components still had an effect on the subgroups under most of the condition categories we set, but the dose, route of administration, and type of garlic seem to be able to have a great effect on the therapeutic effect of garlic that cannot be ignored.
BUN, Scr, and BG index subgroup analysis revealed that mice had a better effect than rats in terms of animal species. (SMD −7.11 vs. SMD −2.54, P < 0.05; SMD −11.90 vs. SMD −1.93, P < 0.05; SMD −7.31 vs. SMD −2.79, P < 0.05). There was no significant difference in 24 h urine volume and 24 h UAER among different animal species (SMD −2.92 vs. SMD −2.53, P = 0.66; SMD −4.52 vs. SMD −2.91, P = 0.32). The BUN and BG outcome indexes’ heterogeneity marginally decreased after subgrouping by animal species. Stratified analysis of 24 h urine volume showed that the effect size of the modeling category for the T2DM model showed better results than that for the T1DM model (SMD −24.05 vs. SMD −2.26, P < 0.0001). In comparison, the effect size of the garlic category for garlic products showed better results than that for garlic components (SMD −6.43 vs. SMD −1.84, P = 0.03). By subgrouping the model category, the heterogeneity of 24 h urine volume and 24 h UAER could be slightly reduced compared with the previous studies. Subgroup analysis of BUN and BG revealed that the effect of free access with meals was superior to that of intraperitoneal injection and intragastric administration in terms of the route of administration (SMD −7.42 vs. SMD –4.52 vs. SMD −1.84, P = 0.002; SMD −7.31 vs. SMD –6.47 vs. SMD −1.82, P = 0.0002). However, subgroup analysis of Scr and 24 h urine volume indicated that the effect of intraperitoneal injection was better than that of free access with meals and intragastric administration (SMD −4.63 vs. SMD NM vs. SMD −1.01, P < 0.00001; SMD −5.01 vs. SMD –2.92 vs. SMD −1.25, P = 0.002), and the heterogeneity was slightly lower than before. A significant difference was seen in the subgroup analysis of BUN and 24 h urine volume between the high-dose treatment group (>400 mg kg−1 day−1), middle-dose treatment group (100–400 mg kg−1 day−1), and low-dose treatment group (<100 mg kg−1 day−1) (SMD −4.53 vs. SMD −6.02 vs. SMD −2.01, P = 0.03; SMD −4.56 vs. SMD −3.28 vs. SMD −0.5, P < 0.0001). Additionally, after being divided into subgroups according to the dosage of garlic, the heterogeneity of the 24 h urine volume partially decreased. In terms of the period of treatment, only subgroup analysis of 24 h UAER showed that a more extended period of treatment (≥10 weeks) was better than that of shorter treatment (<10 weeks) (SMD −4.99 vs. SMD –1.53, P = 0.008), and the heterogeneity was partially lower than before. However, the heterogeneity persisted even when the outcome data had been divided up into several subgroups, indicating that the predetermined groupings did not entirely account for the cause of the result heterogeneity. Based on the findings of the subgroup analysis, we advise further investigation of garlic constituents in comparison with garlic extract for the treatment of DKD and using the component to create intraperitoneal formulations or design nutritional regimens that can be added to meals for concurrent administration. Due to the significant variations in the doses utilized for garlic components and garlic extracts, we performed time–dose interval analysis based on the classification of garlic types further in the text to better guide future experimental dose design and clinical application. ESI Table 3† displays the results.
We conducted a meta-regression analysis from the study quality score, sample size, region (Western, China, others), and year of publication (2007 to 2016, 2017 to 2022) to further investigate the sources of heterogeneity in BUN, Scr, 24 h urine volume, 24 h UAER, and BG. The findings suggested that the “Year of Publication” may be a source contributing to the heterogeneity of the BUN index. “Study quality score” is a potential source leading to heterogeneity of the Scr index. For 24 h urine volume-outcome indicators, “Region” and “Year of Publication” are the sources of heterogeneity between studies. In terms of BG index, “Sample size” is a potential source leading to heterogeneity. The details of the meta-regression and subgroup analysis are shown in ESI Table 4.†
3.7. Publication bias
For BUN, Scr, 24 h urine volume, 24 h UAER, and BG (Fig. 13), Egger's test revealed a statistically significant publication bias (P < 0.05). The trim-and-fill technique was used to address the asymmetry. The results of the trim-and-fill analysis revealed that the size of the overall pooled effect size was unaffected by the data from these missed studies. Table 2 presents the results of the trim-and-fill analysis.
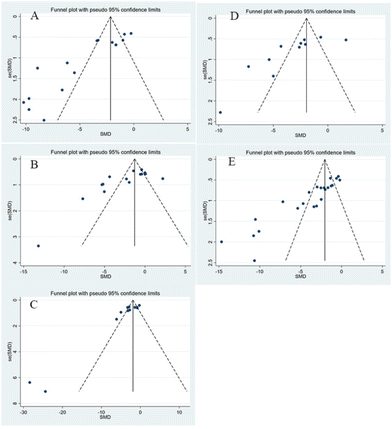 |
| Fig. 13 Funnel plots of (A) BUN, (B) Scr, (C) 24 h urine volume, (D) 24 h UAER, and (E) BG. | |
Table 2 Results from Egger's test and trim-and-fill analysis on BUN, SCr, 24 h urine volume, 24 h UAER, and BG
Outcomes |
Egger's P value |
|
Before trim and fill |
|
|
After trim and fill |
No. studies |
P value |
Total effect sizes [95% CI] |
No. studies |
P value |
Total effect sizes [95% CI] |
BUN, blood urea nitrogen; Scr, serum creatinine; 24 h, 24 hours; UAER, urinary albumin excretion rates; BG, blood glucose; CI, confidence interval. |
BUN |
0.000 |
0.000 |
−4.21 [−5.44, −2.98] |
16 |
0.000 |
−2.68 [−3.93, −1.44] |
21 |
Scr |
0.007 |
0.000 |
−2.32 [−3.45, −1.20] |
15 |
0.003 |
−1.78 [−2.94, −0.63] |
16 |
24 h urine volume |
0.001 |
0.000 |
−2.83 [−4.01, −1.65] |
12 |
0.000 |
−2.49 [−3.81, −1.18] |
14 |
24 h UAER |
0.008 |
0.000 |
−3.24 [−4.70, −1.78] |
11 |
0.000 |
0.06 [0.01, 0.24] |
12 |
BG |
0.000 |
0.000 |
−3.86 [−4.87, −2.84] |
23 |
0.000 |
−3.86 [−4.87, −2.84] |
23 |
3.8. Time–dose interval analysis
In this investigation, the effect results of BUN, Scr, 24 h urine volume, 24 h UAER, and BG included in the study were incorporated into the time–dose table along with the dosage and treatment cycle of garlic and its derivatives. The larger spheres are used to display garlic extracts or products, while the smaller spheres are used to display garlic components. Since Kemmak et al.78 used 10 ml kg−1 d−1 of garlic juice and did not elaborate on the preparation of the garlic juice, this literature was not included in this analysis. In addition, Huang et al. did not mention the statistical difference in 24 h urine volume before and after garlic administration (P value).72 Thus, we excluded the two articles from this analysis. Then we conducted a categorization discussion to provide better results for future research in light of the notable variations in the dosage of garlic products and components.
The lowest dose of a garlic product that significantly decreased BUN (P < 0.05) was 300 mg kg−1, the highest dose was 2000 mg kg−1, and the treatment period ranged from 4 weeks to 10 weeks. The doses of garlic components needed to lower BUN (P < 0.05) ranged from 45 mg kg−1 to 850 mg kg−1, while the duration of the intervention ranged from 4 to 12 weeks (Fig. 14(a)). To effectively reduce Scr levels (p < 0.05), garlic products were provided at a minimum dose of 300 mg kg−1 and a maximum dose of 500 mg kg−1 for a minimum treatment period of 4 weeks and a maximum treatment period of 10 weeks; the minimum amount of garlic component required was 45 mg kg−1 and the maximum dose was 150 mg kg−1 for a minimum and maximum intervention period of 4 weeks to 12 weeks, respectively (Fig. 14(b)). In order to significantly increase 24 h urine volume (P < 0.05), garlic products had to be administered at a minimum dose of 300 mg kg−1 and a maximum dose of 500 mg kg−1. The minimum and maximum treatment periods were 4 weeks and 10 weeks, respectively. The needed garlic component was administered at doses ranging from 16 mg kg−1 to 850 mg kg−1, with a minimum administration time of 4 weeks and a maximum treatment period of 10 weeks (Fig. 14(c)). The minimum dose of garlic products that significantly reduced 24 h UAER was 500 mg kg−1, while the maximum dose was 8000 mg kg−1, and the duration of interventional dosing ranged from 8 weeks to 10 weeks. The minimum effective dose of the garlic component was 45 mg kg−1, while the maximum effective dose was 150 mg kg−1, with a minimum interventional dosing period of 4 weeks and a maximum of 12 weeks (Fig. 14(d)). The minimum dose of garlic product required to significantly improve BG was 20 mg kg−1, while the maximum dose was 2000 mg kg−1, administered over a period ranging from 4 weeks to 20 weeks. The minimum effective dose of the garlic component was 16 mg kg−1, while the maximum dose was 850 mg kg−1, and the minimum intervention period for administration was 4 weeks, while the maximum intervention period was 12 weeks (Fig. 14(e)).
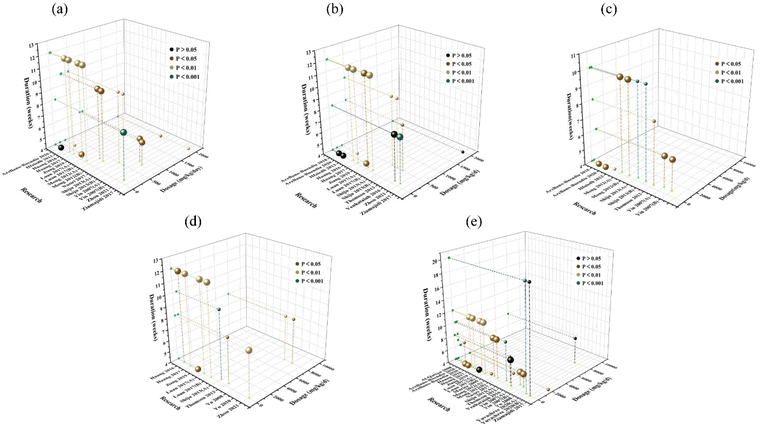 |
| Fig. 14 Time–dose interval analysis scatter plot for (a) BUN, (b) Scr, (c) 24 h urine volume, (d) 24 h UAER, and (e) BG (the larger spheres: garlic products; the smaller spheres: garlic components). | |
Overall, the findings showed that garlic products, supplied at doses of 500 mg kg−1 with interventions lasting 8–10 weeks, and garlic components, administered at doses of 45–150 mg kg−1 with interventions lasting 4–10 weeks, had relatively greater effectiveness. By analyzing the results of the literature with a P > 0.05 effect, we found that allicin with 16 mg kg−1 d−1 had no significant effect on BUN and Scr of DKD after continuous intervention for 4 weeks.59,60 Intervention with 8-week 100 mg kg−1 d−1 diaceto-dipropyl disulphide for 8 weeks had no significant effect on Scr and BG indexes.61 Additionally, there was no significant difference between the DKD group and the treated group in BG after 4-week 150 mg kg−1 d−1 allitride, 20-week 20 mg kg−1 d−1 or 8-week 8000 mg kg−1 d−1 garlic oil intervention.74,77,82 This may be related to the different processes of garlic products and the different types of garlic components. The current evidence is insufficient to prove that allicin and diaceto-dipropyl disulphide have beneficial effects on DKD. By comparing the effects of different doses of allitride (40, 45, 60, 150 mg kg−1 day−1), we found that the lower dose was able to obtain more positive findings than the higher dose (150 mg kg−1 day−1), with the lower dose showing an all-around benefit especially on BG. According to the studies by the teams of Yu82 and Yuvashree,77 it was shown that 2000 mg kg−1 d−1 and 8000 mg kg−1 d−1 garlic essential oil capsules and 20 mg kg−1 d−1 garlic oil blends could not improve BG. Therefore, the available data indicate that garlic oil does not modulate blood glucose in models of DKD. However, the 20 mg kg−1 d−1 nano-emulsified garlic oil blend could significantly improve BG levels, indicating that the development of innovative garlic formulations is equally crucial to improve clinical applications.
4. Discussion
4.1. Summary of evidence
This preclinical systematic review and meta-analysis is, to our knowledge, the first to evaluate the effectiveness and potential mechanisms of action of garlic and its components on diabetes-related kidney injury. It showed that garlic and its components improved renal function indicators, such as BUN, Scr, 24 h urine volume, proteinuria, and KI; glycolipid metabolism indicators, BG, insulin, body weight; oxidative stress indicators, such as MDA, SOD, GPx, GSH, TBARS, and ROS; inflammatory indicators, such as IL-6, TNF-α, NF-κB, IL-1β; and anti-fibrosis indicators, such as TGF-β1 and Collagen I. There is a lack of discussion on the safety and toxicity of garlic in the studies we included. Previous preclinical toxicity studies suggested that garlic and its derivatives have a credible safety profile.86,87 Thus, it provides prospective value for developing functional foods and dietary supplements for garlic in clinical practice.
4.2. Possible protective mechanisms of garlic and its components
4.2.1. Alleviating oxidative stress.
Oxidative stress in the kidneys plays a vital role in the occurrence and development of DKD.88 The two primary categories of oxidants produced within the body are ROS and reactive nitrogen species (RNS).89 A significant mechanism for cellular damage brought on by ROS, such as free oxygen radicals, is excessive MDA or TBARS production, a consequence of lipid peroxidation.90 Antioxidants like SOD, GPx, GSH, and CAT regularly eliminate these harmful active substances to protect lipids, proteins, and DNA.91 However, the equilibrium between the antioxidant and oxidation systems is harmed in an aberrant condition. There is evidence that garlic products and their associated compounds could restore the balance of oxidation and antioxidation and significantly decrease the oxidants MDA and TBARS while increasing the antioxidant agents SOD, CAT, GPx, and GSH. Normally, an inducible antioxidant program that responds to cellular stresses tightly regulates ROS levels. This program is principally regulated by the transcription factor Nrf2 and its repressor protein Keap1.92 Arellano Buendía et al. reported that allicin could appropriately regulate oxidative stress mediated by the Nrf2-Keap1 pathway.60 Besides, the Nrf2-Keap1 pathway regulated ROS production, especially through NADPH oxidase.93 Jiang et al. found that after allitride administration, the expression of NADPH oxidase subunit p22phox in the kidney tissue of diabetic rats also was significantly decreased, which reduced the level of oxidative stress in the kidney tissue of diabetic rats, thus playing a role in reducing proteinuria and protecting renal function.74 As one of the most important RNSs, NO can be produced enzymatically via NOS.94 Mong et al. reported that S-allyl cysteine and S-propyl cysteine significantly alleviate oxidative stress by inhibiting NO and NOS levels.76 Meanwhile, NO can also be transformed into nitrate, nitrogen dioxide, nitrogen trioxide, and several other RNS.95 2000 mg kg−1 d−1 aqueous garlic extract was reported to have antioxidant properties through their scavenging ability against stable metabolites of NO.62,66 Unfortunately, in our meta-analysis, garlic failed to inhibit nitrite levels.
4.2.2. Suppressing inflammatory reactions.
The failure of chronic inflammation regression is the main factor in the progress of DKD.96 Inflammatory cell infiltration and interstitial fibrosis of DKD are related to many inflammatory cytokines, such as IL-1β, IL-6, and TNF-α.97–99 Garlic preparations have been proved to significantly reduce inflammatory infiltration and IL-1β, IL-6, and TNF-α levels.59,60,62,66,76,83,84 The available research demonstrates that IL-4 and IL-10 can not only prevent non-obese diabetic mice from developing autoimmune diabetes, but also improve the inflammatory infiltration of DKD.100,101 Recent studies strongly suggested the potential of PPARα and PPARγ agonists against inflammation and their contribution to inhibiting development of DKD.102,103S-Allyl cysteine and S-propyl cysteine, according to Mong et al., restored renal PPAR-α and PPAR-γ mRNA and protein expression, further inhibiting NF-κB activation and reducing the generation of inflammatory factors.76 The NF-κB proteins are a family of transcription factors that play a critical role in inflammation.104 Meta-analysis of the results showed that garlic preparations targeting NF-κB could significantly reduce renal inflammation and improve renal function.59,76,84 In addition, the increased NF-κB and related factors and the decreased NF-κB inhibitor Iκβ also could be reversed by garlic.59,76,84
Pyroptosis, a programmed cell death dependent on caspase-1 and mediated by inflammatory corpuscles, has increasingly caught the attention of researchers in recent years.105 Thus, pyroptosis could be a promising target for DKD therapy.106,107 Only one study reported that the expressions of NLRP3, apoptosis-associated spot-like protein, caspase-1, and IL-18 in the renal tissue of the established DKD model rats were increased. After allitride treatment, the level of NLRP3, apoptosis-associated spot-like protein, Caspase-1, and IL-18 decreased and significantly attenuated pyroptosis.73
4.2.3. Delaying renal fibrosis.
Extracellular matrix (ECM) is deposited excessively in renal fibrosis, which damages normal kidney architecture and impairs renal function.108 A unique profibrotic microenvironment is produced by the recruitment of soluble factors, including TGF-β and WNTs, from the extracellular milieu by ECM proteins in the fibrogenic niche.109 TGF-β is essential to kidney fibrosis by up-regulating matrix protein synthesis and inhibiting matrix degradation.110,111 An increase in fibrosis-related factors (CTGF, α-SMA, fibronectin, vimentin, and collagen I/IV) was detected in the diabetic kidney and correlated with proteinuria, Scr, and interstitial fibrosis.112–114 Inhibition of ECM accumulation induced by TGF-β1 and its related fibrosis markers is expected to reduce extracellular deposition and prevent renal fibrosis in DKD.115 Garlic derivatives (allicin, allitride, S-methyl cysteine, and S-ethyl cysteine) significantly reversed renal fibrosis by targeting TGF-β1 and related proteins.59,60,71,72,84 In addition, after garlic administration, fibrosis-related factors (CTGF, α-SMA, fibronectin, vimentin, and collagen I/IV) were also decreased.60,68,69,71,72,84
Mitogen-activated protein kinase (ERK, c-Jun N-terminal kinase (JNK), and p38) signaling pathways have been proposed as additional downstream pathways of TGF-β that have been overactivated in several pathophysiological processes related to renal fibrosis.116,117 Allitride, S-allyl cysteine, and S-propyl cysteine could inhibit the level of p-ERK1/2.71,76 Moreover, the dietary intake of SAC and SPC suppressed expression of the ERK1/2, p38, p-p38, JNK, and p-JNK in the kidneys of diabetic mice and improved renal functions.76,80 Renal fibrosis is also tightly correlated with the activity of the Wnt/β-catenin pathway, and the Wnt receptors low-density lipoprotein receptor-related protein 5/6 (LRP5/6) are responsible for stabilizing cytoplasmic β-catenin upon activation.118,119 By regulating Wnt/β-catenin and downstream transcription factor Snail, the process of fibrosis can be delayed after S-allyl mercaptocysteine cyclodextrin inclusion compound treatment in DKD animals.84 Because of its extreme sensitivity to variations in oxygen pressure, the kidneys are particularly vulnerable to hypoxia.120,121 Chronic renal interstitial hypoxia is characteristic of late DKD, which is usually related to the coexistence of increased oxidative stress and ROS production.122,123 HIF-1α is a key regulator of cell response to hypoxia.120,124 Subsequently, HIF-1α leads to its downstream proteins, including VEGF, Epo, and Epo-R, which are closely related to the hypoxic response induced by oxidative stress.125,126 It is reported that the level of HIF-1α could be decreased by garlic and its components.60,84 In addition, the downstream VEGF also has been inhibited after garlic treatment.60,80 However, there is no significant difference in Epo and Epo-R levels between the intervention group and control group.60
4.2.4. Improving glucose metabolism.
The increase in renal glucose uptake may be related to DKD.127,128 Through a class of glucose transporters named GLUT1–GLUT14, glucose enters the majority of cells via facilitated diffusion transport.129 GLUT1 is the main transporter of glucose uptake by mesangial cells, and its functional state is the key to glucose uptake by mesangial cells.130 Increased glucose metabolic flux caused by GLUT1 overexpression in turn activates PKC and the polyol pathway. This could increase the production of advanced glycosylation end products, and diacylglycerol synthesis.131,132 Luan et al. noted that allicin can significantly reverse the abnormally increased protein expression of GLUT1 and its downstream PKC level in DKD (P < 0.01).69 In addition, Mong et al. reported that S-allyl cysteine, S-propyl cysteine, S-methyl cysteine, and S-ethyl cysteine inhibited the increased activity and expression of PKC in the kidneys of diabetic mice.76,83 In diabetic models, the expression of GLUT4 protein decreased; on the contrary, insulin receptor substrate (IRS-1) and IRS-2 protein expression increased, although the insulin level decreased partially. As a result, the findings of Mong et al. revealed that allicin has an anti-DKD mechanism that involves both an insulin secretagogue mechanism and a signaling pathway that affects GLUT4 and IRSs.76
4.3. Limitations and considerations
The scientific use of food supplements requires the utilization of data from animal research to verify the benefits of the nutrients.
Methodological issues with preclinical animal studies include a high risk of experimental bias and a limited repeatability of the results. Our analysis highlights several limitations in the existing evidence base, which future studies can build upon. First, because the risk assessment tool of the SYRCLE animal experiment is still subjective with no detailed example information under many items, which leads to the uncertainty of assessment, we cannot completely objectively evaluate the biased risk assessment. Developing a more objective animal experiment assessment tool can provide more convincing evidence for further clinical trials. At the same time, it is impossible to know the animal experiment plan, so it is of little significance to evaluate the selective results report through the consistency of the method and the results part of the article. In addition, in animal experiments, there are almost no reports on hidden grouping and blindness, and baseline data are rarely reported. Therefore, we propose enhancing the registration of animal experiments and standardization of the reporting requirements for animal experiments, so as to reduce the risk of various biases, effectively improve the quality of animal experiments and increase the reliability of research results. Secondly, some of the data used in this study were not directly obtained from experiments, but rather were obtained indirectly through the use of a data extraction tool, which may have resulted in measurement bias. Thirdly, the high heterogeneity among the BUN, Scr, 24 h urine volume, 24 h UAER and BG of this preclinical evidence could not be ignored, which may have caused the computations in this research to yield conclusions that were off from the actual value. Although we tried to find out the source of heterogeneity through preset subgroup and meta-regression analysis, it seems that the “year of publication”, “study quality score”, “region”, and “sample size” are important reasons for the heterogeneity of some results. However, the specific differences between these studies are still unclear. Fourth, animal models of diabetes usually exhibit only modest renal abnormalities. Future studies with animal models of diabetic comorbidity and control group settings for diabetic animals are warranted, and animal models of DKD that more closely replicate human disease should be developed. Fifth, the ameliorative effect of garlic on DKD seems to be related to garlic-specific components. From the available evidence, it seems that allicin, diaceto-dipropyl disulphide, garlic essential oil capsules, and garlic oil blend did not show comprehensive improvement in DKD. Surprisingly, we also discovered that the dietary regimen designed to be taken with meals or the intraperitoneal formulation was more beneficial than the oral formulation of garlic for improving DKD. Therefore, future research should focus on improving the extraction process, creating novel dosage forms, and screening the most potent components in order to maximize the therapeutic effects of garlic. Sixth, we still do not know which molecules or pathways are most crucial, despite several encouraging findings showing garlic products and their derivatives can significantly improve the aberrant status of the diabetic kidney in multiple ways. High-throughput analysis is required to identify garlic components as important targets or DKD defense mechanisms. Seventh, in the preclinical studies available, we found that there was no positive correlation between dose and effect, with the low-dose group outperforming the high-dose group in some outcome indicators. Finding the ideal dosage of garlic may be an important strategy to improve clinical benefit.
5. Conclusions
In conclusion, garlic and its derived components could significantly improve BUN, Scr, 24 h urine volume, proteinuria (24 h UP, 24 h UAER, and albumin/creatinine ratio), KI, BG, insulin, and body weight. In terms of the mechanisms underlying therapy of DKD, garlic supplements exert anti-DKD properties possibly through alleviating oxidative stress (Nrf2/Keap1 pathway and NO pathway), suppressing inflammatory reactions (NF-κB pathway and PPAR pathway), delaying renal fibrosis (MAPK pathways, Wnt/β-catenin pathway, HIF-1α pathways, and pyroptosis), and improving glucose metabolism (GLUT1/PKC pathway and GLUT4/IRSs pathway). Furthermore, positive effects were exhibited for garlic products, supplied at doses of 500 mg kg−1 with interventions lasting 8–10 weeks, and garlic components, administered at doses of 45–150 mg kg−1 with interventions lasting 4–10 weeks, based on dosage-duration analyses. However, the inclusion of articles of poor methodological quality and publication bias may have weakened the validity of the findings, therefore caution must be taken when evaluating the results. Clinical application can be challenging due to the limitations of current preclinical investigations. Therefore, large-scale, long-term and high-quality trials are needed to demonstrate the long-term tolerability and efficacy of garlic and its derivatives in modulating DKD in the future before these findings can be applied to humans.
Abbreviations
24 h | 24 hours |
α-SMA | α-Smooth muscle actin |
ACEI | Angiotensin-converting enzyme inhibitors |
ARBs | Angiotensin II receptor blockers |
BG | Blood glucose |
BMI | Body mass index |
BUN | Blood urea nitrogen |
BW | Body weight |
CAT | Catalase |
CBM | China biology medicine disc |
CI | Confidence interval |
CNKI | China National Knowledge Internet |
CTGF | Connective tissue growth factor |
DKD | Diabetic kidney disease |
ECM | Extracellular matrix |
Epo | Erythropoietin |
Epo-R | Erythropoietin receptor |
ESRD | End-stage renal disease |
FBG | Fasting blood glucose |
GLUT | Glucose transporter |
GPx | Glutathione peroxidase |
GSH | Glutathione |
HIF-1α | Hypoxia inducible factor-1α |
IL | Interleukin |
iNOS | Inducible nitric oxide synthase |
IRS | Insulin receptor substrate |
JNK | c-Jun N-terminal kinase |
Keap1 | Kelch-like erythroid cell-derived protein with CNC homology (ECH)-associated protein 1 |
KI | Kidney index |
LRP5/6 | Low density lipoprotein receptor-related protein 5/6 |
MDA | Malondialdehyde |
NADPH | Reduced nicotinamide adenine dinucleotide phosphate |
NF-κB | Nuclear factor kappa-light-chain-enhancer of activated B cells |
NLRP3 | NOD-like receptor family 3 |
NM | Not mentioned |
NO | Nitric oxide |
NOS | Nitric oxide synthase |
Nrf2 | NFE2-related factor 2 |
NS | Normal saline |
p-ERK | Phosphorylated extracellular signal-regulated kinase |
PKC | Protein kinase C |
PPAR | Peroxisome proliferator-activated receptor |
RAAS | Renin–angiotensin–aldosterone system |
RNS | Reactive nitrogen species |
ROS | Reactive oxygen species |
Scr | Serum creatinine |
SD | Standard deviation |
SD rats | Sprague-Dawley rats |
SE | Standard error |
SGLT-2i | Sodium–glucose cotransporter 2 inhibitors |
SIJ | Single intraperitoneal injection |
SMD | Standard mean difference |
SOD | Superoxidase dismutase |
T1DM | Type 1 diabetes mellitus |
T2DM | Type 2 diabetes mellitus |
TBARS | Thiobarbituric acid-reacting substances |
TGF-β1 | Transforming growth factor-β1 |
TNFα | Tumor necrosis factor α |
UAER | Urinary albumin excretion rate |
UP | Urine protein |
UV | Urine volume |
VEGF | Vascular endothelial growth factor |
VIP | VIP Information Chinese Periodical Service Platform |
Wanfang | Wanfang Data Knowledge Service Platform |
Wnt | Wingless-type MMTV integration site family |
Author contributions
Yayi Jiang, Zihan Li, and Rensong Yue designed the research. All members of the team jointly formulated a retrieval strategy. Maoyi Yang and Dawei Yan screened the literature and extracted the data. Data analysis and research quality evaluation were conducted by Guojie Liu and Caiyi Long. Yayi Jiang wrote the manuscript. Zihan Li designed graphical abstract. Rensong Yue revised the manuscript. Yayi Jiang, Zihan Li, and Rensong Yue contributed equally and are co-first authors. All authors read and approved the final manuscript.
Conflicts of interest
The authors declare that there are no conflicts of interest.
Acknowledgements
We would like to thank BioRender (https://www.biorender.com) for its help in creating the Graphical abstract. This work was supported by the National Natural Science Foundation of China [grant number 82274486] and the Sichuan Science and Technology Program [grant numbers 2022YFS0382].
References
- K. Reidy, H. M. Kang, T. Hostetter and K. Susztak, Molecular mechanisms of diabetic kidney disease, J. Clin. Invest., 2014, 124, 2333–2340 CrossRef CAS
.
- S. R. K. Seshasai, S. Kaptoge, A. Thompson, E. Di Angelantonio, P. Gao, N. Sarwar, P. H. Whincup, K. J. Mukamal, R. F. Gillum, I. Holme, I. Njølstad, A. Fletcher, P. Nilsson, S. Lewington, R. Collins, V. Gudnason, S. G. Thompson, N. Sattar, E. Selvin, F. B. Hu and J. Danesh, Diabetes mellitus, fasting glucose, and risk of cause-specific death, N. Engl. J. Med., 2011, 364, 829–841 CrossRef CAS
.
- P. H. Groop, M. C. Thomas, J. L. Moran, J. Wadèn, L. M. Thorn, V. P. Mäkinen, M. Rosengård-Bärlund, M. Saraheimo, K. Hietala, O. Heikkilä and C. Forsblom, The presence and severity of chronic kidney disease predicts all-cause mortality in type 1 diabetes, Diabetes, 2009, 58, 1651–1658 CrossRef CAS
.
- T. J. Orchard, A. M. Secrest, R. G. Miller and T. Costacou, In the absence of renal disease, 20 year mortality risk in type 1 diabetes is comparable to that of the general population: a report from the Pittsburgh Epidemiology of Diabetes Complications Study, Diabetologia, 2010, 53, 2312–2319 CrossRef CAS PubMed
.
- T. Costacou and T. J. Orchard, Cumulative Kidney Complication Risk by 50 Years of Type 1 Diabetes: The Effects of Sex, Age, and Calendar Year at Onset, Diabetes Care, 2018, 41, 426–433 CrossRef PubMed
.
- H. H. Parving, J. B. Lewis, M. Ravid, G. Remuzzi and L. G. Hunsicker, Prevalence and risk factors for microalbuminuria in a referred cohort of type II diabetic patients: a global perspective, Kidney Int., 2006, 69, 2057–2063 CrossRef
.
- R. A. Bailey, Y. Wang, V. Zhu and M. F. Rupnow, Chronic kidney disease in US adults with type 2 diabetes: an updated national estimate of prevalence based on Kidney Disease: Improving Global Outcomes (KDIGO) staging, BMC Res. Notes, 2014, 7, 415 CrossRef
.
- G. C. K. D. Collaboration, Global, regional, and national burden of chronic kidney disease, 1990–2017: a systematic analysis for the Global Burden of Disease Study 2017, Lancet, 2020, 395, 709–733 CrossRef
.
- R. Z. Alicic, M. T. Rooney and K. R. Tuttle, Diabetic Kidney Disease: Challenges, Progress, and Possibilities, Clin. J. Am. Soc. Nephrol., 2017, 12, 2032–2045 CrossRef CAS PubMed
.
- I. H. de Boer, T. C. Rue, Y. N. Hall, P. J. Heagerty, N. S. Weiss and J. Himmelfarb, Temporal trends in the prevalence of diabetic kidney disease in the United States, J. Am. Med. Assoc., 2011, 305, 2532–2539 CrossRef CAS PubMed
.
-
D. J. Magliano and E. J. Boyko, IDF Diabetes Atlas, International Diabetes Federation, Brussels, 10th edn, 2021 Search PubMed
.
- J. A. Jefferson, S. J. Shankland and R. H. Pichler, Proteinuria in diabetic kidney disease: a mechanistic viewpoint, Kidney Int., 2008, 74, 22–36 CrossRef CAS PubMed
.
- T. W. Tervaert, A. L. Mooyaart, K. Amann, A. H. Cohen, H. T. Cook, C. B. Drachenberg, F. Ferrario, A. B. Fogo, M. Haas, E. de Heer, K. Joh, L. H. Noël, J. Radhakrishnan, S. V. Seshan, I. M. Bajema and J. A. Bruijn, Pathologic classification of diabetic nephropathy, J. Am. Soc. Nephrol., 2010, 21, 556–563 CrossRef
.
- D. M. Tanase, E. M. Gosav, M. I. Anton, M. Floria, P. N. Seritean Isac, L. L. Hurjui, C. C. Tarniceriu, C. F. Costea, M. Ciocoiu and C. Rezus, Oxidative Stress and NRF2/KEAP1/ARE Pathway in Diabetic Kidney Disease (DKD): New Perspectives, Biomolecules, 2022, 12, 1227 CrossRef CAS PubMed
.
- P. H. Hung, Y. C. Hsu, T. H. Chen and C. L. Lin, Recent Advances in Diabetic Kidney Diseases: From Kidney Injury to Kidney Fibrosis, Int. J. Mol. Sci., 2021, 22, 11857 CrossRef CAS
.
- T. Vartak, C. Godson and E. Brennan, Therapeutic potential of pro-resolving mediators in diabetic kidney disease, Adv. Drug Delivery Rev., 2021, 178, 113965 CrossRef CAS
.
- M. E. Cooper, Interaction of metabolic and haemodynamic factors in mediating experimental diabetic nephropathy, Diabetologia, 2001, 44, 1957–1972 CrossRef CAS
.
- R. E. Pérez-Morales, M. D. Del Pino, J. M. Valdivielso, A. Ortiz, C. Mora-Fernández and J. F. Navarro-González, Inflammation in Diabetic Kidney Disease, Nephron, 2019, 143, 12–16 CrossRef
.
- R. Boussageon, T. Bejan-Angoulvant, M. Saadatian-Elahi, S. Lafont, C. Bergeonneau, B. Kassaï, S. Erpeldinger, J. M. Wright, F. Gueyffier and C. Cornu, Effect of intensive glucose lowering treatment on all cause mortality, cardiovascular death, and microvascular events in type 2 diabetes: meta-analysis of randomised controlled trials, Br. Med. J., 2011, 343, d4169 CrossRef PubMed
.
- Y. Tanaka, Y. Atsumi, K. Matsuoka, T. Onuma, T. Tohjima and R. Kawamori, Role of glycemic control and blood pressure in the development and progression of nephropathy in elderly Japanese NIDDM patients, Diabetes Care, 1998, 21, 116–120 CrossRef CAS
.
- C. A. Emdin, K. Rahimi, B. Neal, T. Callender, V. Perkovic and A. Patel, Blood pressure lowering in type 2 diabetes: a systematic review and meta-analysis, J. Am. Med. Assoc., 2015, 313, 603–615 CrossRef
.
- M. Kato and R. Natarajan, Epigenetics and epigenomics in diabetic kidney disease and metabolic memory, Nat. Rev. Nephrol., 2019, 15, 327–345 CrossRef
.
- C. A. Ricciardi and L. Gnudi, Kidney disease in diabetes: From mechanisms to clinical presentation and treatment strategies, Metabolism, 2021, 124, 154890 CrossRef CAS PubMed
.
- H. H. Parving, B. M. Brenner, J. J. McMurray, D. de Zeeuw, S. M. Haffner, S. D. Solomon, N. Chaturvedi, F. Persson, A. S. Desai, M. Nicolaides, A. Richard, Z. Xiang, P. Brunel and M. A. Pfeffer, Cardiorenal end points in a trial of aliskiren for type 2 diabetes, N. Engl. J. Med., 2012, 367, 2204–2213 CrossRef CAS PubMed
.
- G. L. Bakris, B. Pitt, M. R. Weir, M. W. Freeman, M. R. Mayo, D. Garza, Y. Stasiv, R. Zawadzki, L. Berman and D. A. Bushinsky, Effect of Patiromer on Serum Potassium Level in Patients With Hyperkalemia and Diabetic Kidney Disease: The AMETHYST-DN Randomized Clinical Trial, J. Am. Med. Assoc., 2015, 314, 151–161 CrossRef CAS
.
- E. M. Wright and E. Turk, The sodium/glucose cotransport family SLC5, Pflugers Arch., 2004, 447, 510–518 CrossRef CAS PubMed
.
- J. E. Gerich, Role of the kidney in normal glucose homeostasis and in the hyperglycaemia of diabetes mellitus: therapeutic implications, Diabetic Med., 2010, 27, 136–142 CrossRef CAS PubMed
.
- R. A. DeFronzo, J. A. Davidson and S. Del Prato, The role of the kidneys in glucose homeostasis: a new path towards normalizing glycaemia, Diabetes, Obes. Metab., 2012, 14, 5–14 CrossRef CAS
.
- R. Z. Alicic, E. J. Johnson and K. R. Tuttle, SGLT2 Inhibition for the Prevention and Treatment of Diabetic Kidney Disease: A Review, Am. J. Kidney Dis., 2018, 72, 267–277 CrossRef CAS
.
- A. D. Kaze, M. Zhuo, S. C. Kim, E. Patorno and J. M. Paik, Association of SGLT2 inhibitors with cardiovascular, kidney, and safety outcomes among patients with diabetic kidney disease: a meta-analysis, Cardiovasc. Diabetol., 2022, 21, 47 CrossRef CAS PubMed
.
- S. I. Taylor, J. E. Blau and K. I. Rother, SGLT2 Inhibitors May Predispose to Ketoacidosis, J. Clin. Endocrinol. Metab., 2015, 100, 2849–2852 CrossRef CAS
.
- S. Dutta, T. Kumar, S. Singh, S. Ambwani, J. Charan and S. B. Varthya, Euglycemic diabetic ketoacidosis associated with SGLT2 inhibitors: A systematic review and quantitative analysis, J. Fam. Med. Prim. Care, 2022, 11, 927–940 CrossRef
.
- J. Rosenstock and E. Ferrannini, Euglycemic Diabetic Ketoacidosis: A Predictable, Detectable, and Preventable Safety Concern With SGLT2 Inhibitors, Diabetes Care, 2015, 38, 1638–1642 CrossRef CAS
.
- P. Rossing, Diabetic nephropathy: worldwide epidemic and effects of current treatment on natural history, Curr. Diabetes Rep., 2006, 6, 479–483 CrossRef
.
- K. R. Tuttle, The landscape of diabetic kidney disease transformed, Nat. Rev. Nephrol., 2020, 16, 67–68 CrossRef
.
- D. J. Newman and G. M. Cragg, Natural Products as Sources of New Drugs from 1981 to 2014, J. Nat. Prod., 2016, 79, 629–661 CrossRef CAS
.
- A. Drogosis, C. Mamoulakis, E. Chrysos, G. Tsoucalas, S. N. Michaleas and M. Karamanou, Medicinal plants in the treatment of urinary tract malignancies during the Araboislamic period (7(th)- 14(th) century AD), Arab. J. Urol., 2022, 20, 219–223 CrossRef PubMed
.
- J. G. Dausch and D. W. Nixon, Garlic: a review of its relationship to malignant disease, Prev. Med., 1990, 19, 346–361 CrossRef CAS
.
- X. Shi, Y. Lv, C. Mao, J. Yuan, Z. Yin, X. Gao and Z. Zhang, Garlic Consumption and All-Cause Mortality among Chinese Oldest-Old Individuals: A Population-Based Cohort Study, Nutrients, 2019, 11, 1504 CrossRef CAS PubMed
.
- P. Zou, Traditional Chinese Medicine, Food Therapy, and Hypertension Control: A Narrative Review of Chinese Literature, Am. J. Chin. Med., 2016, 44, 1579–1594 CrossRef CAS
.
- M. Iranshahy, B. Javadi and A. Sahebkar, Protective effects of functional foods against Parkinson's disease: A narrative review on pharmacology, phytochemistry, and molecular mechanisms, Phytother. Res., 2022, 36, 1952–1989 CrossRef CAS
.
- M. Kumar, V. Rakesh Sharma, V. Kumar, U. Sirohi, V. Chaudhary, S. Sharma, G. Saripalli, R. K. Naresh, H. K. Yadav and S. Sharma, Genetic diversity and population structure analysis of Indian garlic (Allium sativum L.) collection using SSR markers, Physiol. Mol. Biol. Plants, 2019, 25, 377–386 CrossRef CAS PubMed
.
- A. Mondal, S. Banerjee, S. Bose, S. Mazumder, R. A. Haber, M. H. Farzaei and A. Bishayee, Garlic constituents for cancer prevention and therapy: From phytochemistry to novel formulations, Pharmacol. Res., 2022, 175, 105837 CrossRef CAS PubMed
.
- S. N. Stabler, A. M. Tejani, F. Huynh and C. Fowkes, Garlic for the prevention of cardiovascular morbidity and mortality in hypertensive patients, Cochrane Database Syst. Rev., 2012, 2012, Cd007653 Search PubMed
.
- T. Zeng, F. F. Guo, C. L. Zhang, F. Y. Song, X. L. Zhao and K. Q. Xie, A meta-analysis of randomized, double-blind, placebo-controlled trials for the effects of garlic on serum lipid profiles, J. Sci. Food Agric., 2012, 92, 1892–1902 CrossRef CAS
.
- K. Sundaram, J. Mu, A. Kumar, J. Behera, C. Lei, M. K. Sriwastva, F. Xu, G. W. Dryden, L. Zhang, S. Chen, J. Yan, X. Zhang, J. W. Park, M. L. Merchant, N. Tyagi, Y. Teng and H. G. Zhang, Garlic exosome-like nanoparticles reverse high-fat diet induced obesity via the gut/brain axis, Theranostics, 2022, 12, 1220–1246 CrossRef CAS
.
- C. T. Liu, L. Y. Sheen and C. K. Lii, Does garlic have a role as an antidiabetic agent?, Mol. Nutr. Food Res., 2007, 51, 1353–1364 CrossRef CAS
.
- S. B. Bhatwalkar, R. Mondal, S. B. N. Krishna, J. K. Adam, P. Govender and R. Anupam, Antibacterial Properties of Organosulfur Compounds of Garlic (Allium sativum), Front. Microbiol., 2021, 12, 613077 CrossRef PubMed
.
- Y. Wang, K. Wei, X. Han, D. Zhao, Y. Zheng, J. Chao, J. Gou, F. Kong and C. S. Zhang, The Antifungal Effect of Garlic Essential Oil on Phytophthora nicotianae and the Inhibitory Component Involved, Biomolecules, 2019, 9, 632 CrossRef CAS
.
- E. Lissiman, A. L. Bhasale and M. Cohen, Garlic for the common cold, Cochrane Database Syst. Rev., 2014, 2014, Cd006206 Search PubMed
.
- P. Shakib, H. Kalani, A. S. M. Ali, M. Zebardastpour, K. Moradpour, J. Ho, V. H. Nazarabad and K. Cheraghipour, In Vivo and In Vitro Anti-schistosomiasis Effect of Garlic: A Systematic Review, Curr. Drug Discovery Technol., 2022, 19, e280222201511 CrossRef CAS
.
- J. L. Sánchez-Gloria, K. M. Rada, J. G. Juárez-Rojas, L. G. Sánchez-Lozada, I. Rubio-Gayosso, F. Sánchez-Muñoz and H. Osorio-Alonso, Role of Sulfur Compounds in Garlic as Potential Therapeutic Option for Inflammation and Oxidative Stress in Asthma, Int. J. Mol. Sci., 2022, 23, 15599 CrossRef PubMed
.
- R. Arreola, S. Quintero-Fabián, R. I. López-Roa, E. O. Flores-Gutiérrez, J. P. Reyes-Grajeda, L. Carrera-Quintanar and D. Ortuño-Sahagún, Immunomodulation and anti-inflammatory effects of garlic compounds, J. Immunol. Res., 2015, 2015, 401630 Search PubMed
.
- F. Q. Alali, T. El-Elimat, L. Khalid, R. Hudaib, T. S. Al-Shehabi and A. H. Eid, Garlic for Cardiovascular Disease: Prevention or Treatment?, Curr. Pharm. Des., 2017, 23, 1028–1041 CrossRef CAS PubMed
.
- G. El-Saber Batiha, A. Magdy Beshbishy, L. G. Wasef, Y. H. A. Elewa, A. A. Al-Sagan, M. E. A. El-Hack, A. E. Taha, Y. M. Abd-Elhakim and H. P. Devkota, Chemical Constituents and Pharmacological Activities of Garlic (Allium sativum L.): A Review, Nutrients, 2020, 12, 872 CrossRef
.
- L. Melguizo-Rodríguez, E. García-Recio, C. Ruiz, E. De Luna-Bertos, R. Illescas-Montes and V. J. Costela-Ruiz, Biological properties and therapeutic applications of garlic and its components, Food Funct., 2022, 13, 2415–2426 RSC
.
- Y. Jiang, R. Yue, G. Liu, J. Liu, B. Peng, M. Yang, L. Zhao and Z. Li, Garlic (Allium sativum L.) in diabetes and its complications: Recent advances in mechanisms of action, Crit. Rev. Food Sci. Nutr., 2022, 1–51, DOI:10.1080/10408398.2022.2153793
.
- W. Yu, J. Zhang and R. Zhang, The protective effects of allcin on the kidney of diabetic rats and the study of mechanism, Chinese Journal of clinical rational drug use, 2010, 3, 7–8 Search PubMed
.
- A. S. Arellano Buendía, M. T. González, O. S. Reyes, F. E. G. Arroyo, R. A. García, E. Tapia, L. G. S. Lozada and H. O. Alonso, Immunomodulatory Effects of the Nutraceutical Garlic Derivative Allicin in the Progression of Diabetic Nephropathy, Int. J. Mol. Sci., 2018, 19, 3107 CrossRef
.
- A. S. Arellano Buendía, L. G. C. Lara, M. L. L. Mendoza, F. E. G. Arroyo, P. R. Morales, R. A. García, J. G. J. Rojas, E. Tapia, J. P. Chaverri, L. G. S. Lozada and H. O. Alonso, Effects of Allicin on Pathophysiological Mechanisms during the Progression of Nephropathy Associated to Diabetes, Antioxidants, 2020, 9, 1134 CrossRef
.
- V. Venkataiah, Vickram, K. R. Thirumalarao, V. G. Raiker and S. H. Puttaswamy, Effects of diaceto-dipropyl-disulphide on plasma sialic acid and renal tissue thiol levels in alloxan diabetic rats, J. Clin. Diagn. Res., 2016, 10, BF06–BF08 CAS
.
- N. Ziamajidi, A. Nasiri, R. Abbasalipourkabir and S. Sadeghi Moheb, Effects of garlic extract on TNF-α expression and oxidative stress status in the kidneys of rats with STZ + nicotinamide-induced diabetes, Pharm. Biol., 2017, 55, 526–531 CrossRef PubMed
.
- M. J. Page, J. E. McKenzie, P. M. Bossuyt, I. Boutron, T. C. Hoffmann, C. D. Mulrow, L. Shamseer, J. M. Tetzlaff, E. A. Akl, S. E. Brennan, R. Chou, J. Glanville, J. M. Grimshaw, A. Hróbjartsson, M. M. Lalu, T. Li, E. W. Loder, E. Mayo-Wilson, S. McDonald, L. A. McGuinness, L. A. Stewart, J. Thomas, A. C. Tricco, V. A. Welch, P. Whiting and D. Moher, The PRISMA 2020 statement: an updated guideline for reporting systematic reviews, Br. Med. J., 2021, 372, n71 CrossRef
.
- D. K. Lee, J. In and S. Lee, Standard deviation and standard error of the mean, Korean J. Anesthesiol., 2015, 68, 220–223 CrossRef
.
- M. Cumpston, T. Li, M. J. Page, J. Chandler, V. A. Welch, J. P. Higgins and J. Thomas, Updated guidance for trusted systematic reviews: a new edition of the Cochrane Handbook for Systematic Reviews of Interventions, Cochrane Database Syst. Rev., 2019, 10, Ed000142 Search PubMed
.
- N. Abolfazl, Z. Nasrin, A. Roghayeh, G. M. Taghi, S. Massoud, B. Hamid and S. A. Sara, Beneficial Effect of Aqueous Garlic Extract on Inflammation and Oxidative Stress Status in the Kidneys of Type 1 Diabetic Rats, Indian J. Clin. Biochem., 2017, 32, 329–336 CrossRef PubMed
.
- K. Al-Qattan, M. Thomson and M. Ali, Garlic (Allium sativum) and ginger (Zingiber officinale) attenuate structural nephropathy progression in streptozotocin-induced diabetic rats, e-SPEN, 2008, 3, e62–e71 CrossRef
.
- H. Luan, Y. Han, Z. Guan, X. Wang, L. Jia, X. Jia, X. Yang and M. Guo, Regulation of Allicin on Diabetic Renal Interstitial Fibrosis, Heilongjiang Medicine and Pharmacy, 2017, 40, 18–19 Search PubMed
.
- H. Luan, Y. Han, Z. Guan, Z. Ye, X. Zhang and L. Yang, Regulation of Allicin on GLUT1/PKC Pathway in Diabetic Nephropathy Rats, Chin. J. Gerontol., 2017, 37, 2347–2349 CAS
.
- N. Hfaiedh, J. C. Murat and A. Elfeki, Diabetes-Induced Damages in Rat Kidney and Brain and Protective Effectsof Natural Antioxidants, Food Nutr. Sci., 2013, 3, 436–444 Search PubMed
.
- H. Huang, Y. Jiang, F. Yuan, H. Zheng, Y. Ruan and T. Wu, Renal protective effect of allicin on rats with experimental diabetic nephropathy, Zhejiang Med. J., 2016, 38, 1557–1561 Search PubMed
.
- H. Huang, Y. Jiang, G. X. Mao, F. Yuan, H. X. Zheng, Y. Ruan and T. F. Wu, Protective effects of allicin on streptozotocin-induced diabetic nephropathy in rats, J. Sci. Food Agric., 2017, 97, 1359–1366 CrossRef CAS
.
- J. Shen, H. Zhu, F. Hu, Z. Yan, Y. Li and S. Zhong, Effect of Diallyl Trisulfide on Pyroptosis in Renal Tissue of Diabetic Nephropathy Rats, J. Guangzhou Univ. Tradit. Chin. Med., 2022, 39, 1643–1650 Search PubMed
.
- J. Jiang, X. Tang and J. Yang, Protective effects and its mechanism of garlicin on the renal function in type 1 diabetic rats, J. Clin. Nephrol., 2019, 19, 525–528 Search PubMed
.
- A. D. Mariee, G. M. Abd-Allah and M. F. El-Yamany, Renal oxidative stress and nitric oxide production in streptozotocin-induced diabetic nephropathy in rats: The possible modulatory effects of garlic (Allium sativum L.), Biotechnol. Appl. Biochem., 2009, 52, 227–232 CrossRef CAS PubMed
.
- M. C. Mong and M. C. Yin, Nuclear factor κB-dependent anti-inflammatory effects of s-allyl cysteine and s-propyl cysteine in
kidney of diabetic mice, J. Agric. Food Chem., 2012, 60, 3158–3165 CrossRef CAS PubMed
.
- M. Yuvashree, N. Ganesh Rajesh and P. Viswanathan, Potential application of nanoemulsified garlic oil blend in mitigating the progression of type 2 diabetes-mediated nephropathy in Wistar rats, 3 Biotech, 2020, 10, 272 CrossRef
.
- M. R. Kemmak and A. Gol, Preventive and protective effects of garlic on serum albumin levels and diabetic nephropathy improvement of male rats, J. Med. Plants, 2011, 10, 39–47 Search PubMed
.
- T. M. Shiju, N. G. Rajesh and P. Viswanathan, Renoprotective effect of aged garlic extract in streptozotocin-induced diabetic rats, Indian J. Pharmacol., 2013, 45, 18–23 CrossRef CAS PubMed
.
- T. M. Shiju, R. Rajkumar, N. G. Rajesh and P. Viswanathan, Aqueous extract of Allium sativum L bulbs offer nephroprotection by attenuating vascular endothelial growth factor and extracellular signal-regulated kinase-1 expression in diabetic rats, Indian J. Exp. Biol., 2013, 51, 139–148 CAS
.
- M. Thomson, K. K. Al-Qattan, J. S. Divya and M. Ali, Ameliorative actions of garlic (Allium sativum) and ginger (Zingiber officinale) on biomarkers of diabetes and diabetic nephropathy in rats: Comparison to aspirin, Int. J. Pharmacol., 2013, 9, 501–512 CAS
.
- W. Yu, The protective effects of allicin on the kidney of diabetic rats and the study of mechanism Master Degree, Hebei Medical University, 2008 Search PubMed
.
- M. C. Yin, C. C. Hsu, P. F. Chiang and W. J. Wu, Antiinflammatory and antifibrogenic effects of s-ethyl cysteine and s-methyl cysteine in the kidney of diabetic mice, Mol. Nutr. Food Res., 2007, 51, 572–579 CrossRef CAS
.
-
Y. Zhou, Preparation and preliminary pharmacological evaluation of S-allylmercaptocysteine cyclodextrin complex for the treatment of diabetic nephropathy, Master Degree, Shandong University, 2021
.
- Y. Zhang, B. Li, Y. Liu, W. Gao, K. Chen, A. Wang, X. Tang, L. Yan, Z. Luo, G. Qin, L. Chen, Q. Wan, Z. Gao, W. Wang, G. Ning and Y. Mu, Association between metabolic phenotype and urinary albumin-creatinine ratio in Chinese community adults: A cross-sectional study, J. Diabetes, 2022, 14, 541–550 CrossRef CAS
.
- Y. E. Lin, M. H. Lin, T. Y. Yeh, Y. S. Lai, K. H. Lu, H. S. Huang, F. C. Peng, S. H. Liu and L. Y. Sheen, Genotoxicity and 28-day repeated dose oral toxicity study of garlic essential oil in mice, J. Tradit. Complementary Med., 2022, 12, 536–544 CrossRef CAS
.
- O. Yamato, T. Tsuneyoshi, M. Ushijima, H. Jikihara and A. Yabuki, Safety and efficacy of aged garlic extract in dogs: upregulation of the nuclear factor erythroid 2-related factor 2 (Nrf2) signaling pathway and Nrf2-regulated phase II antioxidant enzymes, BMC Vet. Res., 2018, 14, 373 CrossRef CAS PubMed
.
- Y. C. Lin, Y. H. Chang, S. Y. Yang, K. D. Wu and T. S. Chu, Update of pathophysiology and management of diabetic kidney disease, J. Formosan Med. Assoc., 2018, 117, 662–675 CrossRef CAS PubMed
.
- S. S. Ali, H. Ahsan, M. K. Zia, T. Siddiqui and F. H. Khan, Understanding oxidants and antioxidants: Classical team with new players, J. Food Biochem., 2020, 44, e13145 Search PubMed
.
- N. Kashihara, Y. Haruna, V. K. Kondeti and Y. S. Kanwar, Oxidative stress in diabetic nephropathy, Curr. Med. Chem., 2010, 17, 4256–4269 CrossRef CAS PubMed
.
- M. Wang, X. Zhang, W. Jia, C. Zhang, T. Boczek, M. Harding, Y. Liu, M. Li, S. Zhang, S. Lei, D. Zhang and F. Guo, Circulating glutathione peroxidase and superoxide dismutase levels in patients with epilepsy: A meta-analysis, Seizure, 2021, 91, 278–286 CrossRef PubMed
.
- S. Sajadimajd and M. Khazaei, Oxidative Stress and Cancer: The Role of Nrf2, Curr. Cancer Drug Targets, 2018, 18, 538–557 CrossRef CAS
.
- I. Bellezza, I. Giambanco, A. Minelli and R. Donato, Nrf2-Keap1 signaling in oxidative and reductive stress, Biochim. Biophys. Acta, Mol. Cell Res., 2018, 1865, 721–733 CrossRef CAS PubMed
.
- P. J. Andrew and B. Mayer, Enzymatic function of nitric oxide synthases, Cardiovasc. Res., 1999, 43, 521–531 CrossRef CAS PubMed
.
- L. Lamattina, C. García-Mata, M. Graziano and G. Pagnussat, Nitric oxide: the versatility of an extensive signal molecule, Annu. Rev. Plant Biol., 2003, 54, 109–136 CrossRef CAS PubMed
.
- J. Wada and H. Makino, Inflammation and the pathogenesis of diabetic nephropathy, Clin. Sci., 2013, 124, 139–152 CrossRef CAS PubMed
.
- Y. Li, J. Liu, G. Liao, J. Zhang, Y. Chen, L. Li, L. Li, F. Liu, B. Chen, G. Guo, C. Wang, L. Yang, J. Cheng and Y. Lu, Early intervention with mesenchymal stem cells prevents nephropathy in diabetic rats by ameliorating the inflammatory microenvironment, Int. J. Mol. Med., 2018, 41, 2629–2639 CAS
.
- Y. Bai, J. Wang, Z. He, M. Yang, L. Li and H. Jiang, Mesenchymal Stem Cells Reverse Diabetic Nephropathy Disease via Lipoxin A4 by Targeting Transforming Growth Factor β (TGF-β)/smad Pathway and Pro-Inflammatory Cytokines, Med. Sci. Monit., 2019, 25, 3069–3076 CrossRef CAS PubMed
.
- H. Yaribeygi, S. L. Atkin and A. Sahebkar, Interleukin-18 and diabetic nephropathy: A review, J. Cell Physiol., 2019, 234, 5674–5682 CrossRef CAS PubMed
.
- K. S. Ko, M. Lee, J. J. Koh and S. W. Kim, Combined administration of plasmids encoding IL-4 and IL-10 prevents the development of autoimmune diabetes in nonobese diabetic mice, Mol. Ther., 2001, 4, 313–316 CrossRef CAS PubMed
.
- B. Masola, O. O. Oguntibeju and A. B. Oyenihi, Centella asiatica ameliorates diabetes-induced stress in rat tissues via influences on antioxidants and inflammatory cytokines, Biomed. Pharmacother., 2018, 101, 447–457 CrossRef CAS PubMed
.
- A. C. Calkin, S. Giunti, K. A. Jandeleit-Dahm, T. J. Allen, M. E. Cooper and M. C. Thomas, PPAR-alpha and -gamma agonists attenuate diabetic kidney disease in the apolipoprotein E knockout mouse, Nephrol., Dial., Transplant., 2006, 21, 2399–2405 CrossRef CAS PubMed
.
- P. Balakumar, S. Kadian and N. Mahadevan, Are PPAR alpha agonists a rational therapeutic strategy for preventing abnormalities of the diabetic kidney?, Pharmacol. Res., 2012, 65, 430–436 CrossRef CAS PubMed
.
- M. J. Morgan and Z. G. Liu, Crosstalk of reactive oxygen species and NF-κB signaling, Cell Res., 2011, 21, 103–115 CrossRef CAS
.
- P. Liu, Z. Zhang and Y. Li, Relevance of the Pyroptosis-Related Inflammasome Pathway in the Pathogenesis of Diabetic Kidney Disease, Front. Immunol., 2021, 12, 603416 CrossRef CAS
.
- Z. Cao, D. Huang, C. Tang, Y. Lu, S. Huang, C. Peng and X. Hu, Pyroptosis in diabetes and diabetic nephropathy, Clin. Chim. Acta, 2022, 531, 188–196 CrossRef CAS
.
- A. Al Mamun, A. Ara Mimi, Y. Wu, M. Zaeem, M. Abdul Aziz, S. Aktar Suchi, E. Alyafeai, F. Munir and J. Xiao, Pyroptosis in diabetic nephropathy, Clin. Chim. Acta, 2021, 523, 131–143 CrossRef CAS PubMed
.
- Q. Yuan, R. J. Tan and Y. Liu, Myofibroblast in Kidney Fibrosis: Origin, Activation, and Regulation, Adv. Exp. Med. Biol., 2019, 1165, 253–283 CrossRef CAS PubMed
.
- L. Li, H. Fu and Y. Liu, The fibrogenic niche in kidney fibrosis: components and mechanisms, Nat. Rev. Nephrol., 2022, 18, 545–557 CrossRef CAS PubMed
.
- R. Mishra, L. Zhu, R. L. Eckert and M. S. Simonson, TGF-beta-regulated collagen type I accumulation: role of Src-based signals, Am. J. Physiol.: Cell Physiol., 2007, 292, C1361–C1369 CrossRef CAS PubMed
.
- Y. Isaka, Targeting TGF-β Signaling in Kidney Fibrosis, Int. J. Mol. Sci., 2018, 19, 2532 CrossRef PubMed
.
- N. Chen, J. Hao, L. Li, F. Li, S. Liu and H. Duan, Carboxy-terminal modulator protein attenuated extracellular matrix deposit by inhibiting phospho-Akt, TGF-β1 and α-SMA in kidneys of diabetic mice, Biochem. Biophys. Res. Commun., 2016, 474, 753–760 CrossRef CAS PubMed
.
- G. Tang, S. Li, C. Zhang, H. Chen, N. Wang and Y. Feng, Clinical efficacies, underlying mechanisms and molecular targets of Chinese medicines for diabetic nephropathy treatment and management, Acta Pharm. Sin. B, 2021, 11, 2749–2767 CrossRef CAS PubMed
.
- S. Wang, B. Li, C. Li, W. Cui and L. Miao, Potential Renoprotective Agents through Inhibiting CTGF/CCN2 in Diabetic Nephropathy, J. Diabetes Res., 2015, 2015, 962383 Search PubMed
.
- L. H. Wang, J. S. Liu, W. B. Ning, Q. J. Yuan, F. F. Zhang, Z. Z. Peng, M. M. Lu, R. N. Luo, X. Fu, G. Y. Hu, Z. H. Wang and L. J. Tao, Fluorofenidone attenuates diabetic nephropathy and kidney fibrosis in db/db mice, Pharmacology, 2011, 88, 88–99 CrossRef CAS PubMed
.
- T. W. Hung, J. P. Tsai, S. H. Lin, C. H. Lee, Y. H. Hsieh and H. R. Chang, Pentraxin 3 Activates JNK Signaling and Regulates the Epithelial-To-Mesenchymal Transition in Renal Fibrosis, Cell. Physiol. Biochem., 2016, 40, 1029–1038 CrossRef CAS PubMed
.
- H. Watanabe, M. P. de Caestecker and Y. Yamada, Transcriptional cross-talk between Smad, ERK1/2, and p38 mitogen-activated protein kinase pathways regulates transforming growth factor-beta-induced aggrecan gene expression in chondrogenic ATDC5 cells, J. Biol. Chem., 2001, 276, 14466–14473 CrossRef CAS PubMed
.
- Y. Feng, J. Ren, Y. Gui, W. Wei, B. Shu, Q. Lu, X. Xue, X. Sun, W. He, J. Yang and C. Dai, Wnt/β-Catenin-Promoted Macrophage Alternative Activation Contributes to Kidney Fibrosis, J. Am. Soc. Nephrol., 2018, 29, 182–193 CrossRef CAS PubMed
.
- B. T. MacDonald and X. He, Frizzled and LRP5/6 receptors for Wnt/β-catenin signaling, Cold Spring Harbor Perspect. Biol., 2012, 4, 1–23 CAS
.
- B. Wang, Z. L. Li, Y. L. Zhang, Y. Wen, Y. M. Gao and B. C. Liu, Hypoxia and chronic kidney disease, EBioMedicine, 2022, 77, 103942 CrossRef CAS PubMed
.
- Y. Liu, Cellular and molecular mechanisms of renal fibrosis, Nat. Rev. Nephrol., 2011, 7, 684–696 CrossRef CAS
.
- M. Packer, Mechanisms Leading to Differential Hypoxia-Inducible Factor Signaling in the Diabetic Kidney: Modulation by SGLT2 Inhibitors and Hypoxia Mimetics, Am. J. Kidney Dis., 2021, 77, 280–286 CrossRef CAS PubMed
.
- S. Y. Pan, P. Z. Tsai, Y. H. Chou, Y. T. Chang, F. C. Chang, Y. L. Chiu, W. C. Chiang, T. Hsu, Y. M. Chen, T. S. Chu and S. L. Lin, Kidney pericyte hypoxia-inducible factor regulates erythropoiesis but not kidney fibrosis, Kidney Int., 2021, 99, 1354–1368 CrossRef CAS PubMed
.
- S. Shu, Y. Wang, M. Zheng, Z. Liu, J. Cai, C. Tang and Z. Dong, Hypoxia and Hypoxia-Inducible Factors in Kidney Injury and Repair, Cells, 2019, 8, 207 CrossRef CAS PubMed
.
- P. A. Campochiaro, Molecular pathogenesis of retinal and choroidal vascular diseases, Prog. Retinal Eye Res., 2015, 49, 67–81 CrossRef CAS PubMed
.
- W. Liu, W. Zhang, T. Wang, J. Wu, X. Zhong, K. Gao, Y. Liu, X. He, Y. Zhou, H. Wang and H. Zeng, Obstructive sleep apnea syndrome promotes the progression of aortic dissection
via a ROS- HIF-1α-MMPs associated pathway, Int. J. Biol. Sci., 2019, 15, 2774–2782 CrossRef PubMed
.
- A. Mitrakou, Kidney: its impact on glucose homeostasis and hormonal regulation, Diabetes Res. Clin. Pract., 2011, 93(Suppl 1), S66–S72 CrossRef CAS PubMed
.
- R. C. Stanton, Role of Glucose Metabolism and Mitochondrial Function in Diabetic Kidney Disease, Curr. Diabetes Rep., 2021, 21, 6 CrossRef CAS PubMed
.
- D. Deng and N. Yan, GLUT, SGLT, and SWEET: Structural and mechanistic investigations of the glucose transporters, Protein Sci., 2016, 25, 546–558 CrossRef CAS PubMed
.
- V. Vallon, Glucose transporters in the kidney in health and disease, Pflugers Arch., 2020, 472, 1345–1370 CrossRef CAS PubMed
.
- F. C. Brosius and C. W. Heilig, Glucose transporters in diabetic nephropathy, Pediatr. Nephrol., 2005, 20, 447–451 CrossRef PubMed
.
- F. P. Schena and L. Gesualdo, Pathogenetic mechanisms of diabetic nephropathy, J. Am. Soc. Nephrol., 2005, 16(Suppl 1), S30–S33 CrossRef CAS PubMed
.
|
This journal is © The Royal Society of Chemistry 2024 |
Click here to see how this site uses Cookies. View our privacy policy here.