DOI:
10.1039/D3FO03464J
(Paper)
Food Funct., 2024,
15, 284-294
Betanin protects against bleomycin-induced pulmonary fibrosis by regulating the NLRP3/IL-1β/TGF-β1 pathway-mediated epithelial-to-mesenchymal transition†
Received
19th August 2023
, Accepted 27th November 2023
First published on 12th December 2023
Abstract
Idiopathic pulmonary fibrosis (IPF) is a life-threatening disease that leads to dyspnea and progressive loss of lung function. This study aimed to investigate the protective effect of betanin (BET), the major pigment in red beetroot, on pulmonary fibrosis induced by bleomycin (BLM) in rats and to assess the underlying mechanisms. In this view, total and differential cell counts and LDH activity in bronchoalveolar lavage fluid were estimated. Furthermore, MDA and GSH contents in the lungs were colorimetrically measured, while hydroxyproline, NLRP3, ASC, caspase-1, TGF-β1, and vimentin levels in lung tissue were evaluated using the ELISA technique. Moreover, IL-1β, E-cadherin, and α-SMA expressions were analyzed by immunostaining of lung specimens. BET treatment protects against pulmonary fibrosis as indicated by the reduction in total and differential cell counts, LDH activity, hydroxyproline, NLRP3, ASC, caspase-1, IL-1β, and TGF-β1 levels. MDA content was also decreased following BET administration, while GSH content was elevated. Additionally, BET suppressed the EMT process as evidenced by an increase in E-cadherin expression besides the reduction in vimentin and α-SMA expressions. To conclude, these results revealed the protective effect of BET against pulmonary fibrosis that might be attributed to the attenuation of the NLRP3/IL-1β/TGF-β1 signaling pathway and EMT process.
1. Introduction
Pulmonary fibrosis is a chronic and irreversible lung disease with restricted therapeutic regimens and therefore advances in elucidating the pathophysiology as well as exploring new therapeutic interventions are urgently needed.1 Among the pulmonary fibrotic disorders, idiopathic pulmonary fibrosis (IPF) is acknowledged to exhibit the most severe manifestations with the poorest clinical outcomes.2 IPF is a specific form of interstitial pneumonia that results in progressive and irreversible scarring of the lung and death from respiratory failure. Its prevalence is increasing globally and incidence is rising with age.3
Increasing evidence suggests that the epithelial–mesenchymal transition (EMT) plays a crucial role in IPF development.4,5 EMT is a pathophysiological process that triggers the apoptotic transformation of alveolar epithelial cells, the accumulation of fibroblasts/myofibroblasts, and the deposition of extracellular matrix in response to the abnormal activation of several pathways that favors the development of excessive fibrosis.3 In other words, it is a process in which epithelial cells lose their characteristics with downregulated epithelial markers, such as E-cadherin, while gaining mesenchymal features with upregulated mesenchymal markers, such as α-smooth muscle actin (α-SMA) and vimentin.6
Pathogens, irritants, or dead cells can activate the inflammasome, which is a protein complex comprising sensor, adaptor, and effector proteins.7 Upon activation, it stimulates caspase-1 maturation, which in turn triggers the release of interleukin-1β (IL-1β) to mediate immuno-inflammatory responses.8 Many studies revealed a significant increase in the levels of NLRP3 (NOD-like receptor family, pyrin domain-containing protein 3), caspase-1, and IL-1β in IPF patients.9 Moreover, it is suggested that NLRP3 can regulate EMT by transforming growth factor-β1 (TGF-β1) signaling in hepatic and renal fibrosis.10,11 However, a better understanding of the role of NLRP3 in lung fibrosis development by targeting EMT and exploring therapeutics to prevent fibrosis require further studies.
Bleomycin (BLM) is an anticancer antibiotic used in cancer treatment.12 Even though there is no experimental model mimicking all characteristics of human diseases, BLM-induced lung fibrosis in rats is widely used to investigate pathophysiological mechanisms of IPF and to investigate potential therapeutic targets.13 BLM can induce the best-characterized model because of its reproducibility, ease of induction, and representation of most aspects of the IPF features.14 Generation of the reactive oxygen species (ROS), triggering of inflammatory and fibro-proliferative responses, initiation of DNA damage, diminution of endogenous antioxidants, and aggravation of ROS-mediated tissue injury are considered pathogenic mechanisms behind BLM-induced pulmonary fibrosis.15
Bioactive food components have received great scientific attention because of their beneficial health effects including decreasing inflammation, scavenging free radicals, and regulating different cell signaling pathways.16 Betanin (BET) is a heterocyclic compound most abundant in beetroot (Beta vulgaris L.), conferring its red-violet coloring. It gained popularity for use as a natural colorant in the food industry.17 Previous studies have revealed that BET has anti-diabetic, antioxidant, and anti-inflammatory properties.18,19
To the best of our knowledge, the ability of BET to manage pulmonary fibrosis has not yet been studied. Thus, this study was aimed at investigating the potential protective effect of BET in ameliorating BLM-induced pulmonary fibrosis and highlighting the underlying mechanism implicated in BET's anti-fibrotic effect.
2. Materials and methods
2.1. Drugs and chemicals
BET was purchased from Sigma-Aldrich (St Louis, MO, USA). BLM was supplied in the form of a pharmaceutical product (Bleocel®; 15 mg per vial, Cell Pharm GmbH, Bad Vilbel, Germany). Other chemicals and the feeding ingredients were of high quality and analytical grades.
2.2. Animals
Thirty male Sprague–Dawley rats (200 ± 20 g) were obtained from the Egyptian Organization for Biological Products and Vaccines (Giza, Egypt) and allowed to acclimatize for two weeks under standard laboratory conditions (controlled room temperature (25 ± 1 °C), 12 h light/dark cycle, with free access to water and food). The research protocol was approved by the Animal Care and Use Committee of Mansoura University (MU-ACUC), Egypt (Ethical code: PHARM.R.23.03.8), in accordance with “Guide for the Care and Use of Laboratory Animals” (National Research Council publication 8th ed, USA, 2011).
2.3. Induction of pulmonary fibrosis
Pulmonary fibrosis was induced as previously described by Zaghloul et al.20 Rats were anesthetized using thiopental sodium (i.p., 20 mg kg−1). A middle neck incision was made, the trachea was exposed, and BLM (5 mg kg−1) was slowly infused into the trachea using a 26-gauge needle. Rats were maintained at their dorsal side and repetitively swiveled to ensure uniform spread of BLM into the lungs. All groups were subjected to the same procedures except normal control, which instead underwent intratracheal instillation of normal saline. This was followed by surgical suturing and topical application of sodium fusidate (2%).
2.4. Experimental design and sample collection
Rats were randomly divided into 3 groups (10 rats each) as follows; normal control group, BLM group: pulmonary fibrosis was induced as previously mentioned in section 2.3, and BLM + BET group: rats orally received BET 25 mg kg−1 day−1 for 3 days before BLM instillation to the 28th day21,22 (Fig. 1).
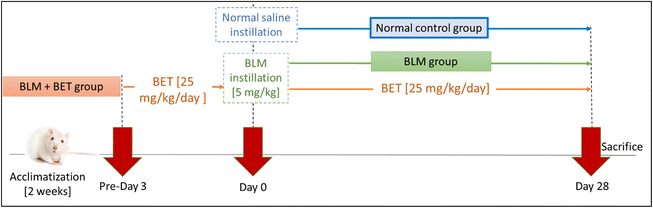 |
| Fig. 1 Experimental design. BET: betanin, BLM: bleomycin. | |
At the end of the study, rats were sacrificed under deep thiopental sodium anesthesia (i.p., 40 mg kg−1). Both lobes of the lungs were excised and weighed to calculate the lung/body weight index. The left lobes were fixed in 10% buffered formalin for histopathological and immunohistochemical analyses. The right lobes were snap-frozen in liquid nitrogen and stored at −80 °C for further biochemical analyses.
2.5. Collection of bronchoalveolar lavage fluid (BALF)
BALF was collected as previously described by Zaghloul et al.20 Briefly, the exposed tracheas were cannulated, and the lungs were infused with 0.9% ice-cold sterile saline (2 ml for 3 times). Lungs were gently squeezed to guarantee efficient recovery of the infused solution. The collected BALF (50–70% yield) was centrifuged for 10 min at 2000 rpm, 4 °C. To quantify the lung inflammatory cells, the cell sediments were re-suspended in sterile saline. BALF's LDH activity was assessed using a commercially available kit supplied by Human Diagnostics (Wiesbaden, Germany), following the manufacturer's instructions.
2.6. Histopathological and immunohistochemical analyses
Formalin-fixed pulmonary tissues were embedded in paraffin wax. 5 μm thickness sections were cut for further processing and staining with hematoxylin and eosin (H&E) and Masson's trichrome. Inflammation score was assessed in the H&E slides according to the following criteria: 0, no inflammation; 1, marginal inflammatory-cell infiltration; 2, mild inflammatory-cell infiltration; 3, moderate inflammatory-cell infiltration; 4, severe inflammatory-cell infiltration; and 5, very extreme inflammatory-cell infiltration.23
Sections of 4 μm thickness were mounted on slides and deparaffinized with xylene and ethanol solutions of different grades. Citrate buffer (pH = 6) was added for antigen retrieval and slides were blocked using 1% bovine serum albumin. Following blocking, slides were incubated at 4 °C overnight with primary antibodies for α-SMA (ab7817), IL-1β (ab283818), and E-cadherin (ab231303) supplied by Abcam, Inc. (Cambridge, UK). Slides were washed three times and incubated with a species-matched secondary antibody that was added for 1 h at room temperature. The reaction was visualized using an ABC system. Sections were counterstained using Mayer's hematoxylin and dibutylphthalate polystyrene xylene was used as a mounting medium.
Slides were blindly observed and photographed using a MIC-W16 digital camera installed on a MEIJI MX5200L microscope, using 20× and 40× objectives. The resulting 20× images were analyzed on Intel® core I7® based computer using Fiji ImageJ (version 1.51r; NIH, Maryland, USA) software. For measuring the staining surface area, the color deconvolution 2 plugin was used. Five random fields from each tissue specimen were analyzed.
2.7. Preparation of lung tissue homogenate
Lung tissues were homogenized in ice-cold phosphate buffered saline pH 7.4 and then centrifuged for 20 min at 8000 rpm, 4 °C. The supernatant was separated for further use in biochemical analyses.
2.8. Biochemical assays
Lung tissue homogenates were assessed for malondialdehyde (MDA) and glutathione (GSH) spectrophotometrically using commercially available kits provided by Biodiagnostic (Giza, Egypt) for each parameter following the manufacturer's instructions. Also, the lung tissue content of hydroxyproline, NLRP3, apoptosis-associated speck-like protein containing a CARD (ASC), vimentin, caspase-1, and TGF-β1 were assessed using rat ELISA kits provided by CUSABIO (CSB-E08838r, Houston, USA), Aviva Systems Biology Corporation (#KD0290, San Diego, USA), antibodies-online GmbH (#ABIN5665033, Aachen, Germany), Lifespan Biosciences, Inc. (#LSF15721, Washington, USA), BioVision (# E4594-100, California, USA), and Cloud-Clone Corp (#SEA124Ra, TX, USA), respectively. Absorbance was measured using the BioTek ELISA Reader (Winooski, USA).
2.9. Statistical analysis
Data were expressed as the mean ± standard error of the mean (SEM). Parametric data were analyzed by the one-way analysis of variance (ANOVA) followed by Tukey's Post-hoc test. Non-parametric data that failed the Shapiro–Wilk normality test were analyzed by the Kruskal–Wallis test followed by the Dunn's post hoc test. p values of less than 0.05 were considered statistically significant. Statistical analyses and Graphs were plotted using GraphPad Prism version 8.0.0 (Graph Pad Software Inc., SanDiego, USA).
3. Results
3.1. Effect of BET on total and differential cell counts in BALF
BLM group revealed a significant elevation in total cell count, neutrophils, and lymphocytes in BALF by 4.3-, 5.9-, and 1.7-fold, respectively as compared to the normal control group. However, rats receiving 25 mg kg−1 BET exhibited a significant decrease in total cell count, neutrophils, and lymphocytes in BALF by 2.5-, 2.6-, and 1.6-fold, respectively, compared with rats from the BLM group (Table 1).
Table 1 The effect of betanin (BET) on total and differential cell counts in BALF
Groups |
Total cell count (cell per mm3) × 106 |
Neutrophils (cell per mm3) × 106 |
Lymphocytes (cell per mm3) × 106 |
BALF: bronchoalveolar lavage fluid, BLM: bleomycin. Data are presented as mean ± SEM. (n = 6). #P < 0.05 against the normal control group and * P < 0.05 against the BLM group. |
Normal control |
0.44 ± 0.02 |
0.26 ± 0.01 |
0.13 ± 0.01 |
BLM |
1.88 ± 0.02# |
1.54 ± 0.02# |
0.23 ± 0.01# |
BLM + BET |
0.75 ± 0.02# * |
0.59 ± 0.02# * |
0.14 ± 0.00* |
3.2. Effect of BET on LDH activity in BALF
As shown in (Fig. 2a), LDH activity in BALF markedly increased by about 5.3-fold in the BLM group, compared with the normal control group, while oral administration of BET was accompanied by a significant diminution in LDH activity by 2.5-fold, compared to the BLM group.
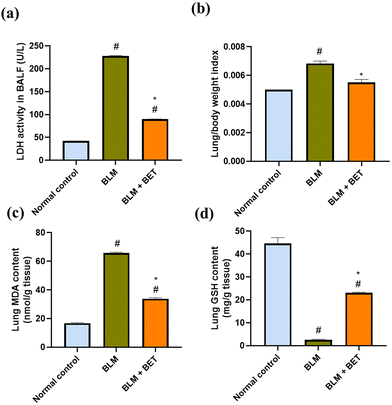 |
| Fig. 2 The effect of betanin (BET) on LDH activity in BALF (a), lung/body weight index (b), lung MDA content (c) and lung GSH content (d). BALF: bronchoalveolar lavage fluid, BLM: bleomycin. Data are presented as mean ± SEM. (n = 6). #P < 0.05 against normal control group and * P < 0.05 against BLM group. | |
3.3. Effect of BET on the lung/body weight index
The rats from the BLM group showed a notable elevation in the lung/body weight index by 36% as compared to those from the normal control group. BET treatment caused a substantial reduction in lung/body weight index by approximately 19.1% as compared to BLM-instilled rats (Fig. 2b). Moreover, no significant difference was observed between the BET group and the normal control group.
3.4. Effect of BET on MDA and GSH contents in the lung
As shown in (Fig. 2c and d), BLM instillation in rats revealed a significant augmentation in MDA content in the lung by about 3.9-fold and a substantial reduction in the lung GSH content by nearly 17.2-fold as compared to that in the normal control group. On the contrary, the BLM + BET group showed a significant attenuation in lung MDA content by 1.9-fold and a remarkable elevation in lung GSH content by 9-fold as compared to the BLM group.
3.5. Effect of BET on histopathological examination of lung tissues
H&E stained lung specimens showed intact lung architecture with no evidence of edema, inflammation, emphysema, or fibrosis in the normal control rats. The BLM-induced group revealed areas of increased thickening of alveolar septa, inflammatory cell infiltration in alveolar walls, obliteration of air spaces, and fibroplasia. On the other hand, the administration of 25 mg kg−1 BET showed a mild to moderate degree of septal thickening with few inflammatory cell infiltration, and fibroplasia (Fig. 3a).
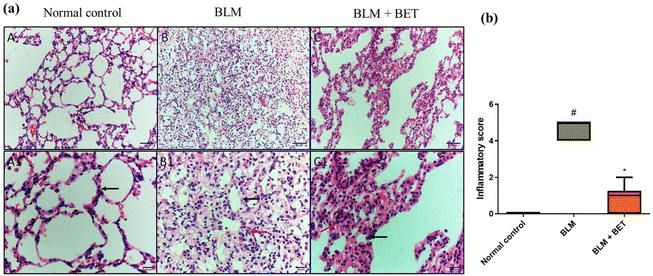 |
| Fig. 3 The effect of betanin (BET) on histopathological examination of lung tissues. (a) The microscopy pictures of lung sections stained with hematoxylin and eosin (H&E). The black arrow indicates alveolar walls. The red arrow indicates inflammatory cells infiltration. (A, B and C) in upper row (X: 200, bar 50). (A1, B1, and C1) in lower row (X: 400, bar 20). (b) Inflammatory score. Data are presented as median. (n = 6). BLM: bleomycin. #P < 0.05 against the normal control group and * P < 0.05 against the BLM group. | |
Moreover, the inflammation score significantly increased in the BLM group as compared to that in the normal control group, while the BLM + BET group revealed a significant reduction in inflammation score as compared to the BLM group (Fig. 3b).
3.6. Effect of BET on collagen fiber deposition in lung tissues
Cross-sectional images of the lung tissues stained with Masson's trichrome showed minimal blue staining mostly in the wall of the blood vessels indicating no collagen deposition in interstitium and alveolar septae in normal rats. The intratracheal instillation of BLM revealed intense bluish discoloration of collagen deposition at interstitial spaces. Contrariwise, treatment with BET caused mild to moderate bluish discoloration of collagen deposition at interstitial spaces (Fig. 4a).
 |
| Fig. 4 The effect of betanin (BET) on collagen fiber deposition in lung tissues. (a) The microscopy pictures of lung sections stained with Masson's trichrome. The black arrow indicates collagen deposition at interstitial spaces. (A, B, and C) in upper row (X: 200, bar 50). (A1, B1, and C1) in lower row (X: 400, bar 20). (b) Collagen fiber area%. (c) Hydroxyproline level. Data are presented as mean ± SEM. (n = 6). BLM: bleomycin. #P < 0.05 against normal control group and * P < 0.05 against BLM group. | |
Additionally, the BLM group revealed a marked increase in the percentage area of collagen fiber deposition by 2.1-fold, as compared to the normal control group, while treatment with BET caused a significant decrease in collagen fiber area percentage in comparison with BLM-induced rats. The BET treatment was able to restore collagen fiber area percentage to normal levels (Fig. 4b).
3.7. Effect of BET on the hydroxyproline level in lung tissues
The BLM instillation in rats revealed a substantial elevation in the lung hydroxyproline level by approximately 2.9-fold as compared to the normal control rats. In contrast, administration of BET caused a significant reduction in the hydroxyproline level by 1.8 folds in the lung in comparison with the BLM group (Fig. 4c).
3.8. Effect of BET on lung NLRP3, ASC, and caspase-1 levels
As shown in (Fig. 5a, b, and c), the BLM group showed remarkable elevations by approximately 3.4-, 4.1-, and 1.9-fold in NLRP3, ASC, and caspase-1 levels in the lung, respectively, as compared to those in the normal control group. After the BET administration, lung NLRP3, ASC, and caspase-1 levels decreased notably by approximately 1.7-, 2-, and 1.5-fold, respectively, in comparison to those in the BLM group.
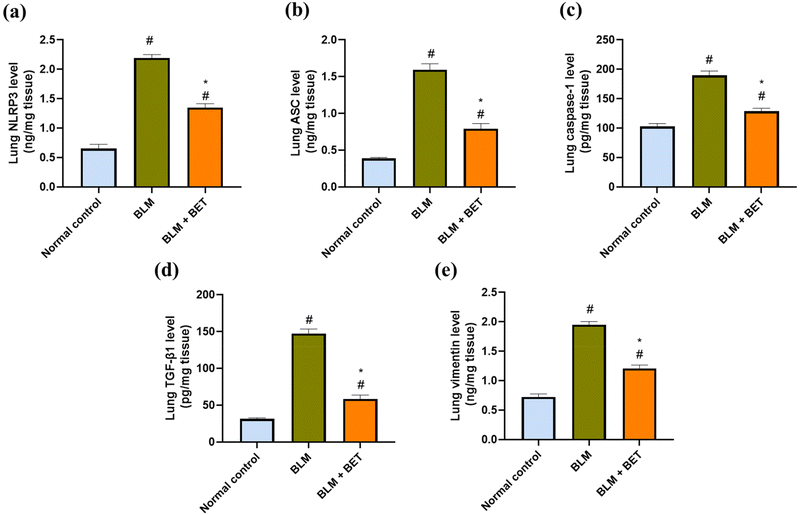 |
| Fig. 5 The effect of betanin (BET) on lung NLRP3 (a), ASC (b), caspase-1 (c), TGF-β1 (d) and vimentin (e) levels. ASC: apoptosis-associated speck-like protein containing a CARD, BLM: bleomycin, NLRP3: NOD-like receptor family, pyrin domain-containing protein 3, TGF-β1: transforming growth factor-β1. Data are presented as mean ± SEM. (n = 6). #P < 0.05 against normal control group and * P < 0.05 against BLM group. | |
3.9. Effect of BET on lung TGF-β1 and vimentin levels
Lung TGF-β1 and vimentin levels significantly increased in the BLM group by 4.7- and 2.7-fold, respectively, in comparison to those in the normal control group. Conversely, administration of BET exhibited a remarkable attenuation in TGF-β1 and vimentin levels in the lung by 60.3% and 38.5%, respectively as compared to that in the BLM group (Fig. 5d and e).
3.10. Effect of BET on lung IL-1β, E-cadherin, and α-SMA expression
The examination of immunostained lung specimens from the BLM group revealed a substantial increment in the percentage area immunostained with IL-1β by approximately 5.8-fold as compared to that in the normal control group. Surprisingly, daily oral administration of BET exhibited approximately 52.3% marked decrease in the percentage area immunostained with IL-1β in the lung as compared to that in the BLM group (Fig. 6a and b).
 |
| Fig. 6 The effect of betanin (BET) on lung IL-1β, E-cadherin, and α-SMA expression. (a) The microscopy pictures of immunostained-lung sections against IL-1β, E-cadherin and α-SMA. (b, c, and d) The % area of lung sections stained with anti-IL-1β, anti-E-cadherin, and anti-α-SMA antibodies, (X: 200, bar 50). BLM: bleomycin, IL-1β: interleukin-1β. α-SMA: α-smooth muscle actin. Data are presented as mean ± SEM. (n = 6). #P < 0.05 against normal control group and * P < 0.05 against BLM group. | |
BLM instillation in rats showed a significant reduction in the percentage area of lung E-cadherin immunostaining by about 5.2-fold as well as a significant increase in the percentage area immunostained with α-SMA in the lung by nearly 4.6-fold, compared with the normal control group. Meanwhile, lung sections from the BLM + BET group displayed a notable surge in E-cadherin expression by 3.7-fold accompanied by a marked diminution in α-SMA expression by approximately 1.9-folds compared to that in the BLM group (Fig. 6a, c and d).
4. Discussion
IPF is a heterogenous progressive disease that is characterized by the proliferation and differentiation of the fibroblasts into myofibroblasts leading to excessive extracellular matrix deposition and finally respiratory failure and death.24 Guidelines for the IPF treatment recommend the use of nintedanib and pirfenidone, which have restricted efficacy for the prevention of disease progression and enhancement of life quality.25 Thus, new therapeutic potential agents are needed.
Natural products are considered a rich source of biologically active molecules, enabling drug discovery.26 The promising anti-fibrotic role of natural compounds has gathered growing interest over the last two decades. The potential benefits of natural products include their robust safety standards and numerous biological properties, which are vital elements in the prevention and treatment of pulmonary fibrosis.27 Accordingly, natural products with potent anti-inflammatory effects were considered for preventing pulmonary fibrosis in COVID-19 patients.26 Thus, we investigated the protective impact of BET, the most abundant compound in beetroot, against the NLRP3-mediated EMT in BLM-induced pulmonary fibrosis in rats.
The study showed a massive infiltration of inflammatory cells, involving neutrophils and lymphocytes and deterioration of alveolar epithelial cells upon examination of total and differential cell count after instillation of BLM. These results are in agreement with previous studies.28,29 Moreover, LDH activity in BALF was increased in BLM rats indicating interstitial lung injury, which is in line with previous studies.29,30 Surprisingly, BET revealed an anti-inflammatory effect emphasized by attenuating total and differential cell counts (neutrophils and lymphocytes counts) and LDH activity. This is in harmony with the reported anti-inflammatory effect of BET in lipopolysaccharide-induced acute lung injury in mice.31 These findings were further reinforced through the histopathological examination of lung tissues stained with H&E, which indicated the ability of BET to protect against pathological alterations in the lung structure and inflammatory cell infiltration occurring after BLM induction.
In pulmonary fibrosis, the massive deposition of the extracellular matrix such as collagen causes an increase in lung weight and subsequent elevation in the lung/body weight index, which was further confirmed by histopathological examination using Masson's trichrome staining that demonstrates the substantial increase in collagen fiber area% and elevated the hydroxyproline level indicating an increased accumulation of collagen. This is in agreement with a previous report that showed a similar increment in the lung/body weight index and lung collagen deposition after endotracheal instillation of BLM.32 The BET treatment notably attenuated the increase in lung/body weight index, %fibrosis area, and the hydroxyproline level in BLM rats, which might illustrate its anti-fibrotic effect.
Inflammation and oxidative stress are critical hallmarks of pulmonary fibrosis. The increased numbers of inflammatory cells and cytokines induce oxidative stress and eventually lung injury.33 BLM administration increases the levels of ROS as proven by increased MDA, which demonstrates the lipid peroxidation level in the cells, leading to intense consumption of antioxidant defenses in lung cells such as GSH depletion that hinders its vital role in scavenging free radicals.34,35 However, following the BET treatment, the MDA content was significantly decreased and GSH content was notably boosted, pointing to the antioxidant effect of BET. This is in accordance with previous studies that reported the antioxidant effect of BET.36,37
The activation of NLRP3 inflammasome plays a key role in promoting IPF via the amplification of the pro-inflammatory cascade.5 Therefore, suppression of the NLRP3 inflammasome activation can diminish inflammation and fibrosis in the lung.38,39 NLRP3 inflammasomes involve the receptor or sensor protein NLRP3, adaptor protein ASC, and effector protein caspase-1. Upon exposure to stimuli, NLRP3 recruits the adapter protein ASC via its PYD domain, then binds procaspase-1 to the ASC via its CARD domain leading to maturation of the effector caspase-1. Cleaved caspase-1 in turn activates proteolytic cleavage of pro-IL-1β into the active form IL-1β which drives proinflammatory responses that participate in cellular damage. Moreover, IL-1β promotes ROS accumulation, which in turn stimulates the activation of NLRP3 inflammasome, resulting in the formation of more IL-1β and initiation of a feedback loop between the activation of NLRP3 inflammasome and oxidative stress.40–42 Our study revealed the activation of NLRP3 inflammasome pathway in the BLM group indicated by an increased NLPP3, ASC, caspase-1, and IL-1β, which is in line with previous studies39,43 that demonstrate the activation of NLRP3 inflammasome after instillation of BLM. Contrariwise, the enhanced NLPP3, ASC, caspase-1, and IL-1β in BLM rats were attenuated after BET administration. These findings suggest that attenuation of the pro-inflammatory response cascade by BET may be attributed to inhibiting the stimulation of NLRP3 inflammasome.
Additionally, the activation of NLRP3 inflammasome modulates EMT via TGF-β1 in BLM-induced pulmonary fibrosis.5 NLRP3 regulates TGF-β1 expression by affecting the IL-1β level, which can up-regulate TGF-β1 in lung fibrosis.44 EMT is a pathophysiological process that allows the losing part of the markers and characteristics of epithelial cells while earning mesenchymal ones. EMT plays a crucial role in the development of IPF. TGF-β1 is a pro-fibrotic factor that induces EMT and promotes the production and deposition of collagen. In addition, TGF-β1 mediates epithelial cell apoptosis and recruitment, activation, and differentiation of fibroblasts into myofibroblasts to produce α-SMA, which is vital in pulmonary fibrosis.45,46 Moreover, in pathological conditions, elevated levels of ROS in the lung epithelium are a well-defined primary trigger causing epithelial damage, including EMT.47 Oxidative stress is also harmful as it is linked to the TGF-β1 pro-fibrogenic activities.48 Indeed, the present study revealed that TGF-β1 and EMT were enhanced in the lungs of BLM-induced rats and this comes in accordance with previous reports.49,50 BET treatment attenuated the increase in TGF-β1 and EMT evidenced by an elevation in the epithelial cells marker E-cadherin, as well as a reduction in the mesenchymal cells markers vimentin and α-SMA when compared with the corresponding levels of these markers in the BLM rats. This is in agreement with a previous study that reported the efficacy of BET in the treatment of renal fibrosis by regulating the EMT process in NRK-52E cells.51 These results indicated that BET regulates EMT by suppressing oxidative stress, TGF-β1, and regulating NLRP3.
It has been reported that beetroot is the major source of BET and contains 300–600 mg kg−1 BET.17,52 According to the United States Department of Agriculture (USDA), the typical serving size for 60 or 70 kg human is one cup of beetroot juice per day, which is equivalent to 350 g of beetroot. Thus, considering a 60 kg individual consuming one cup of raw beetroot (350 g day−1), which contains 600 mg kg−1 BET at max, the typical human BET dose will be 210 mg day−1 per 60 kg individual. Meanwhile, the human BET equivalent dose is 4 mg kg−1 according to the conversion dose calculation formula from rats to humans, as previously described by Reagan-Shaw et al.53 Therefore, the total human BET equivalent dose for a 60 kg individual is 240 mg. According to the previously mentioned calculations, we found that the total human equivalent dose = the amount of BET in one serving of beetroot. Therefore, we conclude that consuming one cup of beetroot juice per day may prevent the development of lung fibrosis.
5. Conclusions
In conclusion, the protective effect of BET against BLM-induced pulmonary fibrosis in rats could be attributed to modulating inflammatory response and EMT process by targetting the NLRP3/IL-1β/TGF-β1 signaling pathway, supplying a novel candidate for protection against pulmonary fibrosis.
Abbreviations
ASC | Apoptosis-associated speck-like protein containing a CARD |
α-SMA | α-Smooth muscle actin |
BALF | Bronchoalveolar lavage fluid |
BET | Betanin |
BLM | Bleomycin |
EMT | Epithelial–mesenchymal transition |
GSH | Glutathione |
H&E | Hematoxylin and eosin |
IPF | Idiopathic pulmonary fibrosis |
IL-1β | Interleukin-1β |
MDA | Malondialdehyde |
NLRP3 | NOD-like receptor family, pyrin domain-containing protein 3 |
ROS | Reactive oxygen species |
TGF-β1 | Transforming growth factor-β1 |
TLC | Total leukocyte count. |
Author contributions
NAA and SAH: conceptualization; performed the practical work, and data acquisition; formal analysis; writing and revising the manuscript.
Conflicts of interest
There are no conflicts of interest to declare.
References
- H. Jin, M. Jeong, G. Lee, M. Kim, Y. Yoo, H. J. Kim, J. Cho, Y.-S. Lee and H. Lee, Engineered Lipid Nanoparticles for the Treatment of Pulmonary Fibrosis by Regulating Epithelial-Mesenchymal Transition in the Lungs, Adv. Funct. Mater., 2023, 33, 2209432 CrossRef.
- H. Robbie, C. Daccord, F. Chua and A. Devaraj, Evaluating disease severity in idiopathic pulmonary fibrosis, Eur. Respir. Rev., 2017, 26, 170051 CrossRef PubMed.
- F. Salton, M. C. Volpe and M. Confalonieri, Epithelial-Mesenchymal Transition in the Pathogenesis of Idiopathic Pulmonary Fibrosis, Medicina, 2019, 55, 83 CrossRef PubMed.
- A. Andersson-Sjöland, K. Nihlberg, L. Eriksson, L. Bjermer and G. Westergren-Thorsson, Fibrocytes and the tissue niche in lung repair, Respir. Res., 2011, 12, 76 CrossRef PubMed.
- R. Tian, Y. Zhu, J. Yao, X. Meng, J. Wang, H. Xie and R. Wang, NLRP3 participates in the regulation of EMT in bleomycin-induced pulmonary fibrosis, Exp. Cell Res., 2017, 357, 328–334 CrossRef PubMed.
- M. A. Nieto, R. Y. Huang, R. A. Jackson and J. P. Thiery, EMT: 2016, Cell, 2016, 166, 21–45 CrossRef PubMed.
- H. Guo, J. B. Callaway and J. P. Ting, Inflammasomes: mechanism of action, role in disease, and therapeutics, Nat. Med., 2015, 21, 677–687 CrossRef PubMed.
- T. Strowig, J. Henao-Mejia, E. Elinav and R. Flavell, Inflammasomes in health and disease, Nature, 2012, 481, 278–286 CrossRef.
- I. Lasithiotaki, I. Giannarakis, E. Tsitoura, K. D. Samara, G. A. Margaritopoulos, C. Choulaki, E. Vasarmidi, N. Tzanakis, A. Voloudaki, P. Sidiropoulos, N. M. Siafakas and K. M. Antoniou, NLRP3 inflammasome expression in idiopathic pulmonary fibrosis and rheumatoid lung, Eur. Respir. J., 2016, 47, 910–918 CrossRef.
- W. Wang, X. Wang, J. Chun, A. Vilaysane, S. Clark, G. French, N. A. Bracey, K. Trpkov, S. Bonni, H. J. Duff, P. L. Beck and D. A. Muruve, Inflammasome-independent NLRP3 augments TGF-β signaling in kidney epithelium, J. Immunol., 2013, 190, 1239–1249 CrossRef PubMed.
- L. L. Zhang, S. Huang, X. X. Ma, W. Y. Zhang, D. Wang, S. Y. Jin, Y. P. Zhang, Y. Li and X. Li, Angiotensin(1–7) attenuated Angiotensin II-induced hepatocyte EMT by inhibiting NOX-derived H2O2-activated NLRP3 inflammasome/IL-1β/Smad circuit, Free Radical Biol. Med., 2016, 97, 531–543 CrossRef PubMed.
- K. Yamauchi, Y. Kasuya, F. Kuroda, K. Tanaka, J. Tsuyusaki, S. Ishizaki, H. Matsunaga, C. Iwamura, T. Nakayama and K. Tatsumi, Attenuation of lung inflammation and fibrosis in CD69-deficient mice after intratracheal bleomycin, Respir. Res., 2011, 12, 131 CrossRef PubMed.
- J. D. Williamson, L. R. Sadofsky and S. P. Hart, The pathogenesis of bleomycin-induced lung injury in animals and its applicability to human idiopathic pulmonary fibrosis, Exp. Lung Res., 2015, 41, 57–73 CrossRef PubMed.
- A. Moeller, K. Ask, D. Warburton, J. Gauldie and M. Kolb, The bleomycin animal model: a useful tool to investigate treatment options for idiopathic pulmonary fibrosis?, Int. J. Biochem. Cell Biol., 2008, 40, 362–382 CrossRef.
- L. Atzori, F. Chua, S. E. Dunsmore, D. Willis, M. Barbarisi, R. J. McAnulty and G. J. Laurent, Attenuation of bleomycin induced pulmonary
fibrosis in mice using the heme oxygenase inhibitor Zn-deuteroporphyrin IX-2,4-bisethylene glycol, Thorax, 2004, 59, 217–223 CrossRef PubMed.
- C. Gupta and D. Prakash, Phytonutrients as therapeutic agents, J. Complementary Integr. Med., 2014, 11, 151–169 CrossRef.
- D. Vieira Teixeira da Silva, D. dos Santos Baião, F. de Oliveira Silva, G. Alves, D. Perrone, E. Mere Del Aguila and V. M. Flosi Paschoalin, Betanin, a natural food additive: Stability, bioavailability, antioxidant and preservative ability assessments, Molecules, 2019, 24, 458 CrossRef PubMed.
- E. Madadi, S. Mazloum-Ravasan, J. S. Yu, J. W. Ha, H. Hamishehkar and K. H. Kim, Therapeutic Application of Betalains: A Review, Plants, 2020, 9, 1219 CrossRef.
- D. V. Silva, O. Silva Fde, D. Perrone, A. P. Pierucci, C. A. Conte-Junior, S. Alvares Tda, E. M. Aguila and V. M. Paschoalin, Physicochemical, nutritional, and sensory analyses of a nitrate-enriched beetroot gel and its effects on plasmatic nitric oxide and blood pressure, Food Nutr. Res., 2016, 60, 29909 CrossRef PubMed.
- M. S. Zaghloul, E. Said, G. M. Suddek and H. A. Salem, Crocin attenuates lung inflammation and pulmonary vascular dysfunction in a rat model of bleomycin-induced pulmonary fibrosis, Life Sci., 2019, 235, 116794 CrossRef.
- T. K. Motawi, S. A. Ahmed, N. A. El-Boghdady, N. S. Metwally and N. N. Nasr, Protective effects of betanin against paracetamol and diclofenac induced neurotoxicity and endocrine disruption in rats, Biomarkers, 2019, 24, 645–651 Search PubMed.
- W. Du, Z. Tang, F. Yang, X. Liu and J. Dong, Icariin attenuates bleomycin-induced pulmonary fibrosis by targeting Hippo/YAP pathway, Biomed. Pharmacother., 2021, 143, 112152 CrossRef PubMed.
- M. H. Zohny, S. Cavalu, M. E. Youssef, M. M. Y. Kaddah, A. A. E. Mourad, A. G. A. Gaafar, E. El-Ahwany, N. A. Amin, H. M. Arakeep, A. Shata, S. Saleh, M. M. Hafez, S. T. Elazab, R. Abdelhady, R. M. El Shahat, G. Yahya and S. Saber, Coomassie brilliant blue G-250 dye attenuates bleomycin-induced lung fibrosis by regulating the NF-κB and NLRP3 crosstalk: A novel approach for filling an unmet medical need, Biomed. Pharmacother., 2022, 148, 112723 CrossRef.
- L. Richeldi, H. R. Collard and M. G. Jones, Idiopathic pulmonary fibrosis, Lancet, 2017, 389, 1941–1952 CrossRef.
- P. Spagnolo, J. A. Kropski, M. G. Jones, J. S. Lee, G. Rossi, T. Karampitsakos, T. M. Maher, A. Tzouvelekis and C. J. Ryerson, Idiopathic pulmonary fibrosis: Disease mechanisms and drug development, Pharmacol. Ther., 2021, 222, 107798 CrossRef PubMed.
- I. Arslan, Natural PAK1 inhibitors: potent anti-inflammatory effectors for prevention of pulmonary fibrosis in COVID-19 therapy, Nat. Prod. Res., 2023, 1–13, DOI:10.1080/14786419.2023.2254454.
- M. Hasan, N. C. Paul, S. K. Paul, A. S. M. Saikat, H. Akter, M. Mandal and S. S. Lee, Natural Product-Based Potential Therapeutic Interventions of Pulmonary Fibrosis, Molecules, 2022, 27, 1481 CrossRef PubMed.
- M. O. Kseibati, G. S. Shehatou, M. H. Sharawy, A. E. Eladl and H. A. Salem, Nicorandil ameliorates bleomycin-induced pulmonary fibrosis in rats through modulating eNOS, iNOS, TXNIP and HIF-1α levels, Life Sci., 2020, 246, 117423 CrossRef PubMed.
- R. Alaaeldin, R. H. Mohyeldin, A. A. Bekhit, W. Gomaa, Q.-L. Zhao and M. Fathy, Vincamine Ameliorates Epithelial-Mesenchymal Transition in Bleomycin-Induced Pulmonary Fibrosis in Rats; Targeting TGF-β/MAPK/Snai1 Pathway, Molecules, 2023, 28, 4665 CrossRef PubMed.
- J. L. Judge, D. J. Nagel, K. M. Owens, A. Rackow, R. P. Phipps, P. J. Sime and R. Kottmann, Prevention and treatment of bleomycin-induced pulmonary fibrosis with the lactate dehydrogenase inhibitor gossypol, PLoS One, 2018, 13, e0197936 CrossRef PubMed.
- Z. Wu, L. Chen, Q. Wang, C. Govindasamy, A. S. Sivakumar and X. Chen, Betanin ameliorates Lipopolysaccharide-induced acute lung injury in mice via inhibition of inflammatory response and oxidative stress, Arabian J. Chem., 2023, 16, 104763 CrossRef.
- M. S. Zaghloul, E. Said, G. M. Suddek and H. A. Salem, Crocin attenuates lung inflammation and pulmonary vascular dysfunction in a rat model of bleomycin-induced pulmonary fibrosis, Life Sci., 2019, 235, 116794 CrossRef PubMed.
- M. Raish, A. Ahmad, M. A. Ansari, A. Ahad, F. I. Al-Jenoobi, A. M. Al-Mohizea, A. Khan and N. Ali, Sinapic acid ameliorates bleomycin-induced lung fibrosis in rats, Biomed. Pharmacother., 2018, 108, 224–231 CrossRef PubMed.
- H. H. Mansour, M. M. Omran, H. F. Hasan and S. M. El Kiki, Modulation of bleomycin–induced oxidative stress and pulmonary fibrosis by N–acetylcysteine in rats via AMPK/SIRT1/NF–κβ, Clin. Exp. Pharmacol. Physiol., 2020, 47, 1943–1952 CrossRef.
- R. M. Hussein, E.-S. A. Arafa, S. A. Raheem and W. R. Mohamed, Thymol protects against bleomycin-induced pulmonary fibrosis via abrogation of oxidative stress, inflammation, and modulation of miR-29a/TGF-β and PI3K/Akt signaling in mice, Life Sci., 2023, 314, 121256 CrossRef PubMed.
- M. Mousavi, N. Abedimanesh, K. Mohammadnejad, E. Sharini, M. Nikkhah, M. R. Eskandari, B. Motlagh, J. Mohammadnejad, H. Khodabandehloo and M. Fathi, Betanin alleviates oxidative stress through the Nrf2 signaling pathway in the liver of STZ-induced diabetic rats, Mol. Biol. Rep., 2022, 49, 9345–9354 CrossRef PubMed.
- D. V. T. d. Silva, D. d. S. Baiao, V. F. Ferreira and V. M. F. Paschoalin, Betanin as a multipath oxidative stress and inflammation modulator: A beetroot pigment with protective effects on cardiovascular disease pathogenesis, Crit. Rev. Food Sci. Nutr., 2021, 62, 539–554 CrossRef.
- J. Wang, H. Wang, F. Fang, C. Fang, S. Wang, C. Lu and N. Liu, Danggui Buxue Tang ameliorates bleomycin-induced pulmonary fibrosis by suppressing the TLR4/NLRP3 signaling pathway in rats, Evidence-Based Complementary Altern. Med., 2021, 2021, 8030143 Search PubMed.
- J. Li, X. Yang, P. Yang, K. Xu, X. Peng, W. Cai, S. Zhao, L. Hu, Z. Li and F. Cui, Andrographolide alleviates bleomycin-induced NLRP3 inflammasome activation and epithelial-mesenchymal transition in lung epithelial cells by suppressing AKT/mTOR signaling pathway, Ann. Transl. Med., 2021, 9, 764 CrossRef PubMed.
- Y. Chen, Y. Zhang, N. Li, Z. Jiang and X. Li, Role of mitochondrial stress and the NLRP3 inflammasome in lung diseases, Inflammation Res., 2023, 72, 829–846 CrossRef PubMed.
- A. Dominic, N.-T. Le and M. Takahashi, Loop between NLRP3 inflammasome and reactive oxygen species, Antioxid. Redox Signaling, 2022, 36, 784–796 CrossRef PubMed.
- R. M. C. Biancatelli, P. A. Solopov and J. D. Catravas, The inflammasome NLR family Pyrin domain-containing protein 3 (NLRP3) as a novel therapeutic target for idiopathic pulmonary fibrosis, Am. J. Pathol., 2022, 192, 837–846 CrossRef PubMed.
- L. Peng, L. Wen, Q.-F. Shi, F. Gao, B. Huang, J. Meng, C.-P. Hu and C.-M. Wang, Scutellarin ameliorates pulmonary fibrosis through inhibiting NF-κB/NLRP3-mediated epithelial–mesenchymal transition and inflammation, Cell Death Dis., 2020, 11, 978 CrossRef PubMed.
- L. Borthwick, E. McIlroy, M. Gorowiec, M. Brodlie, G. Johnson, C. Ward, J. Lordan, P. Corris, J. Kirby and A. Fisher, Inflammation and epithelial to mesenchymal transition in lung transplant recipients: role in dysregulated epithelial wound repair, Am. J. Transplant., 2010, 10, 498–509 CrossRef PubMed.
- F. Salton, M. C. Volpe and M. Confalonieri, Epithelial–mesenchymal transition in the pathogenesis of idiopathic pulmonary fibrosis, Medicina, 2019, 55, 83 CrossRef PubMed.
- W. Qian, X. Cai, Q. Qian, W. Zhang and D. Wang, Astragaloside IV modulates TGF–β1−dependent epithelial–mesenchymal transition in bleomycin–induced pulmonary fibrosis, J. Cell. Mol. Med., 2018, 22, 4354–4365 CrossRef CAS PubMed.
- M. R. Gorowiec, L. A. Borthwick, S. M. Parker, J. A. Kirby, G. C. Saretzki and A. J. Fisher, Free radical generation induces epithelial-to-mesenchymal transition in lung epithelium via a TGF-β1-dependent mechanism, Free Radicals Biol. Med., 2012, 52, 1024–1032 CrossRef CAS PubMed.
- L.-Y. Peng, L. An, N.-Y. Sun, Y. Ma, X.-W. Zhang, W.-H. Liu, B.-L. Liu, P. Li and J. Chen, Salvia miltiorrhiza restrains reactive oxygen species-associated pulmonary fibrosis via targeting Nrf2-Nox4 redox balance, Am. J. Chin. Med., 2019, 47, 1113–1131 CrossRef CAS PubMed.
- L. Wang, M. Shao, W. Jiang and Y. Huang, Resveratrol alleviates bleomycin-induced pulmonary fibrosis by inhibiting epithelial-mesenchymal transition and down-regulating TLR4/NF-κB and TGF-β1/smad3 signalling pathways in rats, Tissue Cell, 2022, 79, 101953 CrossRef CAS PubMed.
- S. B. Andugulapati, K. Gourishetti, S. K. Tirunavalli, T. B. Shaikh and R. Sistla, Biochanin-A ameliorates pulmonary fibrosis by suppressing the TGF-β mediated EMT, myofibroblasts differentiation and collagen deposition in in vitro and in vivo systems, Phytomedicine, 2020, 78, 153298 CrossRef CAS PubMed.
- B. Sutariya, N. Taneja, L. Badgujar and M. Saraf, Modulatory effect of betanin on high glucose induced epithelial to mesenchymal transition in renal proximal tubular cells, Biomed. Pharmacother., 2017, 89, 18–28 CrossRef CAS PubMed.
- T. Clifford, G. Howatson, D. J. West and E. J. Stevenson, The potential benefits of red beetroot supplementation in health and disease, Nutrients, 2015, 7, 2801–2822 CrossRef CAS PubMed.
- S. Reagan-Shaw, M. Nihal and N. Ahmad, Dose translation from animal to human studies revisited, FASEB J., 2008, 22, 659–661 CrossRef CAS PubMed.
|
This journal is © The Royal Society of Chemistry 2024 |
Click here to see how this site uses Cookies. View our privacy policy here.