DOI:
10.1039/D4MA00020J
(Paper)
Mater. Adv., 2024,
5, 6516-6534
Advancing diabetes treatment: novel formulation of polydatin long-circulating liposomes and their glucose-regulating impact on hyperlipidemia-induced type 2 diabetic mice
Received
9th January 2024
, Accepted 8th July 2024
First published on 11th July 2024
Abstract
Polydatin, a monomer derived from the dried roots and stems of traditional Chinese medicinal herb, Polygonum cuspidatum, boasts extensive pharmacological activities. Existing nanocarriers of polydatin have not been able to prolong the circulation time for enhanced antidiabetic potential of the stilbenoid polyphenol. This study aims to improve the sustained release and antidiabetic potential of polydatin by fabricating long circulating liposomes (PLLs) via a membrane dispersion method. An orthogonal optimized PLL composed of lecithin, DSPE-PEG2000, and cholesterol was produced, wherein evaluation of the physical properties showed that the prepared PLL had a spherical shape with uniform size, small particle size, and even distribution as well as high encapsulation efficiency and drug loading, along with good physical stability at 4 and 25 °C for 30 days. In vivo release experiments in different buffers via the dialysis bag method suggested that the PLL provided a sustained release of polydatin. Also, in vitro cell uptake and in vivo pharmacokinetics studies of the PLL respectively demonstrated improved cellular uptake and prolonged in vivo circulation time and bioavailability. Using a high-fat diet-induced obese type 2 diabetes mellitus (T2DM) mouse model, it was observed that the PLL could lower blood sugar, improve weight loss, reduce antioxidant stress, lower blood lipids, and ameliorate liver, spleen, and pancreatic tissue damage. This study successfully prepared long-circulating liposomes of polydatin, which sustained in vitro release of polydatin, improved bioavailability in vivo, and enhanced the activity of the drug in treating obese diabetic mice.
1. Introduction
Diabetes, a chronic metabolic disease, is characterized by hyperglycemia.1,2 Diabetic patients often have lipid, protein, and water electrolyte disorders.3 Because of its high incidence rate, increased prevalence of complications and high mortality, it has become one of the world's biggest health problems. Research data suggest that in 2021, the global prevalence of diabetes among people aged 20 to 79 years was 536.6 million, which is expected to rise to 783.2 million by 2045. Among them, the prevalence of diabetes among people aged 20 to 79 years in China is ranked first.4 Ninety percent (90%) of the total number of diabetics are accounted by type 2 diabetes mellitus (T2DM), which is a sub-type of diabetes.5 The occurrence of diabetes has been closely related to diet, environment and other factors. The eating habits of people today, the intake of high sugar and calories, as well as fried food has significantly increased, coupled with the current fast pace of life, high work pressure, and lack of exercise, which makes the incidence of type 2 diabetes significantly increased.6 After the occurrence of diabetes, patients are often accompanied by polydipsia, polyuria, emaciation, and other conditions. As the disease course develops, there will also be various acute and chronic complications that involve multiple organs and tissues such as the kidneys, eyes, feet, etc., which will seriously affect the health and quality of life of patients.7
Although there are many different types of anti-diabetic drugs to select from at present,8 only 49.2% of T2DM patients in China reached the control target of glycosylated hemoglobin level <7.0%. At present, there is no effective radical cure plan for diabetes, whilst exogenous insulin is clinically used to treat type I diabetes. T2DM is mainly treated with oral hypoglycemic drugs such as biguanides, sulfonylureas, glucosidase inhibitors, and thiazolidinedione derivatives.9 These drugs treat diabetes through different modes of action, but there are generally problems such as large adverse drug reactions, and inability to prevent diabetes and improve its complications. For example, the long-term use of insulin preparations can reduce the sensitivity of pancreatic islets, thus leading to reduced efficacy and insulin resistance.10 Bisguanidine drugs can cause loss of appetite, amid patients being prone to diarrhea, malignancy, and abdominal distension, as well as even liver and kidney failure. Sulfonylurea insulin secretagogues can compete with plasma protein binding sites and increase their efficacy when combined with liver inhibitory enzyme drugs, thereby making them more prone to hypoglycemia. After taking glucosidase inhibitors, they are rarely absorbed by the body into the bloodstream, and a large amount of carbohydrates are broken down and digested in the intestines, thus causing gastrointestinal reactions such as bloating and abdominal pain.11 In recent years, some new target drugs for the treatment of diabetes have been approved, such as glucagon like peptide-1 (GLP-1) receptor agonist, dipeptidyl peptidase IV (DPP-4) inhibitor, glucokinase (GK) agonist, protein tyrosine phosphatase 1B (PTP-1B) and sodium glucose cotransporter 2 (SGLT2).12–14 Single or combined use can effectively control blood sugar and provide patients with more medication options, but there are drawbacks such as low activity, poor kinetic characteristics, and toxic side effects.15 Therefore, it was of great significance to find new anti-diabetes drugs to better control blood sugar by reducing the occurrence of adverse reactions, delaying or decreasing the complications of diabetes.
In recent years, traditional Chinese medicine and its active ingredients have shown greater structural diversity and less side effects than synthetic compounds, thus showing great advantages and potential in the treatment of diabetes, a chronic disease, and playing a role in the discovery of new drug research lead compounds.16 Currently, various natural active ingredients have been isolated from traditional Chinese medicine for lowering blood sugar, including capsaicin,17 ursolic acid,18 dioscin19 and polysaccharides.20
As a medicinal plant with long historical use, “Huzhang” is documented as the dry root and stem of Polygonum cuspidatum Sieb. et Zucc (Polygonaceae).21 The main functions include dispelling wind and dampness, dispelling phlegm and relieving cough, clearing heat and detoxifying, promoting blood circulation and resolving blood stasis, etc. P. cuspidatum contains various active ingredients, mainly including resveratrol, P. cuspidatum glycoside, emodin and its glycosides.22 Polydatin is a stilbene compound isolated from P. cuspidatum. Modern pharmacological data shows that polydatin has a series of different pharmacological activities, including hypolipidemic, anti-atherosclerosis, antioxidant, anti-inflammatory and neuroprotective functions.23–25 Further research has shown that polydatin can significantly reduce blood sugar, glycosylated hemoglobin value and serum creatine kinase activity in diabetes rats and improve the biomarkers of cardiac function.26 Zheng et al.27 also confirmed that polydatin could regulate lipid metabolism by inhibiting adipose tissue inflammation in high-fat mice. Hao et al.28 used low-dose streptozotocin (STZ) and high fat and sugar diets to induce a diabetes rat model and palmitic acid-induced insulin resistance HepG2 cell model. The results showed that polydatin significantly reduced fasting blood glucose, glycosylated hemoglobin, glycosylated serum protein, total cholesterol, triglycerides, and low-density lipoprotein cholesterol by regulating the Akt signaling pathway, increased glucose uptake and consumption, and increased serum insulin levels. It was reported that in the model of diabetes, polydatin protected the heart from ischemic reperfusion injury via regulation of the renin angiotensin system and reduction of reactive oxygen species (ROS) production.29,30 Also, earlier works have established the potential of polydatin to inhibit impaired insulin sensitivity and improve liver steatosis.31 Therefore, the hypoglycemic effect of polydatin was studied to explore a new drug for diabetes.
However, critical cornerstone issues such as low solubility, chemical instability in an aqueous alkaline medium, short peak time, and low oral bioavailability of polydatin in vivo still need to be considered.32 Lv et al.33 reported decreased pharmacokinetic profile after oral administration of polydatin in male Wistar rats. Another study showed that the absolute bioavailability of polydatin was as low as 2.9% after administration to polydatin in rats.34 Therefore, researchers have attempted to address these issues through drug delivery systems. The development of a polydatin inclusion complex has increased the oral bioavailability of polydatin.35 Wang et al.36 prepared a polydatin liposome system (PLD) with a smaller particle size (PS) and acceptably high encapsulation efficiency (EE). The authors studied the in vitro release of polydatin from the conventional liposomes within 24 hours, which demonstrated a short time of drug circulation in the blood.37 Also, the PLD liposomes could substantially sustain the circulation time of polydatin in vivo and improve oral bioavailability of the drug. Abdel et al.38 developed a new oral formulation of polydatin-loaded chitosan nanoparticles (PD-CSNP) to improve the therapeutic potential of PD for type 2 diabetes. The actual results showed that the EE of PD-CSNP was high, whereas the particle size (PS) was around 144.25 nm. The release in vitro had a certain sustained-release effect, while in vivo studies showed that compared with free PD, PD-CSNPs showed a very significant anti-diabetic effect in T2DM rats. Despite these results, existing literature suggests that surface modified liposomes like long-circulating liposomes could prolong the time of circulation for increased accumulation of drugs in targeted sites compared to conventional liposomal drugs. Besides, the above-mentioned research did not evaluate the in vitro cellular uptake and in vivo pharmacological application of polydatin liposomes.
Liposomes are a widely used nano drug delivery system, which have many advantages such as good histocompatibility, slow release and high cellular affinity, and are one of the most promising candidate dosage forms.39,40 Liposomes can encapsulate hydrophilic, lipophilic, and amphoteric drug molecules in the core or lipid bilayer.41,42 However, after entering the body's circulation, ordinary liposomes undergo a regulatory effect by adsorbing plasma proteins, which in turn are absorbed by the reticuloendothelial tissue system (RES) and cleared, thus resulting in their inability to exert their pharmacological effects.43 The surface property of liposomes is an important factor affecting the clearance rate of liposomes in systemic circulation.44 After modifying the phospholipids with polyethylene glycol (PEG), such as N-(carbonyl-methoxypolyethylene glycol 2000)-1,2-distearoyl-sn-glycerol-3-phosphoethanolamine (DSPE-PEG2000), long-circulating liposomes could be prepared, which could significantly increase the circulation time of drugs in the blood circulation system, and increase the in vivo bioavailability of drugs.45,46 DSPE-PEG2000 is a polymer material with one end hydrophilic and one end lipophilic. The mechanism by which PEG prolongs the half-life of liposomes in the blood circulation was as follows: there were a large number of ethoxyl groups in PEG molecules that can form a large number of hydrogen bonds with water molecules, thus making PEG highly water-soluble and capable of forming a hydration film on the surface of liposomes.47–49 The PEG intersected and overlapped on the surface of liposomes, thus forming a dense conformational cloud and steric hindrance, as well as hindering the adsorption of certain proteins and cell adhesion, along with masking the hydrophobic binding sites on the surface of liposomes, reducing the van der Waals force between plasma proteins and liposomes, and hindering the approach of plasma components to liposomes, thus effectively avoiding the recognition and phagocytosis of RES.50,51 Nowadays, DSPE-PEG2000 is widely used in the preparation of long-circulating liposomes to improve the in vivo bioavailability of insoluble drugs.52–54
In this study, polydatin long-circulating liposomes (PLLs) were prepared to improve the in vivo bioavailability of insoluble drugs. Afterward, the PLLs were characterized in terms of PS, electrokinetic potential (EKP), drug loading (DL), EE, stability, and release behavior. Also, the anti-diabetic effect and mechanism of the PLLs were evaluated in high fat diet (HFT)-induced diabetes in mice.
2. Materials and methods
2.1 Materials
Aladdin Industrial Corporation (Shanghai-China) supplied polydatin (99% purity). HPLC acetonitrile was bought from Sinopharm Chemical Reagent Co. Ltd (Shanghai, China). Bistearyl phosphatidylethanolamine-polyethylene glycol 2000 (DSPE-PEG200) was supplied by AVT Pharmaceutical Co., Ltd (Shanghai-China). Phospholipid and sodium cholate were bought from Aladdin Industrial Corporation (Shanghai, China). Malondialdehyde (MDA), superoxide dismutase (SOD), glutathione peroxidase (GSH-Px), tumor necrosis factor-α (TNF-α), high-density lipoprotein cholesterol (HDL-C) and low-density lipoprotein cholesterol (LDL-C), triglyceride (TG) as well as total cholesterol (TC) Elisa kits were from Nanjing JianCheng Bioengineering Institute (Nanjing, China).
2.2 Cells and animals
HCT116 cells were provided by the Academy of Science for cell bank (Shanghai-China). C57bl/6J mice (6-week-old, male) and Sprague Dawley (SD) rats (200 ± 20 g) were supplied by Jiangsu University laboratory center (Zhenjiang-China). The conducting of animal experiments was carried out in accordance with guidelines and regulations laid down for Care and management of laboratory animals. The protocol for the animal experiments was subjected to thorough review by The Committee of Institute of Animal Care and Use of Jiangsu University.
2.3 HPLC analysis of polydatin
The concentration of polydatin was determined via HPLC (Shimadzu Corporation, Tokyo Japan). First, the maximum absorption wavelength of polydatin was determined using full wavelength scanning under an ultraviolet spectrophotometer (UV2600, Shimadzu, Japan). Then, the chromatographic conditions were established as follows: the mobile phase was acetonitrile/water (20/80, v/v), the flow rate was 1.0 mL min−1, the detection wavelength was 306 nm, and the injection volume was 20 μL. By preparing the gradient concentration of polydatin standard solution (in vitro: 0.1, 0.2, 0.5, 1, 2, 5, 10, 25, 50, 100 and 200 μg mL−1; in vivo: 0.5, 1, 2, 5, 10 and 25 μg mL−1) and 40 μg mL−1 astragaloside solution (internal standard in vivo), we established the in vivo (1) and in vitro (2) standard curves of polydatin as follows: | Y = 102.05C − 39.572 (R2 = 0.9999) | (1) |
| A = 0.225C + 0.0037 (R2 = 0.9984) | (2) |
where C is the concentration of polydatin, Y is the peak area of polydatin, and A is the ratio of polydatin peak area to baicalin. These two equations showed a good linear relation in the range of 0.1–200 μg mL−1 (1) and 0.1–25 μg mL−1 (2).
2.4 Equilibrium solubility of polydatin
Excessive polydatin was added to four flasks containing 20 mL of the respective dissolution media ((phosphate buffer solution (PBS)–pH 6.8, 7.4, purified water–pH 7.0 and HCl–pH 1.2)). Afterward, the flasks were shaken at 37.0 ± 0.5 °C for 72 hours through a constant temperature water bath oscillator. After the equilibrium had been reached, centrifugation was performed at 10
000 rpm for 10 minutes to remove the undissolved polydatin. After the supernatant was properly diluted, the drug solubility could be determined by HPLC analysis.
2.5 Preparation of PLLs
Herein, PLLs were prepared by the membrane dispersion method. Briefly, DSPE-PEG200, cholesterol, lecithin and polydatin were dissolved in an appropriate amount of methanol. After ultrasonic dissolution, methanol was removed at 40 °C using a rotary evaporator. Afterwards, purified water was used to disperse the liposomes into a uniform membrane. Subsequently, the liposomal solution was obtained and stored at 4 °C for further study. Based on the results of a single factor experiment, an orthogonal design experiment (as shown in Table 1) was carried out with the ratio of phospholipid to cholesterol, phospholipid to drug, phospholipid to DSPE-PEG200 as the investigated factors, whereas PS and EE of the PLLs were employed as the investigation indicators.
Table 1 Factor level table of the orthogonal test
Level |
Phospholipid : cholesterol |
Phospholipid : polydatin |
Phospholipid : DSPE-PEG2000 |
10 : 1 |
10 : 1 |
6 : 1 |
10 : 1 |
8 : 1 |
8 : 1 |
5 : 1 |
8 : 1 |
6 : 1 |
6 : 1 |
4 : 1 |
6 : 1 |
2.6 Characterization of the PLLs
2.6.1 Measurement of PS, EKP and polydispersed index (PDI).
The PLLs were diluted with purified water, and then the PS, EKP and PDI of the diluted solution were measured with the laser PS analyzer, Nano Brook 90 Plus.
2.6.2 Estimation of EE and DL.
In brief, a 0.22 μm microporous membrane was applied for the separation of free and encapsulated drugs in the PLLs. Then, the PLLs that were obtained before and after filtration were diluted with methanol. The solution was filtered with a 0.22 μm microporous membrane, before injection of 20 μL samples to HPLC according to the established liquid phase method. The peak area of the drug was measured and substituted into the standard curve equation to calculate the EE of the PLLs in vitro. | 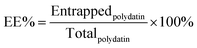 | (3) |
| 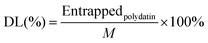 | (4) |
wherein Entrappedpolydatin is the content of polydatin in the filtered solution, and Totalpolydatin means the total content of polydatin in the preparation, whilst the total amount of PLLs was denoted by M.
2.6.3 Stability study.
The storage stability study of the PLLs was evaluated by storing them at 4 °C and 25 °C for 30 days. The DL%, PS, PDI and EE% of the preparations were determined after 0, 15 and 30 days, respectively.
2.7 Cumulative release testing in vitro
The cumulative release of raw materials and PLL in different buffer media was tested by the dialysis bag method. The buffer media were PBS (pH 6.8 and 7.4), purified water (pH 7.0) and HCl–pH 1.2. The specific operation method was as follows: 1 mg of API or PLLs was added into a 3500D dialysis bag, while the two ends were fastened and then placed in a triangular bottle containing 100 mL of the media. The triangular flask was placed in a water bath that has been set at a constant temperature of 37 °C and oscillating at 100 rpm. Aliquots (1 mL) of release solution were collected from four triangular bottles and placed into a centrifuge tube at different time points (0.167, 0.333, 0.5, 0.75, 1, 1.5, 2, 3, 4, 6, 8, 10, 12, 24, 36, and 48 hours), whereas 1 mL of fresh release medium (37 °C) was added simultaneously into the triangular bottle. The samples were centrifuged at 10
000 rpm for 10 min, the supernatant was detected according to the established chromatographic conditions. Ultimately, the cumulative drug release rate could be calculated in vitro. The origin was used to fit the release kinetics equation of the in vitro release results.
2.8 Cell uptake test in vitro
To evaluate the cellular uptake of PLL, HCT116 cells were used as a model cell. The cells were seeded at a density of 1 × 105 mL−1, 0.1 mL per well in 96-well plates and cultured in McCoy's 5A and 10% fetal bovine serum for 24 hours at 37 °C with 5% CO2. The medium was replaced with fresh medium containing PLL loaded with coumarin (fluorescent dye) at 10 μg mL−1. The cells were further incubated for 1, 2, 4, 6, 12, and 24 hours under the same conditions. After each time point, the cells were washed 3 times with cold phosphate-buffered saline (PBS) to remove any unbound PLL. The cells were then lysed with 1% Triton X-100 for 45 min and observed under a fluorescence microscope.
2.9 Pharmacokinetic experiment in vivo
2.9.1 Oral test method in rats.
To compare the oral bioavailability of free polydatin and PLL, healthy male SD (with average weight of 200 ± 20 g) rats (supplied by Jiangsu University Animal Research and Experimental Center, Zhenjiang, China) were randomly divided into two groups (n = 5). One group received an oral dose of free polydatin (200 mg kg−1), while the other group received an oral dose of PLL (200 mg kg−1).55 The rats were fasted for 12 hours before the experiment and had limited drinking water. Blood samples were collected from the ophthalmic veins at different time points (0.083, 0.25, 0.5, 0.75, 1, 1.5, 2, 3, 4, 6, 8, 10, 12, and 16 hours) after dosing. The blood samples were centrifuged at 3700 rpm for 10 minutes to obtain plasma. The plasma samples were processed according to Section 2.9.1 and analyzed by HPLC according to Section 2.3. The pharmacokinetic parameters were calculated using DAS software (version 3.2.6).
2.9.2 Plasma sample treatment.
Plasma samples were obtained by centrifuging blood samples collected from mice at 3700 rpm for 10 minutes. The supernatant (100 μL) was transferred to another tube, and mixed with 800 μL of ethyl acetate and 50 μL of astragaloside solution by vortexing for 30 seconds. The mixture was centrifuged again at 10
000 rpm for 10 minutes, whereas the organic layer was transferred to a clean tube. The extraction was repeated with another 800 μL of fresh ethyl acetate. The combined organic layers were evaporated to dryness under a stream of nitrogen at 37 °C. The residue was reconstituted in 100 μL of chromatographic grade methanol and vortexed for 30 seconds. The solution was filtered through a 0.22 μm membrane and 20 μL was injected into the HPLC system for analysis.
2.10 Hypoglycemic experiment in vivo
To evaluate the hypoglycemic effect of free polydatin and PLLs, six-week-old male C57bl/6J mice were randomly divided into model and control groups and were maintained in a specific pathogen-free environment for one week. The fasting blood glucose of all mice was measured prior to the experiment. The enrolled mice were required to have a fasting blood glucose less than 7 mmol L−1, while the mice with congenital abnormal blood glucose were excluded. After weighing the mice, their toes were cut and numbered. They were divided into 10 groups according to the random number table. The groupings were A: blank group, B: model group, C: blank liposome group (equivalent to blank liposome in PLL), D: positive drug group (metformin), E: high dose group of polydatin raw material (200 mg kg−1), F: medium dose group of polydatin raw material (100 mg kg−1), G: low dose group of polydatin raw material (50 mg kg−1), H: high dose group of PLLs (200 mg kg−1), I: medium dose group of PLLs (100 mg kg−1), and J: low dose group of PLLs (50 mg kg−1). The blank group was fed with 11 kcal% fat Surwit Diet (Research Diets Company, article number: D12329). The other groups were fed with 60 kcal% fat (Research DIETS, article number: D12492). Mice fed and drank freely. The mice were fed the HFT regime for 8 weeks to construct a model of type 2 diabetes, while the body weight of the mice was measured regularly.56 The mice whose body weight was not up to the standard were excluded. The screening criteria were x ± s of the blank group whose body weight was greater than or equal to the standard. Those who were not up to the standard suggested tolerance or insensitivity to obesity induction and were eliminated. After 8 weeks, the blank and model groups were administered with normal saline, whereas the other groups were administered according to the requirements of group administration.
2.11 ELISA reagent kit test physical and chemical indicators
After 8 weeks, the mice were killed, before collection of blood from the tail of the mice to measure the glucose level in the bloodstream through the blood glucose meter. Later, blood samples (0.5 mL) were taken from orbital veins and placed in EP tubes containing heparin sodium, prior to centrifugation at 3700 rpm for 10 minutes to collect the upper plasma. The levels of MDA, SOD, GSH-Px, TNF-α, TC, TG, HDL-C and LDL-C in the plasma were tested in strict accordance with the standard operating procedures of the ELISA kit. Briefly, 100 μL of plasma sample or standard solution was added to each well of a 96-well plate pre-coated with specific antibodies. After incubation at 37 °C for 30 minutes, the wells were washed three times with washing buffer and 100 μL of biotinylated detection antibody was added to each well. After another incubation at 37 °C for 30 minutes, the wells were washed again and 100 μL of horseradish peroxidase (HRP)-conjugated streptavidin was added to each well. After incubation at 37 °C for 20 minutes, the wells were washed and 100 μL of substrate solution was added to each well. The color reaction was stopped by adding 50 μL of stop solution and the optical density (OD) was measured at 450 nm using a microplate reader. The concentration of each analyte in the plasma samples was calculated from the standard curve generated by the standard solutions.
2.12 HE staining
The liver, spleen and pancreas of mice were collected and fixed in 4% paraformaldehyde solution for 24 hours at 4 °C. The fixed tissues were dehydrated, cleared and embedded in paraffin blocks. Sections of 5 μm thickness were cut and mounted on glass slides. The sections were deparaffinized in xylene and rehydrated in a graded ethanol solution. Afterward, the sections were differentiated in 1% acid alcohol for 10 seconds and rinsed again with water. The sections were blued in ammonia water for 30 seconds and washed with water. Next, the sections were stained with eosin for 2 minutes and dehydrated in graded ethanol solutions. Later, the sections were cleared in xylene and mounted with neutral balsam. The stained sections were observed and photographed under a light microscope.
2.13 Statistical analysis
Data were represented by mean ± standard deviation. Student's t-test was used to compare the differences between the two groups. One-way analysis of variance (ANOVA) followed by Tukey's post hoc test was used to compare the differences among multiple groups. A p-value of less than 0.05 was considered statistically significant. All statistical analyses were performed using SPSS software version 26.0.
3. Results and discussion
3.1 HPLC analysis results
The UV full wavelength scanning results showed that polydatin had maximum absorption at a wavelength of 306 nm (Fig. 1(a)). Therefore, 306 nm was selected as the detection wavelength to determine the polydatin concentration using the HPLC analytical method. The in vivo and in vitro retention times of polydatin were around 10.08 minutes, wherein the peak had good shape without any interference (Fig. 1(b)–(d)). The retention time of the internal standard was 14.67 minutes, which indicates that the two peaks of polydatin and the internal standard were well separated, while endogenous substances in the body did not interfere with the analysis and detection of polydatin. Therefore, this chromatographic method was considered as suitable for the determination of the in vivo and in vitro content of polydatin. These results are consistent with previous studies that have used HPLC to measure the concentration of polydatin in different samples. Hence, the HPLC method is a reliable and accurate technique for quantifying polydatin and evaluating its pharmacokinetics and bioavailability.
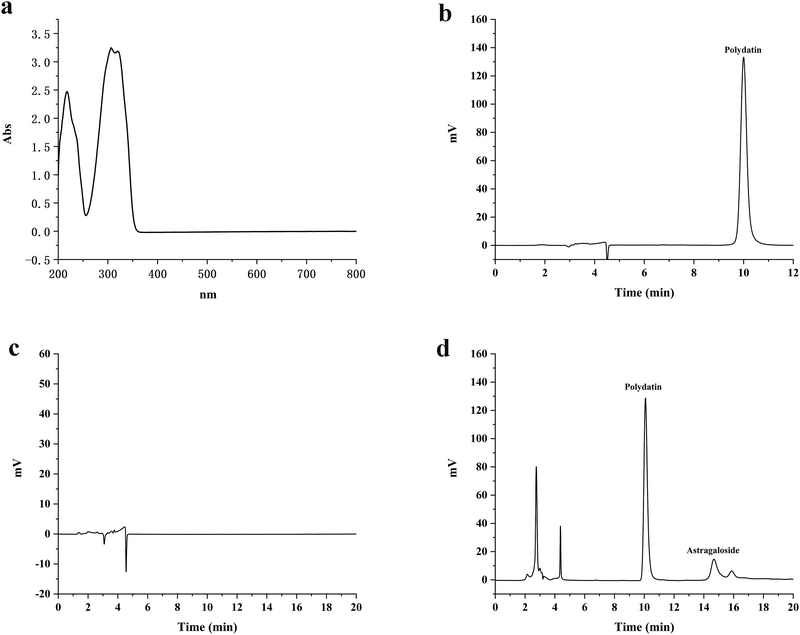 |
| Fig. 1 Polydatin analysis: (a) full wavelength ultraviolet scanning diagram of polydatin. (b) In vitro HPLC chromatogram of polydatin. (c) HPLC chromatogram of a rat blank blood sample. (d) HPLC chromatogram of a polydatin, astragaloside, and rat blank blood sample mixture. | |
3.2 Equilibrium solubility
The findings of the equilibrium solubility experiment demonstrated that polydatin solubility in all four media was relatively low (Table 2). Among them, the solubility in pH 6.8 PBS solution (712.82 ± 7.82 μg mL−1) was slightly higher than that in pH 1.2 HCl solution (676.22 ± 5.18 μg mL−1), and pH 7.4 PBS solution (562.56 ± 4.18 μg mL−1), wherein a slight difference in solubility was observed after comparison with water (701.28 ± 4.39 μg mL−1). Based on the above results, polydatin belongs to the category of insoluble drugs, which may be attributed to its molecular structure.57 The low solubility of polydatin is a major challenge for its oral administration and bioavailability. Previous studies have also reported similar findings on the poor solubility of polydatin in various media.35 Therefore, this study aimed to improve the solubility of polydatin through preparation methods, thereby increasing its in vivo bioavailability and efficacy. Therefore, this study aimed to improve the solubility of polydatin through preparation methods, thereby increasing its in vivo bioavailability and efficacy.
Table 2 The equilibrium solubility of polydatin in different media (n = 3)
Media |
Hydrochloric acid (pH 1.2) |
Phosphate buffer solution (PBS, pH 6.8) |
Water |
PBS (pH 7.4) |
Solubility (μg mL−1) |
676.22 ± 5.18 |
712.82 ± 7.82 |
701.28 ± 4.39 |
562.56 ± 4.18 |
3.3 Optimization testing of the PLL preparation
The orthogonal design experiment was carried out to optimize the formulation of PLL with the ratio of phospholipid to cholesterol, phospholipid to drug, and phospholipid to DSPE-PEG200 as the investigation factors, wherein PS and EE of PLL were used as the investigation indicators (Table 3). Based on the EE and PS results of each group of formulations, it could be seen that the optimal formula was as follows: phospholipid
:
cholesterol = 8
:
1; phospholipid
:
drug = 10
:
1; and phospholipid
:
DSPE-PEG200 = 5
:
1. Of note, the optimal formula was selected according to the principle that EE should be high and PS should be small.58 The ratio of phospholipid to cholesterol affects the fluidity and stability of the liposome membrane,59 whilst the ratio of phospholipid to drug affects the drug loading capacity and release behavior of the liposomes.41 Also, the ratio of phospholipid to DSPE-PEG200 affects the surface properties and circulation time of the liposomes.60 Thus, the orthogonal design experiment is a useful method for screening out the best combination of factors from multiple levels.61 More importantly, the PLL was developed with the aim of prolonging the circulation of polydatin to enhance its ability to prevent diabetes induced by HFT and STZ. In this regard, cholesterol was selected to increase liposomal fluidity and stability as well as alteration of the rigidity of the liposomal bilayer.62,63 Also, lecithin as a major natural source of phospholipid was chosen because it serves as an emulsifier and can potentially enhance the penetration of other components.64 With regards to DSPE-PEG2000, it was selected based on its potential to prolong the circulation time of drugs in the blood.65 Thus, we selected these components after the optimization process for the development of polydatin into long-circulating liposomes based on the above-mentioned criteria.
Table 3 Orthogonal design experiment table
Test no. |
Level of factor |
PS (nm) |
EE (%) |
Phospholipid : cholesterol |
Phospholipid : polydatin |
Phospholipid : DSPE-PEG200 |
PS: particle size, EE: encapsulation efficiency. |
1 |
10 : 1 |
10 : 1 |
6 : 1 |
156.81 ± 4.39 |
67.39 ± 1.18 |
2 |
10 : 1 |
8 : 1 |
5 : 1 |
165.16 ± 4.17 |
81.49 ± 1.62 |
3 |
10 : 1 |
6 : 1 |
4 : 1 |
204.62 ± 6.64 |
82.98 ± 1.85 |
4 |
8 : 1 |
10 : 1 |
5 : 1 |
107.15 ± 5.43 |
93.31 ± 1.86 |
5 |
8 : 1 |
8 : 1 |
4 : 1 |
131.61 ± 4.18 |
91.16 ± 2.17 |
6 |
8 : 1 |
6 : 1 |
6 : 1 |
148.41 ± 6.14 |
90.41 ± 2.01 |
7 |
6 : 1 |
10 : 1 |
4 : 1 |
203.82 ± 6.79 |
89.32 ± 1.98 |
8 |
6 : 1 |
8 : 1 |
6 : 1 |
189.87 ± 5.17 |
93.21 ± 2.17 |
9 |
6 : 1 |
6 : 1 |
5 : 1 |
213.86 ± 5.61 |
92.72 ± 2.13 |
3.4 Characterization of the PLLs
The average PS of the PLLs was 107.15 ± 5.43 nm, with a single and narrow particle size distribution (as shown in Fig. 2(a)). The EKP of the PLLs was −23.19 ± 0.18 mV, indicating that the liposomes had relatively good stability. As indicated by the TEM image, particles of the PLLs showed spherical shapes with a small size, which was consistent with the DLS results. In addition, the prepared liposomes showed high EE and DL, which were 93.31 ± 1.86% and 7.21 ± 0.12%, respectively. These results demonstrate that PLLs were successfully prepared with desirable characteristics for drug delivery. Smaller PS is important for drug delivery in vivo, as it can enhance the penetration and accumulation of liposomes in target tissues.66 Higher EE and DL can also improve the drug loading efficiency and reduce the waste of drugs.67 Negative EKP can reduce the aggregation and clearance of liposomes in the blood circulation.68 Besides, spherical shape can facilitate the interaction and uptake of liposomes by cells.69
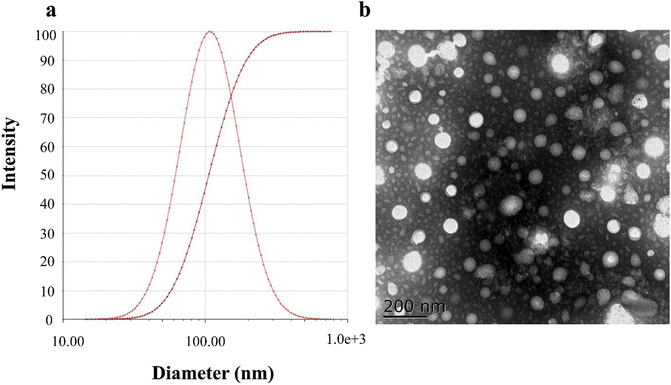 |
| Fig. 2 Characterization of polydatin long-circulating liposomes (PLLs). (a) Particle size diagram of the PLLs. (b) TEM image of the PLLs. | |
3.5 Stability study
After storage at 25 °C and 4 °C for 1 month, the drug content, EE, PS, and PDI of the PLLs were estimated (Table 4). The average PS slightly increased from 107.23 ± 4.19 nm to 109.55 ± 4.17 nm and from 107.15 ± 5.43 nm to 110.46 ± 6.42 nm at 4 °C and 25 °C, respectively. After one month of storage under these two conditions, the EE and drug content of the PLLs were still relatively high, with EE higher than 92% and drug content still above 6.9%. These results indicated that the physical stability of the PLLs was good at 4 and 25 °C for 30 days. The relatively good stability of the PLLs could be ascribed to the increased encapsulation of polydatin in the core of the PLLs and the protective effect of DSPE-PEG200 on the surface of the liposomes.70 The molecular weight and chain length of PEG are closely related to the stability of the liposomes, while the optimal PEG chain length for prolonging the circulation time of the liposomes is between 1000 and 2000.51,71 Liposomes should be stable at physiological temperatures to prevent release of drugs prematurely in vivo.72 Thus, to confirm the stability of the PLLs, the formulation should be stored in the long-term (about 12 months) at various conditions of temperature and relative humidity.
Table 4 Stability study (n = 3)
Time (days) |
Drug content (%) |
EE (%) |
PS (nm) |
PDI |
4 °C |
25 °C |
4 °C |
25 °C |
4 °C |
25 °C |
4 °C |
25 °C |
PS: particle size, EE: encapsulation efficiency, PDI: polydispersed index. |
0 |
7.19 ± 0.11 |
7.21 ± 0.12 |
93.27 ± 1.17 |
93.31 ± 1.86 |
107.23 ± 4.19 |
107.15 ± 5.43 |
0.241 ± 0.011 |
0.242 ± 0.013 |
15 |
7.21 ± 0.18 |
7.11 ± 0.22 |
92.67 ± 1.26 |
92.18 ± 1.98 |
108.39 ± 4.28 |
109.42 ± 5.82 |
0.245 ± 0.011 |
0.251 ± 0.021 |
30 |
7.16 ± 0.26 |
6.91 ± 0.33 |
92.43 ± 1.12 |
92.01 ± 2.31 |
109.55 ± 4.17 |
110.46 ± 6.12 |
0.251 ± 0.014 |
0.253 ± 0.017 |
3.6
In vitro release testing
To maintain, the sink volume of a poorly soluble drug like polydatin, it is important that the dissolution volume should be selected to ensure that the drug concentration should remain low relative to its solubility. In this case, 100 mL of each of the four media was selected to ensure accurate and reproducible results. The cumulative release rates of free polydatin and the PLLs in the above-mentioned four media are shown in Fig. 3. The experimental results showed that in pH 7.4 PBS, the cumulative release rate of both free polydatin and PLLs was slower than that of HCl, (pH 6.8), and water. In the same pH release media, similar rates of cumulative release of free polydatin and PLLs after 72 h were observed. However, the PLLs exhibited certain sustained-release characteristics in all four media. These findings indicate that PLLs could slowly release polydatin in vitro, which might result in maintaining a high blood drug concentration in vivo for a long time, thereby prolonging the circulation time of the polydatin in vivo, increasing in vivo bioavailability and the therapeutic efficacy of polydatin. Previous studies have also shown that DSPE-PEG2000 modified liposomes could have a certain sustained-release effect compared to free drugs.73,74 The fitting results of the in vitro release kinetics equation are shown in Table 5. By comparing the R2 values of the three equations, it was found that the release process of the active ingredient of polydatin in all four media conforms to the first-order release equation fitting, while the release process of the PLL in pH 1.2 and pH 7.4 media conforms to the Higuchi release equation fitting. The release process in pH 6.8 and DDW media conforms to the first-order release equation fitting. This result indicates that the PLL has a sustained-release effect.
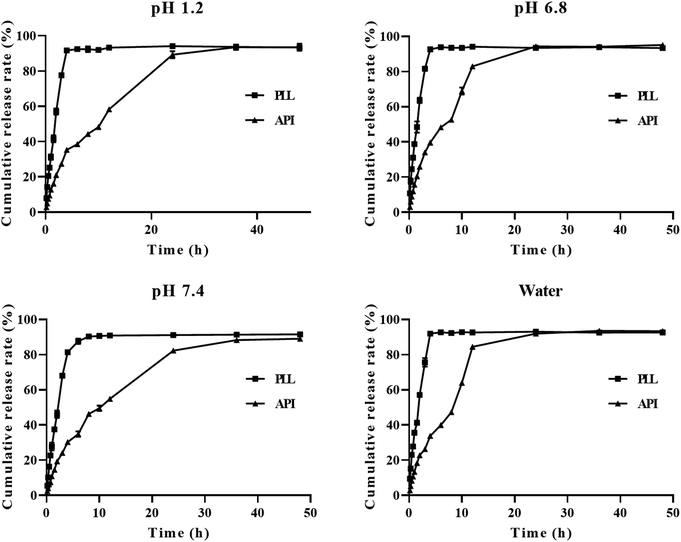 |
| Fig. 3 Cumulative release in vitro of polydatin long-circulating liposomes (PLLs) in different media (hydrochloric acid–pH 1.2, phosphate buffer solution (PBS–pH 6.8), PBS–pH 7.4, water). | |
Table 5 Results of in vitro release kinetics fitting equations
Polydatin |
Fitted equation |
PLLs |
Fitted equation |
pH 1.2 |
M
t = 1.521t + 38.567 R2 = 0.439 |
pH 1.2 |
M
t = 2.925t + 8.077 R2 = 0.768 |
M
t = 94.409(1 − e−0.501t) R2 = 0.993 |
M
t = 79.993(1 − e−0.133t) R2 = 0.952 |
M
t = 13.063x1/2 + 22.538 R2 = 0.657 |
M
t = 17.047x1/2 − 4.571 R2 = 0.987 |
pH 6.8 |
M
t = 0.662t + 75.985 R2 = 0.094 |
pH 6.8 |
M
t = 2.504t + 8.086 R2 = 0.743 |
M
t = 94.477(1 − e−0.605t) R2 = 0.988 |
M
t = 94.284(1 − e−0.161t) R2 = 0.990 |
M
t = 8.492x1/2 + 57.149 R2 = 0.657 |
M
t = 17.705x1/2 − 3.099 R2 = 0.931 |
DDW |
M
t = 1.332t + 47.137 R2 = 0.282 |
DDW |
M
t = 2.105t + 8.094 R2 = 0.855 |
M
t = 92.300(1 − e−0.540t) R2 = 0.993 |
M
t = 94.176(1 − e−0.126t) R2 = 0.994 |
M
t = 12.942x1/2 + 28.811 R2 = 0.657 |
M
t = 15.467x1/2 − 2.227 R2 = 0.958 |
pH 7.4 |
M
t = 2.116t + 37.009 R2 = 0.421 |
pH 7.4 |
M
t = 2.895t + 4.993 R2 = 0.993 |
M
t = 92.338(1 − e−0.389t) R2 = 0.996 |
M
t = 65.744(1 − e−0.199t) R2 = 0.921 |
M
t = 17.242x1/2 + 15.187 R2 = 0.723 |
M
t = 16.465x1/2 − 5.257 R2 = 0.989 |
3.7 Cell uptake test experiment
To efficiently and accurately image the uptake of fluorescently labelled drugs using fluorescent spectroscopy, it is important that the cells are lysed to break their cell membranes in order to access the intracellular components.75 The results of the in vitro cell uptake experiment are shown in Fig. 4. It could be observed that with the prolongation of time, the fluorescence intensity inside HCT116 cells significantly weakened and the cell uptake substantially decreased. This suggested that over time, the uptake of liposome nanocarrier systems by HCT116 cells decreased. This result might be explained by the fact that PLLs were internalized by HCT116 cells through endocytosis, and then released polydatin into the cytoplasm through endosomal escape or lysosomal degradation.76 As more polydatin was released from the PLLs, less fluorescence was detected inside the cells. This result also implied that PLLs could effectively deliver polydatin into HCT116 cells, which might prospectively enhance the anticancer activity of the stilbenoid glucoside. However, this preliminary study did not investigate the influence of liposomal size and variations in PS on cellular uptake of the PLLs. Also, the mechanism of PLL uptake by HCT116 cells was not investigated in this study. More importantly, the intracellular fate after the PLLs had been trafficked and their toxicity have not been studied. In view of the importance of the above-mentioned parameters for drug discovery, our future experiments will comprehensively investigate these variables.
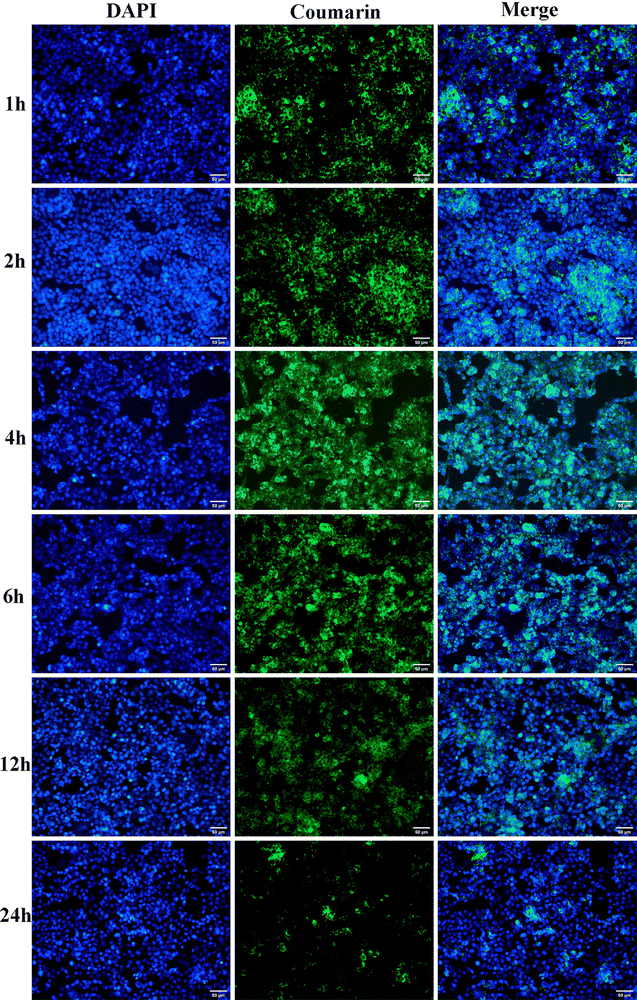 |
| Fig. 4 Results of the in vitro cell uptake experiment. | |
3.8 Pharmacokinetics experiment
Compared to free polydatin, the plasma drug concentration of PLL was higher at most time points (Fig. 5). The pharmacokinetic parameters in rats (Table 6) showed that compared to free polydatin (T1/2 = 1.02 ± 0.07 h, and MRT = 3.32 ± 1.20 h), the in vivo T1/2 (5.15 ± 0.47 h) and MRT (12.02 ± 2.28 h) of the PLLs were significantly longer, which indicates that the PLLs prolonged the circulation time of polydatin in vivo, which may be beneficial for enhancing the therapeutic effect of polydatin. Moreover, compared with free polydatin (AUC0-t = 3.58 ± 0.14 h mg mL−1), the AUC0-t of the PLLs significantly increased (13.03 ± 0.30 h mg mL−1, P < 0.01) with about 372.50% of Fr, thereby suggesting that the PLLs improved the bioavailability of polydatin. These results demonstrated that long-circulating liposomes could significantly increase the peak concentration of polydatin in the blood, improve the bioavailability of polydatin, and prolong the circulation time of polydatin in vivo. The long-circulating liposomal system has been suggested to increase the bioavailability of lipophilic drugs like polydatin by extending the time of blood circulation of drugs via reduction of their uptake by the mononuclear phagocytic system (MPS).77 The enhanced pharmacokinetics of the PLLs could be attributed to the effects of lecithin, cholesterol, and DSPE-PEG2000 on the liposome membrane. Lecithin, as a component of lipid membranes, had a high affinity with cell membranes and could reduce the protein binding level of liposomes in the human body, thereby prolonging the drug circulation time, promoting drug absorption, and improving drug bioavailability.78 Cholesterol could increase the rigidity and stability of the liposome membrane and prevent drug leakage.79 DSPE-PEG2000 can prevent the adsorption and adhesion of liposomes by proteins, antibodies, cells, etc., and cover the hydrophobic part of the phospholipid molecules, so that particles can avoid the combination with blood components, and avoid the recognition and phagocytosis of MPS, thus prolonging the internal circulation time.45 Among the currently marketed liposomes, there are also many formulations that have used DSPE-MPEG2000 as an excipient to improve the pharmacokinetics of drugs.80 One of the significant approaches to improve bioavailability is through targeted distribution of drugs to tissues and sites of diseases. In this regard, our subsequent experiments will investigate approaches to ideally target PLL delivery using specific ligands to modify the surface of the liposome for its enhanced retention in tissues.
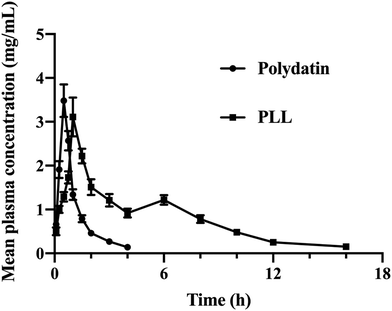 |
| Fig. 5 Profiles of the drug concentration–time curve in the plasma of rats after administration of free polydatin and polydatin long-circulating liposomes (PLLs). | |
Table 6 Pharmacokinetic parameters of free polydatin and polydatin long-circulating liposomes (PLLs) (mean ± SD, n = 5)
Parameters |
Free polydatin |
PLLs |
P < 0.01, compared with free polydatin.
|
C
max (mg mL−1) |
3.48 ± 0.37 |
3.11 ± 0.44 |
T
max (h) |
0.5 ± 0 |
1 ± 0 |
AUC0-t (h mg mL−1) |
3.58 ± 0.14 |
13.03 ± 0.30a |
T
1/2 (h) |
1.02 ± 0.07 |
5.15 ± 0.47a |
MRT (h) |
3.32 ± 1.20 |
12.02 ± 2.28a |
3.9 Hypoglycemic effect of PLLs
3.9.1 Blood glucose, AUC of the OGTT level and weight in mice.
As shown in Fig. 6, it could be intuitively seen that compared with the blank control group, the blood glucose level and AUC of OGTT in the model group significantly increased (P < 0.01), thus indicating successful establishment of a hyperglycemic animal model. The blood glucose and AUC of the OGTT levels in the blank formulation group were like those in the model group, which suggests that the excipients in the PLLs had no effect on these parameters. In comparison with the model group, the mice in the positive drug group showed a significant decrease in blood glucose and AUC of the OGTT levels (P < 0.01), thus demonstrating the excellent hypoglycemic effect of metformin. It was discovered that the blood glucose and AUC of the OGTT levels in mice decreased significantly (P < 0.01) after the administration of free polydatin and PLLs compared to the model group. Specifically, as the dosage of polydatin increased, the reducing effect of free polydatin and PLLs on blood glucose as well as the AUC of the OGTT levels in mice gradually increased, thereby showing a dose dependent relationship. In addition, compared with the free polydatin group, the blood glucose and AUC of the OGTT levels in the PLL group decreased further (P < 0.05 or P < 0.01). Among them, high-dose PLL had the strongest hypoglycemic effect compared to the model group, thereby respectively reducing blood glucose and the AUC of the OGTT levels in mice by about 40.8% and 15.9% (PLL high-dose group) as well as roughly 16.5% and 12.2% (model group). This indicates that polydatin may regulate the uptake and utilization of glucose in hyperglycemic mice, thereby reducing their blood sugar levels and increasing their OGTT levels. It could be seen that polydatin improved the glucose tolerance of hyperglycemic mice, while PLLs enhanced this effect, further improving the blood glucose regulatory function of mice.
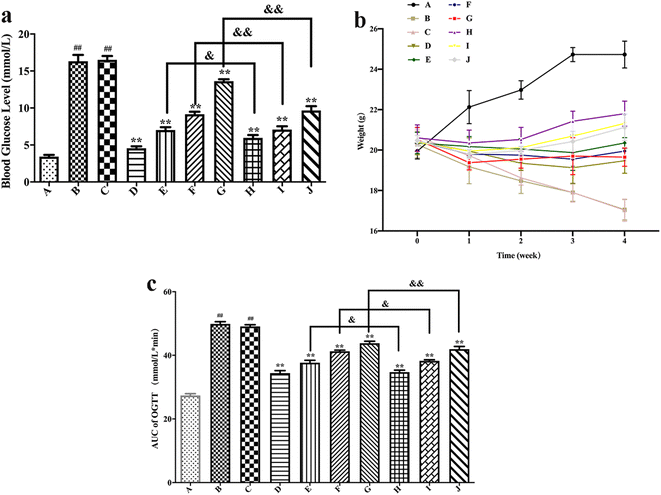 |
| Fig. 6 Effect of free polydatin and polydatin long-circulating liposomes (PLLs) on blood glucose levels, body weight, and glucose tolerance AUC in mice with high-fat diet induced hyperglycemia: (a) blood glucose levels in each group. (b) Body weight of mice in each group at different times. (c) Glucose tolerance AUC of mice in each group. Statistical significance was indicated as follow: ##P < 0.01 (compared with blank control group), *P < 0.05 (compared with model control group), **P < 0.01 (compared with model control group), &P < 0.05 (compared with free polydatin group), &&P < 0.01 (compared with free polydatin group). A: blank group, B: model group, C: blank liposome group, D: positive drug group, E: high dose group of polydatin raw material, F: medium dose group of polydatin raw material, G: low dose group of polydatin raw material, H: high dose group of PLLs, I: medium dose group of PLLs, J: low dose group of PLLs. | |
The body weights of different groups of mice are shown in Fig. 6(b). It could be seen from the figure that the weight of the blank control group mice slowly increased over time, while after the induction of HFT, the mice showed a typical weight loss phenomenon of hyperglycemia. The weight of the blank formulation group mice decreased over time, whereas the situation was like that of the model group. A comparison of the model group with the positive drug group showed that the body weight of mice that received positive drug slowly increased over time. Compared with the model group, the weight of the mice increased slowly with time after free polydatin and PLL administration, which indicates that polydatin could alleviate weight loss caused by hyperglycemia. Moreover, the body weight of PLL mice was slightly higher than that of the free polydatin and positive drug groups, thus implying that PLL enhanced the effect of polydatin. The effect of polydatin and PLLs may be a preventive mechanism against diabetes through mitigation of damage to beta cells of the pancreas via its antioxidant property.81 In view of the susceptibility of beta cells of the pancreas to endogenously ROS-triggered oxidative stress coupled with very low antioxidant enzymes levels, protection of the function of the beta cells with natural compounds that possess antioxidant properties may be the best strategy for diabetes treatment.82 Hence, the mechanism underlying the protective effect of PLLs on pancreatic beta cells will be investigated in our not-too distant future works. More importantly, the potential of PLLs to target beta cells of the pancreas will be studied in detail.
3.9.2 Detection of physical and chemical indicators in mice.
This study further determined whether polydatin had the effect of regulating related enzymes. The SOD is an important antioxidant enzyme system in biological systems, while GSH is a widely present peroxidase lyase, and MDA is a product of the lipid peroxidation reaction.83 TNF-α has been proven to be involved in the occurrence and development of diabetes.84 Studies have revealed that TNF-α upregulated the expression of sterol regulatory element binding protein 1 in both liver cells and sebaceous gland cells, thus leading to intracellular lipid accumulation.85
As shown in Fig. 7, compared with the blank control group, the model group mice had significantly increased MDA and TNF-α levels (P < 0.01), while the SOD and GSH-Px levels substantially decreased (P < 0.01), thus suggesting successful establishment of a hyperglycemic animal model. Blank formulation group mice had similar levels of MDA, TNF-α, SOD and GSH-Px as the model group, thus indicating that the excipients in the PLLs had no effect on these parameters. Compared with the model group, the positive drug group mice showed a significant decrease in MDA and TNF-α (P < 0.01), while the SOD and GSH-Px levels substantially increased (P < 0.01), thus suggesting that metformin could effectively regulate the antioxidant enzymes of hyperglycemic mice. Compared with the model group, treatment with free polydatin and PLL significantly reduced the MDA and TNF-α levels (P < 0.01), but substantially increased the SOD and GSH-Px levels (P < 0.05 or P < 0.01), wherein the findings showed a dose dependence. This result indicates that polydatin had a certain effect on regulating antioxidant enzymes in hyperglycemic mice. The results are consistent with previous studies.86,87 It was speculated that polydatin may have a hypoglycemic effect by improving the level of oxidative stress in vivo. In addition, compared with the free polydatin group, the PLL group displayed a further decrease in MDA and TNF-α levels (P < 0.05 or P < 0.01), as well as a further increase in SOD and GSH-Px levels (P < 0.05 or P < 0.01). This observation indicates that PLL could significantly enhance antioxidant capacity and inhibit lipid peroxidation compared to free polydatin. Overall, the high-dose PLL showed the strongest effect.
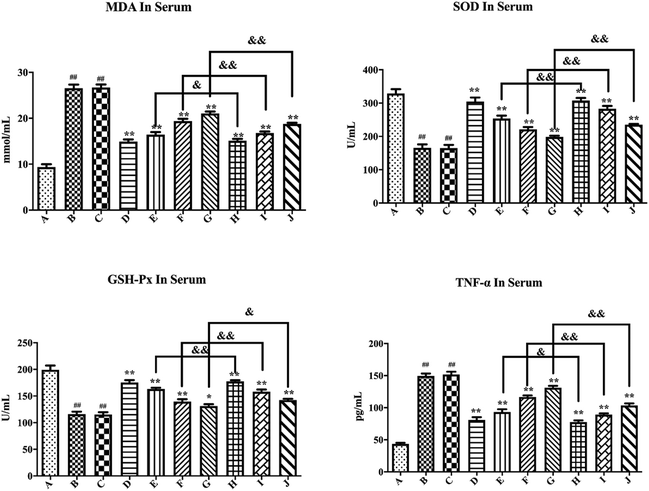 |
| Fig. 7 Effect of free polydatin and polydatin long-circulating liposomes (PLL) on plasma SOD, GSH-Px, MDA and TNF-α levels in mice with high-fat diet-induced hyperglycemia. Statistical significance is indicated as follows: ##P < 0.01 (compared with blank control group), *P < 0.05 (compared with model control group), **P < 0.01 (compared with model control group), &P < 0.05 (compared with free polydatin group), &&P < 0.01 (compared with free polydatin group). SOD: superoxide dismutase, GSH-Px: glutathione peroxidase, MDA: malondialdehyde, TNF-α: tumor necrosis factor-α. A: blank group, B: model group, C: blank liposome group, D: positive drug group, E: high dose group of polydatin raw material, F: medium dose group of polydatin raw material, G: low dose group of polydatin raw material, H: high dose group of PLLs, I: medium dose group of PLLs, J: low dose group of PLLs. | |
As shown in Fig. 8, compared with the blank control group, the model group demonstrated significantly increased serum TC, TG, and LDL-C levels (P < 0.01), while the HDL-C levels significantly reduced (P < 0.01), further confirming the successful establishment of a hyperglycemic mouse model. The blank formulation group had similar serum TC, TG, HDL-C, and LDL-C levels as the model group, thus indicating that the inactive components in the PLLs had no effect on these parameters. Compared with the model group, the positive drug group displayed substantially reduced serum TC, TG, and LDL-C levels (P < 0.01), while the HDL-C levels significantly increased (P < 0.01), thus suggesting that metformin could significantly reduce blood lipid levels in hyperglycemic mice. Compared with the model group, free polydatin and PLL administration markedly reduced the serum TC, TG, and LDL-C levels (P < 0.01), but significantly increased the HDL-C levels (P < 0.01). The blood lipid levels showed a dose-dependent effect. Specifically, high doses of free polydatin and PLLs had more significant lipid-lowering effects than low doses. This result indicates that polydatin could reduce blood lipid levels in hyperglycemic mice. More importantly, compared with the free polydatin group, the PLL group further reduced the serum levels of TC, TG, and LDL-C (P < 0.05 or P < 0.01), but the HDL-C levels increased further (P < 0.01). The data showed that compared to the model group, the serum TC, LDL-C, and TG levels were respectively reduced by about 38.3%, 63.2%, and 37.4% in the low-dose PLL group, but the serum HDL-C levels increased by about 145.1%. The respective serum TC, LDL-C, and TG levels decreased by approximately 28.7%, 46.4%, and 29.8% in the same dose of free polydatin, but the HDL-C levels increased by approximately 69.4%. This discovery indicates that the lipid-lowering effect of the PLLs was more significant than that of free polydatin. Zhou et al.88 also showed that polydatin treatment significantly improved blood glucose, serum ALT, AST, TC, TG, LDL-C and HDL-C levels, as well as changes in MDA, SOD and miR-214 content in the liver tissue (P < 0.05), thereby reducing the blood glucose and lipid levels in atherosclerosis mice and protecting liver function. In addition, related studies have shown that polydatin could inhibit the increase in the levels of ROS, activity of NADPH oxidase, and production of in vitro and in vivo hyperglycemic-induced inflammatory cytokines.89
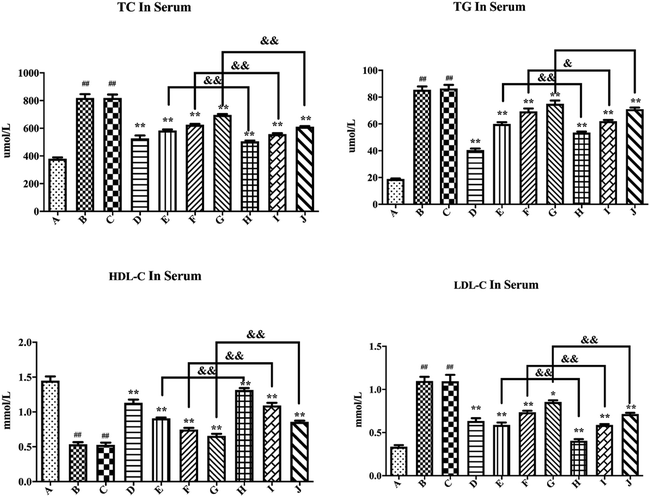 |
| Fig. 8 Effect of free polydatin and polydatin long-circulating liposomes (PLLs) on the plasma lipid profile level in mice with high-fat diet-induced hyperglycemia. Statistical significance is indicated as follows: ##P < 0.01 (compared with blank control group), *P < 0.05 (compared with model control group), **P < 0.01 (compared with model control group), &P < 0.05 (compared with free polydatin group), &&P < 0.01 (compared with free polydatin group.) TG: triglyceride, TC: total cholesterol, LDL-C: low-density lipoprotein cholesterol, HDL-C: reduction in high-density lipoprotein cholesterol. A: blank group, B: model group, C: blank liposome group, D: positive drug group, E: high dose group of polydatin raw material, F: medium dose group of polydatin raw material, G: low dose group of polydatin raw material, H: high dose group of PLLs, I: medium dose group of PLLs, J: low dose group of PLLs. | |
The enhanced efficacy may be attributed to the drug being encapsulated in long-circulating liposomes, which could slowly release the drug in the body and prolong its retention time in the body. In general, the reduction of the PS of nanoparticles could improve the rate of drugs’ dissolution by increasing the surface area, which could increase the drugs’ absorption in the gastro-intestinal tract and be conducive to the drug's effectiveness.90 Moreover, it might be because the PEG molecules in DSPE-PEG2000 could form hydrogen bonds with the aqueous phase, thus forming a hydration membrane structure on the liposomal surface to reduce the binding of liposomes with proteins, enzymes, and other components in plasma, thereby enhancing its stability in the blood.48,91 In addition, phospholipids could increase the solubility of drugs through their amphiphilic surfactants’ properties and their wetting and dispersing effects.92 Better solubility may play an important role in improving polydatin bioavailability and efficacy. In general, nanoparticles that have increased aqueous solubility are well absorbed into the blood circulation after oral delivery, which may result in quick action and possibly heightened the efficacy of the encapsulated drug. In recent times, scientists have explored delivery of liposomal insulin and other lead diabetic compounds via the oral route.93 Shafiq and colleagues successfully developed fat globule membrane-based liposomes to orally deliver insulin to diabetic rats, wherein the nanoparticles displayed substantial hypoglycemic performance in diabetic animals.94 Also, Zhang et al. fabricated biotinylated liposomes for oral delivery of insulin, amid increased absorption and bioavailability of the peptide.95
3.9.3 Pathological observation of each tissue in mice.
The HE staining diagram (Fig. 9(A)) showed that the liver cells of the blank control group mice had a clear structure, regular arrangement, uniform size, normal nuclear morphology and good cell status. On the contrary, the liver cells of the model group mice were irregularly arranged, swollen, contained abnormal unstained particles, had vacuoles of different sizes in the cytoplasm gap, and showed severe tissue damaged, steatosis and inflammatory cell infiltration, thus indicating successful modeling. The liver tissue morphology of the blank preparation group was like that of the model group, which implies that blank preparation had no effect on liver damage in mice. In the positive control group, and the free polydatin high-dose and PLL high-dose groups, the liver cell structure of the mice was significantly improved, with the cell arrangement being tidier than that of the model group. Also, cell swelling, and inflammatory cell infiltration were improved, whilst abnormal particles and necrotic cells were decreased. Again, almost no vacuolar degeneration of hepatocytes was observed, amid being close to the normal liver cell tissue morphology. The results indicate that polydatin had a significant alleviating effect on HFT-induced liver injury in rats.
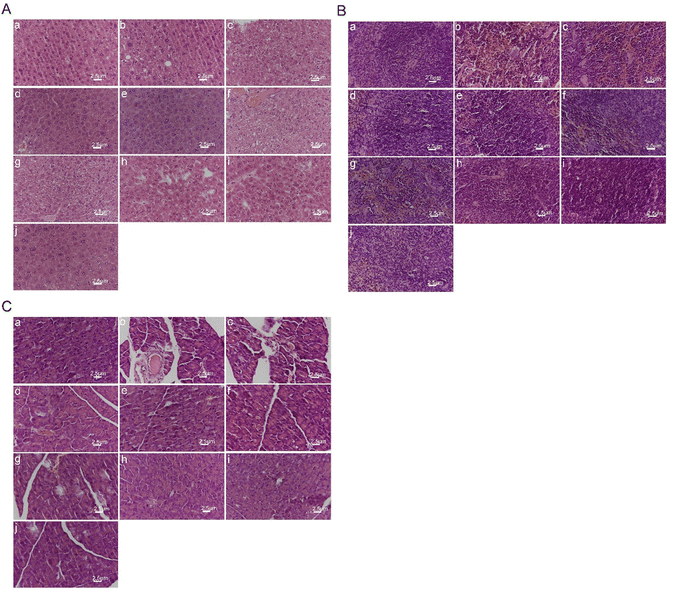 |
| Fig. 9 Pathological observation of the livers (A), spleens (B) and pancreas (C) of mice in different groups. a: blank group, b: model group, c: blank liposome group, d: positive drug group, e: high dose group of polydatin raw material, f: medium dose group of polydatin raw material, g: low dose group of polydatin raw material, h: high dose group of polydatin long-circulating liposomes (PLLs), i: medium dose group of PLLs, j: low dose group of PLLs. | |
As shown in Fig. 9(B), the spleen tissue structure of the blank control group mice was normal. The spleen tissue membrane of the model group mice shrank with the structure at the boundary between red and white pulp being disordered, whilst the edge area became thinner. Besides, the number of splenic nodules decreased, whereas the number of silted red blood cells increased with a large amount of hemosiderin deposition, amid the arrangement and number of lymphocytes being sparse and reduced. The spleen status of the blank preparation group mice was like that of the model group. Compared with the model group, each medication group showed gradual improvement in membrane shrinkage with red and white pulp structure and hemosiderin deposition, as well as lymphocyte arrangement and number, and splenic nodule number. Damage to the mouse spleen tissue was alleviated, especially in the high-dose PLL group, which approached the level of the blank control group. The results indicate that polydatin had a significant alleviating effect on high-fat diet-induced spleen injury in rats.
The experimental results shown in Fig. 9(C) showed that the pancreatic tissue of the blank control group mice was normal, with normal pancreatic cell structure, and clear cell membrane. There were no abnormalities in the morphology and number of pancreatic islets. The pancreatic tissue in the model group was abnormal, with irregular atrophy of pancreatic islets and blurred boundaries. The acinar cells were loosely arranged and detached. The number of cells in the pancreatic islets decreased. Also, some cells showed obvious vacuolar degeneration and vascular basement membrane thickening in pancreatic islet stroma. The pancreatic tissue morphology of the blank preparation group was like that of the model group, thus indicating that the blank preparation had no effect on pancreatic tissue damage in hyperglycemic mice. Compared with the model group, each medication group showed improvement in pancreatic tissue cell arrangement, number of pancreatic islets, and reduction of pathological changes such as congestion, swelling, and glassy degeneration in cells. The pancreatic tissue damage was well recovered after treatment. The free polydatin and PLL dosage groups showed closer arrangement of pancreatic tissue and cells than the model group, and partial recovery of the pancreatic islets. The higher the dosage of polydatin, the more normal the pancreatic tissue morphology. Notably, the high-dose PLL group had normal pancreatic islet morphology, without any signs of pancreatic atrophy. The number of pancreatic islets was large, while the boundary was clear. The results suggest that polydatin demonstrated a substantial protective effect on the pancreas of hyperglycemic mice.
4. Conclusion
In this study, successful preparation of polydatin long circulating liposomes (PLLs) was accomplished using DSPE-PEG2000 as a modifier to enhance the solubility, bioavailability, and hypoglycemic effect of polydatin. An HPLC method was established to measure the concentration of polydatin in vitro and in vivo. Also, it was discovered that polydatin was a poorly soluble drug in different media. Furthermore, optimization of the formulation of PLL was performed using orthogonal design experiments to obtain PLLs with small and uniform PS, high EE and DL, and stability. Also, evaluation of the in vitro release, in vivo pharmacokinetics, and hypoglycemic activity of the PLLs showed that the PLLs could prolong the release of polydatin, increase its blood concentration and bioavailability, and improve the anti-diabetic effect on HFT-induced hyperglycemia in mice. Therefore, PLL is considered as a promising drug delivery system for polydatin and deserves further research and development.
Data availability
Data will be available upon request to the corresponding author.
Conflicts of interest
The authors declare that they have no conflicts of interest or personal relationships that could have appeared to influence the findings reported in this work.
Acknowledgements
This work was supported by A Double-blind Randomized Clinical Study on Multiple Center observing the Effect of Daixie Granule Combined with Metformin On Type 2 Diabetes with Obesity (SHDC2020CR3028A) and Mechanism of Daixie Granule Regulating Sp1 Transcription Factor to Improve Diabetes Obese Mice (Y21007).
References
- Y. Chen, C. Liang, J. Li, L. Ma, B. Wang, Z. Yuan, S. Yang and X. Nong, Effect of artesunate on cardiovascular complications in periodontitis in a type I diabetes rat model and related mechanisms, J. Endocrinol. Invest., 2023, 46(10), 2031–2053, DOI:10.1007/s40618-023-02052-0
.
- M. Gallego, J. Zayas-Arrabal, A. Alquiza, B. Apellaniz and O. Casis, Electrical Features of the Diabetic Myocardium. Arrhythmic and Cardiovascular Safety Considerations in Diabetes, Front. Pharmacol., 2021, 12, 687256 CrossRef CAS
.
- J. B. Buse, M. H. Tan, M. J. Prince and P. P. Erickson, The effects of oral anti-hyperglycaemic medications on serum lipid profiles in patients with type 2 diabetes, Diabetes, Obes. Metab., 2004, 6(2), 133–156 CrossRef CAS
.
- H. Sun, P. Saeedi, S. Karuranga, M. Pinkepank, K. Ogurtsova, B. B. Duncan, C. Stein, A. Basit, J. C. N. Chan, J. C. Mbanya, M. E. Pavkov, A. Ramachandaran, S. H. Wild, S. James, W. H. Herman, P. Zhang, C. Bommer, S. Kuo, E. J. Boyko and D. J. Magliano, IDF Diabetes Atlas: Global, regional and country-level diabetes prevalence estimates for 2021 and projections for 2045, Diabetes Res. Clin. Pract., 2022, 183, 109119 CrossRef
.
- J. Cheng, X. Zhou, J. Lu, X. Guo and L. Ji, Trends and regional differences in glycemic control of patients with type 2 diabetes in China, 2009–2013, Chin. Med. J., 2022, 135(13), 1637–1638 CrossRef PubMed
.
- T. Mallick, A. Karmakar, A. Mukhuty, C. Fouzder, J. Mandal, S. Mondal, A. Pramanik, R. Kundu and N. A. Begum, Exploring the Propensities of Fluorescent Carbazole Analogs toward the Inhibition of Amyloid Aggregation in Type 2 Diabetes: An Experimental and Theoretical Endeavor, J. Phys. Chem. B, 2021, 125(37), 10481–10493 CrossRef CAS
.
- P. A. Hart, D. K. Andersen, M. S. Petrov and M. O. Goodarzi, Distinguishing diabetes secondary to pancreatic diseases from type 2 diabetes mellitus, Curr. Opin. Gastroenterol., 2021, 37(5), 520–525 CrossRef CAS
.
- S. I. Taylor, Z. S. Yazdi and A. L. Beitelshees, Pharmacological treatment of hyperglycemia in type 2 diabetes, J. Clin. Invest., 2021, 131(2), e142243, DOI:10.1172/JCI142243
.
- E. Ferrannini and R. A. DeFronzo, Impact of glucose-lowering drugs on cardiovascular disease in type 2 diabetes, Eur. Heart J., 2015, 36(34), 2288–2296 CrossRef CAS
.
- J. M. Forbes and M. E. Cooper, Mechanisms of diabetic complications, Physiol. Rev., 2013, 93(1), 137–188 CrossRef CAS
.
- C. M. Apovian, J. Okemah and P. M. O’Neil, Body Weight Considerations in the Management of Type 2 Diabetes, Adv. Ther., 2019, 36(1), 44–58 CrossRef PubMed
.
- Y. Liu, M. Si, L. Tang, S. Shangguan, H. Wu, J. Li, P. Wu, X. Ma, T. Liu and Y. Hu, Synthesis and biological evaluation of novel benzyl-substituted (S)-phenylalanine derivatives as potent dipeptidyl peptidase 4 inhibitors, Bioorg. Med. Chem., 2013, 21(18), 5679–5687 CrossRef CAS PubMed
.
- N. Katsiki, E. Ferrannini and C. Mantzoros, New American Diabetes Association (ADA)/European Association for the Study of Diabetes (EASD) guidelines for the pharmacotherapy of type 2 diabetes: Placing them into a practicing physician's perspective, Metabolism, 2020, 107, 154218 CrossRef CAS
.
- T. D. Filippatos, T. V. Panagiotopoulou and M. S. Elisaf, Adverse Effects of GLP-1 Receptor Agonists, Rev. Diabet. Stud., 2014, 11(3–4), 202–230 CrossRef PubMed
.
- Z. X. He, Z. W. Zhou, Y. Yang, T. Yang, S. Y. Pan, J. X. Qiu and S. F. Zhou, Overview of clinically approved oral antidiabetic agents for the treatment of type 2 diabetes mellitus, Clin. Exp. Pharmacol. Physiol., 2015, 42(2), 125–138 CrossRef CAS PubMed
.
- Y. Jin and R. Arroo, The protective effects of flavonoids and carotenoids against diabetic complications-A review of in vivo evidence, Front. Nutr., 2023, 10, 1020950 CrossRef PubMed
.
- Z. Q. Kang, J. L. Hu, M. Y. Chen, Y. Mao, L. F. Xie, N. Yang, T. Liu, W. Zhang and W. H. Huang, Effects of Capsaicin on the Hypoglycemic Regulation of Metformin and Gut Microbiota Profiles in Type 2 Diabetic Rats, Am. J. Chin. Med., 2022, 50(3), 839–861 CrossRef CAS PubMed
.
- J. Wang, J. Zhao, Y. Yan, D. Liu, C. Wang and H. Wang, Inhibition of glycosidase by ursolic acid: in vitro, in vivo and in silico study, J. Sci. Food Agric., 2020, 100(3), 986–994 CrossRef CAS PubMed
.
- L. N. Xu, L. H. Yin, Y. Jin, Y. Qi, X. Han, Y. W. Xu, K. X. Liu, Y. Y. Zhao and J. Y. Peng, Effect and possible mechanisms of dioscin on ameliorating metabolic glycolipid metabolic disorder in type-2-diabetes, Phytomedicine, 2020, 67, 153139 CrossRef CAS
.
- X. Chen, C. Chen and X. Fu, Hypoglycemic effect of the polysaccharides from Astragalus membranaceus on type 2 diabetic mice based on the “gut microbiota-mucosal barrier”, Food Funct., 2022, 13(19), 10121–10133 RSC
.
- J. Ke, M. T. Li, S. Xu, J. Ma, M. Y. Liu and Y. Han, Advances for pharmacological activities of Polygonum cuspidatum – A review, Pharm. Biol., 2023, 61(1), 177–188 CrossRef
.
- H. Zhang, C. Li, S. T. Kwok, Q. W. Zhang and S. W. A. Chan, Review of the Pharmacological Effects of the Dried Root of Polygonum cuspidatum (Hu Zhang) and Its Constituents, J. Evidence-Based Complementary Altern. Med., 2013, 2013, 208349 Search PubMed
.
- Q. H. Du, C. Peng and H. Zhang, Polydatin: a review of pharmacology and pharmacokinetics, Pharm. Biol., 2013, 51(11), 1347–1354 CrossRef CAS PubMed
.
- N. Pang, T. Chen, X. Deng, N. Chen, R. Li, M. Ren, Y. Li, M. Luo, H. Hao, J. Wu and L. Wang, Polydatin Prevents Methylglyoxal-Induced Apoptosis through Reducing Oxidative Stress and Improving Mitochondrial Function in Human Umbilical Vein Endothelial Cells, Oxid. Med. Cell. Longevity, 2017, 2017, 7180943 Search PubMed
.
- H. B. Liu, Q. H. Meng, C. Huang, J. B. Wang and X. W. Liu, Nephroprotective Effects of Polydatin against Ischemia/Reperfusion Injury: A Role for the PI3K/Akt Signal Pathway, Oxid. Med. Cell. Longevity, 2015, 2015, 362158 Search PubMed
.
- F. Mostafa, A. Abdel-Moneim, M. Abdul-Hamid, S. R. Galaly and H. M. Mohamed, Polydatin and polydatin-loaded chitosan nanoparticles attenuate diabetic cardiomyopathy in rats, J. Mol. Histol., 2021, 52(2), 135–152 CrossRef CAS PubMed
.
- L. Zheng, J. Wu, J. Mo, L. Guo, X. Wu and Y. Bao, Polydatin Inhibits Adipose Tissue Inflammation and Ameliorates Lipid Metabolism in High-Fat-Fed Mice, BioMed Res. Int., 2019, 2019, 7196535 Search PubMed
.
- J. Hao, C. Chen, K. Huang, J. Huang, J. Li, P. Liu and H. Huang, Polydatin improves glucose and lipid metabolism in experimental diabetes through activating the Akt signaling pathway, Eur. J. Pharmacol., 2014, 745, 152–165 CrossRef CAS PubMed
.
- D. Ming, L. Songyan, C. Yawen, Z. Na, M. Jing, X. Zhaowen, L. Ye, D. Wa and L. Jie,
trans-Polydatin protects the mouse heart against ischemia/reperfusion injury via inhibition of the renin-angiotensin system (RAS) and Rho kinase (ROCK) activity, Food Funct., 2017, 8(6), 2309–2321 RSC
.
- L. Yu, Z. Li, X. Dong, X. Xue, Y. Liu, S. Xu, J. Zhang, J. Han, Y. Yang and H. Wang, Polydatin Protects Diabetic Heart against Ischemia-Reperfusion Injury via Notch1/Hes1-Mediated Activation of Pten/Akt Signaling, Oxid. Med. Cell. Longevity, 2018, 2018, 2750695 Search PubMed
.
- Q. Zhang, Y. Tan, N. Zhang and F. Yao, Polydatin supplementation ameliorates diet-induced development of insulin resistance and hepatic steatosis in rats, Mol. Med. Rep., 2015, 11(1), 603–610 CrossRef CAS PubMed
.
- M. Das and D. K. Das, Resveratrol and cardiovascular health, Mol. Aspects Med., 2010, 31(6), 503–512 CrossRef CAS
.
- C. Lv, L. Zhang, Q. Wang, W. Liu, C. Wang, X. Jing and Y. Liu, Determination of piceid in rat plasma and tissues by high-performance liquid chromatographic method with UV detection, Biomed. Chromatogr., 2006, 20(11), 1260–1266 CrossRef CAS
.
- X. Ding, X. Hou, S. Gao, M. Sun, F. Lin, G. Cai and K. Xiao, Pharmacokinetics and bioavailability study of polydatin in rat plasma by using a LC-MS/MS method, Pak. J. Pharm. Sci., 2014, 27(6), 1931–1937 CAS
.
- B. Liu, Y. Li, H. Xiao, Y. Liu, H. Mo, H. Ma and G. Liang, Characterization of the Supermolecular Structure of Polydatin/6-O–Maltosyl–cyclodextrin Inclusion Complex, J. Food Sci., 2015, 80(6), C1156–C1161 CrossRef CAS PubMed
.
- X. Wang, Q. Guan, W. Chen, X. Hu and L. Li, Novel nanoliposomal delivery system for polydatin: preparation, characterization, and in vivo evaluation, Drug Des., Dev. Ther., 2015, 9, 1805–1813 CAS
.
- B. Ceh, M. Winterhalter, P. M. Frederik, J. J. Vallner and D. D. Lasic, Stealth liposomes: from theory to product, Adv. Drug Delivery Rev., 1997, 24, 165–177 CrossRef CAS
.
- A. Abdel-Moneim, A. El-Shahawy, A. I. Yousef, S. M. Abd El-Twab, Z. E. Elden and M. Taha, Novel polydatin-loaded chitosan nanoparticles for safe and efficient type 2 diabetes therapy: In silico, in vitro and in vivo approaches, Int. J. Biol. Macromol., 2020, 154, 1496–1504 CrossRef CAS
.
- Y. Wei, Z. Xue, Y. Ye, Y. Huang and L. Zhao, Paclitaxel targeting to lungs by way of liposomes prepared by the effervescent dispersion technique, Arch. Pharmacal Res., 2014, 37(6), 728–737 CrossRef CAS PubMed
.
- D. Guimarães, A. Cavaco-Paulo and E. Nogueira, Design of liposomes as drug delivery system for therapeutic applications, Int. J. Pharm., 2021, 601, 120571 CrossRef PubMed
.
- G. Liu, S. Hou, P. Tong and J. Li, Liposomes: Preparation, Characteristics, and Application Strategies in Analytical Chemistry, Crit. Rev. Anal. Chem., 2022, 52(2), 392–412 CrossRef CAS PubMed
.
- Y. Wei and L. Zhao, Passive lung-targeted drug delivery systems via intravenous administration, Pharm. Dev. Technol., 2014, 19(2), 129–136 CrossRef CAS PubMed
.
- S. Deodhar and A. K. Dash, Long circulating liposomes: challenges and opportunities, Ther. Delivery, 2018, 9(12), 857–872 CrossRef CAS
.
- J. Zhou, X. Zang, J. Li, H. Hu, M. Qiao and X. Zhao, Preparation of glycyrrhetinic acid-targeted pH sensitive stealth doxorubicin liposomes and its pharmacokinetics, J. Shenyang Pharm. Univ., 2015, 32(8), 587–592 CAS
.
- Y. Chen, J. Chen, Y. Cheng, L. Luo, P. Zheng, Y. Tong and Z. A. Li, lyophilized sterically stabilized liposome-containing docetaxel: in vitro and in vivo evaluation, J. Liposome Res., 2017, 27(1), 64–73 CrossRef CAS
.
- V. D. Awasthi, D. Garcia, B. A. Goins and W. T. Phillips, Circulation and biodistribution profiles of long-circulating PEG-liposomes of various sizes in rabbits, Int. J. Pharm., 2003, 253(1–2), 121–132 CrossRef CAS
.
- M. L. Immordino, F. Dosio and L. Cattel, Stealth liposomes: review of the basic science, rationale, and clinical applications, existing and potential, Int. J. Nanomed., 2006, 1(3), 297–315 CrossRef CAS
.
- M. C. Woodle and D. D. Lasic, Sterically stabilized liposomes, Biochem. Biophys. Acta, 1992, 1113(2), 171–199 CAS
.
- D. Paolino, M. L. Accolla, F. Cilurzo, M. C. Cristiano, D. Cosco, F. Castelli, M. G. Sarpietro, M. Fresta and C. Celia, Interaction between PEG lipid and DSPE/DSPC phospholipids: An insight of PEGylation degree and kinetics of de-PEGylation, Colloids Surf., B, 2017, 155, 266–275 CrossRef CAS PubMed
.
- V. P. Torchilin, V. G. Omelyanenko, M. I. Papisov, A. A. Bogdanov, V. S. Trubetskoy, J. N. Herron and C. A. Gentry, Poly(ethylene glycol) on the liposome surface: on the mechanism of polymer-coated liposome longevity, Biochem. Biophys. Acta, 1994, 1195(1), 11–20 CAS
.
- D. Needham, T. J. McIntosh and D. D. Lasic, Repulsive interactions and mechanical stability of polymer-grafted lipid membranes, Biochem. Biophys. Acta, 1992, 1108(1), 40–48 CAS
.
- S. Y. Xu, H. Su, X. Y. Zhu, X. Y. Li, J. Li, X. Chen, Q. Wang, R. Y. Hao and X. Y. Yan, Long-circulating doxorubicin and schizandrin A liposome with drug-resistant liver cancer activity: preparation, characterization, and pharmacokinetic, J. Liposome Res., 2022, 32(2), 107–118 CrossRef CAS PubMed
.
- Q. Wang, W. Liu, J. Wang, H. Liu and Y. Chen, Preparation and Pharmacokinetic Study of Daidzein Long-Circulating Liposomes, Nanoscale Res. Lett., 2019, 14(1), 321 CrossRef PubMed
.
- P. Xiao, J. Zhao, Y. Huang, R. Jin, Z. Tang, P. Wang, X. Song, H. Zhu, Z. Yang and N. A. Yu, Novel Long-circulating DOX Liposome: Formulation and Pharmacokinetics, Studies, Pharm. Nanotechnol., 2020, 8(5), 391–398 CrossRef CAS
.
- Y. Chen, D. Q. Zhang, Z. Liao, B. Wang, S. Gong, C. Wang, M. Z. Zhang, G. H. Wang, H. Cai, F. F. Liao and J. P. Xu, Anti-oxidant polydatin (piceid) protects against substantia nigral motor degeneration in multiple rodent models of Parkinson's disease, Mol. Neurodegener., 2015, 10, 4 CrossRef
.
- O. F. Elabi, J. P. M. C. M. Cunha, A. Gaceb, M. Fex and G. Paul, High-fat diet-induced diabetes leads to vascular alterations, pericyte reduction, and perivascular depletion of microglia in a 6-OHDA toxin model of Parkinson disease, J. Neuroinflammation, 2021, 18(1), 175 CrossRef CAS PubMed
.
- Y. L. Liu, B. Y. Chen, J. Nie, G. H. Zhao, J. Y. Zhuo, J. Yuan, Y. C. Li, L. L. Wang and Z. W. Chen, Polydatin prevents bleomycin-induced pulmonary fibrosis by inhibiting the TGF-β/Smad/ERK signaling pathway, Exp. Ther. Med., 2020, 20(5), 62 CAS
.
- V. Kumar, R. Kharb and H. Chaudhary, Optimization & design of isradipine loaded solid lipid nanobioparticles using rutin by Taguchi methodology, Int. J. Biol. Macromol., 2016, 92, 338–346 CrossRef CAS PubMed
.
- P. Nakhaei, R. Margiana, D. O. Bokov, W. K. Abdelbasset, M. A. Jadidi Kouhbanani, R. S. Varma, F. Marofi, M. Jarahian and N. Beheshtkhoo, Liposomes: Structure, Biomedical Applications, and Stability Parameters With Emphasis on Cholesterol, Front. Bioeng. Biotechnol., 2021, 9, 705886, DOI:10.3389/fbioe.2021.705886
.
- G. N. C. Chiu, M. B. Bally and L. D. Mayer, Effects of phosphatidylserine on membrane incorporation and surface protection properties of exchangeable poly(ethylene glycol)-conjugated lipids, Biochim. Biophys. Acta, Biomembr., 2002, 1560(1–2), 37–50 CrossRef CAS PubMed
.
- Y.-X. Lei, J. Gou, C. Wang, W. Luo and Y.-Q. Cai, Improved Differential Evolution With a Modified Orthogonal Learning Strategy, IEEE Access, 2017, 5, 9699–9716 Search PubMed
.
- A. Laouini, C. Jaafar-Maalej, I. Limayem-Blouza, S. Sfar, C. Charcosset and H. J. Fessi, Biotechnology, Preparation, characterization and applications of liposomes: state of the art, J. Colloid Sci. Biotechnol., 2012, 1, 147–168 CrossRef CAS
.
- U. Bulbake, S. Doppalapudi, N. Kommineni and W. Khan, Liposomal formulations in clinical use: an updated review, Pharmaceutics, 2017, 9, 12 CrossRef
.
- D. Taladrid, D. Marín, A. Alemán, I. Álvarez-Acero, P. Montero and M. Gómez-Guillén, Effect of chemical composition and sonication procedure on properties of food-grade soy lecithin liposomes with added glycerol, Food Res. Int., 2017, 100, 541–550 CrossRef CAS PubMed
.
- D. Wang, Y. Wang, G. Zhao, J. Zhuang and W. Wu, Improving systemic circulation of paclitaxel nanocrystals by surface hybridization of DSPE-PEG2000, Colloids Surf., B, 2019, 182, 110337 CrossRef CAS
.
- H. Bshara, R. Osman, S. Mansour and H. El-Shamy Ael, Chitosan and cyclodextrin in intranasal microemulsion for improved brain buspirone hydrochloride pharmacokinetics in rats, Carbohydr. Polym., 2014, 99, 297–305 CrossRef CAS PubMed
.
- Z. L. Tyrrell, Y. Shen and M. Radosz, Near-Critical Fluid Micellization for High and Efficient Drug Loading: Encapsulation of Paclitaxel into PEG-b-PCL Micelles, J. Phys. Chem. C, 2011, 115(24), 11951–11956 CrossRef CAS
.
- H. Choudhury, B. Gorain, S. Karmakar, E. Biswas, G. Dey, R. Barik, M. Mandal and T. K. Pal, Improvement of cellular uptake, in vitro antitumor activity and sustained release profile with increased bioavailability from a nanoemulsion platform, Int. J. Pharm., 2014, 460(1–2), 131–143 CrossRef CAS
.
- L. Jia, J. Shen, D. Zhang, C. Duan, G. Liu, D. Zheng, X. Tian, Y. Liu and Q. Zhang,
In vitro and in vivo evaluation of oridonin-loaded long circulating nanostructured lipid carriers, Int. J. Biol. Macromol., 2012, 50(3), 523–529 CrossRef CAS
.
- D. D. Lasic, Novel applications of liposomes, Trends Biotechnol., 1998, 16(7), 307–321 CrossRef CAS
.
- Z. Liu, M. Chen and J. Zhang, Thermo sensitivity of polymeric liposomes, Chin. Pharm. J., 1997, 10, 19–21 Search PubMed
.
- B. M. Dicheva, A. L. Seynhaeve, T. Soulie, A. M. Eggermont, T. L. Ten Hagen and G. A. Koning, Pharmacokinetics, Tissue Distribution and Therapeutic Effect of Cationic Thermosensitive Liposomal Doxorubicin Upon Mild Hyperthermia, Pharmacogn. Res., 2016, 33(3), 627–638 CrossRef CAS
.
- H. Yan, P. Wei, J. Song, X. Jia and Z. Zhang, Enhanced anticancer activity in vitro and in vivo of luteolin incorporated into long-circulating micelles based on DSPE-PEG2000 and TPGS, J. Pharm. Pharmacol., 2016, 68(10), 1290–1298 CrossRef CAS PubMed
.
- M. Jiang, Y. Jin, G. Fang, J. Ren, L. Han, W. Chen, C. Peng and L. Liu, Preparation and in vitro release of paeonol-ozagrel conjugate long-circulating liposomes, Chin. J. New Drugs, 2019, 28(2), 202–207 Search PubMed
.
- Q. Seisel, F. Pelletier, S. Deshayes and P. Boisguerin, How to evaluate the cellular uptake of CPPs with fluorescence techniques: Dissecting methodological pitfalls associated to tryptophan-rich peptides, Biochim. Biophys. Acta, Biomembr., 2019, 1861(9), 1533–1545 CrossRef CAS PubMed
.
- M. M. Fretz, A. Høgset, G. A. Koning, W. Jiskoot and G. Storm, Cytosolic delivery of liposomally targeted proteins induced by photochemical internalization, Pharm. Res., 2007, 24(11), 2040–2047 CrossRef CAS
.
- J. S. Lee, S. Y. Hwang and E. K. Lee, Imaging-based analysis of liposome internalization to macrophage cells: effects of liposome size and surface modification with PEG moiety, Colloids Surf., B, 2015, 136, 786–790 CrossRef CAS
.
- B. Liu, Y. Wang and N. Du, Interactions between Layered Double Hydroxide Nanoparticles and Egg Yolk Lecithin Liposome Membranes, Molecules, 2023, 28(9), 3929, DOI:10.3390/molecules28093929
.
- N. Oku and Y. Namba, Long-circulating liposomes, Crit. Rev. Ther. Drug Carrier Syst., 1994, 11(4), 231–270 CAS
.
- S. Geng, B. Yang, G. Wang, G. Qin, S. Wada and J.-Y. Wang, Two cholesterol derivative-based PEGylated liposomes as drug delivery system, study on pharmacokinetics and drug delivery to retina, Nanotechnology, 2014, 25(27), 275103, DOI:10.1088/0957-4484/25/27/275103
.
- A. I. Yousef, H. H. Shawki, A. A. El-Shahawy, S. M. A. El-Twab, A. Abdel-Moneim and H. Oishi, Polydatin mitigates pancreatic β-cell damage through its antioxidant activity, Biomed. Pharmacother., 2021, 133, 111027 CrossRef CAS
.
- J. Pi, Q. Zhang, J. Fu, C. G. Woods, Y. Hou, B. E. Corkey, S. Collins and M. E. Andersen, ROS signaling, oxidative stress and Nrf2 in pancreatic beta-cell function, Toxicol. Appl. Pharmacol., 2010, 244(1), 77–83 CrossRef CAS
.
- J. Su, P. Zhang, J. J. Zhang, X. M. Qi, Y. G. Wu and J. J. Shen, Effects of total glucosides of paeony on oxidative stress in the kidney from diabetic rats, Phytomedicine, 2010, 17(3–4), 254–260 CrossRef CAS PubMed
.
- M. Endo, T. Masaki, M. Seike and H. Yoshimatsu, TNF-alpha induces hepatic steatosis in mice by enhancing gene expression of sterol regulatory element binding protein-1c (SREBP-1c), Exp. Biol. Med., 2007, 232(5), 614–621 CAS
.
- J. J. Choi, M. Y. Park, H. J. Lee, D. Y. Yoon, Y. Lim, J. W. Hyun, C. C. Zouboulis and M. Jin, TNF-α increases lipogenesis via JNK and PI3K/Akt pathways in SZ95 human sebocytes, J. Dermatol. Sci., 2012, 65(3), 179–188 CrossRef CAS PubMed
.
- H. L. Wang, J. P. Gao, Y. L. Han, X. Xu, R. Wu, Y. Gao and X. H. Cui, Comparative studies of polydatin and resveratrol on mutual transformation and antioxidative effect in vivo, Phytomedicine, 2015, 22(5), 553–559 CrossRef CAS PubMed
.
- Y. Wang, J. Ye, J. Li, C. Chen, J. Huang, P. Liu and H. Huang, Polydatin ameliorates lipid and glucose metabolism in type 2 diabetes mellitus by downregulating proprotein convertase subtilisin/kexin type 9 (PCSK9), Cardiovasc. Diabetol., 2016, 15, 19 CrossRef PubMed
.
- F. H. Zhou, Z. Y. Wen, Z. H. He, M. Li, Q. L. Yin, C. G. Shi and C. L. Cheng, [Effect of polydatin on miR-214 expression and liver function in ApoE−/− mice], J. South. Med. Univ., 2016, 36(6), 763–767 CAS
.
- Y. Y. Tan, L. X. Chen, L. Fang and Q. Zhang, Cardioprotective effects of polydatin against myocardial injury in diabetic rats via inhibition of NADPH oxidase and NF-κB activities, BMC Complementary Med. Ther., 2020, 20(1), 378 CrossRef CAS PubMed
.
- K. Hu, S. Cao, F. Hu and J. Feng, Enhanced oral bioavailability of docetaxel by lecithin nanoparticles: preparation, in vitro, and in vivo evaluation, Int. J. Nanomed., 2012, 7, 3537–3545 CrossRef CAS
.
- G. Blume and G. Cevc, Liposomes for the sustained drug release in vivo, Biochem. Biophys. Acta, 1990, 1029(1), 91–97 CAS
.
- X. Yanyu, S. Yunmei, C. Zhipeng and P. Qineng, The preparation of silybin-phospholipid complex and the study on its pharmacokinetics in rats, Int. J. Pharm., 2006, 307(1), 77–82 CrossRef PubMed
.
- C. Y. Wong, H. Al-Salami and C. R. Dass, Recent advancements in oral administration of insulin-loaded liposomal drug delivery systems for diabetes mellitus, Int. J. Pharm., 2018, 549(1–2), 201–217 CrossRef CAS PubMed
.
- S. Shafiq, M. Siddiq Abduh, F. Iqbal, K. Kousar, S. Anjum and T. Ahmad, A novel approach to insulin delivery via oral route: Milk fat globule membrane derived liposomes as a delivery vehicle, Saudi J. Biol. Sci., 2024, 31(3), 103945 CrossRef CAS
.
- X. Zhang, J. Qi, Y. Lu, W. He, X. Li and W. Wu, Biotinylated liposomes as potential carriers for the oral delivery of insulin, Nanomedicine, 2014, 10(1), 167–176 CrossRef CAS PubMed
.
Footnote |
† These authors contributed equally to this work. |
|
This journal is © The Royal Society of Chemistry 2024 |
Click here to see how this site uses Cookies. View our privacy policy here.