DOI:
10.1039/D4NJ04061A
(Paper)
New J. Chem., 2024,
48, 18066-18074
Syntheses and coordination chemistry of thiosemicarbazone-based titanium complexes†
Received
17th September 2024
, Accepted 26th September 2024
First published on 27th September 2024
Abstract
Two routes leading to thiosemicarbazone-based complexes are reported with the view of developing ionic titanium complexes as cytotoxic metallodrugs. The reaction of bis(π-η5:σ-η1-pentafulvene)titanium complexes with most thiosemicarbazones gives κ2N,S thiosemicarbazonido complexes via deprotonation of the acidic N–H bond by the pentafulvene ligand. The use of an o-cresyl TSCN revealed a κ2N,O coordination mode by deprotonation of the O–H bond. The protonolysis of the remaining pentafulvene unit in these complexes with Brønsted acids and further functionalization with multiple bond substrates were attempted. The reaction of titanocene(III) triflate with TSCN reveals an unprecedented reactivity and leads to Ti(III) thiosemicarbazone complexes. The Ti(IV) complexes were characterized using NMR experiments and the nitrogen cores were identified via1H,15N HMBC experiments by coupling with the adjacent aldimine proton or the methyl group of the thiosemicarbazone. These are the first structural examples of thiosemicarbazone-based titanium complexes obtained from single-crystal X-ray diffraction.
Introduction
Thiosemicarbazones (TSCN) are an important type of compound in both chemistry and medicine because of their role as ligands in coordination chemistry1–4 and their pharmacological properties.2–5 They count as Schiff bases and can be synthesized accordingly in a condensation reaction of a thiosemicarbazide with an aldehyde or a ketone.6 In metal complexes, a series of different coordination modes have been observed, such as the κ1S,7,8 κ2N,S9 and κ3N,S,X10,11 (X = additional donor) coordination modes (Fig. 1, top), while also multimetallic bonding is possible.7,11,12 The κ2N,S coordination mode often resembles a five-membered ring system, where the Nα atom coordinates, which is considered more stable than the respective four-membered ring.13 Although less common, there are also examples of the four-membered κ2Nβ,S coordination mode.13,14 While TSCN complexes are present in catalysis,15 their main purpose lie in the pharmacological and biological applications.16,17 TSCN show antimicrobial,18 antiviral19 and, predominantly, antitumoral activities.20 For instance, the TSCN Triapine is currently in medical trials as a potential antitumoral agent.21 By metalation, the biological activities of TSCN increase significantly,22 which is why much of recent research focuses on TSCN metal complexes.17,23 As Cisplatin and its derivatives are the standout examples of antitumoral metal complexes,24 many related studies were centered around heavier metals.4,25 Titanium-based metal complexes also have shown promising results regarding cytotoxic properties,26 but despite their promises, less attention has been paid to them and only a couple of works feature TSCN as ligands in neutral complexes.27 In this work, we developed a facile route toward Ti(IV) TSCN complexes from bis(pentafulvene) complexes (Fig. 1, bottom left) and discussed the role of an additional donor in the ligand backbone.
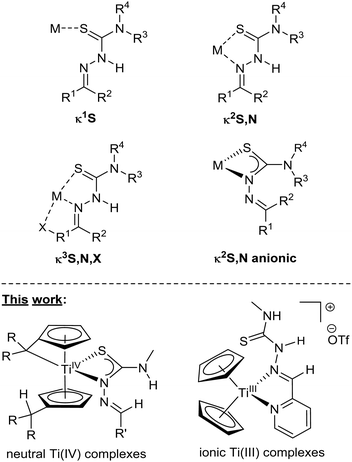 |
| Fig. 1 Common coordination modes of TSCN complexes (top). Examples of TSCN-based Ti(IV) and Ti(III) complexes obtained in this work (bottom). | |
Because of the multifaceted molecular bond activation28 and functionalization29,30 reactivity of bis(pentafulvene) complexes, they are excellent precursors for the creation of novel metallodrugs, while the adamantyl groups of one of the complexes is an interesting feature because of the biological properties of adamantane derivatives.31 This work also features 15N NMR values for the nitrogen cores within the TSCN and the first molecular structures of thiosemicarbazone-based titanium complexes obtained from single-crystal X-ray diffraction. First attempts have been made to obtain ionic complexes, either by protonation of mono(pentafulvene) titanium complexes or via ligand exchange of titanocene(III) triflate (Fig. 1, bottom right).32
Results and discussion
Reaction of TSCN with Bis(pentafulvene)titanium complexes
Thiosemicarbazones a–d were synthesized according to a general procedure via condensation of 4-methylthiosemicarbazide and the corresponding aldehyde.33 While a has no additional donor site, b–d contain heterocycles such as thiophenyl (b) and pyridinyl (c) rests. Thiosemicarbazone d provides a donor site via the OH group of the o-cresyl rest and there is also potential for deprotonation of this group (Fig. 2). Our initial studies focused on thiosemicarbazones derived from thiosemicarbazide. Although this approach works in principle, the poor solubility of the TSCN in the commonly used solvents for bis(pentafulvene)titanium complexes (n-hexane, toluene, THF) led to incomplete reactions and therefore to an inferior purity of the obtained complexes. Therefore, we used TSCN based on 4-methylthiosemicarbazide because of the superior solubility, reactivity and higher purity of the obtained complexes.
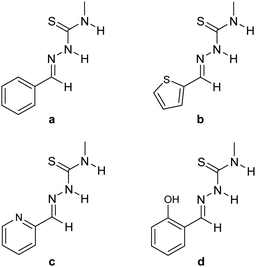 |
| Fig. 2 Scope of thiosemicarbazone ligands a–d used in this work. | |
Reactions of bis(π-η5:σ-η1-pentafulvene)titanium complexes Ti1 and Ti2 with the thiosemicarbazones a–c yielded the κ2N,S-thiosemicarbazonate complexes Ti1a–c and Ti2a,bvia deprotonation of the acidic Nβ-proton by one pentafulvene unit (Scheme 1). The reaction of Ti2 with c resulted in a mixture of products, which was not further characterized.
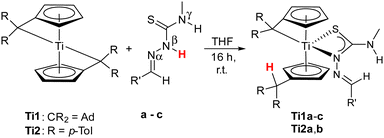 |
| Scheme 1 Reaction of bis(π-η5:σ-η1-pentafulvene)titanium complexes Ti1 and Ti2 with thiosemicarbazones a–c to κ2Nβ,S-thiosemicarbazonate titanium complexes Ti1a–c and Ti2a,b. | |
The products Ti1a–c and Ti2a,b were characterized by NMR spectroscopy and additionally, Ti1a, Ti1c and Ti2b by single-crystal X-ray diffraction (Fig. 3–5). Despite the multifunctionality of the thiosemicarbazone ligands, only the Nβ-proton was deprotonated as evident by the eight different signals for the Cp-protons of the resulting complexes in the 1H NMR spectra, corresponding to asymmetrical ring systems.30 The 15N NMR shifts of the nitrogen cores were determined via1H,15N-HMBC experiments, with values between 207.0 and 213.3 ppm for the Nα atom, 341.3 and 354.6 ppm for the Nβ atom by coupling with the respective aldimine protons and values between 87.6 and 88.4 ppm for the Nγ atom via coupling with the adjacent methyl group. The signal of the pyridinyl nitrogen core of Ti1c has a chemical shift of 316.8 ppm, which corresponds to a non-coordinating pyridine.34 This was also revealed by the molecular structure obtained by single-crystal X-ray diffraction (Fig. 5).
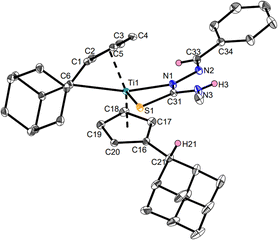 |
| Fig. 3 Molecular structure of complex Ti1a. Displacement ellipsoids are drawn at the 50% probability level. Redundant H atoms (apart from H3, H21 and H33) have been omitted for clarity. Selected bond lengths (Å) and angles (deg): Ti1–N1 2.1839(5), Ti1–S1 2.58107(19), Ti1–C6 2.744, N1–N2 1.3843(6), N2–C33 1.2906(7), N1–C31 1.3354(7), N3–C31 1.3409(7), S1–C31 1.7175(5), N1–Ti1–S1 63.823(13), N1–C31–S1 112.09(4), Ti1–N1–N2 139.81(4), C31–N1–N2 114.93(4), Ct1–Ti1–Ct2 136.7 (Ct1 = centroid of C1–C5; Ct2 = centroid of C16–C20). | |
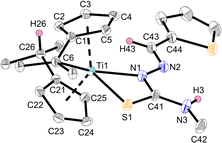 |
| Fig. 4 Molecular structure of complex Ti2b. Displacement ellipsoids are drawn at the 50% probability level. Redundant H atoms (apart from H3, H26 and H43) have been omitted for clarity. Selected bond lengths (Å) and angles (deg): Ti1–N1 2.1750(10), Ti1–S1 2.5798(4), Ti1–C6 2.7556, N1–C41 1.3338(15), S1–C41 1.7165(12), N3–C41 1.3423(15), C1–C6 1.4275(15), N1–N2 1.3785(13), N2–C43 1.2876(16), Ti1–C6–C1 53.570, S1–Ti1–N1 63.99(3), C41–N1–Ti1 104.32(7), C41–S1–Ti1 79.21(4), Ct1–Ti–Ct2 136.0 (Ct1 = centroid of C1–C5; Ct2 = centroid of C21–C25). | |
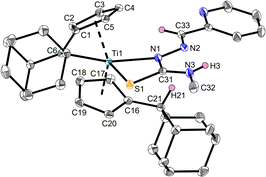 |
| Fig. 5 Molecular structure of complex Ti1c. Displacement ellipsoids are drawn at the 50% probability level. Redundant H atoms (apart from H3, H26 and H43) have been omitted for clarity. Selected bond lengths (Å) and angles (deg): Ti1–N1 2.2222(15), Ti1–S1 2.5823(5), Ti1–C6 2.7516(18), N1–N2 1.374(2), N2–C33 1.286(2), N1–C31 1.349(2), N3–C31 1.329(2), S1–C31 1.7118(18), N2–H21 2.383, N1–Ti1–S1 63.28(4), N1–C31–S1 111.56(13), Ti1–N1–N2 142.32(12), C31–N1–N2 113.55(14), Ct1–Ti1–Ct2 135.1 (Ct1 = centroid of C1–C5; Ct2 = centroid of C16–C20). | |
The molecular structure of Ti1c shows a mono(pentafulvene)titanium complex with an anionic, four-membered κ2Nβ,S-thiosemicarbazonido ligand. The Ti1–N1 bond with 2.2222(15) Å corresponds to an elongated Ti–N single bond according to the sum of covalent radii (Σrcov(Ti–N) = 2.07 Å),35 while the Ti1–S1 bond (2.5823(5) Å) is an elongated Ti–S single bond (Σrcov(Ti–S) = 2.39 Å).35 This bonding situation is due to the negative charge of the thiosemicarbazonido ligand, which is shared across the N and S atoms. Therefore, both the N1–C31 bond (1.349(2) Å) and the S1–C31 bond (1.7118(18) Å) contain double bond character (Σrcov(C–N) = 1.46 Å, Σrcov(C
N) = 1.27 Å; Σrcov(C–S) = 1.78 Å, Σrcov(C
S) = 1.61 Å).36 This structural feature is common for κ2Nβ,S-thiosemicarbazonido complexes.13 The rather long Ti–Cexo bond of the remaining fulvene moiety (Ti1–C6 2.7516(18) Å) is significantly longer than that of the bis(pentafulvene)titanium complex Ti1 (2.341(2) Å and 2.363(2) Å),37 which is a common feature of functionalized mono(pentafulvene)titanium complexes.30 The molecular structures of complexes Ti1a (Fig. 3) and Ti2b (Fig. 4) are similar and the heterocycles provide no additional coordination. The bond parameters and angles of all mono(pentafulvene)-thiosemicarbazonido titanium complexes are summarized in Table 1.
Table 1 Selected bond parameters (bond lengths (Å) and angles (deg)) of complexes Ti1a, Ti1c and Ti2b
Complex |
Ti1–N1 |
Ti1–S1 |
N1–C31/C41 |
S1–C31/C41 |
Ti1–C6 |
N1–Ti1–S1 |
Ti1a
|
2.1839(5) |
2.58107(19) |
1.3354(7) |
1.7175(5) |
2.744 |
63.823(13) |
Ti1c
|
2.2222(15) |
2.5823(5) |
1.349(2) |
1.7118(18) |
2.7516(18) |
63.28(4) |
Ti2b
|
2.1750(10) |
2.5798(4) |
1.3338(15) |
1.7165(12) |
2.756 |
63.99(3) |
As previously reported for mono(pentafulvene)hydrazonido titanium complexes,30 the lack of follow-up chemistry of Ti1a–c and Ti2a,b with multiple bond substrates and Brønsted acids can be explained by the formal 18-electron nature of the complexes.38 Due to this restriction in reactivity, we were unable to further functionalize the complexes or to obtain the desired ionic complexes by protonolysis of the remaining pentafulvene moiety, which is necessary to avoid reactions with water and to improve water-solubility. Therefore, we attempted an intramolecular functionalization reaction by the introduction of an OH group in TSCN d. Previously, this approach led to a double deprotonation reaction to obtain a κ3N,N,O complex.30
In this work, the reactions of bis(π-η5:σ-η1-pentafulvene)titanium complexes Ti1 and Ti2 with the thiosemicarbazone d led to the formation of a mixture of various products (ESI,† Fig. S10). One of the products was determined by single-crystal X-ray diffraction (Fig. 6), revealing a κ2N,O titanium complex Ti1d similarly to that in a previous work.30 Complex Ti1d is obtained via deprotonation of the acidic OH-proton by one pentafulvene unit and insertion of the C
N double bond into the Ti–Cexo bond of the second pentafulvene moiety alongside other byproducts (Scheme 2).
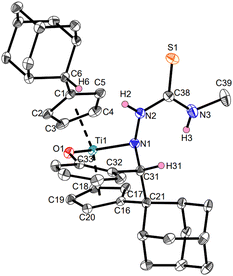 |
| Fig. 6 Molecular structure of complex Ti1d. Displacement ellipsoids are drawn at the 50% probability level. Redundant H atoms and solvent molecules have been omitted for clarity. Selected bond lengths (Å) and angles (deg): Ti1–N1 2.0437(12), Ti1–O1 1.9152(10), N1–N2 1.4100(17), N1–C31 1.4968(18), N2–C38 1.3458(19), O1–C33 1.3455(17), C33–C32 1.415(2), C32–C31 1.5313(19), C31–C21 1.573(2), O1–H6 2.68, C38–S1 1.6934(16), O1–Ti1–N1 88.09(5), N2–C38–S1 119.87(12), Ti1–N1–N2 120.00(9), C38–N1–N2 122.63(13), Ct1–Ti1–Ct2 132.4 (Ct1 = centroid of C1–C5; Ct2 = centroid of C16–C20). | |
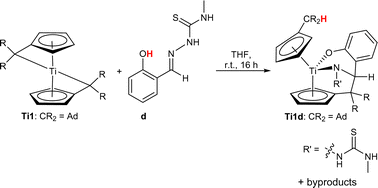 |
| Scheme 2 Reaction of bis(π-η5:σ-η1-pentafulvene)titanium complex Ti1 with thiosemicarbazone d to κ2N,O-thiosemicarbazonate titanium complex Ti1d and additional byproducts. | |
Although the byproducts could not be determined unequivocally, they are most likely precursors of Ti1d – either by single protonolysis or by single insertion, a complex similar to Ti1a, Ti1c and Ti2b, or the desired κ3N,N,O follow-up product. While the mixture cannot be converted into a singular product by heating and changes in the reaction conditions only slightly impact the outcome, the formation of this complex demonstrates an unusual TSCN reactivity pattern.
The molecular structure of Ti1d reveals a slightly shortened Ti–N bond (2.0437(12) Å) and a shortened Ti–O bond (1.9152(10) Å) when compared with the sum of covalent radii (Σrcov(Ti–N) = 2.07 Å, Σrcov(Ti–O) = 1.99 Å).35 These structural features are similar to a suchlike, reported κ2N,O titanium complex (Ti–N: 2.0073(6) Å, Ti–O: 1.9332(6) Å).30
Reaction of TSCN with titanocene(III) triflate
As we were unable to produce ionic complexes using the first route, we attempted to obtain ionic complexes using titanocene(III) triflate (Ti3) as the starting material. Because treatment of Ti3 with bidentate ligands yields ionic triflate complexes by displacement of the coordinating triflato ligand,32 the multidentate TSCN appear to be excellent ligands for this reaction. While no reaction occurred with TSCN a and b, the reaction of Ti3 with c resulted in the formation of the formal κ2N,N titanium(III) complex Ti3c (Scheme 3). Because of the poor crystallization properties of Ti3c, we could not obtain suitable crystals for single-crystal X-ray diffraction, therefore, we calculated the molecular structure of Ti3c (Fig. 7).
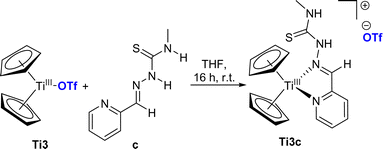 |
| Scheme 3 Reaction of titanocene triflate Ti3 with TSCN c to κ2N,N titanium(III) triflate complex Ti3c. | |
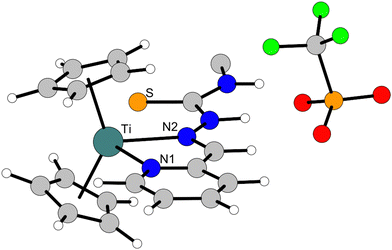 |
| Fig. 7 Ground state optimized geometry of complex Ti3c, calculated in gas phase at the B3LYP/Def2-TZVP level of theory. Selected bond lengths (Å): Ti–N1 2.26, Ti–N2 2.39, Ti–S 3.71. | |
Although a κ3N,N,S coordination mode seems reasonable, the respective complex is not a minimum on the potential energy surface; instead, the κ2N,N coordination mode was revealed, as indicated by the long Ti–S distance (3.71 Å) which does not correlate to a Ti–S bond (Fig. 4). This is most likely due to the higher stability of the formal 17 electron κ2N,N complex compared with the formal 19 electron κ3N,N,S complex. A similar κ2N,N complex (titanocene(III) 2-pyridinecarboxaldehyde phenylhydrazone) has also been reported previously,32 while there are also several other examples of Ti(III) κ2N,N complexes.39 The formation of a Ti(III) complex is supported by EPR spectrosopy, showing a typical signal that correlates to a Ti(III) species (ESI,† Fig. S24, g = 1.980).32,40
In a quite different manner, the reaction of Ti3 with TSCN d produces a dinuclear, dicationic κ3O,N,S titanium complex Ti3dvia protonolysis of one cyclopentadienyl ligand by the acidic OH group of the o-cresyl rest, whereas the former coordinating triflato ligands are displaced by the TSCN and now act as counter ions (Scheme 4). The protonolysis of a cyclopentadienyl ligand of Ti3 is a novel reactivity that has not been reported so far and most likely ocurred due to the acidity of the OH group of TSCN d along with the chelating effect of the tridentate ligand and the oxophilicity of titanium complexes.32
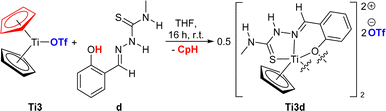 |
| Scheme 4 Reaction of titanocene triflate Ti3 with TSCN d to dinuclear Ti(III) thiosemicarbazonido triflate complex Ti3d. | |
The structure of Ti3d was revealed by single-crystal X-ray diffraction (Fig. 8), while the elemental analysis support the elemental composition of the obtained solid and verify the purity of Ti3d. The paramagnetic nature of Ti3d is supported by EPR spectrosopy, displaying a similar signal compared with Ti3c, corralating to a Ti(III) species (ESI,† Fig. S25, g = 1.977).32,40
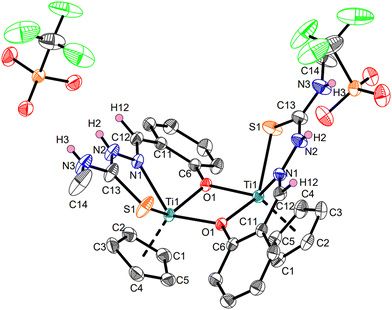 |
| Fig. 8 Crystal structure of complex Ti3d. Displacement ellipsoids are drawn at the 50% probability level. Redundant H (apart from H2, H3 and H12) atoms and have been omitted for clarity. Selected bond lengths (Å) and angles (deg): Ti1–O1 2.0482(15), Ti1–O#1 2.0639(14), Ti1–N1 2.1810(18), Ti1–S1 2.4774(7), O1–C6 1.360(2), N1–N2 1.374(3), N3–C13 1.319(3), N2–C13 1.342(3), N1–C12 1.295(3), S1–C13 1.705(2), O1–Ti1–O#1 77.83(6), Ti1–O1–Ti#1 102.09(6), N1–Ti1–O1 81.00(6), S1–Ti1–O#1 84.33(4), N1–Ti1–S1 76.95(5), N2–C13–S1 120.41(17), Ti1–N1–N2 119.66(14), C13–N2–N1 119.91(19). | |
Similar to the κ2N,S-thiosemicarbazonate titanium complexes Ti1a, Ti1c and Ti2b, the Ti–N and Ti–S bonds are best described as elongated single bonds. The Ti–O bonds are also elongated single bonds (Σrcov(Ti–O) = 1.99 Å)35 as they are shared across two titanium cores. The triflate anions exhibit hydrogen bonds by interaction of the oxygen atoms with the NH groups of the TSCN (H2⋯O4 2.01(3) Å, H3⋯O3 2.20(4) Å). This effect was also observed with other ionic titanium triflate complexes.32
While this series of complexes is not suitable for further biological studies, this work provides great insight into the coordination chemistry of thiosemicarbazones within titanium complexes.
Conclusions
In this work, we employed two synthetic strategies to obtain Ti(IV) and Ti(III) complexes with bis(pentafulvene)titanium complexes and titanocene(III) triflate as starting materials. While most thiosemicarbazones (TSCN) were deprotonated in N-position by one pentafulvene unit and form κ2N,S complexes, the OH group of the o-cresyl TSCN was deprotonated, revealing a κ2N,O titanium complex. The Ti(IV) complexes were characterized by NMR spectroscopy and the 15N NMR values were obtained for each nitrogen core via two-dimensional 1H,15N NMR experiments. Reaction of the pyridinyl TSCN with titanocene(III) triflate yielded a κ2N,N titanium complex via ligand exchange, displacing the former coordinating triflato ligand and forming an ionic complex. Reaction with the o-cresyl TSCN displayed a novel reactivity of titanocene(III) triflate, as the cyclopentadienyl ligand underwent protonolysis, subsequently forming a dinuclear, dicationic κ3O,N,S titanium complex. Herein, the multifaceted coordination chemistry of TSCN was demonstrated and the first structural examples of thiosemicarbazone-based titanium complexes obtained by single-crystal X-ray diffraction are described. Future work will focus on more applicable TSCN-based titanium complexes because none of these complexes are suitable for biological studies.
Experimental section
All reactions were carried out under a dry nitrogen or argon atmosphere using standard Schlenk and glove box techniques. Solvents were dried according to standard procedures over Na/K alloy with benzophenone as indicator and subsequently distilled and stored under a nitrogen atmosphere. The pentafulvene complexes,37 titanocene(III) triflate32 and TSCN33 were prepared according to general methods and published procedures. NMR spectra were recorded on a Bruker AVANCE III 500 spectrometer (1H 500 MHz). IR spectra were recorded on a Bruker Tensor 27 spectrometer using an attenuated total reflection (ATR) method. Elemental analyses were carried out on a Euro EA 3000 Elemental Analyzer. Melting points were determined using a “Mel-Temp” from Laboratory Devices, Cambridge, or a Mettler Toledo MP30. Further exact details of crystallographic data and NMR, IR, EPR spectra are given in the ESI.†
Synthesis of Ti1a
Bis(adamantylidenefulvene)titanium complex Ti1 (300 mg, 0.675 mmol) and benzaldehyde N-methylthiosemicarbazone a (130 mg, 0.675 mmol) were dissolved in 10 ml of dry THF. The reaction mixture was stirred for 16 h at room temperature to give a dark red solution. The solvent was removed under reduced pressure and the residue was washed with 10 mL of n-hexane. All volatile components were removed under reduced pressure and the residue was dried under vacuum to yield the product as a dark red solid. Red crystals suitable for single crystal X-ray diffraction analysis precipitated from a slowly evaporating solution of Ti1a in C6D6 after several days. Yield: 0.330 g, 0.517 mmol, 75%. 1H NMR (500 MHz, C6D6, 305 K): δ = 1.44–2.45 (m, 27 H, Ad–H), 2.64 (d, 3 H, J = 5.0 Hz, N–CH3), 2.74–2.82 (m, 2 H, Ad–H), 4.15–4.18 (m, 1 H, Cp–H), 4.94–4.97 (m, 1 H, Cp–H), 4.98–5.01 (m, 1 H, Cp–H), 5.13–5.17 (m, 1 H, Cp–H), 5.39–5.43 (m, 1 H, Cp–H), 5.45–5.49 (m, 1 H, Cp–H), 5.79–5.83 (m, 1 H, Cp–H), 6.13–6.17 (m, 1 H, Cp–H), 6.67 (q, 1 H, J = 5.0 Hz, N–H), 7.11–7.14 (m, 1 H, Ph–H), 7.19–7.24 (m, 2 H, Ph–H), 7.32 (s, 1 H, aldimine–H), 7.58–7.62 (m, 1 H, Ph–H) ppm. 13C{1H} NMR (125 MHz, C6D6, 305 K): δ = 28.4 (Ad–CH), 28.6 (CH3), 28.7 (Ad–CH), 28.8 (Ad–CH), 29.7 (Ad–CH), 32.8 (Ad–CH2), 32.9 (Ad–CH2), 33.0 (Ad–CH), 33.3 (Ad–CH), 36.3 (Ad–CH), 36.4 (Ad–CH), 37.8 (Ad–CH2), 38.1 (Ad–CH2), 38.4 (Ad–CH2), 38.5 (Ad–CH2), 39.4 (Ad–CH2), 39.5 (Ad–CH2), 44.8 (Ad–CH), 44.9 (Ad–CH2), 45.2 (Ad–CH2), 97.8 (Cp–CH), 99.6 (Cp–CH), 101.5 (Cp–CH), 102.2 (Cp–CH), 103.7 (Cp–CH), 105.1 (Cp–CH), 105.5 (Cp–CH), 118.2 (Cexo–Cq), 123.3 (Cp–CH), 127.3 (2 × Ph–CH), 129.0 (2 × Ph–CH), 129.1 (Ph–CH), 130.0 (Cipso–Cq), 132.9 (Cipso–Cq), 136.5 (Ph–Cq), 143.2 (aldimine–CH), 181.6 (N–Cq–N) ppm. 15N NMR (51 MHz, C6D6, 305 K): δ = 87.6 (H–N–CH3), 211.7 (C![[double bond, length as m-dash]](https://www.rsc.org/images/entities/char_e001.gif)
–N), 346.0 (C
N–
) ppm. IR (ATR): ṽ = 3387, 3059, 3023, 2899, 2844, 2651, 2361, 2101, 1594, 1568, 1531, 1465, 1446, 1429, 1377, 1332, 1297, 1260, 1212, 1168, 1094, 1081, 1061, 1028, 946, 899, 853, 792, 754, 729, 691, 654, 618, 565, 509, 464 cm−1. Mp. 109–114 °C (dec.). EA: calcd for C39H47N3STi: C 73.45, H 7.43, N 6.59. Found: C 73.59, H 7.58, N 6.14.
Synthesis of Ti1b
Bis(adamantylidenefulvene)titanium complex Ti1 (300 mg, 0.675 mmol) and 2-thiophenecarboxaldehyde N-methylthiosemicarbazone b (135 mg, 0.675 mmol) were dissolved in 10 ml of dry THF. The reaction mixture was stirred for 16 h at room temperature to give a dark red solution. The solvent was removed under reduced pressure and the residue was washed with 10 mL of n-hexane. All volatile components were removed under reduced pressure and the residue was dried under vacuum to yield the product as a dark red solid. Yield: 0.376 g, 0.584 mmol, 87%. 1H NMR (500 MHz, C6D6, 305 K): δ = 1.45–2.41 (m, 27 H, Ad–H), 2.51 (d, 3 H, J = 5.0 Hz, N–CH3), 2.70–2.79 (m, 2 H, Ad–H), 4.11–4.15 (m, 1 H, Cp–H), 4.88–4.92 (m, 1 H, Cp–H), 4.96–5.01 (m, 1 H, Cp–H), 5.09–5.14 (m, 1 H, Cp–H), 5.37–5.40 (m, 1 H, Cp–H), 5.44–5.48 (m, 1 H, Cp–H), 5.77–5.82 (m, 1 H, Cp–H), 6.09–6.13 (m, 1 H, Cp–H), 6.62 (q, 1 H, J = 5.0 Hz, N–H), 6.75–6.78 (m, 1 H, Ar–H), 6.82–6.85 (m, 1 H, Ar–H), 6.95–6.98 (m, 1 H, Ar–H), 7.49 (s, 1 H, aldimine–H) ppm. 13C{1H} NMR (125 MHz, C6D6, 305 K): δ = 28.4 (Ad–CH), 28.6 (Ad–CH), 28.6 (CH3), 28.6 (Ad–CH), 29.7 (Ad–CH), 32.8 (Ad–CH2), 32.9 (Ad–CH2), 33.0 (Ad–CH), 33.2 (Ad–CH), 36.2 (Ad–CH), 36.4 (Ad–CH), 37.8 (Ad–CH2), 38.1 (Ad–CH2), 38.4 (Ad–CH2), 38.5 (Ad–CH2), 39.4 (Ad–CH2), 39.5 (Ad–CH2), 44.8 (Ad–CH), 44.9 (Ad–CH2), 45.2 (Ad–CH2), 97.7 (Cp–CH), 99.5 (Cp–CH), 101.5 (Cp–CH), 102.0 (Cp–CH), 103.9 (Cp–CH), 105.2 (Cp–CH), 105.5 (Cp–CH), 118.2 (Cexo–Cq), 123.2 (Cp–CH), 126.4 (Ar–CH), 128.6 (Ar–CH), 129.3 (Ar–CH), 129.9 (Cipso–Cq), 133.1 (Cipso–Cq), 137.3 (aldimine–CH), 142.0 (Ar–Cq), 181.2 (N–Cq–N) ppm. 15N NMR (51 MHz, C6D6, 305 K): δ = 88.4 (H–N–CH3), 211.0 (C![[double bond, length as m-dash]](https://www.rsc.org/images/entities/char_e001.gif)
–N), 342.0 (C
N–
) ppm. IR (ATR): ṽ = 3386, 3099, 3022, 2899, 2845, 2667, 2360, 2341, 2108, 1579, 1536, 1465, 1447, 1429, 1371, 1351, 1331, 1289, 1259, 1212, 1166, 1096, 1071, 1041, 974, 947, 924, 857, 792, 754, 729, 695, 666, 654, 567, 504, 464 cm−1. Mp. 114–121 °C (dec.). EA: calcd for C37H45N3S2Ti: C 69.03, H 7.05, N 6.53. Found: C 68.73, H 7.26, N 6.16.
Synthesis of Ti1c
Bis(adamantylidenefulvene)titanium complex Ti1 (300 mg, 0.675 mmol) and 2-pyridinecarboxaldehyde N-methylthiosemicarbazone c (131 mg, 0.675 mmol) were dissolved in 10 ml of dry THF. The reaction mixture was stirred for 16 h at room temperature to give a dark red solution. The solvent was removed under reduced pressure and the residue was washed with 10 mL of n-hexane. All volatile components were removed under reduced pressure and the residue was dried under vacuum to yield the product as a dark red solid. Orange crystals suitable for single crystal X-ray diffraction analysis precipitated from a slowly evaporating solution of Ti1c in C6D6 after several days. Yield: 0.379 g, 0.593 mmol, 88%. 1H NMR (500 MHz, C6D6, 305 K): δ = 1.42–2.49 (m, 27 H, Ad–H), 2.64 (d, 3 H, J = 5.0 Hz, N–CH3), 2.70–2.83 (m, 2 H, Ad–H), 4.05–4.08 (m, 1 H, Cp–H), 4.81–4.84 (m, 1 H, Cp–H), 5.05–5.08 (m, 1 H, Cp–H), 5.10–5.13 (m, 1 H, Cp–H), 5.34–5.37 (m, 1 H, Cp–H), 5.53–5.57 (m, 1 H, Cp–H), 5.85–5.88 (m, 1 H, Cp–H), 6.14–6.17 (m, 1 H, Cp–H), 6.64–6.68 (m, 1 H, Ar–H), 6.70 (q, 1 H, J = 5.0 Hz, N–H), 7.12–7.15 (m, 1 H, Ar–H), 7.62 (s, 1 H, aldimine–H), 7.72–7.75 (m, 1 H, Ar–H), 8.48–8.52 (m, 1 H, Ar–H) ppm. 13C{1H} NMR (125 MHz, C6D6, 305 K): δ = 28.4 (Ad–CH), 28.7 (CH3), 28.8 (Ad–CH), 29.7 (Ad–CH), 32.0 (Ad–CH), 32.9 (2 × Ad–CH2), 32.9 (Ad–CH), 33.3 (Ad–CH), 36.2 (Ad–CH), 36.3 (Ad–CH), 37.8 (Ad–CH2), 38.1 (Ad–CH2), 38.5 (2 × Ad–CH2), 39.5 (Ad–CH2), 39.5 (Ad–CH2), 44.8 (Ad–CH), 44.9 (Ad–CH2), 45.1 (Ad–CH2), 98.3 (Cp–CH), 100.2 (Cp–CH), 101.8 (Cp–CH), 102.6 (Cp–CH), 103.4 (Cp–CH), 104.9 (Cp–CH), 105.3 (Cp–CH), 118.2 (Cexo–Cq), 119.4 (Ar–CH), 122.8 (Ar–CH), 123.1 (Cp–CH), 129.3 (Ar–Cq), 130.4 (Cipso–Cq), 132.7 (Cipso–Cq), 135.7 (Ar–CH), 144.3 (aldimine–CH), 150.0 (Ar–CH), 182.7 (N–Cq–N) ppm. 15N NMR (51 MHz, C6D6, 305 K): δ = 88.4 (H–N–CH3), 213.3 (C![[double bond, length as m-dash]](https://www.rsc.org/images/entities/char_e001.gif)
–N), 317.1 (pyridine–N), 354.6 (C
N–
) ppm. IR (ATR): ṽ = 3390, 2899, 2844, 2358, 2164, 2111, 1579, 1561, 1526, 1465, 1447, 1430, 1370, 1331, 1276, 1260, 1212, 1146, 1096, 1071, 1032, 992, 945, 931, 853, 795, 775, 730, 694, 670, 657, 618, 582, 565, 519, 464 cm−1. Mp. 91–96 °C (dec.). EA: calcd for C38H46N4STi: C 71.46, H 7.26, N 8.77. Found: C 71.23, H 7.38, N 8.70.
Attempted synthesis of Ti1d
Bis(adamantylidenefulvene)titanium complex Ti1 (200 mg, 0.450 mmol) and salicylaldehyde N-methylthiosemicarbazone d (94 mg, 0.450 mmol) were dissolved in 10 ml of dry THF. The reaction mixture was stirred for 16 h at room temperature to give a dark red brown solution. The solvent was removed under reduced pressure and the residue was washed with 10 mL of n-hexane. All volatile components were removed under reduced pressure and the residue was dried under vacuum to yield a red brown solid. Orange crystals suitable for single crystal X-ray diffraction analysis precipitated from a saturated solution of the product mixture in toluene at −20 °C after several days. Further characterization is ommited as this reaction resulted in a mixture of products which was not further characterized.
Synthesis of Ti2a
Bis(di-para-tolylfulvene)titanium complex Ti2 (300 mg, 0.531 mmol) and benzaldehyde N-methylthiosemicarbazone a (103 mg, 0.531 mmol) were dissolved in 10 ml of dry THF. The reaction mixture was stirred for 16 h at room temperature to give a dark red solution. The solvent was removed under reduced pressure and the residue was washed with 10 mL of n-hexane. All volatile components were removed under reduced pressure and the residue was dried under vacuum to yield the product as a dark red solid. Yield: 0.283 g, 0.373 mmol, 70%. 1H NMR (500 MHz, C6D6, 305 K): δ = 1.99 (s, 3 H, p-Tol–CH3), 2.08 (s, 3 H, p-Tol–CH3), 2.12 (s, 6 H, 2 × p-Tol–CH3), 2.49 (d, 3 H, J = 4.9 Hz, N–CH3), 4.80–4.83 (m, 1 H, Cp–H), 5.02–5.05 (m, 1 H, Cp–H), 5.10–5.13 (m, 1 H, Cp–H), 5.16–5.18 (m, 1 H, Cp–H), 5.19 (s, 1 H, Cexo–H), 5.64–5.69 (m, 2 H, 2 × Cp–H), 5.73–5.76 (m, 1 H, Cp–H), 5.90–5.93 (m, 1 H, Cp–H), 6.58 (q, 1 H, J = 4.9 Hz, N–H), 6.79–6.82 (m, 3 H, Ar–H), 6.99 (s, 1 H, aldimine–H), 6.99–7.15 (m, 10 H, Ar–H), 7.35–7.39 (m, 4 H, Ar–H), 7.45–7.48 (m, 2 H, Ar–H), 7.50–7.55 (m, 2 H, Ar–H) ppm. 13C{1H} NMR (125 MHz, C6D6, 305 K): δ = 20.9 (2 × p-Tol–CH3), 21.0 (2 × p-Tol–CH3), 28.5 (N–CH3), 53.1 (CexoH), 99.0 (Cp–CH), 100.3 (Cp–CH), 104.7 (Cp–CH), 107.2 (Cp–CH), 108.5 (Cp–CH), 108.7 (Cp–CH), 110.0 (Cp–CH), 120.9 (Cexo–Cq), 123.6 (Cp–CH), 127.4 (2 × Ar–CH), 127.5 (Ar–Cq), 128.6 (Ar–Cq), 128.7 (2 × Ar–CH), 128.8 (2 × Ar–CH), 129.0 (2 × Ar–CH), 129.0 (Ar–CH), 129.1 (2 × Ar–CH), 129.2 (2 × Ar–CH), 129.3 (2 × Ar–CH), 129.5 (2 × Ar–CH), 129.6 (2 × Ar–CH), 129.9 (Cipso–Cq), 130.8 (2 × Ar–CH), 135.4 (2 × Ar–Cq), 135.9 (2 × Ar–Cq), 136.2 (Ar–Cq), 140.7 (2 × Ar–Cq), 142.9 (aldimine–CH), 143.2 (Cipso–Cq), 143.2 (2 × Ar–Cq), 178.1 (N–Cq–N) ppm. 15N NMR (51 MHz, C6D6, 305 K): δ = 87.6 (H–N–CH3), 207.8 (C![[double bond, length as m-dash]](https://www.rsc.org/images/entities/char_e001.gif)
–N), 345.2 (C
N–
) ppm. IR (ATR): ṽ = 3399, 3020, 2919, 2862, 2360, 1594, 1568, 1531, 1508, 1467, 1447, 1431, 1379, 1332, 1301, 1261, 1220, 1188, 1160, 1085, 1066, 1042, 1020, 946, 886, 795, 765, 759, 730, 694, 656, 619, 593, 575, 563, 544, 510, 458, 465 cm−1. Mp. 184–187 °C (dec.). EA: calcd for C49H47N3STi: C 77.66, H 6.25, N 5.54. Found: C 77.66, H 6.42, N 5.28.
Synthesis of Ti2b
Bis(di-para-tolylfulvene)titanium complex Ti2 (300 mg, 0.531 mmol) and 2-thiophenecarboxaldehyde N-methylthiosemicarbazone b (106 mg, 0.531 mmol) were dissolved in 10 ml of dry THF. The reaction mixture was stirred for 16 h at room temperature to give a dark red solution. The solvent was removed under reduced pressure and the residue was washed with 10 mL of n-hexane. All volatile components were removed under reduced pressure and the residue was dried under vacuum to yield the product as a dark red solid. Red crystals suitable for single crystal X-ray diffraction analysis precipitated from a slowly evaporating solution of Ti2b in C6D6 after several days. Yield: 0.323 g, 0.423 mmol, 80%. 1H NMR (500 MHz, C6D6, 305 K): δ = 2.01 (s, 3 H, p-Tol–CH3), 2.06 (s, 3 H, p-Tol–CH3), 2.12 (s, 3 H, p-Tol–CH3), 2.14 (s, 3 H, p-Tol–CH3), 2.36 (d, 3 H, J = 4.9 Hz, N–CH3), 4.76–4.79 (m, 1 H, Cp–H), 5.00–5.03 (m, 1 H, Cp–H), 5.07–5.10 (m, 1 H, Cp–H), 5.14 (s, 1 H, Cexo–H), 5.18–5.21 (m, 1 H, Cp–H), 5.61–5.64 (m, 1 H, Cp–H), 5.67–5.70 (m, 1 H, Cp–H), 5.71–5.74 (m, 1 H, Cp–H), 5.89–5.92 (m, 1 H, Cp–H), 6.56 (q, 1 H, J = 4.9 Hz, N–H), 6.70–6.72 (m, 2 H, Ar–H), 6.78–6.87 (m, 5 H, Ar–H), 6.99–7.03 (m, 4 H, Ar–H), 7.04–7.07 (m, 2 H, Ar–H), 7.13 (s, 1 H, aldimine–H), 7.34–7.39 (m, 2 H, Ar–H), 7.43–7.45 (m, 2 H, Ar–H), 7.47–7.52 (m, 2 H, Ar–H) ppm. 13C{1H} NMR (125 MHz, C6D6, 305 K): δ = 20.9 (2 × p-Tol–CH3), 21.1 (2 × p-Tol–CH3), 28.7 (N–CH3), 53.1 (CexoH), 99.1 (Cp–CH), 100.4 (Cp–CH), 104.4 (Cp–CH), 107.3 (Cp–CH), 108.5 (Cp–CH), 108.6 (Cp–CH), 109.9 (Cp–CH), 121.0 (Cexo–Cq), 123.5 (Cp–CH), 126.3 (Ar–CH), 127.4 (2 × Ar–CH), 127.5 (Ar–Cq), 128.6 (Ar–Cq), 128.7 (Ar–CH), 128.8 (2 × Ar–CH), 128.9 (2 × Ar–CH), 129.1 (2 × Ar–CH), 129.2 (2 × Ar–CH), 129.3 (Ar–CH), 129.6 (2 × Ar–CH), 129.6 (2 × Ar–CH), 129.8 (Cipso–Cq), 130.7 (2 × Ar–CH), 135.4 (2 × Ar–Cq), 135.9 (2 × Ar–Cq), 137.1 (Ar–Cq), 141.7 (2 × Ar–Cq), 143.0 (aldimine–CH), 143.3 (Cipso–Cq), 177.7 (N–Cq–N) ppm. 15N NMR (51 MHz, C6D6, 305 K): δ = 87.6 (H–N–CH3), 207.0 (C![[double bond, length as m-dash]](https://www.rsc.org/images/entities/char_e001.gif)
–N), 341.3 (C
N–
) ppm. IR (ATR): ṽ = 3395, 3020, 2916, 2358, 1579, 1536, 1507, 1467, 1448, 1430, 1372, 1332, 1289, 1232, 1214, 1180, 1159, 1112, 1082, 1042, 1020, 930, 885, 858, 828, 806, 793, 767, 755, 730, 710, 697, 654, 593, 576, 563, 544, 506, 486, 464 cm−1. Mp. 182–186 °C (dec.). EA: calcd for C47H45N3S2Ti: C 73.90, H 5.94, N 5.50. Found: C 74.09, H 5.84, N 5.57.
Synthesis of Ti3c
Titanocene(III)triflate Ti3 (100 mg, 0.306 mmol) and 2-pyridinecarboxaldehyde N-methylthiosemicarbazone c (59.4 mg, 0.306 mmol) were dissolved in 10 ml of dry THF. The reaction mixture was stirred for 16 h at room temperature to give a dark red solution. The solvent was removed under reduced pressure and the residue was washed with n-hexane (3 × 10 mL). All volatile components were removed under reduced pressure and the residue was dried under vacuum to yield the product as a red solid. Yield: 121 mg, 0.232 mmol, 76%. IR (ATR): ṽ = 3283, 3120, 2944, 1587, 1526, 1469, 1435, 1325, 1236, 1212, 1164, 1109, 1083, 1019, 998, 929, 814, 778, 766, 726, 676, 629, 592, 516, 467, 412 cm−1. Mp. 166 °C (dec.). EPR: g = 1.980. EA: calcd for C19H20F3N4S2O3Ti: C 43.77, H 3.87, N 10.75. Found: C 43.73, H 3.91, N 10.44.
Synthesis of Ti3d
Titanocene(III)triflate Ti3 (100 mg, 0.306 mmol) and salicylaldehyde N-methylthiosemicarbazone d (64 mg, 0.306 mmol) were dissolved in 10 ml of dry THF. The reaction mixture was stirred for 16 h at room temperature to give a yellow solution. The solvent was removed under reduced pressure and the residue was washed with n-hexane (3 × 10 mL). All volatile components were removed under reduced pressure and the residue was dried under vacuum to yield the product as a brown solid. Yield: 121 mg, 0.232 mmol, 76%. IR (ATR): ṽ = 3241, 3051, 2942, 1602, 1560, 1480, 1468, 1440, 1401, 1360, 1339, 1272, 1243, 1223, 1157, 1026, 964, 917, 816, 794, 764, 731, 696, 626, 591, 576, 544, 516, 456, 405 cm−1. Mp. 195 °C (dec.). EPR: g = 1.977. EA: calcd for C15H15F3N3S2O4Ti: C 38.31, H 3.21, N 8.93. Found: C 38.85, H 3.64, N 9.10.
Author contributions
Conceptualization, methodology, synthesis, characterization, writing – original draft preparation, writing – review and editing: K. S., synthesis, characterization: M. C., X-ray crystallography: M. S., supervision, funding acquisition, writing – review and editing: R. B.
Data availability
CCDC 2377765 (Ti1a), 2377767 (Ti1c), 2377764 (Ti1d), 2377768 (Ti2b), 2377766 (Ti3d) contain supplementary crystallographic data for this paper. The data supporting this article have been included as part of the ESI.†
Conflicts of interest
The authors declare no competing financial interest.
Acknowledgements
Financial support by the DFG Research Training Group 2226 is kindly acknowledged.
References
-
(a) T. S. Lobana, R. Sharma, G. Bawa and S. Khanna, Coord. Chem. Rev., 2009, 253, 977–1055 CrossRef CAS;
(b) S. Padhyé and G. B. Kauffman, Coord. Chem. Rev., 1985, 63, 127–160 CrossRef.
- S. Gupta, N. Singh, T. Khan and S. Joshi, Results Chem., 2022, 4, 100459 CrossRef CAS.
- J. R. Dilworth and R. Hueting, Inorg. Chim. Acta, 2012, 389, 3–15 CrossRef CAS.
- A. G. Quiroga and C. N. Ranninger, Coord. Chem. Rev., 2004, 248, 119–133 CrossRef CAS.
- D. S. Kalinowski, P. Quach and R. Des Richardson, Future Med. Chem., 2009, 1, 1143–1151 CrossRef CAS PubMed.
- T. S. Lobana, RSC Adv., 2015, 5, 37231–37274 RSC.
- T. S. Lobana, R. Rekha, R. J. Butcher, A. Castineiras, E. Bermejo and P. V. Bharatam, Inorg. Chem., 2006, 45, 1535–1542 CrossRef CAS PubMed.
-
(a) T. S. Lobana, S. Khanna, R. J. Butcher, A. D. Hunter and M. Zeller, Inorg. Chem., 2007, 46, 5826–5828 CrossRef CAS;
(b) A. Khan, J. P. Jasinski, V. A. Smolenski, E. P. Hotchkiss, P. T. Kelley, Z. A. Shalit, M. Kaur, K. Paul and R. Sharma, Bioorg. Chem., 2018, 80, 303–318 CrossRef CAS PubMed;
(c) L. M. González-Barcia, S. Fernández-Fariña, L. Rodríguez-Silva, M. R. Bermejo, A. M. González-Noya and R. Pedrido, J. Inorg. Biochem., 2020, 203, 110931 CrossRef;
(d) D. E. S. Silva, A. B. Becceneri, J. V. B. Santiago, J. A. Gomes Neto, J. Ellena, M. R. Cominetti, J. C. M. Pereira, M. J. Hannon and A. V. G. Netto, Dalton Trans., 2020, 49, 16474–16487 RSC.
-
(a) T. S. Lobana, P. Kumari, M. Zeller and R. J. Butcher, Inorg. Chem. Commun., 2008, 11, 972–974 CrossRef CAS;
(b) S. Lhuachan, S. Siripaisarnpipat and N. Chaichit, Eur. J. Inorg. Chem., 2003, 263–267 CrossRef CAS;
(c) E. M. Jouad, A. Riou, M. Allain, M. A. Khan and G. M. Bouet, Polyhedron, 2001, 20, 67–74 CrossRef CAS.
-
(a) E. Bermejo, R. Carballo, A. Castiñeiras, R. Domínguez, C. Maichle-Mössmer, J. Strähle and D. X. West, Polyhedron, 1999, 18, 3695–3702 CrossRef CAS;
(b) E. Labisbal, K. D. Haslow, A. Sousa-Pedrares, J. Valdés-Martínez, S. Hernández-Ortega and D. X. West, Polyhedron, 2003, 22, 2831–2837 CrossRef CAS.
- G. Pereiras-Gabián, E. M. Vázquez-López, H. Braband and U. Abram, Inorg. Chem., 2005, 44, 834–836 CrossRef.
-
(a) W. Su, Z. Tang, P. Li, G. Wang, Q. Xiao, Y. Li, S. Huang, Y. Gu, Z. Lai and Y. Zhang, Dalton Trans., 2016, 45, 19329–19340 RSC;
(b) T. S. Lobana, S. Khanna, G. Hundal, R. J. Butcher and A. Castineiras, Polyhedron, 2009, 28, 3899–3906 CrossRef CAS;
(c) T. S. Lobana, G. Bawa and R. J. Butcher, Inorg. Chem., 2008, 47, 1488–1495 CrossRef CAS PubMed.
- S. Argibay-Otero, A. M. Graña, R. Carballo and E. M. Vázquez-López, Inorg. Chem., 2020, 59, 14101–14117 CrossRef CAS PubMed.
-
(a) T. S. Lobana, G. Bawa, R. J. Butcher, B.-J. Liaw and C. W. Liu, Polyhedron, 2006, 25, 2897–2903 CrossRef CAS;
(b) P. Kalaivani, R. Prabhakaran, E. Vaishnavi, T. Rueffer, H. Lang, P. Poornima, R. Renganathan, V. Vijaya Padma and K. Natarajan, Inorg. Chem. Front., 2014, 1, 311 RSC;
(c) X. Jing, C. He, Y. Yang and C. Duan, J. Am. Chem. Soc., 2015, 137, 3967–3974 CrossRef CAS PubMed;
(d) D. Grödler, A. Haseloer, C. Tobeck, Y. Bulut, J. M. Neudörfl, S. Mathur, U. Ruschewitz, A. Klein, M. S. Wickleder and M. Zegke, Eur. J. Inorg. Chem., 2021, 1137–1139 CrossRef.
-
(a) S. Roy, Saswati, S. Lima, S. Dhaka, M. R. Maurya, R. Acharyya, C. Eagle and R. Dinda, Inorg. Chim. Acta, 2018, 474, 134–143 CrossRef CAS;
(b) T. Straistari, A. Morozan, S. Shova, M. Réglier, M. Orio and V. Artero, Eur. J. Inorg. Chem., 2020, 4549–4555 CrossRef CAS;
(c) J. Baruah, R. Gogoi, N. Gogoi and G. Borah, Transition Met. Chem., 2017, 42, 683–692 CrossRef CAS;
(d) P. Paul, P. Sengupta and S. Bhattacharya, J. Organomet. Chem., 2013, 724, 281–288 CrossRef CAS;
(e) I. D. Kostas and B. R. Steele, Catalysts, 2020, 10, 1107 CrossRef CAS;
(f) S. Priyarega, J. Haribabu and R. Karvembu, Inorg. Chim. Acta, 2022, 532, 120742 CrossRef CAS;
(g) S. Datta, D. K. Seth, S. Gangopadhyay, P. Karmakar and S. Bhattacharya, Inorg. Chim. Acta, 2012, 392, 118–130 CrossRef CAS.
- A. I. Matesanz, J. M. Herrero and A. G. Quiroga, Curr. Med. Chem., 2021, 21, 59–72 CAS.
- V. Singh, V. N. V. Palakkeezhillam, V. Manakkadan, P. Rasin, A. K. Valsan, V. S. Kumar and A. Sreekanth, Polyhedron, 2023, 245, 116658 CrossRef CAS.
-
(a) P. P. Netalkar, S. P. Netalkar and V. K. Revankar, Polyhedron, 2015, 100, 215–222 CrossRef CAS;
(b) A. Gaber, M. S. Refat, A. A. M. Belal, I. M. El-Deen, N. Hassan, R. Zakaria, M. Alhomrani, A. S. Alamri, W. F. Alsanie and E. M. Saied, Molecules, 2021, 26 CrossRef CAS.
-
(a) G. Pelosi, F. Bisceglie, F. Bignami, P. Ronzi, P. Schiavone, M. C. Re, C. Casoli and E. Pilotti, J. Med. Chem., 2010, 53, 8765–8769 CrossRef CAS PubMed;
(b) P. Padmanabhan, S. Khaleefathullah, K. Kaveri, G. Palani, G. Ramanathan, S. Thennarasu and U. Tirichurapalli Sivagnanam, J. Med. Virol., 2017, 89, 546–552 CrossRef CAS PubMed.
-
(a) H. Huang, Q. Chen, X. Ku, L. Meng, L. Lin, X. Wang, C. Zhu, Y. Wang, Z. Chen, M. Li, H. Jiang, K. Chen, J. Ding and H. Liu, J. Med. Chem., 2010, 53, 3048–3064 CrossRef CAS;
(b) N. Muhammad and Z. Guo, Curr. Opin. Chem. Biol., 2014, 19, 144–153 CrossRef CAS PubMed.
-
(a) R. A. Finch, M. C. Liu, A. H. Cory, J. G. Cory and A. C. Sartorelli, Adv. Biol. Regul., 1999, 39, 3–12 CrossRef CAS;
(b) S. Plamthottam, D. Sun, J. van Valkenburgh, J. Valenzuela, B. Ruehle, D. Steele, S. Poddar, M. Marshalik, S. Hernandez, C. G. Radu and J. I. Zink, J. Biol. Inorg. Chem., 2019, 24, 621–632 CrossRef PubMed;
(c) S. Huang, D. Zhang, X. Yi, C. Liu, C. Jian and A. Yu, Med. Oncol., 2023, 40, 353 CrossRef CAS PubMed.
- H. Beraldo and D. Gambino, Mini-Rev. Med. Chem., 2004, 4, 31–39 CrossRef CAS PubMed.
-
(a) T. Hidalgo, D. Fabra, R. Allende, A. I. Matesanz, P. Horcajada, T. Biver and A. G. Quiroga, Inorg. Chem. Front., 2023, 10, 1986–1998 RSC;
(b) J. M. Herrero, D. Fabra, A. I. Matesanz, C. Hernández, I. Sánchez-Pérez and A. G. Quiroga, J. Inorg. Biochem., 2023, 246, 112261 CrossRef CAS;
(c) K. Dhariyal, S. Parveen, S. Kumar, M. Banerjee, P. Sharma, S. K. Singh and A. K. Singh, Inorg. Chem. Commun., 2023, 152, 110678 CrossRef CAS.
-
(a) S. Dasari and P. B. Tchounwou, Eur. J. Pharmacol., 2014, 740, 364–378 CrossRef CAS;
(b) D. Wang and S. J. Lippard, Nat. Rev. Drug Discovery, 2005, 4, 307–320 CrossRef CAS;
(c) E. Raymond, S. G. Chaney, A. Taamma and E. Cvitkovic, Ann. Oncol., 1998, 9, 1053–1071 CrossRef CAS PubMed;
(d) J. J. Wilson and S. J. Lippard, Chem. Rev., 2014, 114, 4470–4495 CrossRef CAS PubMed;
(e) X. Wang and Z. Guo, Chem. Soc. Rev., 2013, 42, 202–224 RSC.
-
(a) W. Liu and R. Gust, Chem. Soc. Rev., 2013, 42, 755–773 RSC;
(b) I. Kostova, Curr. Med. Chem., 2006, 13, 1085–1107 CrossRef CAS.
-
(a) M. Cini, T. D. Bradshaw and S. Woodward, Chem. Soc. Rev., 2017, 46, 1040–1051 RSC;
(b) E. Meléndez, Crit. Rev. Oncol. Hematol., 2002, 42, 309–315 CrossRef PubMed;
(c) K. M. Buettner and A. M. Valentine, Chem. Rev., 2012, 112, 1863–1881 CrossRef CAS PubMed;
(d) P. Köpf-Maier, Eur. J. Pharmacol., 1994, 47, 1–16 Search PubMed.
-
(a) G. Vatsa, O. P. Pandey and S. K. Sengupta, Bioinorg. Chem. Appl., 2005, 3, 151–160 CrossRef CAS PubMed;
(b) S. K. Sengupta, O. P. Pandey, B. K. Srivastava and V. K. Sharma, Transition Met. Chem., 1998, 23, 349–353 CrossRef CAS.
-
(a) M. Manßen, N. Lauterbach, J. Dörfler, M. Schmidtmann, W. Saak, S. Doye and R. Beckhaus, Angew. Chem., Int. Ed., 2015, 54, 4383–4387 CrossRef PubMed;
(b) M. Manßen, S. de Graaff, M.-F. Meyer, M. Schmidtmann and R. Beckhaus, Organometallics, 2018, 37, 4506–4514 CrossRef;
(c) T. Oswald, T. Gelert, C. Lasar, M. Schmidtmann, T. Klüner and R. Beckhaus, Angew. Chem., Int. Ed., 2017, 56, 12297–12301 CrossRef CAS PubMed.
-
(a) M. Eilers, K. Schwitalla, T. Dirksen, M. Schmidtmann, M. Fischer and R. Beckhaus, Organometallics, 2023, 42, 1043–1047 CrossRef CAS;
(b) K. Schwitalla, W. Lee, I. Töben, M. Eilers, M. Schmidtmann and R. Beckhaus, Z. Anorg. Allg. Chem., 2024, 650, e202300230 CrossRef CAS.
- K. Schwitalla, F. Sad, M. Schmidtmann and R. Beckhaus, Inorg. Chem., 2024, 63, 3165–3172 CrossRef CAS PubMed.
- L. Wanka, K. Iqbal and P. R. Schreiner, Chem. Rev., 2013, 113, 3516–3604 CrossRef CAS PubMed.
- K. Schwitalla, Z. Yusufzadeh, M. Schmidtmann and R. Beckhaus, Inorg. Chem., 2024, 14392–14401 CrossRef CAS PubMed.
- N. A. Mazlan, T. B. S. A. Ravoof, E. R. T. Tiekink, M. I. M. Tahir, A. Veerakumarasivam and K. A. Crouse, Transition Met. Chem., 2014, 39, 633–639 CrossRef CAS.
-
(a) W. R. Gunther, V. K. Michaelis, R. G. Griffin and Y. Román-Leshkov, J. Phys. Chem. C, 2016, 120, 28533–28544 CrossRef CAS PubMed;
(b) W. Jiang, L. Lumata, W. Chen, S. Zhang, Z. Kovacs, A. D. Sherry and C. Khemtong, Sci. Rep., 2015, 5, 9104 CrossRef PubMed.
- P. Pyykkö and M. Atsumi, Chem. – Eur. J., 2009, 15, 186–197 CrossRef PubMed.
- P. Pyykkö and M. Atsumi, Chem. – Eur. J., 2009, 15, 12770–12779 CrossRef PubMed.
- M. Diekmann, G. Bockstiegel, A. Lützen, M. Friedemann, W. Saak, D. Haase and R. Beckhaus, Organometallics, 2006, 25, 339–348 CrossRef CAS.
- C. A. Tolman, Chem. Soc. Rev., 1972, 1, 337 RSC.
-
(a) R. Gyepes, P. T. Witte, M. Horáček, I. Císařová and K. Mach, J. Organomet. Chem., 1998, 551, 207–213 CrossRef CAS;
(b) D. R. Corbin, L. C. Francesconi, D. N. Hendrikson and G. D. Stucky, Inorg. Chem., 1979, 18, 3069–3074 CrossRef CAS;
(c) P. T. Witte, R. Klein, H. Kooijman, A. L. Spek, M. Polášek, V. Varga and K. Mach, J. Organomet. Chem., 1996, 519, 195–204 CrossRef CAS;
(d) T. Beweries, F. Reiß, J. Rothe, A. Schulz and A. Villinger, Eur. J. Inorg. Chem., 2019, 1993–1998 CrossRef CAS.
- K. Schwitalla, J. Klimek, T. Greven, M. Schmidtmann and R. Beckhaus, ACS Omega, 2024, 9, 29017–29024 CrossRef CAS PubMed.
|
This journal is © The Royal Society of Chemistry and the Centre National de la Recherche Scientifique 2024 |
Click here to see how this site uses Cookies. View our privacy policy here.