DOI:
10.1039/D4OB00433G
(Communication)
Org. Biomol. Chem., 2024,
22, 3589-3591
Total synthesis of diplofuranone A and diapolic acid A†
Received
16th March 2024
, Accepted 9th April 2024
First published on 16th April 2024
Abstract
The first and concise syntheses of the anticancer agent diplofuranone A and the fatty acid-derived metabolite diapolic acid A have been demonstrated using easily accessible and commercially available starting materials. The key feature of these syntheses is the efficient diversification of highly stereo- and chemoselectively constructed (E,E)-1,6-dioxo-2,4-dienes using ruthenium catalytic conditions, which enabled straightforward access to diversely substituted bioactive molecules.
Furanyl lactones or γ-lactones isolated from various phytopathogenic fungi such as Biscognauxia mediterranea, Diplodia corticola, Diplodia mutila and Diplodia quercina are known to exhibit potent biological activities.1 These fungal species are well known as phytotoxin producers and are abundant sources of secondary bioactive metabolites, and some of them show strong activity on host and non-host plants.1 In particular, Diplodia corticola produces structurally diverse and effective plant metabolites including diplofuranones A (1) and B (2), sapinofuranones A–C (3–5) and diplobifuranylone B (6) (Fig. 1).2 Diplofuranone A (1) is isolated from liquid cultures of Diplodia corticola, the anamorph of Botryosphaeria corticola, which is an endophytic fungus that is widespread in Sardinian oak forests. This fungus can affect plants of different ages, inducing symptoms that include dieback, cankers and vascular necrosis.2 Also, diplofuranone A, a metabolite of the plant pathogenic fungus causing the canker disease of cork oak, is known for in vitro growth inhibitory activity against human and mouse cancer cell lines.2 Diplofuranone A tested at 0.2 mg mL−1 on non-host plants did not show phytotoxic activity. Diplofuranone A (1) is a furanyl lactone and its absolute configuration is (4S,9R).2 The absolute configuration of diplofuranone on the secondary hydroxylated carbon of the side chain was determined by Evidente,2 and later, that on the stereogenic carbon atom of the lactone moiety was assigned by Abbate.2 In 2010, stereoselective total synthesis of (+) sapinofuranone B was achieved in 13 steps using L-(+) diethyl tartrate as a starting material.3a Later, in 2019, the Zhang group reported the asymmetric total synthesis of diplobifuranylone B, which was completed in 10 steps, the longest linear sequence, from commercially available methyl (R)-(+)-lactate and L-glutamic acid.4a Although a few syntheses are reported for sapinofuranones3a–d and diplobifuranylones,4a,b so far, there is no synthesis reported for the potent bioactive molecule diplofuranone A. Hence, the development of a concise synthetic route to bioactive γ-lactone, diplofuranone A (1) is highly desirable. In continuation of our programme toward new reaction development and total synthesis of bioactive molecules,5 we have reported a ruthenium-catalyzed protocol for the oxidative cross-coupling of vinyl ketones/vinyl ketone and acrylate.6 This straightforward and atom-economical methodology provides efficient access to highly stereo- and chemoselective synthesis of (E,E)-1,6-dioxo-2,4-dienes. The synthetic potential of this reaction has been further demonstrated for the highly efficient and concise synthesis of various bioactive natural products.6,7 By utilizing the transformative power of stereodefined 1,6-dioxo-2,4-dienes, herein, we report the first and four-step synthesis of the anticancer agent diplofuranone A (1) and the three-step synthesis of diapolic acid A (7), a fatty acid-derived metabolite.
 |
| Fig. 1 Structures of bioactive γ-lactones and diapolic acid A. | |
The retrosynthetic strategy for diplofuranone A (1) is delineated in Scheme 1 by utilizing our key strategy for the synthesis of (E,E)-1,6-dioxo-2,4-dienes as pivotal transformation. We envisaged that diplofuranone A could be easily obtained from stereodefined (E,E)-1,6-dioxo-2.4-diene 8 by reduction/concomitant lactone formation. The stereodefined diene 8 could be easily accessed using our developed protocol, namely, the Ru-catalyzed oxidative coupling reaction of methyl vinyl ketone 9 and vinyl ketone derivative 10. The vinyl ketone derivative 10 can be synthesized from commercially available succinic anhydride 11 (100 g per $10) by ring opening with vinyl magnesium bromide followed by methyl esterification of an acid intermediate.
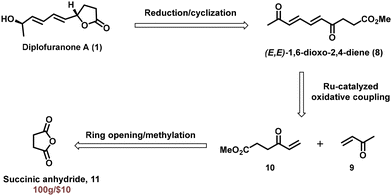 |
| Scheme 1 Retrosynthetic analysis of diplofuranone A, 1. | |
To begin with, key intermediate 8 was synthesized from succinic anhydride 11 using a two-step procedure (Scheme 2). Succinic anhydride 11 upon ring opening with vinyl magnesium bromide afforded an acid intermediate in 60% yield. The in situ generated acid was converted into methyl ester under K2CO3/MeI conditions to obtain an oxidative coupling precursor 10 in 80% yield. The vinyl ketone derivative 10 was then subjected to the ruthenium-catalyzed oxidative coupling reaction with methyl vinyl ketone 9 which delivered the crucial intermediate (E,E)-1,6-dioxo-2,4-diene derivative 8 in 68% yield. Notably, the homodimer of methyl vinyl ketone was obtained as a minor side product in 10% yield. Finally, NaBH4-mediated reduction of both the carbonyl groups in compound 8 followed by concomitant lactone formation completed the synthesis of diplofuranone A 1 in 89% yield.
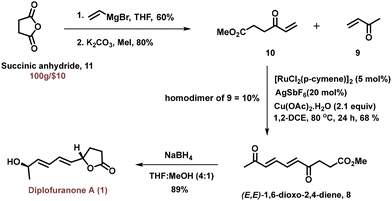 |
| Scheme 2 Highly concise synthesis of diplofuranone A (1). | |
Interestingly, NaBH4 reduction of compound 8 generated a single diastereomer, and this selectivity could be attributed to conformational rigidity due to the presence of two trans olefins in compound 8. Thus, diplofuranone A 1 was synthesized from commercially available succinic anhydride in just 4 steps with an overall yield of 28% in a highly regio- and diastereoselective manner.
After successfully completing the synthesis of diplofuranone A 1, we thought of applying our developed catalytic method in the synthesis of another bioactive natural product containing stereodefined 1,6-dioxo-2,4-diene, diapolic acid A 7. Diapolic acid A (7) is a novel hydroxylated unsaturated fatty acid obtained from the fungal endophyte Diaporthe terebinthifolii GG3F6, isolated from the rhizome of G. glabra collected from Jammu, J&K, India.8 The nucleotide sequence showed 99% homology with Diaporthe terebinthifolii. This biologically active secondary metabolite from endophytic fungi harbored in traditionally used Indian medicinal plants shows excellent cytotoxic and antimicrobial activities. Notably, there are no previous synthetic reports in the literature for diapolic acid A 7. We envisioned that diapolic acid A 7 could be easily synthesized by employing our developed ruthenium-catalyzed oxidative cross-coupling reaction as a pivotal transformation (Scheme 3). The synthesis initiated with ruthenium-catalyzed oxidative cross-coupling of commercially available methyl acrylate 12 with propyl vinyl ketone 13. This pivotal transformation was successful in affording the desired cross-coupled product (E,E)-1,6-oxo-ester-2,4-diene derivative 14 in 65% yield along with the homodimer of 12 as a minor side product (8% yield). Next, derivative 14 was subjected to chemoselective reduction of the keto group under NaBH4 conditions to get the corresponding ester–alcohol derivative 15 in 90% yield. Finally, LiOH-mediated methyl ester hydrolysis of compound 15 completed the synthesis of diapolic acid A 7 in 84% yield.
 |
| Scheme 3 Three-step synthesis of the metabolite diapolic acid A (7). | |
Conclusions
In summary, we have disclosed a concise four-step synthetic strategy for the anticancer agent diplofuranone A with an overall yield of 28%. Also, the first and three-step synthesis of the secondary metabolite diapolic acid A has been described with an overall yield of 50%. Highly efficient and shortest synthetic strategies for diplofuranone A and diapolic acid A have been illustrated by employing a step- and atom-economical ruthenium-catalyzed oxidative cross-coupling reaction for the chemo- and stereoselective synthesis of (E,E)-1,6-dioxo-2,4-dienes and highly diastereoselective reduction of carbonyl groups as pivotal transformation.
Author contributions
D. H. D. directed the project and wrote the manuscript. V. K. and N. C. B. contributed equally.
Conflicts of interest
The authors declare no competing financial interest.
Acknowledgements
V. K. thanks IIT Kanpur for a research fellowship. N. C. B. thanks the UGC, New Delhi, for the award of a research fellowship. Financial support from the SERB Project No. CRG/2022/002967 and the CSIR Project no. 02(0412)/21/EMR-II is gratefully acknowledged.
References
-
(a)
A. Vidente, A. Andolfi, A. Cimmino and M. A. Abouzeid, in Sustainable Agriculture, ed. A. Salazar and I. Rios, Nova Science Publishers, Inc., New York, 2010, pp. 177–234 Search PubMed;
(b) R. Ling, M. Yoshida and P. S. Mariano, J. Org. Chem., 1996, 61, 4439–4449 CrossRef CAS PubMed;
(c) H. Kiyota, Q.-W. Shi and T. Oritani, Nat. Prod. Lett., 2007, 16, 21–24 CrossRef PubMed;
(d) K. W. Cho, H.-S. Lee, J.-R. Rho, T.-S. Kim, S. J. Mo and J. Shin, J. Nat. Prod., 2001, 64, 664–667 CrossRef CAS PubMed;
(e) S. Gunasekera, P. R. A. Isbrucker, R. E. Longley, A. E. Wright, S. A. Pomponi and J. K. Reed, J. Nat. Prod., 2004, 67, 110–111 CrossRef CAS PubMed.
-
(a) G. Mazzeo, A. Cimmino, M. Masi, G. Longhi, L. Maddau, M. Memo, A. Evidente and S. Abbate, J. Nat. Prod., 2017, 80, 2406–2415 CrossRef CAS PubMed;
(b) A. Evidente, A. Andolfi, M. Fiore, E. Spanu, A. Franceschini and L. Maddau, ARKIVOC, 2007,(vii), 318–328 Search PubMed.
-
(a) J. S. Yadav, S. S. Mandal, J. S. S. Reddy and P. Srihari, Tetrahedron, 2011, 67, 4620–4627 CrossRef CAS;
(b) P. Kumar, S. V. Naidu and P. Gupta, J. Org. Chem., 2005, 70, 2843–2846 CrossRef CAS PubMed;
(c) K. S. N. Reddy, G. Sabitha, Y. Poornachandra and C. G. Kumar, RSC Adv., 2016, 6, 101501–101512 RSC;
(d) L. Nagarapu, S. Karnakanti and R. Bantu, Tetrahedron, 2012, 68, 5829–5832 CrossRef CAS.
-
(a) X. Cheng, C. D. Quintanilla and L. Zhang, J. Org. Chem., 2019, 84, 11054–11060 CrossRef CAS PubMed;
(b) Y. Wei, J. Lam and T. Diao, Chem. Sci., 2021, 12, 11414–11419 RSC.
-
(a) D. H. Dethe and C. B. Nagabhushana, Org. Lett., 2020, 22, 1618–1623 CrossRef CAS PubMed;
(b) D. H. Dethe, C. B. Nagabhushana, S. Das and A. K. Nirpal, Chem. Sci., 2021, 12, 4367–4372 RSC.
- D. H. Dethe, A. K. Nirpal, C. B. Nagabhushana, V. Kumar, A. Srivastava, K. B. Mishra and A. A. Bhat, Chem. Commun., 2022, 58, 3063–3066 RSC.
-
(a) D. H. Dethe, V. Kumar, C. B. Nagabhushana, K. B. Mishra and A. K. Nirpal, Org. Lett., 2022, 24, 2203–2207 CrossRef CAS PubMed;
(b) D. H. Dethe, A. Srivastava, A. K. Nirpal, C. B. Nagabhushana, V. Kumar and A. A. Bhat, J. Org. Chem., 2022, 87, 11021–11030 CrossRef CAS PubMed.
- N. Yedukondalu, P. Arora and B. Wadhwa, J. Antibiot., 2017, 70, 212–215 CrossRef CAS PubMed.
Footnotes |
† Electronic supplementary information (ESI) available: Further details of the experimental procedure and 1H, 13C, and NMR spectra. See DOI: https://doi.org/10.1039/d4ob00433g |
‡ Both authors contributed equally. |
|
This journal is © The Royal Society of Chemistry 2024 |
Click here to see how this site uses Cookies. View our privacy policy here.