Gold(I)-catalysed cyclisation of (E)-ketene-N,O-acetals: a synthetic route toward spiro-oxazole-γ-lactones†‡
Received
4th April 2024
, Accepted 19th May 2024
First published on 20th May 2024
Abstract
In this study, we developed a cascade 5,5-cyclisation of internal ketene-N,O-acetals utilizing homogeneous Au(I) catalysis. This process involves an initial 5-exo-dig carbocyclisation, followed by a 5-exo-dig heterocyclisation that stereoselectively incorporates the O-atom of a water molecule into an N-tethered propargyl alkyne. This sequential reaction results in the formation of one C–C, two C–O, and two C–I bonds, ultimately leading to the synthesis of spiro-α-iodo-γ-lactone structures featuring oxazole rings in good yields.
Spirocycles1 are a unique class of molecular structures with prominent three-dimensional characteristics, often found in a variety of natural products and medicinal compounds (Fig. 1a).2,3 Despite their importance, potential methods for the synthesis of functionalized spirocycles have not been fully explored. Notably, gold-catalysed oxidative cyclisation and cycloisomerisation reactions have played vital roles in the efficient crafting of such complex spirocyclic architectures.
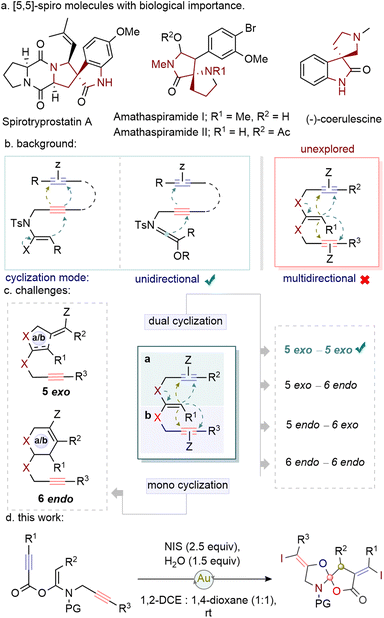 |
| Fig. 1 Background and this work. | |
Ynamides, with their unique polarized nature, exhibit both electrophilic and nucleophilic reactive sites.4 Consequently, alkyne tethered ynamides are amenable to various cyclisation processes. In this context, gold-catalysed activations of alkynes followed by targeted cyclisations have provided a unique means to understand annulation modules (Fig. 1b).5 In this connection, 6/5-endo-dig and 5-exo-dig cyclisation/cycloisomerisation reactions of propargyl ynamides have helped build a variety of N-heterocycles such as dihydropyridinones, benzoisoquinolenes, pyrrolidones, spiro-pyrrolidones, and cyclobutene-fused azepines.5–8 However, the pursuit of a multidirectional strategy, aiming at precise control over specific cyclisation paths among several options, remains a challenging yet promising area for further investigation.
Building upon our recent finding, we hypothesize that subjecting ketene-N,O-acetals to gold catalysis can result in the poly-activation of alkyne moieties, paving the way for a multidirectional cyclisation pathway. However, controlling the mono-cyclisation mode (5-exo/6-endo) for the realization of selective dual cyclisation modes (5-exo-5-exo, 5-exo-6-endo, 5-endo-6-exo, and 6-endo-6-endo) [Scheme 1c] is challenging. In this study, we introduce a highly regioselective multidirectional 5,5-exo-dig cyclisation of di-alkyne tethered ketene-N,O-acetals catalysed by gold. This strategy enables the efficient synthesis of diverse spiro molecular frameworks from (E)-ketene-N,O-acetals.
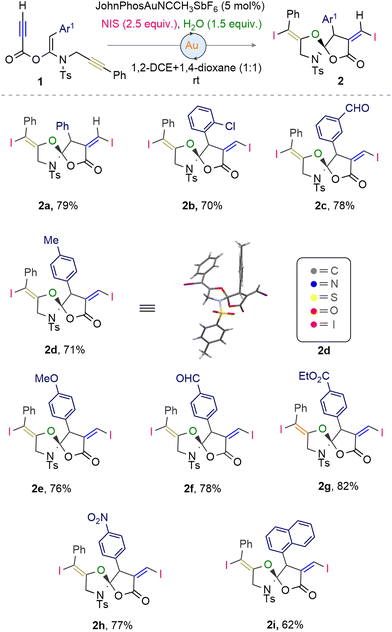 |
| Scheme 1 Substrate scope.a Reaction conditions: 1 (0.25 mmol), catalyst (5.0 mol%), NIS (2.5 equiv.), H2O (1.5 equiv.) in 1,2-DCE : 1,4-dioxane (1 : 1, 0.05 M). aIsolated yield. | |
The study commenced with the reaction of the ketene-N,O-acetals 1a using PPh3AuCl (5.0 mol%) and AgSbF6 (5.0 mol%) in 1,2-dichloroethane at room temperature, yielding the enediyne-cyclisation product 2a in a moderate 38% yield (Table 1, entry 1). The employment of AgNTf2 resulted in a lower 26% yield of 2a and the decomposition of the starting material 1a (entry 2).
Table 1 Screening of reaction conditionsa
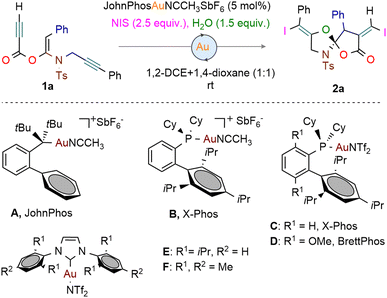
|
Entry |
Catalyst |
X-source |
H2O equiv. |
Solvent |
2a Yield (%) |
Reaction conditions: 1 (0.25 mmol), catalyst (5.0 mol%) in 1,2-DCE : 1,4-dioxane (1 : 1, 0.05 M). Isolated yields. |
1 |
PPh3AuCl/AgSbF6 |
NIS (2) |
2 |
1,2-DCE |
38 |
2 |
PPh3AuCl/AgNTf2 |
NIS (2) |
2 |
1,2-DCE |
26 |
3 |
A
|
NIS (2) |
2 |
1,2-DCE |
55 |
4 |
B
|
NIS (2) |
2 |
1,2-DCE |
39 |
5 |
C
|
NIS (2) |
2 |
1,2-DCE |
25 |
6 |
D
|
NIS (2) |
2 |
1,2-DCE |
16 |
7 |
E
|
NIS (2) |
2 |
1,2-DCE |
Complex |
8 |
F
|
NIS (2) |
2 |
1,2-DCE |
Complex |
9 |
A
|
NIS (2) |
2 |
DCM |
48 |
10 |
A
|
NIS (2) |
2 |
THF |
31 |
11 |
A
|
NIS (2) |
2 |
CH3NO2 |
24 |
12 |
A
|
NIS (2) |
2 |
1,4-Dioxane |
57 |
13 |
A
|
NIS (2) |
2 |
DCE/dioxane (1 : 1) |
69 |
14 |
A
|
NIS (2.5) |
2 |
DCE/dioxane (1 : 1) |
77 |
15 |
A
|
NIS (2.5) |
1.5 |
DCE/dioxane (1 : 1) |
79 |
A significant improvement was achieved with the JohnPhos ligated gold(I) catalyst A, leading to 55% yield of the desired product 2a (entry 3).
However, the use of other Au-catalysts, such as CyJohnPhosgold-SbF6 (B), and those with bulkier phosphine ligands, such as Xphosgold-NTf2 (C) and Brettphosgold-NTf2 (D), did not enhance the yield (entries 4–6). Attempts with the N-heterocyclic carbene (NHC)-based gold catalysts E and F provided a complex mixture (entries 7 and 8). Various parameters were tested, including the water-to-enyne ratio, N-iodosuccinimide (NIS) concentration, solvents, and reaction concentration (Table 1, entries 9–12). The addition of 2.0 equiv. of NIS and 2.0 equiv. of water in 1,4-dioxane improved the yield to 57% (entry 12). Optimally, a 1
:
1 mixture of DCE
:
1,4-dioxane with 2.5 equiv. of NIS and 1.5 equiv. of water yielded 79% of the enediyne-cyclisation product 2a (entry 15). Substituting NIS with NBS resulted in a complex reaction profile, whereas other iodine sources failed to produce the desired product.10
Having established the optimized conditions, we proceeded to investigate the substrate scope and functional-group tolerance of the cyclisation reaction of the propiolic acid derived ketene-N,O-acetals that offers access to the synthesis of peripherally-decorated 1,6-dioxa-4-azaspiro[4.4]nonan-7-one skeletons. We began by testing substrates with modifications at the enamide–alkene terminus. The outcome showed that ketene-N,O-acetals with both electron-neutral and electron-donating groups (p-Me and p-OMe) and electron-withdrawing groups (o-Cl, m-CHO, p-CHO, p-CO2Et, and p-NO2) on the aryl ring successfully yielded the desired [5,5]-spiro compounds 2a–h in 70–82% yields. The crystal structure of 2d was verified using SC-XRD analysis (CCDC 2120244‡). Additionally, ketene-N,O-acetals featuring bulky 1-naphthyl substitutions at the ynamide terminus were compatible, producing compound 2i in a good yield (Scheme 1).
Next, we explored the reactivity of ketene-N,O-acetals with varied substitutions on the propargyl terminus. Substrates with halo and electron-withdrawing substituents at the aryl ring (p-Cl, p-Br, m-Me, and m-NO2) produced the corresponding products 3a (75%), 3b (78%), 3c (67%), and 3d (72%). The 1-thienyl-based ketene-N,O-acetal is not the exception, delivering 69% yield of the desired product 3e. Likewise, the spiro compounds 3f and g were obtained from the alkyl substituted and unsubstituted propargyl terminus of the respective ketene-N,O-acetals in good yields.
The labile N-Ms-protected spiro compound 3h is synthesized in 62% yield (Scheme 2). To further expand the structural diversity of the spirocycles, a Pd-catalyzed Suzuki cross-coupling of the iodo-moiety of spirocycle 3c with the p-methoxyphenyl-boronic acid 4 was performed, which led to the successful synthesis of compound 5 in 58% yield (Scheme 3a).
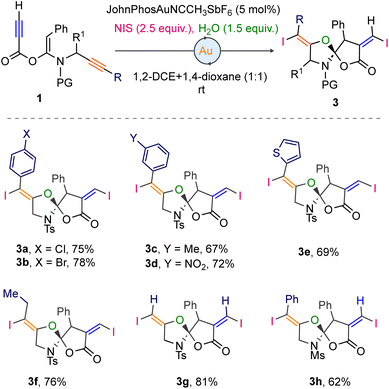 |
| Scheme 2 Substrate scope.a Reactions conditions: 1 (0.25 mmol), catalyst (5.0 mol%), NIS (2.5 equiv.), H2O (1.5 equiv.) in 1,2-DCE : 1,4-dioxane (1 : 1, 0.05 M). aIsolated yield. | |
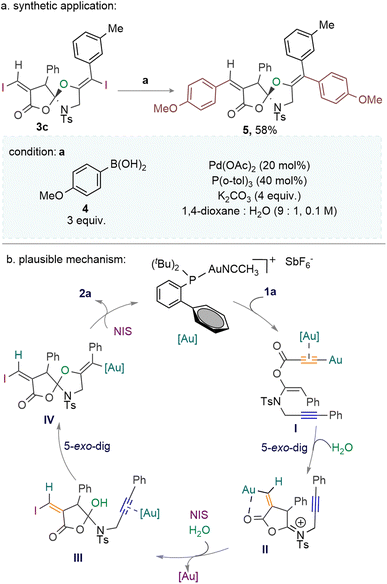 |
| Scheme 3 Synthetic application and plausible mechanism. | |
Based on the observed reactivity, we propose a plausible catalytic cycle (Scheme 3). The reaction begins with the coordination of the cationic JohnPhosAuNCCH3SbF6 catalyst with the nucleophilic terminal alkyne of ketene-N,O-acetal 1a to form the gold-acetylide.9 We believe the gold-acetylide formation through σ-activation of the terminal alkyne is facile compared to the π-activation of the internal alkyne.9 Meanwhile, the simultaneous π-activation by the carbophilic gold-catalyst could provide the dual activated [Au]-species Int-I; although the formation of such an intermediate is less probable, it cannot be ruled out.9 Consequently, the intramolecular 5-exo-dig cyclisation of Int-I provides the vinyl-[Au] intermediate II.11 The trap of water by the iminium-moiety and the deaurative iodination of vinyl-[Au] of Int-II then forms Int-III. Next, the intramolecular 5-exo-dig hetero-cyclisation of aminol to the [Au]-activated alkyne yields the core spirocyclic-vinyl-[Au] intermediate IV. Finally, a second deaurative iodination of Int-IV produces the spirocyclic product 2a with the regeneration of the active gold species.
Conclusions
In summary, we have established a protocol for an Au(I)-catalysed cascade double-5-exo-dig cyclisation utilizing ketene-N,O-acetals. This method facilitates the synthesis of α-methylene-γ-amino-butyrolactones by incorporating isoxazole moieties. Operating under mild conditions, this process demonstrates compatibility with various functional groups and the reaction is scalable. We believe that these spiro-bicyclic lactones hold promise for applications in the pharmaceutical industry, owing to their unique structural characteristics and potential pharmacological relevance.
Experimental
Materials
Unless otherwise noted, all the reagents and intermediates were obtained commercially and used without purification. 1,4-Dioxane, dichloromethane (CH2Cl2; DCM), toluene, acetonitrile (CH3CN), 1,2-dichloroethane (DCE), and acetone were distilled over CaH2. THF was freshly distilled over sodium/benzophenone ketyl under dry nitrogen. All the gold catalysts were purchased from Sigma Aldrich Ltd and used as received. Silver salts such as AgSbF6, AgNTf2, AgBF4, and NIS were purchased from Aldrich Ltd and used as received. PPh3, DEAD, CuSO4·H2O, 1,10-phenanthroline, K3PO4, Na2CO3, and Na2S2O3·5H2O were purchased from Merck. The aryl iodides were purchased from Aldrich and used. Analytical and spectral data of all those known compounds matched exactly with the reported values.
Experimental procedures
General procedure for the Au-catalyzed cascade double 5-exo-dig cyclization of 1: (GP-4)
To a screw capped tube were added, under an argon atmosphere, a solution of 1 (0.25 mmol) in 1,4-dioxane (3.0 mL) and NIS (2.5 equiv.). The catalyst JohnphosAuSbF6 (A; 7.6 mg, 5 mol%) in DCE (3.0 mL) was next introduced along with 1.5 equiv. of H2O. The reaction mixture was stirred for 6–8 h. The reaction progress was periodically monitored by TLC. After 8 h, the reaction mixture was quenched with saturated sodium thiosulfate solution and extracted with ethyl acetate (3 × 5 mL). The combined organic layers were dried over Na2SO4. The solvent was evaporated under reduced pressure and the residue was purified by flash chromatography on silica gel (hexane/EtOAc) to afford the expected products 2a–2i and 3a–3h.
(2E,8Z)-2-(Iodo(phenyl)methylene)-8-(iodomethylene)-9-phenyl-4-tosyl-1,6-dioxa-4-azaspiro[4.4]nonan-7-one (2a)
2a was obtained in 79% yield as a pale-yellow solid; Rf = 0.45 (4
:
1 hexane/EtOAc); [Silica, UV and I2]. IR (Neat)νmax 2983, 1735, 1376, 1214, 1179, 1135, 1099, 865, 733 cm−1. 1H NMR (400 MHz, CDCl3): δ = 7.84 (d, J = 8.4 Hz, 2H), 7.44 (d, J = 8 Hz, 2H), 7.41–7.34 (m, 3H), 7.29–7.26 (m, 1H), 7.26–7.23 (m, 2H), 7.21–7.16 (m, 3H), 7.00–6.91 (m, 2H), 5.41 (d, J = 2.8 Hz, 1H), 4.41 (d, J = 13.6 Hz, 1H), 4.18 (d, J = 13.6 Hz, 1H), 2.49 (s, 3H). 13C NMR (101 MHz, CDCl3): δ = 165.8, 145.3, 143.6, 136.5, 133.4, 133.3, 133.1, 130.2, 129.9, 128.8, 128.6, 128.4, 128.0, 127.8, 128.4, 125.3, 117.6, 101.6, 54.0, 49.4, 21.6. HRMS (ESI): m/z calcd for C27H21I2NNaO5S (M + Na)+: 747.9127, found: 747.9121.
(2E,8Z)-9-(2-Chlorophenyl)-2-(iodo(phenyl)methylene)-8-(iodomethylene)-3-methyl-4-tosyl-1,6-dioxa-4-azaspiro[4.4]nonan-7-one (2b)
2b was obtained in 70% yield as a pale-yellow gummy liquid; Rf = 0.58 (4
:
1 hexane/EtOAc); [Silica, UV and I2]. IR (Neat)νmax 2977, 1766, 1385, 1209, 1176, 1112, 1067, 852, 746 cm−1; 1H NMR (500 MHz, CDCl3): δ = 7.87 (d, J = 8.4 Hz, 2H), 7.48 (m, 1H), 7.43 (d, J = 8.0 Hz, 2H), 7.34 (d, J = 2.8 Hz, 1H), 7.32–7.26(m, 2H), 7.16–7.08 (m, 3H), 7.02–6.97 (m, 1H), 6.99 (dd, J = 7.5, 1.5 Hz, 1H), 6.84–6.82 (m, 1H), 5.98 (d, J = 3.0 Hz, 1H), 4.46 (d, J = 14.0 Hz, 1H), 4.13 (d, J = 14.0 Hz, 1H), 2.48 (s, 3H). 13C NMR (126 MHz, CDCl3): δ = 163.3, 145.5, 144.5, 136.6, 136.2, 135.7, 133.2, 132.5, 131.4, 130.0, 129.8, 129.6, 129.3, 129.2, 129.1 128.9, 127.9, 127.7, 127.6, 126.9, 116.3, 92.7, 70.4, 54.5, 53.5, 21.7. HRMS (ESI): m/z calcd for C27H21ClI2NO5S (M + H)+: 759.8918; found: 759.8912.
3-((2E,8Z)-2-(Iodo(phenyl)methylene)-8-(iodomethylene)-7-oxo-4-tosyl-1,6-dioxa-4-azaspiro[4.4]nonan-9-yl)benzaldehyde (2c)
2c was obtained in 78% yield as a pale-yellow gummy liquid; Rf = 0.51 (4
:
1 hexane/EtOAc); [Silica, UV and I2]. IR (Neat)νmax 2964, 1443, 1388, 1218, 1105, 1037, 865, 744 cm−1. 1H NMR (500 MHz, CDCl3): δ = 9.71 (s, 1H), 7.83 (m, 1H), 7.79 (d, J = 2.5 Hz, 2H), 7.59 (s, 1H), 7.48–7.44 (m, 2H), 7.38 (d, J = 8.5 Hz, 2H), 7.20 (m, 1H), 7.14–7.09 (m, 3H), 6.92–6.86 (m, 2H), 5.41 (d, J = 2.5 Hz, 1H), 4.34 (d, J = 14.0 Hz, 1H), 4.01 (d, J = 14.0 Hz, 1H), 2.43 (s, 3H). 13C NMR (101 MHz, CDCl3): δ = 191.4, 163.1, 145.8, 143.9, 136.9, 136.7, 135.8, 133.9, 132.9, 132.0, 130.1, 129.6, 129.4, 129.3, 129.0, 128.3, 128.1, 116.2, 92.0, 70.5, 57.3, 53.9, 21.7. HRMS (ESI): m/z calcd for C28H22I2NO6S (M + H)+: 753.9257; found: 753.9262.
(2E,8Z)-2-(Iodo(phenyl)methylene)-8-(iodomethylene)-9-(p-tolyl)-4-tosyl-1,6-dioxa-4-azaspiro[4.4]nonan-7-one (2d)
2d was obtained in 71% yield as a pale brown semi solid; Rf = 0.52 (4
:
1 hexane/EtOAc); [Silica, UV and I2]. IR (Neat)νmax 2987, 1753, 1382, 1242, 1139, 1090, 1053, 879, 723 cm−1. 1H NMR (500 MHz, CDCl3): δ = 7.86 (d, J = 8 Hz, 2H), 7.46 (d, J = 8.0 Hz, 2H), 7.27 (d, J = 7.0 Hz, 1H), 7.25–7.17 (m, 5H), 7.15 (d, J = 8.0 Hz, 2H), 7.04–6.98 (m, 2H), 5.38 (d, J = 3.0 Hz, 1H), 4.43 (d, J = 14.0 Hz, 1H), 4.11 (d, J = 14.0 Hz, 1H), 2.51 (s, 3H), 2.41 (s, 3H). 13C NMR (126 MHz, CDCl3): δ = 163.5, 145.6, 144.2, 138.7, 136.8, 136.3, 133.0, 130.5, 130.1, 129.54, 129.46 129.4, 128.9, 128.1, 127.8, 116.8, 91.5, 70.2, 57.2, 54.0, 21.7, 21.2. HRMS (ESI): m/z calcd for C28H24I2NO5S (M + H)+: 739.9465; found: 739.9458.
(2E,8Z)-2-(Iodo(phenyl)methylene)-8-(iodomethylene)-9-(4-methoxyphenyl)-4-tosyl-1,6-dioxa-4-azaspiro[4.4]nonan-7-one (2e)
2e was obtained in 76% yield as a pale brown semi solid; Rf = 0.52 (4
:
1 hexane/EtOAc); [Silica, UV and I2]. IR (Neat)νmax 3049, 2972, 1740, 1354, 1159, 1148, 1073, 852, 698 cm−1. 1H NMR (400 MHz, CDCl3): δ = 7.84 (d, J = 8.5 Hz, 2H), 7.43 (d, J = 8.0 Hz, 2H), 7.26–7.20 (m, 4H), 7.15 (d, J = 8.5 Hz, 2H), 7.06–7.02 (m, 2H), 6.87 (d, J = 8.5 Hz, 2H), 5.34 (d, J = 3.0 Hz, 1H), 4.41 (d, J = 14.0 Hz, 1H), 4.10 (d, J = 14.0 Hz, 1H), 3.84 (s, 3H), 2.49 (s, 3H). 13C NMR (101 MHz, CDCl3): δ = 163.5, 159.9, 145.6, 144.2, 136.8, 136.5, 133.0, 131.8, 130.0, 129.5, 128.9, 128.1, 127.8, 124.2, 116.8, 114.1, 91.3, 70.1, 56.9, 55.3, 53.9, 21.7. HRMS (ESI): m/z calcd for C28H24I2NO6S (M + H)+: 755.9414 found: 755.9422.
4-((2E,8Z)-2-(Iodo(phenyl)methylene)-8-(iodomethylene)-7-oxo-4-tosyl-1,6-dioxa-4-azaspiro[4.4]nonan-9-yl)benzaldehyde (2f)
2f was obtained in 78% yield as a pale-yellow semi solid; Rf = 0.41 (4
:
1 hexane/EtOAc); [Silica, UV and I2]. IR (Neat)νmax 2238, 1756, 1735, 1510, 1371, 1174, 1092 cm−1. 1H NMR (500 MHz, CDCl3): δ = 9.84 (s, 1H), 7.90 (m, 1H), 7.85 (d, J = 8.5 Hz, 2H), 7.65 (s, 1H), 7.55–7.50 (m, 2H), 7.45 (d, J = 8.0 Hz, 2H), 7.27 (d, J = 3.0 Hz, 1H), 7.22–7.15 (m, 3H), 6.98–6.94 (m, 2H), 5.48 (d, J = 3.0 Hz, 1H), 4.41 (d, J = 13.5 Hz, 1H), 4.08 (d, J = 13.5 Hz, 1H), 2.50 (s, 3H). 13C NMR (101 MHz, CDCl3): δ = 191.5, 163.1, 145.83, 143.8, 136.9, 136.7, 135.8, 133.8, 132.8, 132.0, 130.1, 129.6, 129.4, 129.3, 129.0, 128.3, 128.1, 116.2, 92.0, 70.5, 57.2, 53.8, 21.7. HRMS (ESI): m/z calcd for C28H22I2NO6S (M + H)+: 753.9257; found: 753.9260.
Ethyl 4-((2E,8Z)-2-(Iodo(phenyl)methylene)-8-(iodomethylene)-7-oxo-4-tosyl-1,6-dioxa-4-azaspiro[4.4]nonan-9-yl)benzoate (2g)
2g was obtained in 82% yield as a pale-yellow gummy liquid; Rf = 0.45 (4
:
1 hexane/EtOAc); [Silica, UV and I2]. IR (Neat)νmax 1787, 1706, 1443, 1360, 1159, 1092, 911, 823 cm−1. 1H NMR (500 MHz, CDCl3): δ = 7.89–7.82 (m, 4H), 7.32 (d, J = 8.0 Hz, 2H), 7.48–7.38 (m, 3H), 7.32 (d, J = 3.0 Hz, 1H), 7.27–7.22 (m, 2H), 7.03 (d, J = 8.5 Hz, 2H), 5.43 (d, J = 5.0 Hz, 1H), 4.42 (d, J = 14.0 Hz, 1H), 4.38 (q, J = 7.0 Hz, 2H), 4.10 (d, J = 14.0 Hz, 1H), 2.49 (s, 3H), 1.40 (t, J = 7.5 Hz, 3H).13C NMR (126 MHz, CDCl3): δ = 165.9, 163.3, 145.7, 145.5, 141.0, 135.7, 132.8, 132.3, 130.5, 130.0, 129.5, 129.0, 128.91, 128.87, 116.8, 92.2, 68.6, 61.1, 57.5, 54.1, 21.7, 14.3. HRMS (ESI): m/z calcd for C30H26I2NO7S (M + H)+: 797.9519, found 797.9519.
(2E,8Z)-2-(Iodo(phenyl)methylene)-8-(iodomethylene)-9-(4-nitrophenyl)-4-tosyl-1,6-dioxa-4-azaspiro[4.4]nonan-7-one (2h)
2h was obtained in 77% yield as a thick yellow liquid; Rf = 0.56 (4
:
1 hexane/EtOAc); [Silica, UV and I2]. IR (Neat)νmax 2231, 1768, 1489, 1243, 1119, 1187, 890 cm−1. 1H NMR (500 MHz, CDCl3): δ = 8.12 (d, J = 8.5 Hz, 2H), 7.84 (d, J = 8.0 Hz, 2H), 7.45 (d, J = 8.0 Hz, 2H), 7.36 (d, J = 8.5 Hz, 2H), 7.32 (bd, J = 3.0 Hz, 1H), 7.24–7.18 (m, 3H), 6.97–6.89 (m, 2H), 5.49 (d, J = 3.0 Hz, 1H), 4.43 (d, J = 13.5 Hz, 1H), 4.05 (d, J = 13.5 Hz, 1H), 2.50 (s, 3H). 13C NMR (101 MHz, CDCl3): δ = 162.8, 148.1, 145.9, 143.6, 139.8, 136.6, 135.3, 132.7, 131.4, 130.2, 129.2, 128.9, 128.4, 128.0, 123.8, 115.8, 92.7, 70.7, 57.2, 53.6, 21.7. HRMS (ESI): m/z calcd for C27H21I2N2O7S (M + Na)+: 770.9159; found: 770.9160.
(2E,8Z)-2-(Iodo(phenyl)methylene)-8-(iodomethylene)-9-(naphthalen-1-yl)-4-tosyl-1,6-dioxa-4-azaspiro[4.4]nonan-7-one (2i)
2i was obtained in 74% yield as a pale-yellow thick liquid; Rf = 0.54 (4
:
1 hexane/EtOAc); [Silica, UV and I2]. IR (Neat)νmax 2935, 1796, 1623, 1448, 1233, 1032, 868 cm−1. 1H NMR (500 MHz, CDCl3): δ = 8.49 (d, J = 8.5 Hz, 1H), 7.97 (d, J = 8.1 Hz, 1H), 7.93–7.90 (m, 3H), 7.74 (t, J = 8.0 Hz, 1H), 7.62 (t, J = 7.5 Hz, 1H), 7.51–7.47 (m, 2H), 7.45–7.40 (m, 2H), 7.23 (d, J = 7.2 Hz, 1H), 7.17–7.08 (m, 3H), 6.63 (d, J = 7.5 Hz, 2H), 6.31 (d, J = 2.5 Hz, 1H), 4.35 (d, J = 14.0 Hz, 1H), 4.09 (d, J = 13.5 Hz, 1H), 2.52 (s, 3H). 13C NMR (126 MHz, CDCl3): δ = 163.6, 145.7, 144.1, 136.7, 136.4, 133.8, 133.0, 130.6, 130.1, 129.3, 129.2, 129.10, 129.08, 129.0, 128.2, 127.9, 127.7, 127.5, 126.4, 125.1, 122.8, 116.7, 92.9, 70.3, 53.6, 53.1, 21.7. HRMS (ESI): m/z calcd for C31H24I2NO5S (M + H)+: 775.9465; found: 775.9465.
(2E,8Z)-2-((4-Chlorophenyl) iodomethylene)-8-(iodomethylene)-9-phenyl-4-tosyl-1,6-dioxa-4-azaspiro[4.4]nonan-7-one (3a)
3a was obtained in 75% yield as a pale-yellow thick liquid; Rf = 0.60 (4
:
1 hexane/EtOAc); [Silica, UV and I2]. IR (Neat)νmax 2919, 1775, 1558, 1448, 1362, 1238, 1089, 809, 744 cm−1. 1H NMR (500 MHz, CDCl3): δ = 7.84 (d, J = 8.5 Hz, 2H), 7.44 (d, J = 8.0 Hz, 2H), 7.41–7.37 (m, 3H), 7.32 (d, J = 2.5 Hz, 1H), 7.26–7.23 (m, 2H), 7.17–7.13 (m, 2H), 6.87 (d, J = 9.0 Hz, 2H), 5.41 (d, J = 3.0 Hz, 1H), 4.40 (d, J = 14.0 Hz, 1H), 4.06 (d, J = 14 Hz, 1H), 2.49 (s, 3H). 13C NMR (101 MHz, CDCl3): δ = 163.3, 145.7, 144.8, 135.8, 135.2, 133.8, 132.9, 132.6, 130.8, 130.5, 130.1, 129.0, 128.9, 128.1, 116.7, 92.2, 68.4, 57.5, 53.9, 21.8. HRMS (ESI): m/z calcd for C27H20ClI2NNaO5S (M + Na)+: 781.8738, found: 781.8738.
(2E,8Z)-2-((4-Bromophenyl) iodomethylene)-8-(iodomethylene)-9-phenyl-4-tosyl-1,6-dioxa-4-azaspiro[4.4]nonan-7-one (3b)
3b was obtained in 78% yield as a pale-yellow thick liquid; Rf = 0.60 (4
:
1 hexane/EtOAc); [Silica, UV and I2]. IR (Neat)νmax 2929, 1768, 1597, 1478, 1448, 1362, 1089, 887, 736, 680 cm−1. 1H NMR (500 MHz, CDCl3): δ = 7.86 (d, J = 8.0 Hz, 2H), 7.45 (d, J = 8.0 Hz, 2H), 7.44–7.39 (m, 3H), 7.36–7.30 (m, 3H), 7.29–7.24 (m, 2H), 6.83 (d, J = 8.5 Hz, 2H), 5.43 (d, J = 2.5 Hz, 1H), 4.41 (d, J = 14.0 Hz, 1H), 4.08 (d, J = 14.0 Hz, 1H), 2.51 (s, 3H). 13C NMR (101 MHz, CDCl3): δ = 163.3, 145.7, 144.8, 135.9, 135.7, 130.0, 132.6, 131.1, 131.0, 130.4, 130.0, 129.2, 129.1, 128.9, 128.9, 122.0, 116.7, 92.0, 68.4, 57.5, 53.9, 21.7. HRMS (ESI): m/z calcd for C27H20BrI2NNaO5S (M + Na)+: 825.8233; found 825.8238.
(2E,8Z)-2-(Iodo(phenyl)methylene)-8-(iodomethylene)-9-(m-tolyl)-4-tosyl-1,6-dioxa-4-azaspiro[4.4]nonan-7-one (3c)
3c was obtained in 67% yield as a pale brown semi solid; Rf = 0.52 (4
:
1 hexane/EtOAc); [Silica, UV and I2]. IR (Neat)νmax 2985, 1753, 1382, 1245, 1139, 1090, 1053, 910, 719 cm−1. 1H NMR (500 MHz, CDCl3) δ = 7.87 (d, J = 8.3 Hz, 2H), 7.46 (d, J = 8.0 Hz, 2H), 7.45–7.37 (m, 3H), 7.30–7.25 (m, 3H), 7.11 (t, J = 7.6 Hz, 1H), 7.03 (d, J = 7.6 Hz, 1H), 6.91 (s, 1H), 6.77 (d, J = 7.7 Hz, 1H), 5.43 (d, J = 3.0 Hz, 1H), 4.41 (d, J = 13.8 Hz, 1H), 4.11 (d, J = 13.8 Hz, 1H), 2.51 (s, 3H), 2.33 (s, 3H).13C NMR (126 MHz, CDCl3): δ = 163.4, 145.6, 144.0, 137.5, 136.8, 136.2, 133.2, 132.4, 130.6, 130.0, 130.0, 129.0, 128.9, 128.9, 128.8, 127.7, 126.7, 116.7, 91.3, 70.6, 57.5, 54.0, 21.7, 21.3. HRMS (ESI): m/z calcd for C28H24I2NO5S (M + H)+: 739.9465; found: 739.9458.
(2E,8Z)-2-(Iodo(3-nitrophenyl)methylene)-8-(iodomethylene)-9-phenyl-4-tosyl-1,6-dioxa-4-azaspiro[4.4]nonan-7-one (3d)
3d was obtained in 72% yield as a pale-yellow gummy liquid; Rf = 0.48 (4
:
1 hexane/EtOAc); [Silica, UV and I2]. IR (Neat)νmax 3059, 2971, 2227, 1779, 1597, 1370, 1169, 1122, 760 cm−1. 1H NMR (500 MHz, CDCl3): δ = 8.06 (dt, J = 7.5 Hz, 1H), 7.99 (s, 1H), 7.84 (d, J = 8.5 Hz, 2H), 7.47–7.37 (m, 6H), 7.34 (d, J = 3 Hz, 1H), 7.32–7.26 (m, 3H), 5.42 (d, J = 2.5 Hz, 1H), 4.42 (d, J = 14.0 Hz, 1H), 4.13 (d, J = 14.0 Hz, 1H), 2.49 (s, 3H). 13C NMR (126 MHz, CDCl3): δ 163.2, 147.9, 146.5, 145.8, 138.4, 135.63, 135.55, 133.0, 132.0, 130.4, 130.1, 129.1, 128.97, 128.90, 124.1, 122.9, 117.0, 92.1, 66.5, 57.5, 54.2, 21.7. HRMS (ESI): m/z calcd for C27H20I2N2O7S (M + Na)+: 792.8978, found: 792.8970.
(2E,8Z)-2-(Iodo(thiophen-2-yl)methylene)-8-(iodomethylene)-9-phenyl-4-tosyl-1,6-dioxa-4-azaspiro[4.4]nonan-7-one (3e)
3e was obtained in 69% yield as a pale-yellow gummy liquid; Rf = 0.48 (4
:
1 hexane/EtOAc); [Silica, UV and I2]. IR (Neat)νmax 2227, 1722, 1586, 1460, 1228, 1168, 1075, 755 cm−1. 1H NMR (500 MHz, CDCl3): δ = 7.84 (d, J = 8.5 Hz, 2H), 7.30–7.24 (m, 7H), 7.18–7.11 (m, 2H), 7.00 (d, J = 3.5 Hz, 1H), 6.94 (t, J = 5.0 Hz, 1H), 5.06 (d, J = 18 Hz, 1H), 4.69 (dd, J = 17.5, 1.5 Hz, 1H), 4.57 (s, 1H), 2.42 (s, 3H). 13C NMR (101 MHz, CDCl3): δ = 166.8, 145.3, 141.0, 136.6, 134.7, 134.1, 129.4, 129.3, 129.2, 128.7, 128.4, 128.2, 127.4, 126.7, 126.5, 88.1, 58.2, 58.0, 21.7. HRMS (ESI): m/z calcd for C25H20I2NO5S2 (M + H)+: 731.8872, found 731.8879.
(2E,8Z)-8-(Iodomethylene)-2-(1-iodopropylidene)-9-phenyl-4-tosyl-1,6-dioxa-4-azaspiro[4.4]nonan-7-one (3f)
3f was obtained in 76% yield as a pale brown semi solid; Rf = 0.57 (4
:
1 hexane/EtOAc); [Silica, UV and I2]. IR (Neat)νmax 2971, 2227, 1792, 1630, 1453, 1229, 1163, 1091, 808 cm−1. 1H NMR (400 MHz, CDCl3): δ = 7.82 (d, J = 8.4 Hz, 2H), 7.41 (d, J = 8.0 Hz, 1H), 7.39–7.33 (m, 5H), 7.27 (d, J = 3.0 Hz, 1H), 5.36 (d, J = 3.2 Hz, 1H), 4.15 (d, J = 16.5 Hz, 1H), 3.84 (d, J = 16.5 Hz, 1H), 2.48 (s, 3H), 2.25–2.06 (m, 2H), 0.75 (t, J = 7.6 Hz, 3H). 13C NMR (101 MHz, CDCl3): δ 163.7, 145.4, 142.7, 136.5, 133.1, 132.2, 130.6, 129.9, 128.88, 128.78, 128.75, 116.0, 91.0, 77.9, 57.9, 52.5, 28.7, 21.7, 13.9. HRMS (ESI): m/z calcd for C23H21I2NO5S (M + H)+: 677.9308; found 677.9310.
(2E,8Z)-2,8-Bis(iodomethylene)-9-phenyl-4-tosyl-1,6-dioxa-4-azaspiro[4.4]nonan-7-one (3g)
3g was obtained in 81% yield as a pale brown gummy liquid; Rf = 0.43 (4
:
1 hexane/EtOAc); [Silica, UV and I2]. IR (Neat)νmax, 2925, 2232, 1778, 1556, 1347, 1285, 1170, 1089, 777 cm−1. 1H NMR (400 MHz, CDCl3): δ = 7.86 (d, J = 8.4 Hz, 1H), 7.46–7.27 (m, 7H), 7.19 (d, J = 2.68 Hz, 1H), 5.40 (d, J = 2.8 Hz, 1H), 5.26 (s, 1H), 4.19 (d, J = 16.5, 2.0 Hz, 1H), 3.90 (d, J = 13.5, 2.4 Hz, 1H), 2.51 (s, 3H).
13C NMR (101 MHz, CDCl3): δ = 163.4, 149.7, 145.6, 136.3, 133.1, 131.7, 130.7, 130.0, 128.9, 128.8, 116.2, 90.9, 57.6, 51.3, 47.5, 21.7. HRMS (ESI): m/z calcd for C21H17I2NNaO5S (M + Na)+: 671.8814; found 671.8808.
(2E,8Z)-2-(Iodo(phenyl)methylene)-8-(iodomethylene)-4 (methylsulfonyl)-9-phenyl-1,6-dioxa-4-azaspiro[4.4]nonan-7-one (3h)
3h was obtained in 62% yield as a pale-yellow gummy liquid; Rf = 0.38 (4
:
1 hexane/EtOAc); [Silica, UV and I2]. IR (Neat)νmax 2228, 1727, 1598, 1371, 1324, 1264, 1123, 887 cm−1. 1H NMR (400 MHz, CDCl3): δ = 7.41–7.36 (m, 3H), 7.28–7.24 (m, 4H), 7.22 (dd, J = 7.56, 1.44 Hz, 2H), 7.09–7.05 (m, 2H), 5.20 (d, J = 2.8 Hz, 1H), 4.40 (q, J = 27.6, 14.4 Hz, 2H), 3.23 (s, 3H). 13C NMR (101 MHz, CDCl3): δ = 163.3, 143.6, 136.7, 135.8, 130.6, 129.6, 128.9, 128.8, 128.3, 128.0, 116.4, 92.2, 70.8, 57.2, 54.4, 38.7. HRMS (ESI): m/z calcd for C21H17I2NO5S (M + Na)+: 671.8814; found: 671.8819.
(5R,E)-8-((Z)-4-Methoxybenzylidene)-2-((4-methoxyphenyl)(phenyl)methylene)-9-(m-tolyl)-4-tosyl-1,6-dioxa-4-azaspiro[4.4]nonan-7-one (5)
To a mixture of spirocycle 2e (100 mg, 0.14 mmol, 1.0 equiv.), p-methoxy phenyl boronic acid (4; 52 mg, 0.33 mmol, 3.0 equiv.), K2CO3 (93 mg, 0.40 mmol, 3.0 equiv.), (p-tol)3P (17 mg, 0.05 mmol, 0.4 equiv.) and Pd (OAc)2 (6.0 mg, 0.027 mmol, 0.2 equiv.) was added 1,4-dioxane
:
H2O (9
:
1, 0.1 M, 1.4 mL). The reaction mixture was stirred at 40 °C using a preheated heating block for 12 h. The reaction progress was periodically monitored by TLC. Upon complete consumption of 2e, the reaction mixture was diluted with 3 mL of ethyl acetate and filtered through a small pad of Celite. The solvent was concentrated and the crude reaction mixture was purified by column chromatography on silica to afford 5 in 58% yield as a pale-yellow gummy liquid; Rf = 0.2 (4
:
1 hexane/EtOAc); [Silica, UV and I2]. IR (Neat)νmax 2924, 1712, 1599, 1359, 1246, 1088, 905, 727 cm−1. 1H NMR (500 MHz, CDCl3) δ = 7.94 (d, J = 8.9 Hz, 2H), 7.80 (d, J = 8.4 Hz, 2H), 7.49–7.43 (m, 2H), 7.44–7.37 (m, 5H), 7.05 (t, J = 7.7 Hz, 1H), 6.98–6.89 (m, 3H), 6.92–6.85 (m, 2H), 6.87–6.80 (m, 2H), 6.74 (d, J = 3.0 Hz, 2H), 6.63 (d, J = 7.7 Hz, 1H), 5.78 (d, J = 2.8 Hz, 1H), 4.14 (d, J = 13.2 Hz, 1H), 4.07 (d, J = 13.3 Hz, 1H), 3.87 (s, 3H), 3.83 (s, 3H), 2.49 (s, 3H), 2.23 (s, 3H). 13C NMR (126 MHz, CDCl3) δ = 165.3, 161.8, 159.1, 145.5, 143.6, 141.8, 137.7, 137.5, 134.9, 134.1, 133.9, 131.5, 131.4, 131.1, 130.2, 130.1, 129.9, 129.8, 129.3, 129.0, 128.7, 127.9, 127.9, 127.8, 126.8, 126.5, 123.4, 116.9, 114.5, 114.1, 77.7, 77.4, 77.2, 56.0, 55.8, 55.6, 49.4, 30.1, 22.1, 21.8. HRMS (ESI): m/z calcd for C42H39NO7S (M + H)+: 700.2364; found: 700.2367.
Data availability
The ESI‡ contains method description, product characterization data, and NMR spectra.
Author contributions
All authors have given approval to the final version of the manuscript. A. K. S. and R. P. conceived the idea and R. P. and S. K. performed the experiments. The manuscript and the ESI‡ data writing, and execution were done by M. S. and S. K. Review, editing, and supervision were done by A. K. S.
Conflicts of interest
There are no conflicts to declare.
Acknowledgements
The research was supported by SERB-India (SCP-2022-727). We thank the University of Hyderabad (UoH-IOE; UPE-CAS, and PURSE-FIST) for facilities. S. K. thanks Kothari-PDF, and R. P. and M. S. thank UGC and CSIR India, respectively, for fellowships. We thank Manas P. Gogoi for discussions.
References
-
(a) K. Chiyoda, J. Shimokawa and T. Fukuyama, Angew. Chem., Int. Ed., 2012, 51, 2508 CrossRef PubMed;
(b) A. D. Borthwick, Chem. Rev., 2012, 112, 3641 CrossRef CAS PubMed;
(c) S. Edmondson, S. J. Danishefsky and R. Neal, J. Am. Chem. Soc., 1999, 121, 2147 CrossRef CAS;
(d) W. S. Johnson, D. J. Dumas and D. Berner, J. Am. Chem. Soc., 1982, 104, 3510–3511 CrossRef CAS;
(e) R. Rios, Chem. Soc. Rev., 2012, 41, 1060–1074 RSC.
- For references reporting on the biological activity of lactones/lactams, see:
(a) C. P. Mason, K. R. Edwards, R. E. Carlson, J. Pignatello, F. K. Gleason and J. M. Wood, Science, 1982, 215, 400 CrossRef CAS PubMed;
(b) J. Das, D. M. Floyd, S. D. Kimball, K. J. Duff, M. W. Lago, R. V. Moquin, V. G. Lee, J. Z. Gougoutas and C. T. Vu, J. Med. Chem., 1992, 35, 773 CrossRef CAS PubMed;
(c) J. J. Beck and S. C. Chou, J. Nat. Prod., 2007, 70, 891 CrossRef CAS PubMed;
(d) A. N. Pearce, E. W. Chia, M. V. Berridge, E. W. Maas, M. J. Page, V. L. Webb, J. L. Harper and B. R. Copp, J. Nat. Prod., 2007, 70, 111 CrossRef CAS PubMed;
(e) Y. Wang, S. V. Neil, J. A. Wos, K. A. Oppong, M. C. Laufersweiler, D. L. Soper, C. D. Ellis, M. W. Baize, A. N. Fancher, W. Lu, M. K. Suchanek, R. L. Wang, W. P. Schwecke, C. A. Cruze, M. Buchalova, M. Belkin, B. De and J. Demuth, Bioorg. Med. Chem., 2007, 15, 1311 CrossRef CAS PubMed.
-
(a) F. Lovering, J. Bikker and C. Humblet, J. Med. Chem., 2009, 52, 6752 CrossRef CAS PubMed;
(b) T. T. Talele, J. Med. Chem., 2020, 63, 13291 CrossRef CAS PubMed;
(c) Y. Zheng, C. M. Tice and S. B. Singh, Bioorg. Med. Chem. Lett., 2014, 24, 3673 CrossRef CAS PubMed;
(d) Y. J. Zheng and C. M. Tice, Expert Opin. Drug Discovery, 2016, 11, 831 CrossRef PubMed;
(e) K. W. Quasdorf and L. E. Overman, Nature, 2014, 516, 181 CrossRef CAS PubMed;
(f) A. Hager, N. Vrielink, D. Hager, J. Lefranc and D. Trauner, Nat. Prod. Rep., 2016, 33, 491 RSC.
- For recent reviews on ynamides, see:
(a) J. Luo, G. S. Chen, S. J. Chen, J. S. Yu, Z. D. Li and Y. L. Liu, ACS Catal., 2020, 10, 13978 CrossRef CAS;
(b) C. C. Lynch, A. Sripada and C. Wolf, Chem. Soc. Rev., 2020, 49, 8543 RSC;
(c) Y. B. Chen, P. C. Qian and L. W. Ye, Chem. Soc. Rev., 2020, 49, 8897 RSC;
(d) S. Shandilya, M. P. Gogoi, S. Dutta and A. K. Sahoo, Chem. Rec., 2021, 21, 4123 CrossRef CAS PubMed;
(e) F.-L. Hong and L.-W. Ye, Acc. Chem. Res., 2020, 53, 2003 CrossRef CAS PubMed;
(f) A. G. Dehse, R. Hayashi, Z. Lu, Y. Zhang and R. P. Hsung, Chem. Rev., 2010, 110, 5064 CrossRef PubMed;
(g) G. Evano, A. Coste and K. Jouvin, Angew. Chem., Int. Ed., 2010, 49, 2840 CrossRef CAS PubMed;
(h) X. N. Wang, H. S. Yeom, L. C. Fang, S. He, Z. X. Ma, B. L. Kedrowski and R. P. Hsung, Acc. Chem. Res., 2014, 47, 560 CrossRef CAS PubMed;
(i) B. Prabagar, N. Ghosh and A. K. Sahoo, Synlett, 2017, 28, 2539 CrossRef CAS;
(j) G. Evano, C. Theunissen and M. Lecomte, Aldrichimica Acta, 2015, 48, 59 CAS;
(k) F. Pan, C. Shu and L.-W. Ye, Org. Biomol. Chem., 2016, 14, 9456 RSC.
-
(a) J. Hur, J. Jang and J. Sim, Int. J. Mol. Sci., 2021, 22, 2769 CrossRef CAS PubMed;
(b) R. R. A. Kitson, A. Millemaggi and R. J. K. Taylor, Angew. Chem., Int. Ed., 2009, 48, 9426 CrossRef CAS PubMed;
(c) T. P. Montgomery, A. Hassan, B. Y. Park and M. J. Krische, J. Am. Chem. Soc., 2012, 134, 11100 CrossRef CAS PubMed;
(d) E. L. McInturff, J. Mowat, A. R. Waldeck and M. J. Krische, J. Am. Chem. Soc., 2013, 135, 17230 CrossRef CAS PubMed;
(e) D. A. Sandham, R. J. Taylor, J. S. Carey and A. Fassler, Tetrahedron Lett., 2000, 41, 10091 CrossRef CAS.
-
(a) N. Ghosh, S. Nayak and A. K. Sahoo, Chem. – Eur. J., 2013, 19, 9428 CrossRef CAS PubMed;
(b) S. Nayak, N. Ghosh and A. K. Sahoo, Org. Lett., 2014, 16, 2996 CrossRef CAS PubMed;
(c) S. Nayak, N. Ghosh, B. Prabagar and A. K. Sahoo, Org. Lett., 2015, 17, 5662 CrossRef CAS PubMed;
(d) B. Prabagar, S. Nayak, R. Prasad and A. K. Sahoo, Org. Lett., 2016, 18, 3066 CrossRef CAS PubMed;
(e) S. Nayak, B. Prabagar and A. K. Sahoo, Org. Biomol. Chem., 2016, 14, 803 RSC;
(f) S. Nayak, B. Prabagar, N. Ghosh, R. K. Mallick and A. K. Sahoo, Synthesis, 2017, 49, 4261 CrossRef CAS;
(g) R. K. Mallick, B. Prabagar and A. K. Sahoo, J. Org. Chem., 2017, 82, 10583 CrossRef CAS PubMed;
(h) R. Prasad, S. Kanikarapu, S. Dutta, V. Srinivas and A. K. Sahoo, New J. Chem., 2022, 46, 13981 RSC;
(i) S. Kanikarapu, M. P. Gogoi, S. Dutta and A. K. Sahoo, Org. Lett., 2022, 24, 8289 CrossRef CAS PubMed;
(j) W.-D. Rao, D. Susanti, B. J. Ayers and P. W. H. Chan, J. Am. Chem. Soc., 2015, 137, 6350 CrossRef CAS PubMed;
(k) X.-Y. Chen, D. P. Day, W. T. Teo and P. W. H. Chan, Org. Lett., 2016, 18, 5936 CrossRef CAS PubMed.
-
(a) N. V. Shymanska and J. G. Pierce, Angew. Chem., Int. Ed., 2014, 53, 5401 CrossRef CAS PubMed;
(b) W. B. Shen, Q. Sun, L. Li, X. Liu, B. Zhou, J. Z. Yan, X. Lu and L.W. Ye, Nat. Commun., 2017, 8, 1748 CrossRef PubMed;
(c) Z. Yang, Q. Zhong, S. Zheng, G. Wang and L. He, Molecules, 2019, 24, 936 CrossRef PubMed;
(d) X. Cai, W. Liang, M. Liu, X. Li and M. Dai, J. Am. Chem. Soc., 2020, 142, 13677 CrossRef CAS PubMed;
(e) R.-X. Liang, L.-J. Song, J.-B. Lu, W.-Y. Xu, C. Ding and Y.-X. Jia, Angew. Chem., Int. Ed., 2021, 60, 7412 CrossRef CAS PubMed;
(f) C. Yuan, A. Axelrod, M. Varela, L. Danysh and D. Siegel, Tetrahedron Lett., 2011, 52, 2540 CrossRef CAS.
- For selected examples involving N,O-enamide species formation, see:
(a) S. Nayak, B. Prabagar and A. K. Sahoo, Org. Biomol. Chem., 2016, 14, 803 RSC;
(b) N. Ghosh and S. Nayak, Chem. – Eur. J., 2013, 19, 9428 CrossRef CAS PubMed;
(c) S. Nayak, N. Ghosh and A. K. Sahoo, Org. Lett., 2014, 16, 2996 CrossRef CAS PubMed;
(d) S. Nayak, N. Ghosh, B. Prabagar and A. K. Sahoo, Org. Lett., 2015, 17, 5662 CrossRef CAS PubMed.
-
(a) A. S. K. Hashmi, T. Lauterbach, P. Nösel, M. H. Vilhelmsen, M. Rudolph and F. Rominger, Chem. – Eur. J., 2013, 19, 1058 CrossRef CAS PubMed;
(b) P. Nösel, T. Lauterbach, M. Rudolph, F. Rominger and A. S. K. Hashmi, Chem. – Eur. J., 2013, 19, 8634 CrossRef PubMed;
(c) M. M. Hansmann, M. Rudolph, F. Rominger and A. S. K. Hashmi, Angew. Chem., Int. Ed., 2013, 52, 2593 CrossRef CAS PubMed;
(d) I. Braun, A. M. Asiri and A. S. K. Hashmi, Gold Catalysis 2.0, ACS Catal., 2013, 3, 1902 CrossRef CAS;
(e) S. Ferrer and A. M. Echavarren, Organometallics, 2018, 37, 781 CrossRef CAS PubMed;
(f) A. G. Tathe, S. S. Saswade and N. T. Patil, Org. Chem. Front., 2023, 10, 2359–2384 RSC;
(g) V. W. Bhoyare, A. G. Tathe, A. Das, C. C. Chintawar and N. T. Patil, Chem. Soc. Rev., 2021, 50, 10422–10450 RSC.
- See the ESI.‡.
- Since stoichiometric water is used in the reaction, the participation of the di-vinylic-[Au] species obtained through activation of alkyne-moieties with the [Au]-catalyst is unlikely.
Footnotes |
† Dedicated to Prof. Sukh Dev on his 100th birthday for his outstanding scientific contributions to organic chemistry. |
‡ Electronic supplementary information (ESI) available: Experimental procedures, characterisation data for new compounds and crystallographic data. CCDC 2120442 (compound 2d). For ESI and crystallographic data in CIF or other electronic format see DOI: https://doi.org/10.1039/d4ob00551a |
§ These authors contributed equally. |
|
This journal is © The Royal Society of Chemistry 2024 |
Click here to see how this site uses Cookies. View our privacy policy here.