Rapid paper-based optical sensing of Spilosoma obliqua nucleopolyhedrovirus via ester hydrolysis†
Received
9th April 2024
, Accepted 30th June 2024
First published on 25th July 2024
Abstract
We have developed an easily scalable chromogenic probe for the dual-mode sensing of Spilosoma obliqua Nuclear polyhedrosis viruses (SpobNPV) in aqueous media. The mechanistic investigations establish that the imidazole-mediated hydrolysis of acyl ester linkage in which water (general base) acts as a nucleophile induces a pronounced change in the emission colour from blue to cyan. To the best of our knowledge, this is the first attempt at quantifying OBs of SpobNPV using a small molecule-based optical probe with a detection limit of 2.305 × 103 OBs mL−1. The rate of ester hydrolysis was dependent on both substrate and OBs concentration. Due to the naked eye response, paper strips were also developed for the rapid and onsite detection of SpobNPV. The operation procedure is straightforward and does not involve additional sample preparation steps. This makes the present protocol suitable for daily use. Interestingly, the present protocol is also quite efficient in estimating SpobNPV, even in several agricultural crop samples (for at least 15 crops). Such findings will add a new dimension to better managing Spilosoma obliqua and minimizing the extent of crop loss.
Introduction
The economic growth of many countries mainly depends on their agriculture. Moreover, it employs nearly 50% of its population in many countries. However, every year, the loss of crops due to pest attacks has remained one of the significant challenges faced by the farming community of India.1 Among various insect pests, Spilosoma obliqua (Walker) is a sporadic and polyphagous insect pest widely distributed throughout India. It is reported to feed on 126 species of plants belonging to 25 families, including pulses, oilseeds, cereals, vegetables, mulberry, turmeric, and medicinal and aromatic plants and causes heavy economic loss.2,3 The caterpillars feed gregariously during the early larval stages and solitarily in the late larval stages. However, chemical pesticides are no longer considered sustainable since several agrochemicals, when used continuously and indiscriminately, have developed resistance and caused severe environmental problems.4,5 Consumer concern about the chemical pesticide residues on food further drives the search for an alternative pest control method.
New research suggests that bio-control technology using insect viruses could be an effective and environmentally friendly alternative for managing harmful pests. Spilosoma obliqua nucleopolyhedrovirus is one of the microbial pesticides known to be effective against Spilosoma obliqua. The main advantage of using this biopesticide over chemical pesticides is that it is inherently non-toxic and can produce minimal environmental pollution. Unlike chemical pesticides, nucleopolyhedroviruses (NPVs) are highly host-specific and non-pathogenic to other beneficial insects and non-target organisms such as humans, domestic animals, fishes, etc. Moreover, these biopesticides show no long-term effect on soil or water.6–8 As the occlusion bodies (OBs) are the actual structural units for larval infection, the Environmental Protection Agency (EPA) has registered the OBs of viruses as the pesticide active ingredient. Quantifying OBs by counting is one of the reliable procedures for measuring the potency of a virus suspension without knowing the concentration of the exact virus particle.9–12
For bio-molecular analysis (involving a live host), knowing the actual concentration of OBs present in the aqueous suspension is essential. The presence of any insect pathogen other than NPV in the production batches can cause a reduction in virus productivity. Besides avoiding the production of unintended insect pathogens, it is also necessary to prevent the contamination of the final product, which may pose a hazard to production staff or end-users.12,13 Additionally, the large-scale production of viruses also faces several other unavoidable shortcomings, which can eventually bring down its quality. For example, the quality of virus production depends on the procedure followed during their culture, the nature of the water used during their preparation, etc.13–16 Thus, along with the bioassay, which can confirm that the virus is infective or not, it is also essential to check whether the desired virus is being produced in sufficient amount or not. Therefore, quality control for NPVs includes routine examinations of insect stocks, monitoring colony performance, microscopic studies of insects, routine counting bioassays, and so on.
Though there have been several reports in the literature on the occurrence and potential importance of viruses, there still needs to be more known about the identification and morphological characterization of these viruses for their better understanding and exploitation in the present-day plant protection strategy. Presently, counting virus particles (OBs) can be achieved only by using a hemocytometer with improved Neubauer.17 Even then, the light microscopy-based method has several disadvantages. Significantly, the use of ‘poor’-quality microscopes with non-phase optics can add significant errors in counting. The virus suspension should be pure or at least semi-pure for the microscopic analysis to avoid confusion with other polyhedral-like particles (for example, dust or tiny oil droplets). Moreover, as the size and shape of the OBs can be different even among the same species, it is often challenging to differentiate them from other polyhedral-like particles. At the same time, virus suspension should not have any clumping and aggregation of OBs; otherwise, manually counting the actual number becomes difficult. Again, special care must be taken to ensure that the microscopy instrument and its associated accessories, such as coverslips, slides, etc., are free from dust or dirt particles. Therefore, the maintenance of all these facilities needs an uninterrupted source of electricity, specially trained technicians, and a high-quality wet lab facility for sample analysis, etc. However, despite the abovementioned limitations, the quality control of NPV is virtually impossible without involving a light microscope. On the other hand, the amount of these biopesticides that need to be sprayed over a particular area in the cultivation field will depend on the concentration of OBs present in the supplied formulations. Importantly, the concentration of OBs in the tentative mixture may decrease with time due to the attack of other unintended insect pathogens or over-exposure to the hot and humid environment and sunlight. Thus, regularly monitoring NPV levels in the tentative formulations is essential to use them almost daily. Therefore, there is a need to design new optical methods for the rapid and cost-effective quantification of SpobNPV in formulations.
Herein, we have developed a new optical method for the low-cost and rapid quantification of (SpobNPV) in the formulations using probe 1 (Fig. 1a). On interaction with SpobNPV, the probe changes its color from colorless to greenish-yellow. To the best of our knowledge, this is the first attempt at quantifying OBs of SpobNPV using a small molecule-based optical probe. We have also developed low-cost, easily portable paper strips to estimate SpobNPV in the formulations. Moreover, the present findings could be successfully utilized to detect SpobNPV in the extract of leaves of 15 different crop samples.
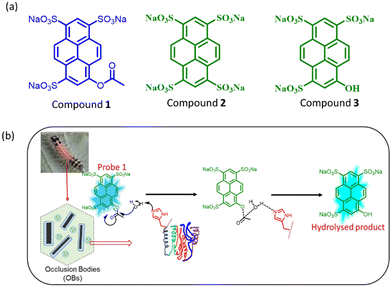 |
| Fig. 1 (a) The molecular structure of the compounds involved under investigation. (b) Diagrammatic illustration for the hydrolysis of probe 1 in the presence of SpobNPV. | |
Results and discussion
Interaction of compound 1 with SpobNPV
Herein, we have developed a small molecule-based optical probe 1 (Fig. 1a) for the dual-mode sensing of Spilosoma oblique nucleopolyhedrovirus in water. Without virus particles, the compound remains colorless in water with strong blue emission (λmax = 410 nm). The addition of SpobNPV (from a stock of 109 OBs mL−1) into the aqueous solution of compound 1 rendered a change in the visible color from colorless to greenish-yellow upon 2 h incubation time at room temperature (Fig. 2a and b). On the other hand, an aqueous solution of 1, when treated with SpobNPV (∼2 h), showed a change in the emission color from blue to cyan under UV light (Fig. 2d). As expected, the addition of SpobNPV resulted in the quenching of emission intensities of 1 at 390 nm and 410 nm bands with the formation of a new fluorescence band at 510 nm (Fig. 2c). These characteristic changes in the absorption and emission signals suggested the formation of a new compound in the reaction mixture via the hydrolysis of the parent compound (Fig. 1b). In the case of 1, the quantification of the product formed during the enzymatic hydrolysis could be achieved using both UV-visible spectrophotometry (absorption maxima at both 418 and 483 nm) and a conventional luminometry (λmax = 510 nm).
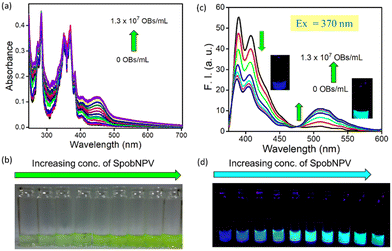 |
| Fig. 2 (a) UV-vis titration of compound 1 (10 μM) with SpobNPV (0–1.3 × 107 OBs mL−1) in water. (b) Change in color of the aqueous solution of 1 (10 μM) upon gradually adding SpobNPV in water. (c) Fluorescence spectral titration of compound 1 (10 μM, λex = 370 nm) with SpobNPV (0–1.3 × 107 OBs mL−1) in water. (d) Change in the color of the aqueous solution of 1 (10 μM) upon gradual addition of a suspension of SpobNPV in water under a UV lamp (>365 nm). | |
Kinetic studies for the interaction of 1 with SpobNPV
An incubation time of 2 h was required because a decrease in the reaction rate was observed beyond this time, probably due to the accumulation of the hydrolyzed product. Further, the time course studies under a pseudo-first-order condition for the ester hydrolysis reaction with respect to 1 suggested a common trait for other microbe-catalyzed ester hydrolysis reactions.18 The hydrolysis extent was dependent on the concentration of both 1 (in μM, Fig. 3a, Table S1a†) and SpobNPV (in OBs mL−1, Fig. S1 and Table S1b†) present in the aqueous extract. Moreover, the extent of hydrolysis (change in the visible color and emission intensity) was found to be increased linearly with the concentration of SpobNPV (0.5–2.0 × 107 OBs mL−1, Fig. S2a† and Fig. 5c). This is indeed an intriguing observation since it will help to quantify SpobNPV in unknown analytical samples.
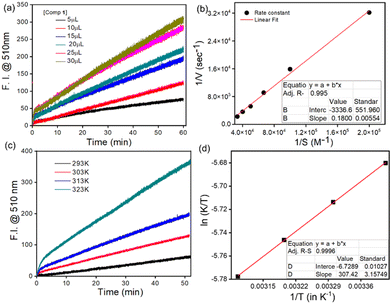 |
| Fig. 3 (a) Effect of the substrate concentration (5–30 μM, λex = 370 nm) on the extent of SpobNPV (1.3 × 107 OBs mL−1) induced emission change at 510 nm. (b) Lineweaver–Burk plot for SpobNPV catalysed hydrolysis of 1 at different substrate concentrations and a fixed concentration of SpobNPV (1.3 × 107 OBs mL−1). (c) Effect of temperature on the extent of change in the emission spectra of 1 (10 μM, λex = 370 nm) induced by SpobNPV at 510 nm. (d) The Eyring–Polanyi plot determines the SpobNPV-mediated ester hydrolysis thermodynamic parameters. | |
From the concentration variation studies, the limit of quantification (LOQ) for SpobNPV was estimated at 2.305 × 103 OBs mL−1.19,20 Further, the quantification of dye released during the enzymatic decomposition was achieved by considering the extinction coefficient of the dye at both 418 and 483 nm absorption spectral bands. An increase in the rate of hydrolysis was observed up to a 30 μM concentration of 1 (Fig. 3a). Here also, at low substrate concentration (<1 μM), the hydrolytic activity was found to be limited by the amount of substrate available. However, a slight deviation from linearity was observed when a significantly large amount of virus (>3.0 × 107 POBs mL−1) was employed, probably due to the large substrate concentration. The substrate concentration vs. activity curves for the ester hydrolysis of 1 was found to follow the Michaelis–Menten equation. Further, the Michaelis constant (Km) and maximum reaction rate (Vmax) were calculated from the Lineweaver–Burk plot (Fig. 3b).21 A Km value of 5.39 × 10−5 M obtained in this case essentially indicated the ‘OBs’ of SpobNPV were quite effective in catalyzing ester compounds even in pure aqueous media.
Effect of temperature and pH of the media on ester hydrolysis
Like any other enzymatic reaction, hydrolysis of 1 was also influenced by the variation in temperature and pH of the medium.22 Thus, we investigated the interaction kinetics at different pH levels, keeping the temperature fixed at 313 K and temperature conditions with a pH fixed at 6.0. An enhancement in the rate of hydrolysis was observed upon increasing the reaction temperature from 298 to 323 K (Fig. 3c, Table S2a†). However, beyond 340 K, a decrease in the rate of hydrolysis was observed, which might be due to thermal denaturation of the outer protein layer of SpobNPV above 323 K.
Further, the activation energy for hydrolysis was found to be 12.3 kcal mol−1 using the Arrhenius equation (Fig. S3a†). In addition, changes in the thermodynamic parameters were also calculated using the Eyring Polanyi equation (Fig. 3d).23 A negative value of ΔS≠ indicates the facile formation of the enzyme–substrate complex when an enzyme binds with the substrate. The release of translational and rotational energies during reactant consumption is mainly responsible for the loss in entropy, a widespread phenomenon during enzymatic hydrolysis.24
The effect of pH on the hydrolysis of 1 was also significant because the H+ ion concentration in the reaction media could influence the ionisation of different functional groups present on the protein surface of OBs and can further alter the substrate's ionisation state. As expected, an increase in the rate of hydrolysis was noticed upon changing the pH of the medium from 6 to 9 (Fig. S2b and Table S2b†). However, no hydrolysis could be discerned below pH 5.0. Under these conditions, the solubility of OBs was suspected to be relatively low, resulting in a lower effective concentration of the catalyst in the reaction medium. On the contrary, the method was also ineffective at high pH conditions (pH > 9.5) because, at alkaline pH, the acetate ester compound was spontaneously degraded hydrolytically even without an enzyme.
Evidence for SpobNPV mediated hydrolysis
We performed a series of spectral investigations to prove the virus particles mediated ester hydrolysis reaction. In the first case, we took compound 2, which is devoid of an ester bond. Fig. 4a suggests that no change in the emission spectra of compound 2 was observed upon the addition of SpobNPV. Further, the ESI-MS mass spectrum of 1 was recorded in the presence and absence of SpobNPV in water. In the presence of SpobNPV, an additional peak could be detected due to the formation of the hydrolyzed product. Again, the FT-IR spectrum of 1 also showed the disappearance of a stretching band due to the ester functional group upon treatment with SpobNPV (Fig. S3b†). This again suggested a virus-mediated diacylation reaction of 1 in the aqueous medium.
 |
| Fig. 4 (a) Comparison plot for the SpobNPV-mediated ester hydrolysis by different substrates. (b) Comparison emission spectra of 1 in the presence of SpobNPV with the commercially available hydrolyzed product (10 μM, λex = 370 nm). (c) Comparative interaction of 1 with SpobNPV and lipase in water. (d) Emission spectra of 1 (10 μM) in the presence of SpobNPV (1.3 × 107 OBs mL−1) in water and D2O. | |
Finally, the absorption and emission spectra of the [1 + SpobNPV] physical mixture were compared with the commercially available hydrolyzed compound (3). A similar spectral signature was observed in both cases, which unambiguously demonstrates that the ester hydrolysis reaction occurred in the presence of SpobNPV OBs (Fig. 4b and S4a†). Moreover, a similar change in the optical signal was observed when, instead of SpobNPV, substrate 1 was incubated with an esterase enzyme (lipase) at pH 7.4 (Fig. 4c). This indicated that the effect of SpobNPV OBs on 1 resembled the activity of the esterase enzyme. Moreover, the UV-visible spectrum of 1 in the presence of SpobNPV showed a linear absorbance increase at the 454 nm (Fig. 5c) band, which might be responsible for the greenish-yellow coloration. This also suggests that one can correlate the change in the absorbance directly with the concentration of SpobNPV present. Further, the kinetics of ester hydrolysis were also monitored in both the D2O (pD = 6.4) and H2O (pH = 6.0) media (Fig. 4d). It was observed that the rate of ester hydrolysis was significantly low in D2O (∼2.97 fold) compared to that of H2O. Thus, the proton transfer is the rate-determining step of the hydrolysis reaction. As a control, non-catalytic proteins such as HSA did not generate any spectral signal even after a long time (∼2 h) under identical reaction conditions (Fig. 5a).
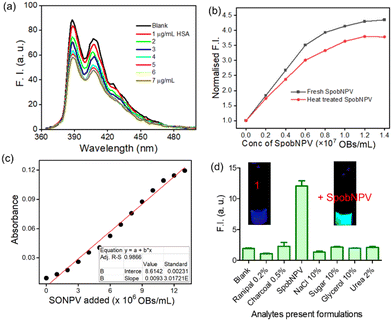 |
| Fig. 5 (a) Fluorescence titration of 1 (10 μM, λex = 370 nm) in the presence of HSA (0–7 μg mL−1) in water. (b) Change in the emission spectra of 1 (10 μM, λex = 454 nm) in the presence of fresh and heat-treated SpobNPV (0–1.3 × 107 OBs mL−1) in water. (c) Change in the absorbance of compound 1 (10 μM) at 454 nm upon adding SpobNPV (0–1.3 × 107 OBs mL−1) in water. (d) Change in the emission intensity of 1 (10 μM, λex = 370 nm) at 510 nm in the presence of different analytes present in commercial formulations. | |
We were interested in investigating whether the ester hydrolysis process in an aqueous medium was promoted by the negatively charged surface of the polyhedral protein or the self-assembled functional groups present on its surface. For this, we incubated the SpobNPV solution at 95 °C for 15 min to trigger heat-induced denaturation of the outer protein core. The heat-treated SpobNPV solution exhibited a significantly different circular dichroism spectrum and emission signal when compared to its counterpart, which was not heat-denatured. The addition of this heat-treated SpobNPV to the aqueous solution of 1 showed only a minor change in the emission signal (Fig. 5b). This observation indicates that the presence of 3D networks of functional groups, such as imidazole, aspartic acids, etc., on the protein surface of OBs does catalyze the ester hydrolysis reaction. To confirm the role of amino acids in hydrolysis, we recorded the emission spectra of the compound in the presence of three different amino acids, namely histidine, cysteine, and serine, under the same experimental condition. We found that only histidine showed the formation of a new band at 510 nm, but not with cysteine or serine. Thus, it may be concluded that the histidine residues present on the surface of the occlusion bodies are involved as catalysts in the ester hydrolysis reaction (Fig. S4b†).
It is essential to check the amount of SpobNPV present in the commercial formulation with its reported value (labelled as OBs mL−1 on the bottle) for quality control purposes. Moreover, the amount of biopesticide required to be sprayed (on a one-acre agricultural field) also depends on its concentration. Thus, estimating OBs in these formulations is essential for farmers to avoid unintended crop loss. For this, it is vital to investigate the cross-reactivity of 1 towards the other additives commonly added in the commercial formulations. Importantly, no changes in the emission (at 510 nm, Fig. 5d) signal of 1 were observed in the presence of these analytes added individually or with their mixtures (without SpobNPV). This ensures that the extent of change in the spectral signal of 1 upon treatment with commercial formulation may be directly correlated with the amount of SpobNPV in the mixture.
Design of portable paper-strips for SpobNPV
The addition of SpobNPV suspension in water onto the precoated (sprayed with compound 1) paper discs resulted in the appearance of bright greenish-yellow color (under normal daylight) with a change in the emission color from blue to cyan within 60 min (under UV lamp). The intensity of bright greenish yellow or cyan emission at any given time (Fig. 6a) was also found to be dependent on the concentration of SpobNPV present in the aqueous suspension (103–109 OBs mL−1). Further, the relative change in the luminescence (or the intensity of greenish-yellow color) could be quantified by using a readily available (downloaded free from the Internet) image processing software, ImageJ (Fig. 6b).25 The minimum detectable concentration of SpobNPV was achieved up to 103 OBs mL−1 by this technique. Further, to prove the specificity of the probe molecule towards SpobNPV, control experiments were performed by applying other components of the mixture (charcoal, sugar, NaCl, glycerol, and Ranipal®) onto dye-coated filter paper discs. No significant change in the color or fluorescence emission of the strips was observed upon incorporating any of these analytes.
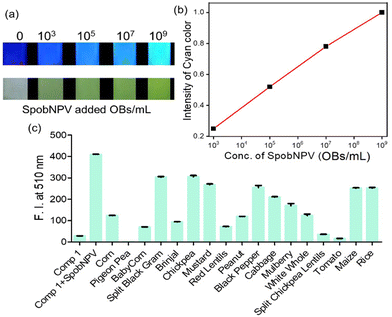 |
| Fig. 6 (a) Changes in the color of the 1-coated paper strips (50 μM, λex = 370 nm) in the presence of different concentrations of SpobNPV (seen under UV light). (b) Change in the emission color and visible color of 1 (50 μM) in the presence of different concentrations of SpobNPV. (c) Change in the emission intensity of 1 (10 μM, λex = 370 nm) at 510 nm in the presence of SpobNPV (1.3 × 107 OBs mL−1) in different crop extracts. | |
SpobNPV Detection in Different Crop Extracts
OBs protect the SpobNPV from the outside environment and keep their viability intact for over 30 years. When a larva dies due to SpobNPV infection, OBs emerge from the larval body and get deposited on the leaf. These OBs can remain infectious until another insect arrives to eat the leaf and ingest the protected virions. Thus, if one can measure the residual concentration of OBs on the vegetable leaves, predicting the interval between two successive spattering of SpobNPV in the same field will be easier. However, for this to be useful, the sensor system mustn't suffer from interference from other components present in the aqueous extracts of these vegetables. To mimic the actual scenario, here we have chosen 18 different types of crops known to be infected by Spilosoma obliqua. Thus, for these crops, using SpobNPV as an insecticide is a common practice for farmers. We first recorded the emission spectra of 1 in diluted aqueous extracts of these crops to rule out any background influence. Then, we monitored the emission signal changes upon adding SpobNPV. In all the cases, changes in the emission signal were recorded only after 2 h of the time interval. Out of these 18 different crop samples (Fig. 6c), the protocol was found to be successful in at least 15 cases (except for pigeon peas, tomatoes, and split chickpea lentils). Thus, the present protocol is quite efficient in estimating SpobNPV, even in the agricultural crop samples.
Conclusions
In conclusion, we have utilized an easily scalable chromogenic probe for the selective ‘naked-eye’ detection (color changes from colorless to greenish-yellow) of SpobNPV suspension in a 100% aqueous medium. For optimal response, probe molecule 1 must be incubated with SpobNPV suspension for ∼2 h at room temperature in water. The mechanistic investigations established that the OBs of SpobNPV can catalyse the hydrolysis of acyl ester linkage and induce a pronounced change in the optical signal. The probe showed a linear change in the absorbance at 454 nm over a wide concentration range of OBs (0–1.3 × 107 POBs mL−1). This indicates that the present method can be useful for quantitatively estimating SpobNPV over a wide range of concentrations in unknown samples. Under a long UV lamp, the probe showed a ratiometric change in the emission color from blue to cyan upon adding SpobNPV. This ratiometric sensing essentially reduces the influence of instrumental parameters and surroundings on the measurement, estimating SpobNPV quantitatively. Easily portable color strips could also be developed for rapid, on-field estimation of SpobNPV whenever required. In addition to this, the protocol was found to be successful in at least 15 cases out of 18 tested crops. We believe such findings will add a new dimension to better managing Spilosoma obliqua and minimize crop loss.
Author contributions
S. B. conceptualised the project, Dipen Biswakarma and ND did data collection and analysis, performing all the systematic studies, and designing experiments to prove the mechanism. Dipen Biswakarma first prepared the original manuscript draft, and Dipen Biswakarma, ND, and SB reviewed and edited it. SB provided the infrastructure and funding for the research. Dr Deepa Bhagat was involved in identifying problems and providing cultures of Spilosoma obliqua nucleopolyhedrovirus. Dr G. Sivakumar provided Spilosoma obliqua nucleopolyhedrovirus seed culture and laboratory facilities.
Data availability
The authors Dipen Biswakarma, Nilanjan Dey, G. Sivakumar, Deepa Bhagat, and Santanu Bhattacharya will provide the data on request.
Conflicts of interest
There are no conflicts to declare.
Acknowledgements
This work was supported by the J. C. Bose Fellowship, Department of Science and Technology (DST) to Prof. S. Bhattacharya. D. Biswakarma thanked UGC for the DSKPDF fellowship. D Bhagat thanks the Directors, ICAR-NBAIR, Bangalore for encouragement.
References
- A. K. Mishra, Agriculture and Rural Development in India Since 1947, Am. J. Agric. Econ., 2010, 92, 1256–1259 CrossRef
.
- G. Sivakumar, M. Kannan, V. R. Babu, M. Mohan, S. Kumari, R. Rangeshwaran, T. Venkatesan and C. R. Ballal, Characterization and Field Evaluation of Tetrahedral and Triangular Nucleopolyhedrovirus of Spilosoma Obliqua (SpobNPV) Strain NBAIR1 Against Jute Hairy Caterpillar, Egypt. J. Biol. Pest Control, 2020, 30, 1–7 CrossRef
.
- C. M. S. Kumar, K. Jeyaram and H. B. Singh, First Record of the Entomopathogenic Fungus Entomophaga Aulicae on the Bihar Hairy Caterpillar Spilarctia Obliqua in Manipur, India, Phytoparasitica, 2011, 39, 67–71 CrossRef
.
- D. Koushik, S. Sen Gupta, S. M. Maliyekkal and T. Pradeep, Rapid Dehalogenation of Pesticides and Organics at the Interface of Reduced Graphene Oxide-Silver Nanocomposite, J. Hazard. Mater., 2016, 308, 192–198 CrossRef CAS PubMed
.
- P. Nicolopoulou-Stamati, S. Maipas, C. Kotampasi, P. Stamatis and L. Hens, Chemical Pesticides and Human Health: The Urgent Need for a New Concept in Agriculture, Public Health Front., 2016, 4, 148 Search PubMed
.
- U. B. Singh, A. Sahu, N. Sahu, R. K. Singh, S. Renu, D. P. Singh, M. C. Manna, B. K. Sarma, H. B. Singh and K. P. Singh, Arthrobotrys Oligospora Mediated Biological Control of Diseases of Tomato (Lycopersicon esculentum Mill.) Caused by Meloidogyne Incognita and Rhizoctonia Solani, J. Appl. Microbiol., 2013, 114, 196–208 CrossRef CAS PubMed
.
- J. A. Essiedua, F. O. Adepojub and M. N. Ivantsova, Benefits and limitations in using biopesticides: A review, AIP Conf. Proc., 2020, 2313, 080002 CrossRef
.
- S. Arthurs and S. K. Dara, Microbial Biopesticides for Invertebrate Pests and Their Markets in the United States, J. Invertebr. Pathol., 2019, 165, 13–21 CrossRef PubMed
.
- C. D. Beaton and B. K. Filshie, Comparative Ultrastructural Studies of Insect Granulosis and Nuclear Polyhedrosis Viruses, J. Gen. Virol., 1976, 31, 151–161 CrossRef CAS PubMed
.
- G. F. Rohrmann, Polyhedrin structure, J. Gen. Virol., 1986, 67, 1499–1513 CrossRef CAS PubMed
.
- D. B. Sajjan and H. B. Hinchigeri, Structural Organization of Baculovirus Occlusion Bodies and Protective Role of Multilayered Polyhedron Envelope Protein, Food Environ. Virol., 2016, 8, 86–100 CrossRef CAS PubMed
.
- T. Martins, R. Montiel, J. Medeiros, L. Oliveira and N. Simões, Occurrence and characterization of a nucleopolyhedrovirus from Spodoptera littoralis (Lepidoptera: Noctuidae) isolated in the Azores, J. Invertebr. Pathol., 2005, 89, 185–192 CrossRef CAS PubMed
.
- C. S. Kumar, G. V. Ranga Rao, K. Sireesha and P. L. Kumar, Isolation and Characterization of Baculoviruses from Three Major Lepidopteran Pests in the Semi-Arid Tropics of India. Isolation and Characterization of Baculoviruses from Three Major Lepidopteran Pests in the Semi-arid Tropics of India, Indian J. Virol., 2011, 22, 29–36 CrossRef PubMed
.
- N. Dey, D. Bhagat and S. Bhattacharya, On-Field Detection of Helicoverpa Armigera Nuclear Polyhedrosis Virus Using Luminescent Amphiphilic Probe: Screening of Agricultural Crops and Commercial Formulations, ACS Sustainable Chem. Eng., 2019, 7, 7667–7675 CrossRef CAS
.
- G. Rosell, C. Quero, J. Coll and Guerrero, Biorational Insecticides in Pest Management, J. Pestic. Sci., 2008, 33, 103–121 CrossRef CAS
.
- E. B. Devi, E. P. Devi and I. Deepshikha, An Overview on Nuclear Polyhedrosis Virus (NPV) as a Valuable Biopesticide in Enhancing Ecofriendly Management of Insect Pests, Int. J. Curr. Res. Biosci. Plant Biol., 2016, 3, 97–106 CAS
.
- D. Biswakarma, N. Dey, D. Bhagat and S. Bhattacharya, Switchable Luminescent Probe for Trace-Level Detection of the Spodoptera litura Nuclear Polyhedrosis Virus via a Color-Changing Response, J. Agric. Sci. Technol., 2021, 1, 322–328 CAS
.
- H. Zhang, X. Xue, Q. Luo, Y. Li, K. Yang, X. Zhuang, Y. Jiang, J. Zhang, J. Liu, G. Zou and X. Liang, Self-Assembled Peptide Nanofibers Designed as Biological Enzymes for Catalyzing Ester Hydrolysis, ACS Nano, 2014, 8, 11715–11723 CrossRef PubMed
.
- E. Ballesteros, D. Moreno, T. Gomez, T. Rodriguez, J. Rojo, M. Garcia-Valverde and T. Torroba, Org. Lett., 2009, 11, 1269 CrossRef CAS PubMed
.
-
D. L. Massart, B. G. M. Vandeginste, L. M. C. Buydens, S. DeJong, P. J. Lewi and J. Smeyers-Verbeke, Handbook of Chemometrics andQualimetrics: Part A, Eselvier, Amsterdan, The Netherlands, 1997, ch. 13, p. 379 Search PubMed
.
-
(a) J. M. G. Rodriguez, N. P. Hux, S. J. Philips and M. H. Towns, Michaelis–Menten Graphs, Lineweaver–Burk Plots, and Reaction Schemes: Investigating Introductory Biochemistry Students’ Conceptions of Representations in Enzyme Kinetics, J. Chem. Educ., 2019, 96, 1833–1845 CrossRef CAS
;
(b) S. Bhattacharya and V. P. Kumar, Evidence of Enhanced Reactivity of DAAP Nucleophiles towards Dephosphorylation and Deacylation Reaction in Cationic Gemini Micellar Media, J. Org. Chem., 2004, 69(2), 559–562 CrossRef CAS PubMed
.
-
(a) S. Bhattacharya, K. Snehalatha and V. P. Kumar, Synthesis of New Cu(II)-Chelating Ligand Amphiphiles and Their Esterolytic Properties in Cationic Micelles, J. Org. chem., 2003, 68(7), 2741–2747 CrossRef CAS PubMed
;
(b) S. Bhattacharya, K. Snehalatha and S. K. George, Synthesis of Some Copper(II)-Chelating (Dialkylamino)pyridine Amphiphiles and Evaluation of Their Esterolytic Capacities in Cationic Micellar Media, J. Org. Chem., 2023, 63(1), 27–35 CrossRef PubMed
.
- H. Eyring, The Activated Complex and the Absolute Rate of Chemical Reactions, Chem. Rev., 1935, 17, 65–77 CrossRef CAS
.
- J. Åqvist, M. Kazemi, G. V. Isaksen and B. O. Brandsdal, Entropy and Enzyme Catalysis, Acc. Chem. Res., 2017, 50, 199–207 CrossRef PubMed
.
- D. Biswakarma, N. Dey and S. Bhattacharya, A Biocompatible Hydrogel as a Template for Oxidative Decomposition Reactions: A Chemodosimetric Analysis and In Vitro Imaging of Hypochlorite, Chem. Sci., 2022, 13, 2286–2295 RSC
.
Footnote |
† Electronic supplementary information (ESI) available: Detailed synthetic procedures and characterisations; representative spectral of UV/Vis spectra. See DOI: https://doi.org/10.1039/d4ob00582a |
|
This journal is © The Royal Society of Chemistry 2024 |
Click here to see how this site uses Cookies. View our privacy policy here.