Synthesis, characterization and photophysical studies of dual-emissive base-modified fluorescent nucleosides†‡
Received
8th May 2024
, Accepted 16th May 2024
First published on 24th May 2024
Abstract
A straightforward and efficient methodology has been employed for the synthesis of a diverse set of base-modified fluorescent nucleoside conjugates via Cu(I)-catalysed cycloaddition reaction of 5-ethynyl-2′,3′,5′-tri-O-acetyluridine/3′,5′-di-O-acetyl-2′-deoxyuridine with 4-(azidomethyl)-N9-(4′-aryl)-9,10-dihydro-2H,8H-chromeno[8,7-e][1,3]oxazin-2-ones in tBuOH to afford the desired 1,2,3-triazoles in 92–95% yields. Treatment with NaOMe/MeOH resulted in the final deprotected nucleoside analogues. The synthesized 1,2,3-triazoles demonstrated a significant emission spectrum, featuring two robust bands in the region from 350–500 nm (with excitation at 300 nm) in fluorescence studies. Photophysical investigations revealed a dual-emissive band with high fluorescence intensity, excellent Stokes shift (140–164 nm) and superior quantum yields (0.068–0.350). Furthermore, the electronic structures of the synthesized triazoles have been further verified by DFT studies. Structural characterization of all synthesized compounds was carried out using various analytical techniques, including IR, 1H-NMR, 13C-NMR, 1H–1H COSY, 1H–13C HETCOR experiments, and HRMS measurements. The dual-emissive nature of these nucleosides would be a significant contribution to nucleoside chemistry as there are limited literature reports on the same.
1. Introduction
For over half a century, modified nucleosides have found applications in the field of nucleic acid chemistry.1 The significance of modified nucleosides was immensely acknowledged in the year 2023 for modifying the nucleoside base. Katalin Karikó and Prof. Drew Weismann received the Nobel Prize for modifying the nucleoside base, in particular a uridine base with a pseudo uridine. The realm of fluorescent analogues for nucleic acid bases has grown considerably in recent times due to the non-emissive nature of canonical bases in nucleic acids.2 The fascination towards fluorescent nucleosides featuring modified bases is due to their extraordinary responsiveness to the microenvironment, making them potent tools for investigating the structural intricacies of nucleic acids. Moreover, they serve as powerful molecules for elucidating the biological processes of nucleic acids, including replication, transcription, recombination, and repair.3–10
Diverse modified nucleosides with extended conjugation have been synthesized and successfully utilized in DNA labelling due to their inherent fluorescent character (Fig. 1)11–17 In general, the 5-position of pyrimidines is a favourable site for transformation because substituents introduced here tend to be accommodated well in the major groove without obstructing the Watson–Crick face.6,18
 |
| Fig. 1 Some literature reports for fluorescent base modified triazole nucleosides. | |
Fluorescent coumarin analogues have been extensively employed in various applications such as laser dyes,19 fluorescent labels,20 chemo-sensors,21 and organic light-emitting diodes.22 The inherent limitations of the unsubstituted coumarin fluorophore, notably its small Stokes shift, pose challenges in various applications such as fluorescent molecular probes.23,24 Dihydro-oxazines are known for their compact nature, improving the Stokes shift values, which have made them promising candidates for application as fluorescent probes.25 To overcome these drawbacks and enhance the utility of the coumarin scaffold as a fluorescent probe with larger Stokes shift values, we have coupled dihydro[1,3]oxazine with coumarin derivatives.
Furthermore, most environment-sensitive fluorescent nucleosides exhibit single-band emission and often lack microenvironment sensitivity and have low quantum yields.26,27 Introducing two-band emission allows for ratiometric sensing through emission ratios.28 However, dual emissive fluorophores are rare due to synthesis challenges. Thus, dual emission-based biomolecular sensing, particularly in DNA analysis, remains poorly investigated.17,29 Developing dual-emissive modified nucleosides is an unavoidable research area for detecting DNA microenvironment changes.
In continuation of our research efforts on developing modified fluorescent nucleosides,30–32 we aimed to develop dual-emissive fluorescent nucleosides with better fluorescence properties. For this purpose, we designed oxazine-coupled coumarin-based triazole-linked33 modified nucleosides.14,34–36 Herein, we present the synthesis and comprehensive fluorescence, photophysical and DFT studies on our newly synthesized novel fluorophores.
2. Results and discussion
2.1 Chemistry
It was envisioned that our desired fluorophores could be obtained by the reaction of dihydro[1,3]-oxazines 6a–f with 5-ethynyl-2′,3′,5′-tri-O-acetyl-2′-deoxyuridine 11a or 3′,5′-di-O-acetyl-2′-deoxyuridine 11b through a copper(I)-catalyzed Huisgen (3 + 2) cycloaddition reaction (Scheme 1).
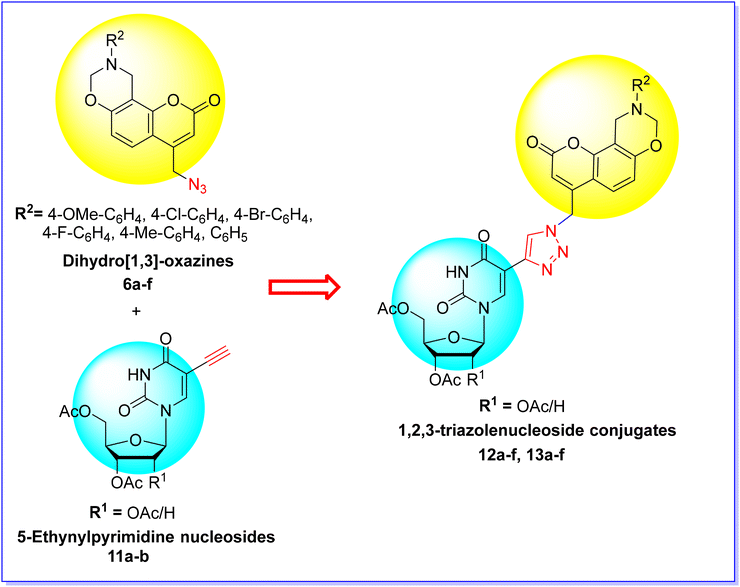 |
| Scheme 1 Precursors for fluorescent coumarin-triazole nucleoside conjugates. | |
The first aim of this study was to construct a series of N-substituted-dihydro[1,3]-oxazine derivatives integrated onto the aryl ring of 7-hydroxy-4-azidomethyl coumarin 4. Our objective was to effectively combine the two motifs in order to generate a molecule with a high Stokes shift value. Consequently, we successfully synthesized the dihydro[1,3]oxazine analogues 6a–f in good yields (Scheme 2).
 |
| Scheme 2 Synthesis of coumarin-based dihydro[1,3]-oxazines 6a–f. | |
To synthesize compounds 6a–f, the precursor 7-hydroxy-4-chloromethylcoumarin 3 was initially prepared using the Pechmann condensation reaction of ethyl 4-chloroacetoacetate 2 and resorcinol 1 in the presence of conc. sulphuric acid.37 The 7-hydroxy-4-chloromethylcoumarin 3 thus obtained on being treated with NaN3 in MeCN at 80 °C resulted in the formation of 7-hydroxy-4-azidomethylcoumarin 4.38 Thereafter, six coumarin based dihydro[1,3]-oxazines were synthesized in 72–86% yields by following the Mannich-type condensation reaction of 7-hydroxy-4-azidomethylcoumarin 4 with formaldehyde and primary amines 5a–f in 1,4-dioxane at 100 °C, as described in Scheme 2.39
The structure of the dihydro[1,3]-oxazine 6f was further confirmed by its single crystal X-ray structural analysis (Fig. 7). Furthermore, the thus synthesized dihydro[1,3]-oxazines were subjected to the Cu(I)-catalyzed reaction. Initially, the click reaction40,41 was carried out between the coumarin-based dihydro[1,3]-oxazine 6a and 5-ethynyl-2′,3′,5′-tri-O-acetyl-2′-deoxyuridine 11a. The reaction was performed in the presence of sodium ascorbate and CuSO4·5H2O in a solvent mixture of THF
:
tBuOH
:
H2O (1
:
1
:
1) at ambient temperature, following the procedure outlined in the literature.42 The reaction using the specified solvent system did not lead to complete consumption of the reactants even after 24 hours. Subsequently, the click reaction between the coumarin-based dihydro[1,3]-oxazine 6a and 5-ethynyl-2′,3′,5′-tri-O-acetyl-2′-deoxyuridine 11a was explored in different solvents and at various temperatures to optimize both the yield and efficiency of the reaction. It was observed that the reaction afforded 95% product yield in neat tBuOH at 80 °C.32,43 Subsequently, the click reaction involving coumarin-based dihydro[1,3]-oxazines 6a–f and the 5-ethynyl-2′,3′,5′-tri-O-acetyl-2′-deoxyuridine 11a or 3′,5′-di-O-acetyl-2′-deoxyuridine 11b was performed in tBuOH at 80 °C. The resultant oxazine coupled coumarin-based 1,2,3-triazole conjugates 12a–f and 13a–f were obtained in 92–95% yields (Scheme 3). Furthermore, the deacetylation of the nucleoside conjugates 12a–f and 13a–f was carried out using NaOMe in MeOH at 25 °C, resulting in the desired 1,2,3-triazole nucleoside conjugates 14a–f and 15a–f in quantitative yields (Scheme 3).
 |
| Scheme 3 Synthesis of oxazine coupled coumarin-based 1,2,3-triazole conjugates 12a–f, 13a–f, 14a–f and 15a–f. | |
The structures of all the known compounds were confirmed by comparison of their spectral data with those reported in the literature.32,37,38,44,45 The structures of the newly synthesized compounds 6a–f, 12a–f, 13a–f, 14a–f and 15a–f were unambiguously established on the basis of their spectral (IR, 1H-NMR, 13C-NMR, 1H–1H COSY NMR, 1H–13C HETCOR NMR, NOESY NMR, DEPT-135 NMR and HRMS) data analysis. The hydroxyl groups present in the sugar moiety of the target compounds 14a–f and 15a–f were confirmed by 1H NMR spectra using D2O exchange experiments (see the ESI‡).
2.2. Photophysical characterization
Fluorescence is the physical property of a compound in which absorbed light is emitted and the extent of emission determines the fluorescence intensity. Numerous modified fluorescent nucleoside analogues have been explored for the study of the local structure and dynamics of nucleic acids. The synthesized compounds herewith have extended conjugations and thus their fluorescence properties were explored.
Photophysical studies of the synthesized nucleoside analogues, 14a–f and 15a–f, were carried out in DMSO. Attempts to perform the experiments in other solvents failed due to the poor solubility of these compounds.
2.2.1. Absorption spectra.
The absorption spectra of the starting materials 11a and 11b were recorded in DMSO at 2 × 10−5 M concentration. No characteristic peak was observed in the emission spectra. However, when the absorption spectra of the 1,2,3-triazoles 14a–f and 15a–f were recorded in DMSO at the same concentration at 25 °C (Fig. 2), a distinct peak at around 300 nm was observed with high absorption for all the compounds. A broad band was observed, which could be possibly due to the extended conjugation at the C-5 position of the nucleoside.
 |
| Fig. 2 UV/Vis absorption spectra for compounds 14a–f and 15a–f in DMSO at 2 × 10−5 M concentration. | |
2.2.2 Emission spectra.
The fluorescence spectra of the 1,2,3-triazoles 14a–f and 15a–f were recorded in DMSO at 2 × 10−5 M concentration at 25 °C (Fig. 3). Excitation of the nucleosides at λabs value = 300 nm resulted in maximum fluorescence of the compounds. The emission spectra of each compound depicted two strong bands at 360–380 nm and 450–480 nm and thus these nucleosides exhibited a dual-emissive behaviour. The electron donating group at the C-4′′′′ position in 14a and 14f resulted in an increase in the fluorescence intensity, while those with electron withdrawing groups at the C-4′′′′ position, i.e., 14b, 14c and 14e, were found to have lower values of fluorescence intensity. In the case of 2′-deoxyuridine analogues 15a–f, the highest fluorescence intensity was observed for compound 15d, followed by 15a and lower values for 15b, 15c and 15e. All the synthesized compounds exhibited high Stokes shift values (140–164 nm). Fluorescent nucleosides with high Stokes shift values have an added advantage as the possible spectral overlap of the absorption and emission spectra is eliminated and fluorescence can thus be easily detected.46
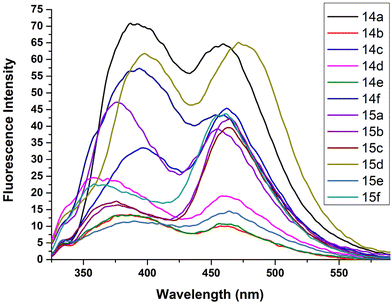 |
| Fig. 3 Emission spectra for compounds 14a–f and 15a–f in DMSO at 2 × 10−5 M concentration (excitation at λex = 300 nm). | |
2.2.3. Quantum yield calculations.
Furthermore, the fluorescence quantum yields (ΦF) of the 1,2,3-triazole conjugates 14a–f and 15a–f were calculated by using the following equation with quinine sulphate as the reference (Φst = 0.54 in 0.1 M H2SO4):
ΦF = Φst × Su/Sst × Ast/Au × nDu2/nDst2 |
here, ΦF = emission quantum yield of the synthesized compounds 14a–f and 15a–f, Φst = emission quantum yield of the standard, Sst = integrated emission band area of the standard, Su = integrated emission band area of the samples, Ast = absorbance of the standard at the excitation wavelength, Au = absorbance of the samples at the excitation wavelength, nDst = solvent refractive index of the standard, and nDu = solvent refractive index of the sample.
The subscripts ‘u’ and ‘st’ are used to denote the unknown samples and the standard, respectively. The quantum yields thus calculated are tabulated in Table 1.
Table 1 Emission spectra data for 1,2,3-triazoles 14a–f and 15a–f
Compound no. |
Absorbance λabs (nm) |
Emission λem (nm) |
Stokes shift (nm) |
Quantum yield (ΦF) |
Extinction coefficient ε (M−1 cm−1) |
14a
|
310 |
392/460 |
150 |
0.350 |
22 850 |
14b
|
311 |
380/463 |
152 |
0.068 |
24 350 |
14c
|
310 |
396/462 |
152 |
0.150 |
23 600 |
14d
|
306 |
368/458 |
152 |
0.159 |
18 750 |
14e
|
315 |
390/465 |
150 |
0.082 |
20 450 |
14f
|
313 |
393/453 |
140 |
0.226 |
19 650 |
15a
|
309 |
376/454 |
145 |
0.261 |
22 350 |
15b
|
310 |
377/464 |
154 |
0.109 |
24 500 |
15c
|
309 |
375/463 |
154 |
0.150 |
21 850 |
15d
|
307 |
397/471 |
164 |
0.243 |
20 800 |
15e
|
306 |
390/463 |
157 |
0.093 |
19 100 |
15f
|
310 |
358/461 |
151 |
0.254 |
16 900 |
The compounds exhibited varied trends of fluorescence properties. The quantum yield values ranged from 0.068–0.350, the highest being 0.350 for compound 14a. The high quantum yields of these compounds can be attributed to the extended conjugation at the C-5 position of the nucleoside. Furthermore, derivatives with electron withdrawing substituents, i.e., 14b, 14c, 14e, 15b, 15c and 15e, exhibited lower values of quantum yields while those with electron donating substituents at the C-4′′′′ position resulted in higher quantum yields. Moreover, since H-bonding often exerts a strong influence on the fluorescence quantum yield, we may attribute the small differences observed in the ribo- vs. the deoxyribo-version to the extra OH group at the C-2′ position in the case of the ribose version, which is absent in the case of the deoxyribose one.
2.2.4. Solvatochromism.
Although the photophysical study of the nucleosides 14a–f and 15a–f was carried out only in DMSO due to their limited solubility in other organic solvents, we performed detailed solvatochromic studies of fluorophores 6a–f in five different organic solvents, acetonitrile, MeOH, chloroform, DMSO, and 1,4-dioxane (Fig. 4 and 5), to study the effect of change of solvent polarity on the photophysical properties of these fluorophores.
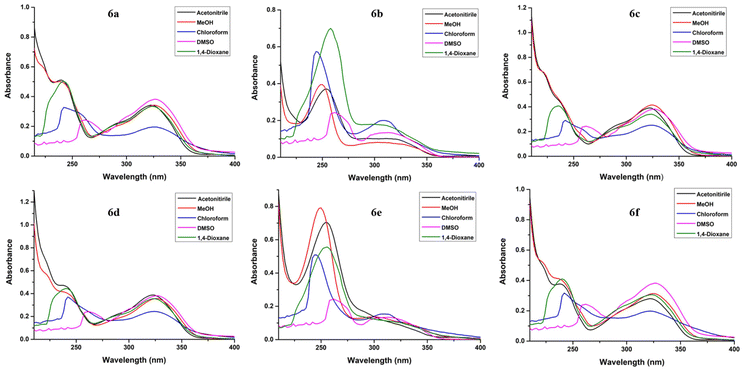 |
| Fig. 4 UV/Vis absorption spectra for fluorophores 6a–f in acetonitrile, MeOH, chloroform, DMSO and 1,4-dioxane at 2 × 10−5 M concentration. | |
 |
| Fig. 5 Emission spectra for fluorophores 6a–f in acetonitrile, MeOH, chloroform, DMSO and 1,4-dioxane at 2 × 10−5 M concentration (excitation at λex = 250 nm). | |
The absorption and emission spectra of fluorophores 6a–f were recorded in acetonitrile, MeOH, chloroform, DMSO, and 1,4-dioxane. The absorption spectra of all the compounds exhibited almost a similar pattern, that is, two absorption bands in the range 234–262 nm and 302–326 nm, in all the studied solvents (Fig. 4) except DMSO, wherein the lower wavelength peak was concealed by the absorption of the solvent.
A distinct hypsochromic shift was observed in the emission spectra of 6a–f for 1,4-dioxane (292–293 nm), while a distinct bathochromic shift was observed for chloroform (390–416 nm) (Table 2). Also, high values of Stokes shift were observed in the case of chloroform (146–174 nm) while lowest values were observed in the case of 1,4-dioxane (35–58 nm). Moreover, the highest value of quantum yield was observed in the case of 1,4-dioxane (0.194–0.498) for each of the compounds 6a–f. The highest fluorescence intensity was observed in the case of 1,4-dioxane for all the compounds. The emission wavelength, quantum yield, Stokes shift values and the molar absorption coefficient of compounds 6a–f in different solvents are tabulated in Table 2.
Table 2 Emission spectra data for fluorophores 6a–f in acetonitrile, MeOH, chloroform, DMSO and 1,4-dioxane at 2 × 10−5 M concentration
Compound no. |
Properties |
Solvent |
Acetonitrile |
MeOH |
Chloroform |
DMSO |
1,4-Dioxane |
6a
|
Absorbance λabs (nm) |
240 |
238 |
242 |
262 |
242 |
Emission λem (nm) |
358 |
357 |
416 |
370 |
292 |
Stokes shift (nm) |
118 |
119 |
174 |
108 |
50 |
Quantum yield (ΦF) |
0.064 |
0.025 |
0.009 |
0.004 |
0.257 |
Extinction coefficient ε (M−1 cm−1) |
14 100 |
19 750 |
16 350 |
12 150 |
25 150 |
6b
|
Absorbance λabs (nm) |
254 |
250 |
245 |
261 |
258 |
Emission λem (nm) |
365 |
356 |
392 |
368 |
293 |
Stokes shift (nm) |
111 |
106 |
147 |
107 |
35 |
Quantum yield (ΦF) |
0.080 |
0.026 |
0.013 |
0.010 |
0.498 |
Extinction coefficient ε (M−1 cm−1) |
18 550 |
19 750 |
28 700 |
12 150 |
35 000 |
6c
|
Absorbance λabs (nm) |
238 |
239 |
242 |
262 |
234 |
Emission λem (nm) |
370 |
371 |
410 |
397 |
292 |
Stokes shift (nm) |
132 |
132 |
168 |
135 |
58 |
Quantum yield (ΦF) |
0.187 |
0.022 |
0.007 |
0.008 |
0.365 |
Extinction coefficient ε (M−1 cm−1) |
7900 |
12 400 |
14 550 |
11 800 |
20 200 |
6d
|
Absorbance λabs (nm) |
240 |
241 |
242 |
260 |
240 |
Emission λem (nm) |
375 |
374 |
416 |
321 |
293 |
Stokes shift (nm) |
135 |
124 |
174 |
61 |
53 |
Quantum yield (ΦF) |
0.083 |
0.025 |
0.004 |
0.006 |
0.268 |
Extinction coefficient ε (M−1 cm−1) |
12 650 |
15 900 |
18 550 |
12 250 |
22 200 |
6e
|
Absorbance λabs (nm) |
255 |
249 |
244 |
260 |
254 |
Emission λem (nm) |
347 |
343 |
390 |
393 |
292 |
Stokes shift (nm) |
92 |
94 |
146 |
133 |
38 |
Quantum yield (ΦF) |
0.001 |
0.017 |
0.010 |
0.014 |
0.194 |
Extinction coefficient ε (M−1 cm−1) |
35 150 |
39 550 |
25 450 |
12 350 |
27 650 |
6f
|
Absorbance λabs (nm) |
239 |
238 |
242 |
259 |
240 |
Emission λem (nm) |
375 |
372 |
414 |
382 |
292 |
Stokes shift (nm) |
136 |
134 |
172 |
123 |
52 |
Quantum yield (ΦF) |
0.051 |
0.105 |
0.009 |
0.012 |
0.332 |
Extinction coefficient ε (M−1 cm−1) |
9150 |
14 350 |
15 850 |
11 900 |
20 500 |
2.2.5. Theoretical calculations/frontier molecular orbitals.
We next carried out the quantum mechanical calculations of the synthesized molecules for better understanding of their electronic structures using the Gaussian 09 program.47 The GaussView program48 was used to visualize the molecular structures. The DFT computations employed the B3LYP functional with the Becke's gradient-exchange correction49 along with the Lee–Yang–Parr correlation functional.50 The geometry optimizations utilized the B3LYP/6-311++G(d,p) basis set.51 The absence of negative vibrational modes in all the optimized structures confirmed their stability as stationary points throughout the optimization process.52
The resulting band gaps as well as the energies of the HOMO and LUMO were determined from the optimized structures (Fig. 6 and Table S1, see the ESI‡). The band gaps range from 0.122 to 0.133 eV in DMSO. Understanding these orbital energies is crucial as they reflect the chemical behaviour of the molecules. The HOMO and LUMO orbital structures of compounds 14a and 15a are shown in Fig. 6 while those for the other compounds are detailed in Table S1 in the ESI‡. In general, the LUMO is a typical electron acceptor, correlated with electron affinity (EA) while the HOMO is a typical electron donor, correlated with ionization potential (IP). The values of the HOMO–LUMO energy gap serve as useful indicators for a molecule's chemical reactivity. The reduction in this gap elucidates the potential charge transfer interactions within the compounds under study, attributed to the strong electron-withdrawing capabilities of certain functional groups. For efficient charge separation, the localization of the HOMO on the donor moiety and the LUMO on the acceptor moiety is considered pertinent.53 In this study, the coumarin and the triazole ring comprised the LUMO whilst the oxazine ring formed the HOMO, irrespective of its substituents.
 |
| Fig. 6 HOMO–LUMO frontier molecular orbitals for nucleosides (a) 14a and (b) 15a calculated using the Gaussian 09 program package. | |
These orbital contour diagrams distinctly suggest the generation of the charge-separated state by the transitions from the highest occupied molecular orbital to the lowest occupied molecular orbital. Moreover, these transitions can be attributed to be the intramolecular charge transfer (ICT) interactions from the donor to the acceptor moieties. Additionally, the significant overlap between the HOMO and LUMO implies effective electron donation and withdrawal properties for the donor and acceptor, respectively. Consequently, this strong overlap enhances the ICT between the donor and acceptor, thereby facilitating fluorescence exhibited by these compounds.
After optimizing the structures, the TDDFT method, known as the time-dependent density functional theory, was then employed to calculate the vertical excited state energies as well the oscillator strengths (f) for the electronic transitions of compound 13a.54 The TDDFT calculations were conducted on the optimized ground state geometries utilizing the 6-31++G(d,p) basis set. Subsequently, the absorption spectra was generated based on the obtained data (Fig. S78, see the ESI‡). The observed difference between the theoretical and experimental results can be attributed to the fact that realistically, the experimental results belong to solution which comprises several inter- and intramolecular interactions.
3. Conclusion
In light of the significance of triazole-linked fluorescent nucleosides, we herein present the synthesis of novel dihydro[1,3]oxazine-coumarin-based-1,2,3-triazole nucleoside analogues via the copper(I)-catalyzed Huisgen (3 + 2) cycloaddition reaction. Subsequently, comprehensive fluorescence and photophysical studies were also performed for the thus synthesized derivatives. The fluorophores 6a–f demonstrated variations in the fluorescence properties with changes in solvent polarity. The higher quantum yield values ranged from 0.194 to 0.498, with the highest fluorescence intensity observed in 1,4-dioxane. Notably, the highest quantum yield of 0.498 was recorded for compound 6b. Moreover, in our study, we witnessed a dual-emission phenomenon for all the synthesized nucleoside analogues, presenting the distinct advantage for DNA sensing in contrast to the commonly employed single-band fluorescent nucleosides. The electronic structure of these nucleosides was thoroughly investigated using the Density Functional Theory (DFT). We hypothesize that certain modified nucleosides among these could prove to be excellent candidates for fluorophores, given their substantial Stokes shifts and high quantum yields. Furthermore, to thoroughly assess the dual-emissive properties of these modified nucleosides within the oligonucleotide framework, it is necessary to synthesize oligonucleotides that incorporate these modified nucleosides.
4. Experimental
All chemicals and solvents were purchased from Alfa Aesar, Thermo Fisher Scientific, India Pvt. Limited, Sigma-Aldrich Chemicals Pvt. Limited, India and from local commercial sources and were used without any further purification unless otherwise specified. Solvents for column chromatography were dried and distilled prior to use. Solvents were removed under reduced pressure using a rotary evaporator, followed by further removal of the residual solvent under high vacuum. Column chromatography was performed on silica gel (100–200 mesh). Melting points were determined on a Buchi M-560 instrument and are uncorrected. HRMS analysis was carried out using an Agilent G6530AA LC Q-TOF mass spectrometer using the ESI method. The IR spectra of compounds were recorded on a PerkinElmer model 2000 FT-IR spectrometer and are expressed as wavenumber (cm−1). The Rf values of compounds are reported from the analytical TLC study using the specified solvents and 0.25 mm silica gel 60 F254 plates that were visualized by UV irradiation or by charring with a 5% alcoholic sulfuric acid solution. The 1H- and the 13C- and other 2D NMR spectra were recorded on JEOL alpha-400 and Bruker-Avance Neo 400 FT-NMR spectrometers by using tetramethylsilane (TMS) as the internal standard. The chemical shift values are on the δ scale and the coupling constant (J) are in Hz. Absorption spectra were recorded between 200 nm and 600 nm using a PerkinElmer UV/Vis spectrometer Lambda 45 at a scan speed 240 nm min−1. All measurements were carried out in PEUV/Vis spectroscopy cells (1 cm). HPLC grade solvents were used for all solution preparation.
4.1. General procedure for the synthesis of 4-(azidomethyl)-N9-(4′-aryl)-9,10-dihydro-2H,8H-chromeno[8,7-e][1,3]oxazin-2-ones (6a–f)
A solution of primary amines 5a–f (1.1 mmol) in dioxane (5 mL) was treated with formalin solution (2.5 mmol). The resulting mixture was stirred at room temperature for 3–5 h. Subsequently, 7-hydroxy-4-azidomethylcoumarin 4 (1 mmol) and a catalytic amount of DMAP were added to the reaction mixture. The reaction was then stirred at 100 °C until complete consumption of the reactants (the progress of the reaction was examined by thin layer chromatography). After the completion of the reaction, the solvent was evaporated under reduced pressure and the crude product was purified by using silica gel column chromatography using ethylacetate and hexane as the solvent to obtain the desired compounds 6a–f in 72–86% yields.
4.1.1 4-(Azidomethyl)-N9-(4′-methoxyphenyl)-9,10-dihydro-2H,8H-chromeno[8,7-e][1,3]oxazin-2-one (6a).
It was obtained as a yellow solid. Yield: 0.28 g (78%). Rf = 0.48 (30% ethyl acetate in petroleum ether); melting point = 140–142 °C; IR (KBr, cm−1): 2116, 1732, 1517, 1412, 1298, 1143, 1057, 926, 781, 719; 1H NMR (CDCl3, 400 MHz): δ 7.29 (d, J = 8.8 Hz, 1H, ArH), 7.10–7.07 (m, 2H, ArH), 6.82–6.79 (m, 3H, ArH), 6.33 (s, 1H, C-3H), 5.38 (s, 2H, CH2), 4.73 (s, 2H, CH2), 4.47 (d, J = 1.0 Hz, 2H, CH2N3), 3.74 (s, 3H, OCH3); 13C NMR (CDCl3, 100 MHz): δ 160.5 (C
O), 158.0 (ArC), 155.4 (ArC), 151.6 (ArC), 149.2 (ArC), 141.7 (ArC), 122.8 (ArC), 120.8 (ArC), 114.6 (ArC), 114.1 (ArC), 111.1 (ArC), 110.5 (C-3), 109.3 (ArC), 81.1 (CH2), 55.6 (OCH3), 50.8 (CH2N3), 46.9 (CH2); HRMS (ESI): m/z calculated for C19H17N4O4+ [M + H]+: 365.1250, found: 365.1255.
4.1.2 4-(Azidomethyl)-N9-(4′-chlorophenyl)-9,10-dihydro-2H,8H-chromeno[8,7-e][1,3]oxazin-2-one (6b).
It was obtained as a yellow solid. Yield: 0.28 g (75%). Rf = 0.52 (30% ethyl acetate in petroleum ether); melting point = 134–136 °C; IR (KBr, cm−1): 2100, 1715, 1591, 1488, 1360, 1307, 1248, 1157, 1091, 916, 796, 713;1H NMR (CDCl3, 400 MHz): δ 7.31 (d, J = 8.8 Hz, 1H, ArH), 7.22 (d, J = 8.7 Hz, 2H, ArH), 7.06 (d, J = 8.7 Hz, 2H, ArH), 6.79 (d, J = 8.8 Hz, 1H, ArH), 6.35 (s, 1H, C-3H), 5.40 (s, 2H, CH2), 4.78 (s, 2H, CH2), 4.48 (d, J = 1.0 Hz, 2H, CH2N3); 13C NMR (CDCl3, 100 MHz): δ 160.2 (C
O), 157.7 (ArC), 151.5 (ArC), 149.0 (ArC), 146.4 (ArC), 129.3 (ArC), 127.3 (ArC), 123.0 (ArC), 119.9 (ArC), 114.0 (ArC), 111.3 (ArC), 110.7 (C-3), 109.0 (ArC), 79.8 (CH2), 50.8 (CH2N3), 46.5 (CH2); HRMS (ESI): m/z calculated for C18H14ClN4O+ [M + H]+: 369.0754, found: 369.0772.
4.1.3 4-(Azidomethyl)-N9-(4′-fluorophenyl)-9,10-dihydro-2H,8H-chromeno[8,7-e][1,3]oxazin-2-one (6c).
It was obtained as a yellow solid. Yield: 0.25 g (72%). Rf = 0.53 (30% ethyl acetate in petroleum ether); melting point = 124 °C; IR (KBr, cm−1): 2118, 1711, 1556, 1414, 1336, 1222, 1154, 1071, 879, 743;1H NMR (CDCl3, 400 MHz): δ 7.30 (d, J = 8.8 Hz, 1H, ArH), 7.08 (dd, J = 9.1 Hz, 4.6 Hz, 2H, ArH), 6.94–6.79 (m, 2H, ArH), 6.78 (d, J = 8.8 Hz, 1H, ArH), 6.33 (s, 1H, C-3H), 5.36 (s, 2H, CH2), 4.74 (s, 2H, CH2), 4.47 (s, 2H, CH2N3); 13C NMR (CDCl3, 100 MHz): δ 160.4 (C
O), 159.7 (ArC), 157.9 (ArC), 157.3 (ArC), 151.5 (ArC), 149.2 (ArC), 144.3 (ArC), 144.3 (ArC), 123.0 (ArC), 120.7 (ArC), 120.6 (ArC), 116.1 (ArC), 115.9 (ArC), 114.1 (ArC), 111.3 (ArC), 110.7 (C-3), 109.1 (ArC), 80.6 (CH2), 50.8 (CH2N3), 46.9 (CH2); HRMS (ESI): m/z calculated for C18H14FN4O3+ [M + H]+: 353.1050, found: 353.1059.
4.1.4 4-(Azidomethyl)-N9-phenyl-9,10-dihydro-2H,8H-chromeno[8,7-e][1,3]oxazin-2-one (6d).
It was obtained as a yellow solid. Yield: 0.27 g (82%). Rf = 0.56 (30% ethyl acetate in petroleum ether); melting point = 129 °C; IR (KBr, cm−1): 2110, 1709, 1595, 1495, 1388, 1296, 1242, 1205, 1158, 1037, 894, 840, 753, 685; 1H NMR (CDCl3, 400 MHz): δ 7.30–7.26 (m, 3H, ArH), 7.15–7.12 (m, 2H, ArH), 6.98–6.94 (m, 1.0 Hz, 1H, ArH), 6.78 (d, J = 8.8 Hz, 1H, ArH), 6.34 (d, J = 1.0 Hz, 1H, C-3H), 5.44 (s, 2H, CH2), 4.82 (s, 2H, CH2), 4.47 (s, 2H, CH2N3); 13C NMR (CDCl3, 100 MHz): δ 160.5 (C
O), 158.5 (ArC), 151.5 (ArC), 149.2 (ArC), 147.9 (ArC), 129.5 (ArC), 122.9 (ArC), 122.2 (ArC), 118.6 (ArC), 117.7 (ArC), 114.1 (ArC), 111.2 (C-3), 109.4 (ArC), 80.0 (CH2), 50.8 (CH2N3), 46.4 (CH2); HRMS (ESI): m/z calculated for C18H15N4O3+ [M + H]+: 335.1144, found: 335.1151.
4.1.5 4-(Azidomethyl)-N9-(4′-bromophenyl)-9,10-dihydro-2H,8H-chromeno[8,7-e][1,3]oxazin-2-one (6e).
It was obtained as a yellow solid. Yield: 0.36 g (86%). Rf = 0.54 (30% ethyl acetate in petroleum ether); melting point = 131–132 °C; IR (KBr, cm−1): 2106, 1732, 1464, 1341, 1224, 1138, 1057, 923, 787, 707;1H NMR (CDCl3, 400 MHz): δ 7.37–7.36 (m, 1H, ArH), 7.35–7.34 (m, 1H, ArH), 7.31 (d, J = 8.9 Hz, 1H, ArH), 7.02–7.01 (m, 1H, ArH), 7.00–6.99 (m, 1H, ArH), 6.78 (d, J = 8.8 Hz, 1H, ArH), 6.34 (s, 1H,C-3H), 5.39 (s, 2H, CH2), 4.78 (s, 2H, CH2), 4.47 (d, J = 0.9 Hz, 2H, CH2N3); 13C NMR (CDCl3, 100 MHz): δ 160.3 (C
O), 157.8 (ArC), 151.5 (ArC), 149.1 (ArC), 147.0 (ArC), 132.4 (ArC), 123.0 (ArC), 120.3 (ArC), 114.8 (ArC), 114.1 (ArC), 111.4 (ArC), 110.7 (C-3), 109.1 (ArC), 79.7 (CH2), 50.9 (CH2N3), 46.5 (CH2); HRMS (ESI): m/z calculated for C18H14BrN4O3+ [M + H]+: 413.0249, found: 413.0266.
4.1.6 4-(Azidomethyl)-N9-(4′-methylphenyl)-9,10-dihydro-2H,8H-chromeno[8,7-e][1,3]oxazin-2-one (6f).
It was obtained as a yellow solid. Yield: 0.28 g (79%). Rf = 0.63 (30% ethyl acetate in petroleum ether); melting point = 118–120 °C; IR (KBr, cm−1): 2103, 1726, 1550, 1478, 1336, 1317, 1231, 1129, 1064, 921, 771, 725;1H NMR (CDCl3, 400 MHz): δ 7.20 (d, J = 8.8 Hz, 1H, ArH), 6.99–6.97 (m, 4H, ArH), 6.70 (d, J = 8.8 Hz, 1H, ArH), 6.25 (s, 1H, C-3H), 5.33 (s, 2H, CH2), 4.70 (s, 2H, CH2), 4.39 (d, J = 0.8 Hz, 2H, CH2N3), 2.18 (s, 3H, CH3); 13C NMR (CDCl3, 100 MHz): δ 160.2 (C
O), 157.7 (ArC), 151.5 (ArC), 149.0 (ArC), 146.4 (ArC), 129.3 (ArC), 127.3 (ArC), 123.0 (ArC), 119.9 (ArC), 114.0 (ArC), 111.3 (ArC), 110.7 (C-3), 109.0 (ArC), 79.8 (CH2), 50.8 (CH2N3), 46.5 (CH2), 20.6 (CH3); HRMS (ESI): m/z calculated for C19H16N4NaO3+ [M + Na]+: 371.1120, found: 371.1104.
4.2. General procedure for the synthesis of N1-(9′′′-(4′′′′-substituted)-9′′′,10′′′-dihydro-2′′′H,8′′′H-chromeno[8′′′,7′′′-e][1′′′,3′′′]oxazin-2′′′-one-4′′′-methyl)-C4-(2′,3′,5′-tri-O-acetyl/3′,5′-di-O-acetyl-β-D-ribofuranos-1′-(2′′,4′′-dioxo-3′′,4′′-dihydropyrimidin))-1,2,3-triazole conjugates (12a–f and 13a–f)
A mixture of freshly synthesized dihydro[1,3]oxazines 6a–f (1.2 mmol) and 5-ethynyl-2′,3′,5′-tri-O-acetyl/3′,5′-di-O-acetyl-2′-deoxyuridines 11a–b (1.0 mmol) was stirred at 80 °C in tBuOH (20 mL). Subsequently, a solution of sodium ascorbate (0.40 mmol) in H2O was added followed by the addition of CuSO4·5H2O (0.20 mmol) solution in H2O. The progress of the reaction was monitored by TLC. After the completion of the reaction (as observed by TLC), the solvent was evaporated under reduced pressure and the crude product was purified by using silica gel column chromatography using methanol in chloroform as the solvent system to obtain the desired compounds 12a–f and 13a–f in 92–95% yields.
4.2.1
N
1-(9′′′-(4′′′′-Methoxy)-9′′′,10′′′-dihydro-2′′′H,8′′′H-chromeno[8′′′,7′′′-e][1′′′,3′′′]oxazin-2′′′-one-4′′′-methyl)-C4-(2′,3′,5′-tri-O-acetyl-β-D-ribofuranos-1′-(2′′,4′′-dioxo-3′′,4′′-dihydropyrimidin))-1,2,3-triazole (12a).
It was obtained as a bright yellow solid. Yield: 0.72 g (95%). Rf = 0.32 (5% methanol in chloroform); melting point = 252 °C; IR (KBr, cm−1): 3318, 3074, 2937, 2840, 1680, 1602, 1514, 1465, 1377, 1217, 1102, 1043, 895, 826, 593; 1H NMR (DMSO-d6, 400 MHz): δ 11.88 (s, 1H, N-3′′H), 8.58 (s, 1H, C-5H), 8.47 (s, 1H, C-6′′H), 7.66–7.63 (m, 1H, ArH), 7.11–7.06 (m, 2H, ArH), 6.86–6.79 (m, 3H, ArH), 6.11–6.08 (d, J = 5.3 Hz, 1H, C-1′H), 5.98 (s, 2H, CH2), 5.68 (s, 1H, ArH), 5.52–5.49 (m, 1H, C-2′H), 5.49–5.48 (m, 2H, CH2), 5.41–5.37 (m, 1H, C-3′H), 4.67 (s, 2H, CH2), 4.39–4.36 (m, 1H, C-4′H), 4.32–4.29 (m, 2H, C-5′H), 3.66 (s, 3H, OCH3), 2.14 (s, 3H, OCOCH3), 2.11 (s, 3H, OCOCH3), 2.06 (s, 3H, OCOCH3); 13C NMR (DMSO-d6, 100 MHz) δ 170.0 (C
O), 170.0 (C
O), 169.9 (C
O), 161.6 (ArC), 160.0 (ArC), 158.1 (ArC), 154.9 (ArC), 151.5 (ArC), 151.3 (ArC), 150.1 (ArC), 141.6 (ArC), 139.5 (C-4), 137.0 (C-6′′), 124.2 (C-5), 124.2 (ArC), 120.4 (ArC), 115.0 (ArC), 113.8 (ArC), 110.6 (ArC), 110.5 (ArC), 109.2 (ArC), 106.2 (ArC), 88.2 (C-1′), 81.2 (CH2), 80.0 (C-4′), 72.9 (C-2′), 70.5 (C-3′), 63.6 (C-5′), 55.7 (OCH3), 49.7 (CH2), 45.6 (CH2), 21.1 (OCOCH3), 20.9 (OCOCH3), 20.8 (OCOCH3); HRMS (ESI): m/z calculated for C36H34N6O13+ [M]+: 758.2184, found: 758.2140.
4.2.2
N
1-(9′′′-(4′′′′-Chloro)-9′′′,10′′′-dihydro-2′′′H,8′′′H-chromeno[8′′′,7′′′-e][1′′′,3′′′]oxazin-2′′′-one-4′′′-methyl)-C4-(2′,3′,5′-tri-O-acetyl-β-D-ribofuranos-1′-(2′′,4′′-dioxo-3′′,4′′-dihydropyrimidin))-1,2,3-triazole (12b).
It was obtained as a yellowish white solid. Yield: 0.71 g (93%). Rf = 0.36 (5% methanol in chloroform); melting point = 214 °C; IR (KBr, cm−1): 3037, 2833, 1747, 1711, 1692, 1604, 1496, 1363, 1267, 1225, 1218, 1100, 1059, 1047, 876, 817, 581; 1H NMR (DMSO-d6, 400 MHz) δ 11.83 (s, 1H, N-3′′H), 8.54 (s, 1H, C-5H), 8.43 (s, 1H, C-6′′H), 7.63–7.59 (m, 1H, ArH), 7.25–7.21 (m, 2H, ArH), 7.17–7.13 (m, 2H, ArH), 6.83–6.79 (m, 1H, ArH), 6.07–6.04 (m, 1H, C-1′H), 5.93 (s, 2H, CH2), 5.66 (s, 1H, ArH), 5.52 (s, 2H, CH2), 5.48–5.44 (m, 1H, C-2′H), 5.37–5.33 (m, 1H, C-3′H), 4.71 (s, 2H, CH2), 4.35–4.32 (m, 1H, C-4′H), 4.28–4.24 (m, 2H, C-5′H), 2.09 (s, 3H, OCOCH3), 2.06 (s, 3H, OCOCH3), 2.02 (s, 3H, OCOCH3); 13C NMR (DMSO-d6, 100 MHz) δ 170.7 (C
O), 170.0 (C
O), 170.0 (C
O), 161.6 (ArC), 160.0 (ArC), 157.9 (ArC), 151.2 (ArC), 151.2 (ArC), 150.1 (ArC), 146.9 (ArC), 139.5 (C-4), 137.0 (C-6′′), 129.5 (C-5), 125.6 (ArC), 124.3 (ArC), 124.2 (ArC), 120.1 (ArC), 113.9 (ArC), 110.8 (ArC), 110.7 (ArC), 109.2 (ArC), 106.2 (ArC), 88.2 (C-1′), 80.0 (CH2), 79.9 (C-4′), 72.9 (C-2′), 70.5 (C-3′), 63.6 (C-5′), 49.7 (CH2), 45.1 (CH2), 21.1 (OCOCH3), 20.9 (OCOCH3), 20.8 (OCOCH3); HRMS (ESI): m/z calculated for C35H32ClN6O12+ [M + H]+: 763.1761, found: 763.1760.
4.2.3
N
1-(9′′′-(4′′′′-Fluoro)-9′′′,10′′′-dihydro-2′′′H,8′′′H-chromeno[8′′′,7′′′-e][1′′′,3′′′]oxazin-2′′′-one-4′′′-methyl)-C4-(2′,3′,5′-tri-O-acetyl-β-D-ribofuranos-1′-(2′′,4′′-dioxo-3′′,4′′-dihydropyrimidin))-1,2,3-triazole (12c).
It was obtained as a yellow solid. Yield: 0.69 g (92%). Rf = 0.34 (5% methanol in chloroform); melting point = 222–226 °C; IR (KBr, cm−1): 2982, 2918, 1693, 1596, 1504, 1454, 1368, 1221, 1045, 879, 811, 757, 581; 1H NMR (DMSO-d6, 400 MHz) δ 11.89 (s, 1H, N-3′′H), 8.60–8.58 (m, 1H, C-5H), 8.49–8.47 (m, 1H, C-6′′H), 7.70–7.64 (m, 1H, ArH), 7.20–7.17 (m, 1H, ArH), 7.11–7.05 (m, 2H, ArH), 6.95–6.91 (m, 1H, ArH), 6.89–6.84 (m, 1H, ArH), 6.11–6.08 (m, 1H, C-1′H), 5.98 (s, 2H, CH2), 5.70–5.65 (m, 1H, ArH), 5.55–5.51 (m, 2H, CH2), 5.51–5.48 (m, 1H, C-2′H), 5.42–5.38 (m, 1H, C-3′H), 4.73 (s, 1H, C-4′H), 4.39–4.34 (m, 2H, CH2), 4.33–4.29 (m, 2H, C-5′H), 2.14 (s, 3H, OCOCH3), 2.11 (s, 3H, OCOCH3), 2.06 (s, 3H, OCOCH3); 13C NMR (DMSO-d6, 100 MHz) δ 170.7 (C
O), 170.0 (C
O), 170.0 (C
O), 161.6 (ArC), 160.0 (ArC), 158.0 (ArC), 151.5 (ArC), 151.2 (ArC), 150.1 (ArC), 144.6 (ArC), 139.4 (C-4), 137.0 (C-6′′), 124.3 (C-5), 124.2 (ArC), 120.5 (ArC), 120.4 (ArC), 116.3 (ArC), 116.1 (ArC), 113.8 (ArC), 110.7 (ArC), 110.6 (ArC), 109.1 (ArC), 106.2 (ArC), 88.2 (C-1′), 80.7 (CH2), 80.0 (C-4′), 72.9 (C-2′), 70.5 (C-3′), 63.6 (C-5′), 49.7 (CH2), 45.5 (CH2), 21.1 (OCOCH3), 20.9 (OCOCH3), 20.8 (OCOCH3); HRMS (ESI): m/z calculated for C35H32FN6O12+ [M + H]+: 747.2057, found: 747.2054.
4.2.4
N
1-(9′′′,10′′′-Dihydro-2′′′H,8′′′H-chromeno[8′′′,7′′′-e][1′′′,3′′′]oxazin-2′′′-one-4′′′-methyl)-C4-(2′,3′,5′-tri-O-acetyl-β-D-ribofuranos-1′-(2′′,4′′-dioxo-3′′,4′′-dihydropyrimidin))-1,2,3-triazole (12d).
It was obtained as a yellow solid. Yield: 0.68 g (94%). Rf = 0.36 (5% methanol in chloroform); melting point = 238–240 °C; IR (KBr, cm−1): 3082, 1686, 1598, 1506, 1447, 1364, 1214, 1103, 1049, 894, 820, 748, 691; 1H NMR (DMSO-d6, 400 MHz) δ 11.84 (s, 1H, N-3′′H), 8.54 (s, 1H, C-5H), 8.43 (s, 1H, C-6′′H), 7.63–7.58 (m, 1H, ArH), 7.23–7.18 (m, 2H, ArH), 7.14–7.10 (m, 2H, ArH), 6.88–6.83 (m, 1H, ArH), 6.83–6.79 (m, 1H, ArH), 6.07–6.04 (m, 1H, C-1′H), 5.93 (s, 2H, CH2), 5.64 (s, 1H, ArH), 5.53 (s, 2H, CH2), 5.48–5.43 (m, 1H, C-2′H), 5.37–5.33 (m, 1H, C-3′H), 4.72 (s, 2H, CH2), 4.35–4.31 (m, 1H, C-4′H), 4.28–4.24 (m, 2H, C-5′H), 2.09 (s, 3H, OCOCH3), 2.06 (s, 3H, OCOCH3), 2.02 (s, 3H, OCOCH3); 13C NMR (DMSO-d6, 100 MHz) δ 170.7 (C
O), 170.0 (C
O), 170.0 (C
O), 161.6 (ArC), 160.0 (ArC), 158.1 (ArC), 151.5 (ArC), 151.3 (ArC), 150.1 (ArC), 147.9 (ArC), 139.5 (C-4), 137.0 (C-6′′), 129.8 (ArC), 124.2 (C-5, ArC), 121.8 (ArC), 118.4 (ArC), 113.9 (ArC), 110.7 (ArC), 110.5 (ArC), 109.3 (ArC), 106.2 (ArC), 88.2 (C-1′), 80.2 (CH2), 80.0 (C-4′), 72.9 (C-2′), 70.5 (C-3′), 63.6 (C-5′), 49.7 (CH2), 45.0 (CH2), 21.1 (OCOCH3), 20.9 (OCOCH3), 20.8 (OCOCH3); HRMS (ESI): m/z calculated for C35H33N6O12+ [M + H]+: 729.2151, found: 729.2129.
4.2.5
N
1-(9′′′-(4′′′′-Bromo)-9′′′,10′′′-dihydro-2′′′H,8′′′H-chromeno[8′′′,7′′′-e][1′′′,3′′′]oxazin-2′′′-one-4′′′-methyl)-C4-(2′,3′,5′-tri-O-acetyl-β-D-ribofuranos-1′-(2′′,4′′-dioxo-3′′,4′′-dihydropyrimidin))-1,2,3-triazole (12e).
It was obtained as a yellowish white solid. Yield: 0.77 g (95%). Rf = 0.35 (5% methanol in chloroform); melting point = 275 °C; IR (KBr, cm−1): 3156, 2993, 2829, 1692, 1603, 1479, 1454, 1366, 1215, 1103, 1058, 1006, 887, 812, 581; 1H NMR (DMSO-d6, 400 MHz) δ 11.86 (s, 1H, N-3′′H), 8.59–8.58 (m, 1H, C-5H), 8.49–8.46 (m, 1H, C-6′′H), 7.69–7.64 (m, 1H, ArH), 7.42–7.37 (m, 2H, ArH), 7.17–7.13 (m, 2H, ArH), 6.89–6.84 (m, 1H, ArH), 6.12–6.08 (m, 1H, C-1′H), 5.98 (s, 2H, CH2), 5.73–5.70 (m, 1H, ArH), 5.56 (s, 2H, CH2), 5.53–5.49 (m, 1H, C-2′H), 5.42–5.38 (m, 1H, C-3′H), 4.76 (s, 2H, CH2), 4.39–4.36 (m, 1H, C-4′H), 4.33–4.29 (m, 2H, C-5′H), 2.15–2.14 (m, 3H, OCOCH3), 2.12–2.10 (m, 3H, OCOCH3), 2.08–2.06 (m, 3H, OCOCH3); 13C NMR (DMSO-d6, 100 MHz) δ 170.7 (C
O), 170.0 (C
O), 169.9 (C
O), 161.6 (ArC), 160.0 (ArC), 157.9 (ArC), 151.5 (ArC), 151.2 (ArC), 150.1 (ArC), 147.3 (ArC), 139.5 (C-4), 137.0 (C-6′′), 132.4 (ArC), 129.3 (C-5), 124.2 (ArC), 120.5 (ArC), 113.9 (ArC), 113.4 (ArC), 110.8 (ArC), 109.2 (ArC), 106.2 (ArC), 88.2 (C-1′), 80.0 (CH2), 79.8 (C-4′), 72.9 (C-2′), 70.5 (C-3′), 63.6 (C-5′), 49.7 (CH2), 45.1 (CH2), 21.1 (OCOCH3), 20.9 (OCOCH3), 20.8 (OCOCH3); HRMS (ESI): m/z calculated for C35H32BrN6O12+ [M + H]+: 807.1256, found: 807.1265.
4.2.6
N
1-(9′′′-(4′′′′-Methyl)-9′′′,10′′′-dihydro-2′′′H,8′′′H-chromeno[8′′′,7′′′-e][1′′′,3′′′]oxazin-2′′′-one-4′′′-methyl)-C4-(2′,3′,5′-tri-O-acetyl-β-D-ribofuranos-1′-(2′′,4′′-dioxo-3′′,4′′-dihydropyrimidin))-1,2,3-triazole (12f).
It was obtained as a yellow solid. Yield: 0.69 (93%). Rf = 0.38 (5% methanol in chloroform); melting point = 198 °C; IR (KBr, cm−1): 3302, 2921, 1693, 1602, 1506, 1435, 1371, 1223, 1092, 1039, 878, 814, 760, 711; 1H NMR (DMSO-d6, 400 MHz) δ 11.87 (brs, 1H, N-3′′H), 8.58 (s, 1H, C-5H), 8.47 (s, 1H, C-6′′H), 7.66–7.63 (m, 1H, ArH), 7.05–7.04 (m, 4H, ArH), 6.85–6.82 (m, 1H, ArH), 6.11–6.08 (m, 1H, C-1′H), 5.97 (s, 2H, CH2), 5.69–5.68 (m, 1H, ArH), 5.53–5.51 (m, 2H, CH2), 5.51–5.49 (m, 1H, C-2′H), 5.41–5.38 (m, 1H, C-3′H), 4.71 (s, 2H, CH2), 4.39–4.36 (m, 1H, C-4′H), 4.33–4.29 (m, 2H, C-5′H), 2.18 (s, 3H, OCOCH3), 2.14 (s, 3H, OCOCH3), 2.11 (s, 3H, CH3), 2.06 (m, 3H, OCOCH3); 13C NMR (DMSO-d6, 100 MHz) δ 170.7 (C
O), 170.0 (C
O), 169.9 (C
O), 161.6 (ArC), 160.0 (ArC), 158.1 (ArC), 151.5 (ArC), 151.2 (ArC), 150.1 (ArC), 145.6 (ArC), 139.5 (C-4), 137.0 (C-6′′), 130.8 (C-5), 130.2 (ArC), 124.2 (ArC), 118.6 (ArC), 113.8 (ArC), 113.4 (ArC), 110.6 (ArC), 109.3 (ArC), 106.2 (ArC), 88.2 (C-1′), 80.6 (CH2), 80.0 (C-4′), 72.9 (C-2′), 70.5 (C-3′), 63.6 (C-5′), 49.7 (CH2), 45.2 (CH2), 21.1 (OCOCH3), 20.9 (OCOCH3), 20.8 (OCOCH3), 20.6 (CH3); HRMS (ESI): m/z calculated for C36H35N6O12+ [M + H]+: 743.2307, found: 743.2295.
4.2.7
N
1-(9′′′-(4′′′′-Methoxy)-9′′′,10′′′-dihydro-2′′′H,8′′′H-chromeno[8′′′,7′′′-e][1′′′,3′′′]oxazin-2′′′-one-4′′′-methyl)-C4-(3′,5′-di-O-acetyl-β-D-ribofuranos-1′-(2′′,4′′-dioxo-3′′,4′′-dihydropyrimidin))-1,2,3-triazole (13a).
It was obtained as a yellow solid. Yield: 0.65 g (93%). Rf = 0.30 (5% methanol in chloroform); melting point = 226 °C; IR (KBr, cm−1): 3414, 2927, 1683, 1586, 1511, 1414, 1226, 1097, 1034, 825, 760, 647; 1H NMR (DMSO-d6, 400 MHz) δ 11.82 (brs, 1H, N-3′′H), 8.61–8.56 (m, 1H, C-5H), 8.46–8.43 (m, 1H, C-6′′H), 7.66–7.52 (m, 2H, ArH), 7.11–7.06 (m, 2H, ArH), 6.85–6.80 (m, 2H, ArH), 6.29–6.25 (m, 1H, C-1′H), 5.97 (s, 2H, CH2), 5.68–5.63 (m, 1H, ArH), 5.49–5.47 (m, 1H, C-3′H), 5.27–5.24 (m, 1H, C-4′H), 4.67 (s, 2H, CH2), 4.33–4.24 (m, 4H, CH2, ArH), 3.66 (s, 3H, CH3), 2.51–2.44 (m, 1H, C-2′H), 2.44–2.40 (m, 1H, C-2′H), 2.14 (s, 3H, OCOCH3), 2.09 (s, 3H, OCOCH3); 13C NMR (DMSO-d6,100 MHz) δ 170.9 (C
O), 170.6 (C
O), 161.6 (ArC), 160.0 (ArC), 158.1 (ArC), 154.9 (ArC), 151.5 (ArC), 151.3 (ArC), 150.1 (ArC), 141.6 (ArC), 139.7 (C-4), 136.1 (C-6′′), 124.2 (C-5), 124.0 (ArC), 120.4 (ArC), 115.4 (ArC), 115.0 (ArC), 113.8 (ArC), 110.6 (ArC), 109.2 (ArC), 105.9 (ArC), 85.5 (C-1′), 82.2 (C-4′), 81.8 (CH2), 74.7 (C-3′), 64.3 (C-5′), 55.7 (OCH3), 49.7 (CH2), 45.6 (CH2), 37.3 (C-2′), 21.3 (OCOCH3), 21.1 (OCOCH3); HRMS (ESI): m/z calculated for C34H33N6O11+ [M + H]+: 701.2202, found: 701.2187.
4.2.8
N
1-(9′′′-(4′′′′-Chloro)-9′′′,10′′′-dihydro-2′′′H,8′′′H-chromeno[8′′′,7′′′-e][1′′′,3′′′]oxazin-2′′′-one-4′′′-methyl)-C4-(3′,5′-di-O-acetyl-β-D-ribofuranos-1′-(2′′,4′′-dioxo-3′′,4′′-dihydropyrimidin))-1,2,3-triazole (13b).
It was obtained as a yellowish white solid. Yield: 0.65 g (92%). Rf = 0.33 (5% methanol in chloroform); melting point = 210 °C; IR (KBr, cm−1): 3350, 2933, 1611, 1571, 1441, 1360, 1232, 1108, 1049, 873, 754, 717; 1H NMR (DMSO-d6, 400 MHz) δ 11.80 (brs, 1H, N-3′′H), 8.61–8.55 (m, 1H, C-5H), 8.48–8.42 (m, 1H, C-6′′H), 7.68–7.62 (m, 1H, ArH), 7.42–7.36 (m, 2H, ArH), 7.17–7.11 (m, 2H, ArH), 6.89–6.83 (m, 1H, ArH), 6.31–6.23 (m, 1H, C-1′H), 6.00–5.95 (s, 2H, CH2), 5.71–5.66 (m, 1H, ArH), 5.55 (m, 2H, CH2), 5.27–5.22 (m, 1H, C-3′H), 4.75 (s, 2H, CH2), 4.32–4.28 (m, 1H, C-4′H), 4.28–4.23 (m, 2H, C-5′H), 2.46–2.37 (m, 2H, C-2′H), 2.13 (s, 3H, OCOCH3), 2.08 (s, 3H, OCOCH3); 13C NMR (DMSO-d6, 100 MHz) δ 170.9 (C
O), 170.6 (C
O), 161.6 (ArC), 160.0 (ArC), 157.9 (ArC), 151.5 (ArC), 151.3 (ArC), 150.1 (ArC), 147.2 (ArC), 139.7 (C-4), 136.1 (C-6′′), 132.4 (ArC), 124.3 (C-5), 124.0 (ArC), 120.5 (ArC), 113.9 (ArC), 113.4 (ArC), 110.6 (ArC), 109.2 (ArC), 105.9 (ArC), 85.5 (C-1′), 82.2 (C-4′), 79.8 (CH2), 74.7 (C-3′), 64.3 (C-5′), 49.7 (CH2), 45.0 (CH2), 37.3 (C-2′), 21.3 (OCOCH3), 21.1 (OCOCH3); HRMS (ESI): m/z calculated for C33H30ClN6O10+ [M + H]+: 705.1706, found: 705.1728.
4.2.9
N
1-(9′′′-(4′′′′-Fluoro)-9′′′,10′′′-dihydro-2′′′H,8′′′H-chromeno[8′′′,7′′′-e][1′′′,3′′′]oxazin-2′′′-one-4′′′-methyl)-C4-(3′,5′-di-O-acetyl-β-D-ribofuranos-1′-(2′′,4′′-dioxo-3′′,4′′-dihydropyrimidin))-1,2,3-triazole (13c).
It was obtained as a yellowish brown solid. Yield: 0.63 g (92%). Rf = 0.32 (5% methanol in chloroform); melting point = 194–196 °C; IR (KBr, cm−1): 3288, 1687, 1597, 1512, 1453, 1382, 1228, 1096, 1039, 817, 608, 552; 1H NMR (DMSO-d6, 400 MHz) δ 11.82 (brs, 1H, N-3′′H), 8.57–8.56 (m, 1H, C-5H), 8.44–8.43 (m, 1H, C-6′′H), 7.67–7.64 (m, 1H, ArH), 7.21–7.16 (m, 2H, ArH), 7.10–7.05 (m, 2H, ArH), 6.87–6.84 (m, 1H, ArH), 6.28–6.25 (m, 1H, C-1′H), 5.97 (s, 2H, CH2), 5.69–5.67 (m, 1H, ArH), 5.53 (m, 2H, CH2), 5.26–5.24 (m, 1H, C-3′H), 4.73 (s, 2H, CH2), 4.31–4.29 (m, 1H, C-4′H), 4.28–4.25 (m, 2H, C-5′H), 2.46–2.44 (m, 1H, C-2′H), 2.43–2.40 (m, 1H, C-2′H), 2.14 (s, 3H, OCOCH3), 2.09 (s, 3H, OCOCH3); 13C NMR (DMSO-d6, 100 MHz) δ 170.9 (C
O), 170.6 (C
O), 161.6 (ArC), 160.0 (ArC), 158.0 (ArC), 151.5 (ArC), 151.3 (ArC), 150.1 (ArC), 144.6 (ArC), 139.7 (C-4), 136.1 (C-6′′), 124.3 (C-5), 124.0 (ArC), 120.5 (ArC), 120.4 (ArC), 116.3 (ArC), 116.1 (ArC), 113.9 (ArC), 110.7 (ArC), 110.5 (ArC), 109.1 (ArC), 105.9 (ArC), 85.5 (C-1′), 82.2 (C-4′), 80.7 (CH2), 74.7 (C-3′), 64.3 (C-5′), 49.7 (CH2), 45.5 (CH2), 37.3 (C-2′), 21.3 (OCOCH3), 21.1 (OCOCH3); HRMS (ESI): m/z calculated for C33H30FN6O10+ [M + H]+: 689.2002, found: 689.2041.
4.2.10
N
1-(9′′′,10′′′-Dihydro-2′′′H,8′′′H-chromeno[8′′′,7′′′-e][1′′′,3′′′]oxazin-2′′′-one-4′′′-methyl)-C4-(3′,5′-di-O-acetyl-β-D-ribofuranos-1′-(2′′,4′′-dioxo-3′′,4′′-dihydropyrimidin))-1,2,3-triazole (13d).
It was obtained as a yellow solid. Yield: 0.63 g (94%). Rf = 0.34 (5% methanol in chloroform); melting point = 246 °C; IR (KBr, cm−1): 2889, 2106, 1720, 1585, 1495, 1359, 1302, 1251, 1162, 1097, 914, 797, 713; 1H NMR (DMSO-d6, 400 MHz) δ 11.81 (brs, 1H, N-3′′H), 8.57–8.55 (m, 1H, C-5H), 8.44–8.43 (m, 1H, C-6′′H), 7.66–7.62 (m, 1H, ArH), 7.27–7.22 (m, 2H, ArH), 7.17–7.14 (m, 2H, ArH), 6.92–6.89 (m, 1H, ArH), 6.86–6.82 (m, 1H, ArH), 6.28–6.24 (m, 1H, C-1′H), 5.96 (s, 2H, CH2), 5.67–5.64 (m, 1H, ArH), 5.57 (m, 2H, CH2), 5.26–5.23 (m, 1H, C-3′H), 4.76 (s, 2H, CH2), 4.30–4.29 (m, 1H, C-4′H), 4.27–4.25 (m, 2H, C-5′H), 2.46–2.43 (m, 1H, C-2′H), 2.43–2.41 (m, 1H, C-2′H), 2.13 (s, 3H, OCOCH3), 2.08 (s, 3H, OCOCH3); 13C NMR (DMSO-d6, 100 MHz) δ 170.9 (C
O), 170.6 (C
O), 161.6 (ArC), 160.0 (ArC), 158.0 (ArC), 151.5 (ArC), 151.3 (ArC), 150.1 (ArC), 144.6 (ArC), 139.7 (C-4), 136.1 (C-6′′), 124.3 (C-5), 124.0 (ArC), 120.5 (ArC), 120.4 (ArC), 116.3 (ArC), 116.1 (ArC), 113.9 (ArC), 110.7 (ArC), 110.5 (ArC), 109.1 (ArC), 105.9 (ArC), 85.5 (C-1′), 82.2 (C-4′), 80.7 (CH2), 74.7 (C-3′), 64.3 (C-5′), 49.7 (CH2), 45.5 (CH2), 37.3 (C-2′), 21.3 (OCOCH3), 21.1 (OCOCH3); HRMS (ESI): m/z calculated for C33H31N6O10+ [M + H]+: 671.2096, found: 671.2111.
4.2.11
N
1-(9′′′-(4′′′′-Bromo)-9′′′,10′′′-dihydro-2′′′H,8′′′H-chromeno[8′′′,7′′′-e][1′′′,3′′′]oxazin-2′′′-one-4′′′-methyl)-C4-(3′,5′-di-O-acetyl-β-D-ribofuranos-1′-(2′′,4′′-dioxo-3′′,4′′-dihydropyrimidin))-1,2,3-triazole (13e).
It was obtained as a yellowish brown solid. Yield: 0.69 g (93%). Rf = 0.33 (5% methanol in chloroform); melting point = 249 °C; IR (KBr, cm−1): 3054, 2811, 1707, 1687, 1599, 1492, 1228, 1052, 817, 602, 554; 1H NMR (DMSO-d6, 400 MHz) δ 11.76 (brs, 1H, N-3′′H), 8.57–8.50 (m, 1H, C-5H), 8.45–8.38 (m, 1H, C-6′′H), 7.64–7.59 (m, 1H, ArH), 7.38–7.32 (m, 2H, ArH), 7.13–7.07 (m, 2H, ArH), 6.85–6.79 (m, 1H, ArH), 6.28–6.19 (m, 1H, C-1′H), 5.98–5.90 (m, 2H, CH2), 5.69–5.63 (m, 1H, ArH), 5.52 (m, 2H, CH2), 5.24–5.18 (m, 1H, C-3′H), 4.71 (s, 2H, CH2), 4.28–4.24 (m, 1H, C-4′H), 4.23–4.19 (m, 2H, C-5′H), 2.43–2.40 (m, 1H, C-2′H), 2.39–2.36 (m, 1H, C-2′H), 2.10 (s, 3H, OCOCH3), 2.04 (s, 3H, OCOCH3); 13C NMR (DMSO-d6, 100 MHz) δ 170.9 (C
O), 170.6 (C
O), 161.6 (ArC), 160.0 (ArC), 157.9 (ArC), 151.5 (ArC), 151.3 (ArC), 150.1 (ArC), 147.2 (ArC), 139.7 (C-4), 136.1 (C-6′′), 132.4 (ArC), 124.3 (C-5), 124.0 (ArC), 120.5 (ArC), 113.9 (ArC), 110.8 (ArC), 110.6 (ArC), 109.2 (ArC), 105.9 (ArC), 85.5 (C-1′), 82.2 (C-4′), 79.8 (CH2), 74.7 (C-3′), 64.3 (C-5′), 49.7 (CH2), 45.0 (CH2), 37.3 (C-2′), 21.3 (OCOCH3), 21.1 (OCOCH3); HRMS (ESI): m/z calculated for C33H30BrN6O10+ [M + H]+: 749.1201, found: 749.1179.
4.2.12
N
1-(9′′′-(4′′′′-Methyl)-9′′′,10′′′-dihydro-2′′′H,8′′′H-chromeno[8′′′,7′′′-e][1′′′,3′′′]oxazin-2′′′-one-4′′′-methyl)-C4-(3′,5′-di-O-acetyl-β-D-ribofuranos-1′-(2′′,4′′-dioxo-3′′,4′′-dihydropyrimidin))-1,2,3-triazole (13f).
It was obtained as a yellow solid. Yield: 0.63 g (92%). Rf = 0.35 (5% methanol in chloroform); melting point = 217 °C; IR (KBr, cm−1): 1689, 1597, 1509, 1444, 1361, 1226, 1103, 1045, 814; 1H NMR (DMSO-d6, 400 MHz) δ 11.81 (brs, 1H, N-3′′H), 8.59–8.55 (m, 1H, C-5H), 8.46–8.42 (m, 1H, C-6′′H), 7.66–7.62 (m, 1H, ArH), 7.07–7.02 (m, 4H, ArH), 6.85–6.82 (m, 1H, ArH), 6.29–6.24 (m, 1H, C-1′H), 5.97 (m, 2H, CH2), 5.67–5.61 (m, 1H, ArH), 5.53 (s, 2H, CH2), 5.27–5.23 (m, 1H, C-3′H), 4.71 (s, 2H, CH2), 4.33–4.29 (m, 1H, C-4′H), 4.27–4.25 (m, 2H, C-5′H), 2.48–2.39 (m, 2H, C-2′H), 2.18 (s, 3H, CH3), 2.15 (s, 3H, OCOCH3), 2.09 (s, 3H, OCOCH3); 13C NMR (DMSO-d6, 100 MHz) δ 170.9 (C
O), 170.6 (C
O), 161.6 (ArC), 160.0 (ArC), 158.1 (ArC), 151.5 (ArC), 151.3 (ArC), 150.1 (ArC), 145.6 (ArC), 139.7 (C-4), 136.1 (C-6′′), 130.2 (ArC), 124.2 (C-5), 124.0 (ArC), 118.6 (ArC), 113.8 (ArC), 113.0 (ArC), 110.6 (ArC), 110.4 (ArC), 109.3 (ArC), 105.9 (ArC), 85.5 (C-1′), 82.2 (C-4′), 80.5 (CH2), 74.7 (C-3′), 64.3 (C-5′), 49.7 (CH2), 45.2 (CH2), 37.3 (C-2′), 21.3 (OCOCH3), 21.1 (OCOCH3), 20.6 (CH3); HRMS (ESI): m/z calculated for C34H33N6O10+ [M + H]+: 685.2253, found: 685.2280.
4.3. General procedure for the synthesis of N1-(9′′′-(4′′′′-substituted)-9′′′,10′′′-dihydro-2′′′H,8′′′H-chromeno[8′′′,7′′′-e][1′′′,3′′′]oxazin-2′′′-one-4′′′-methyl)-C4-(β-D-ribofuranos-/2′-deoxy-β-D-ribofuranos-1′-(2′′,4′′-dioxo-3′′,4′′-dihydropyrimidin))-1,2,3-triazole conjugates (14a–f and 15a–f)
To a solution of N1-(9′′′-(4′′′′-substituted)-9′′′,10′′′-dihydro-2′′′H,8′′′H-chromeno[8′′′,7′′′-e][1′′′,3′′′]oxazin-2′′′-one-4′′′-methyl)-C4-(2′,3′,5′-tri-O-acetyl/3′,5′-di-O-acetyl-β-D-ribofuranos-1′-(2′′,4′′-dioxo-3′′,4′′-dihydropyrimidin))-1,2,3-triazole conjugates (12a–f/13a–f, 1.0 mmol) in methanol, NaOMe (2.2 mmol) was added. The reaction mixture was allowed to stir at 25 °C for 10–15 minutes. After the completion of the reaction (as monitored by TLC), the reaction was neutralized with seralite (H+) resin. The solution was filtered through a cotton plug and the solvent was evaporated under vacuum. The crude product thus obtained was purified by silica gel column chromatography using methanol in chloroform as the solvent system to afford the desired products 14a–f and 15a–f in quantitative yields.
4.3.1
N
1-(9′′′-(4′′′′-Methoxy)-9′′′,10′′′-dihydro-2′′′H,8′′′H-chromeno[8′′′,7′′′-e][1′′′,3′′′]oxazin-2′′′-one-4′′′-methyl)-C4-(β-D-ribofuranos-1′-(2′′,4′′-dioxo-3′′,4′′-dihydropyrimidin))-1,2,3-triazole (14a).
It was obtained as a yellow solid. Yield: 0.61 g (97%). Rf = 0.24 (10% methanol in chloroform); melting point = 258 °C; IR (KBr, cm−1): 3393, 1683, 1586, 1500, 1495, 1452, 1377, 1232, 1039, 818; 1H NMR (DMSO-d6,400 MHz) δ 11.75 (brs, 1H, N-3′′H), 9.25–9.21 (m, 1H, C-6′′H), 8.69–8.66 (m, 1H, C-5H), 8.60–8.55 (m, 1H, ArH), 7.94–7.87 (m, 1H, ArH), 7.57–7.52 (m, 2H, ArH), 7.08–7.03 (m, 2H, ArH), 6.99–6.95 (m, 1H, ArH), 6.04–6.00 (m, 1H, C-1′H), 5.93–5.88 (m, 2H, CH2), 5.70 (brs, 1H, OH), 5.44 (brs, 1H, OH), 5.19–5.14 (m, 2H, CH2), 4.18–4.14 (m, 1H, C-2′H), 4.04–4.00 (m, 1H, C-3′H), 3.93–3.89 (m, 1H, C-4′H), 3.81 (s, 3H, CH3), 3.66–3.61 (m, 2H, C-5′H), 3.51 (m, 2H, CH2); 13C NMR (DMSO-d6, 100 MHz) δ 166.9 (ArC), 161.6 (ArC), 159.6 (ArC), 159.5 (ArC), 154.7 (ArC), 151.6 (ArC), 150.5 (ArC), 139.7 (C-4), 137.1 (C-6′′), 129.9 (ArC), 124.3 (C-5), 124.0 (ArC) 123.2 (ArC), 115.3 (ArC) 109.6 (ArC), 108.6 (ArC), 106.8 (ArC), 105.6 (ArC), 88.4 (C-1′), 85.6 (C-4′), 74.3 (CH2), 70.7 (C-3′), 70.2 (C-2′), 61.5 (C-5′), 55.9 (CH3), 49.7 (CH2), 45.1 (CH2); HRMS (ESI): m/z calculated for C30H29N6O10+ [M + H]+: 633.1940, found: 633.1944.
4.3.2
N
1-(9′′′-(4′′′′-Chloro)-9′′′,10′′′-dihydro-2′′′H,8′′′H-chromeno[8′′′,7′′′-e][1′′′,3′′′]oxazin-2′′′-one-4′′′-methyl)-C4-(β-D-ribofuranos-1′-(2′′,4′′-dioxo-3′′,4′′-dihydropyrimidin))-1,2,3-triazole (14b).
It was obtained as a yellow solid. Yield: 0.62 g (98%). Rf = 0.26 (10% methanol in chloroform); melting point = 262–264 °C; IR (KBr, cm−1): 3307, 3153, 2928, 1686, 1591, 1495, 1459, 1400, 1317, 1257, 1044, 818; 1H NMR (DMSO-d6, 400 MHz) δ 11.75 (brs, 1H, N-3′′H), 8.69–8.67 (m, 1H, C-6′′H), 8.58–8.55 (m, 1H, C-5H), 7.73–7.66 (m, 1H, ArH), 7.30–7.26 (m, 1H, ArH), 7.21–7.18 (m, 1H, ArH), 7.10–7.05 (m, 1H, ArH), 6.96–6.85 (m, 1H, ArH), 6.75–6.65 (m, 1H, ArH), 5.98–5.94 (m, 2H, CH2), 5.93–5.90 (m, 1H, C-1′H), 5.74–5.69 (m, 1H, ArH), 5.62–5.48 (m, 2H, CH2), 5.48–5.40 (brs, 1H, OH), 5.15 (brs, 2H, OH), 4.84–4.68 (m, 2H, CH2), 4.18–4.14 (m, 1H, C-2′H), 4.03–4.01 (m, 1H, C-3′H), 3.93–3.91 (m, 1H, C-4′H), 3.66–3.59 (m, 2H, C-5′H); 13C NMR (DMSO-d6, 100 MHz) δ 161.6 (ArC), 159.9 (ArC), 157.8 (ArC), 154.1 (ArC), 151.5 (ArC), 150.5 (ArC), 148.2 (ArC), 146.8 (ArC), 139.7 (C-4), 137.1 (C-6′′), 129.5 (ArC), 128.9 (ArC), 125.5 (ArC), 124.0 (C-5), 120.0 (ArC), 113.8 (ArC), 112.9 (ArC), 110.8 (ArC), 109.1 (ArC), 105.6 (ArC), 88.4 (C-1′), 85.6 (C-4′), 79.9 (CH2), 74.3 (C-2′), 70.7 (C-3′), 61.5 (C-5′), 49.6 (CH2), 45.1 (CH2); HRMS (ESI): m/z calculated for C29H26ClN6O9+ [M + H]+: 637.1444, found: 637.1425.
4.3.3
N
1-(9′′′-(4′′′′-Fluoro)-9′′′,10′′′-dihydro-2′′′H,8′′′H-chromeno[8′′′,7′′′-e][1′′′,3′′′]oxazin-2′′′-one-4′′′-methyl)-C4-(β-D-ribofuranos-1′-(2′′,4′′-dioxo-3′′,4′′-dihydropyrimidin))-1,2,3-triazole (14c).
It was obtained as a yellow solid. Yield: 0.60 g (97%). Rf = 0.26 (10% methanol in chloroform); melting point = 241–243 °C; IR (KBr, cm−1): 3314, 2718, 1684, 1596, 1512, 1455, 1396, 1338, 1269, 1211, 1103, 1054, 830, 644, 517; 1H NMR (DMSO-d6, 400 MHz) δ 11.74 (brs, 1H, N-3′′H), 8.69–8.66 (m, 1H, C-6′′H), 8.58–8.55 (m, 1H, C-5H), 7.72–7.67 (m, 1H, ArH), 7.22–7.05 (m, 2H, ArH), 7.02–6.98 (m, 1H, ArH), 6.94–6.88 (m, 2H, ArH), 6.71–6.66 (m, 1H, ArH), 5.99–5.94 (m, 2H, CH2), 5.93–5.89 (m, 1H, C-1′H), 5.73–5.66 (m, 1H, CH2), 5.54–5.46 (m, 1H, CH2), 5.16 (brs, 2H, OH), 4.25 (brs, 1H, OH), 4.18–4.14 (m, 1H, C-2′H), 4.04–4.01 (m, 1H, C-3′H), 3.93–3.90 (m, 1H, C-4′H), 3.67–3.58 (m, 4H, C-5′H, CH2); 13C NMR (DMSO-d6,100 MHz) δ 161.6 (ArC), 160.7 (ArC), 160.4 (ArC), 154.0 (ArC), 153.7 (ArC), 151.4 (ArC), 150.5 (ArC), 146.0 (ArC), 139.7 (C-4), 137.1 (C-6′′), 125.1 (ArC), 124.0 (C-5), 120.4 (ArC), 120.4 (ArC), 116.3 (ArC), 116.0 (ArC), 115.7 (ArC), 115.5 (ArC), 113.4 (ArC), 113.3 (ArC), 113.1 (ArC), 113.0 (ArC), 109.8 (ArC), 109.6 (ArC), 105.6 (ArC), 88.5 (C-1′), 85.6 (C-4′), 74.3 (CH2), 70.7 (C-3′, C-2′), 61.5 (C-5′), 49.7 (CH2), 36.4 (CH2); HRMS (ESI): m/z calculated for C29H26FN6O9+ [M + H]+: 621.1740, found: 621.1716.
4.3.4
N
1-(9′′′,10′′′-Dihydro-2′′′H,8′′′H-chromeno[8′′′,7′′′-e][1′′′,3′′′]oxazin-2′′′-one-4′′′-methyl)-C4-(β-D-ribofuranos-1′-(2′′,4′′-dioxo-3′′,4′′-dihydropyrimidin))-1,2,3-triazole (14d).
It was obtained as a yellow solid. Yield: 0.58 g (96%). Rf = 0.27 (10% methanol in chloroform); melting point = 216–220 °C; IR (KBr, cm−1): 1686, 1591, 1511, 1451, 1359, 1226, 1104, 1045, 807; 1H NMR (DMSO-d6, 400 MHz) δ 11.74 (brs, 1H, N-3′′H), 8.69–8.65 (m, 1H, C-6′′H), 8.57–8.53 (m, 1H, C-5H), 7.70–7.64 (m, 1H, ArH), 7.29–7.19 (m, 2H, ArH), 7.18–7.14 (m, 1H, ArH), 7.09–7.00 (m, 1H, ArH), 6.96–6.84 (m, 2H, ArH), 6.70–6.50 (m, 1H, ArH), 5.96 (m, 2H, CH2), 5.92–5.88 (m, 1H, C-1′H), 5.72–5.66 (m, 1H, CH2), 5.59–5.56 (m, 1H, CH2), 5.14 (brs, 1H, OH), 4.76 (brs, 1H, OH), 4.28 (brs, 1H, OH), 4.17–4.13 (m, 1H, C-2′H), 4.03–3.99 (m, 1H, C-3′H), 3.93–3.89 (m, 1H, C-4′H), 3.69–3.54 (m, 4H, C-5′H, CH2); 13C NMR (DMSO-d6, 100 MHz) δ 161.6 (ArC), 160.0 (ArC), 158.0 (ArC), 151.5 (ArC), 151.2 (ArC), 150.5 (ArC), 149.3 (ArC), 147.9 (ArC), 139.7 (C-4), 137.1 (C-6′′), 129.7 (ArC), 129.3 (ArC), 124.0 (C-5), 121.8 (ArC), 118.4 (ArC), 113.8 (ArC), 112.6 (ArC), 110.7 (ArC), 109.3 (ArC), 105.6 (ArC), 88.4 (C-1′), 85.6 (C-4′), 80.1 (CH2), 74.3 (C-3′), 70.7 (C-2′), 61.5 (C-5′), 49.6 (CH2), 45.0 (CH2); HRMS (ESI): m/z calculated for C29H27N6O9+ [M + H]+: 603.1834, found: 603.1859.
4.3.5
N
1-(9′′′-(4′′′′-Bromo)-9′′′,10′′′-dihydro-2′′′H,8′′′H-chromeno[8′′′,7′′′-e][1′′′,3′′′]oxazin-2′′′-one-4′′′-methyl)-C4-(β-D-ribofuranos-1′-(2′′,4′′-dioxo-3′′,4′′-dihydropyrimidin))-1,2,3-triazole (14e).
It was obtained as a yellow solid. Yield: 0.67 g (98%). Rf = 0.28 (10% methanol in chloroform); melting point = 254 °C; IR (KBr, cm−1): 3324, 2741, 1644, 1531, 1415, 1367, 1332, 1258, 1123, 1035, 826, 643, 509; 1H NMR (DMSO-d6, 400 MHz) δ 11.74 (brs, 1H, N-3′′H), 8.67–8.66 (m, 1H, C-6′′H), 8.56–8.54 (m, 1H, C-5H), 7.70–7.66 (m, 1H, ArH), 7.40–7.38 (m, 1H, ArH), 7.19–7.17 (m, 1H, ArH), 7.15–7.13 (m, 1H, ArH), 6.93–6.85 (m, 1H, ArH), 6.66–6.64 (m, 1H, ArH), 5.96–5.89 (m, 4H, CH2, C-1′H, ArH), 5.72–5.66 (m, 1H, CH2), 5.56–5.45 (m, 1H, CH2), 5.13 (brs, 1H, OH), 4.75 (brs, 1H, OH), 4.24 (brs, 1H, OH), 4.16–4.13 (m, 1H, C-2′H), 4.01–3.99 (m, 1H, C-3′H), 3.91–3.89 (m, 1H, C-4′H), 3.66–3.56 (m, 4H, C-5′H, CH2); 13C NMR (DMSO-d6, 100 MHz) δ 161.6 (ArC), 160.4 (ArC), 154.1 (ArC), 150.5 (ArC), 148.6 (ArC), 147.2 (ArC), 139.7 (C-4), 137.1 (C-6′′), 132.4 (ArC), 131.7 (ArC), 124.0 (C-5), 114.5 (ArC), 110.8 (ArC), 109.1 (ArC), 106.7 (ArC), 105.6 (ArC), 88.4 (C-1′), 85.6 (C-4′), 74.3 (CH2), 70.7 (C-2′, C-3′), 61.5 (C-5′), 60.2 (CH2), 49.6 (CH2); HRMS (ESI): m/z calculated for C29H26BrN6O9+ [M + H]+: 681.0939, found: 681.1012.
4.3.6
N
1-(9′′′-(4′′′′-Methyl)-9′′′,10′′′-dihydro-2′′′H,8′′′H-chromeno[8′′′,7′′′-e][1′′′,3′′′]oxazin-2′′′-one-4′′′-methyl)-C4-(β-D-ribofuranos-1′-(2′′,4′′-dioxo-3′′,4′′-dihydropyrimidin))-1,2,3-triazole (14f).
It was obtained as a yellow solid. Yield: 0.60 g (98%). Rf = 0.29 (10% methanol in chloroform); melting point = 235–237 °C; IR (KBr, cm−1): 3309, 2722, 1676, 1545, 1501, 1452, 1337, 1242, 1112, 1067, 805, 663, 503;1H NMR (DMSO-d6, 400 MHz) δ 11.71 (brs, 1H, N-3′′H), 8.67–8.64 (m, 1H, C-6′′H), 8.56–8.53 (m, 1H, C-5H), 7.68–7.63 (m, 1H, ArH), 7.40–7.33 (m, 1H, ArH), 7.04 (brs, 2H, ArH), 6.94–6.82 (m, 2H, ArH), 5.97–5.93 (m, 2H, CH2), 5.91–5.88 (m, 1H, C-1′H), 5.73–5.70 (m, 1H, ArH), 5.56–5.50 (m, 2H, CH2), 5.14 (brs, 2H, OH), 4.71 (brs, 1H, OH), 4.18–4.13 (m, 1H, C-2′H), 4.02–3.99 (m, 1H, C-3′H), 3.92–3.89 (m, 1H, C-4′H), 3.68–3.63 (m, 2H, C-5′H), 3.61–3.56 (m, 2H, CH2), 2.18 (s, 3H, CH3); 13C NMR (DMSO-d6, 100 MHz) δ 161.6 (ArC), 160.0 (ArC), 158.0 (ArC), 154.0 (ArC), 151.5 (ArC), 150.5 (ArC), 147.0 (ArC), 145.5 (ArC), 139.7 (C-4), 137.1 (C-6′′), 130.1 (ArC), 129.7 (ArC), 124.0 (C-5), 118.6 (ArC), 112.9 (ArC), 109.9 (ArC), 109.6 (ArC), 109.2 (ArC), 105.6 (ArC), 88.4 (C-1′), 85.6 (C-4′), 74.3 (CH2), 70.7 (C-2′, C-3′), 61.5 (C-5′), 49.7 (CH2), 45.1 (CH2), 20.5 (CH3); HRMS (ESI): m/z calculated for C30H29N6O9+ [M + H]+: 617.1991, found: 617.1997.
4.3.7
N
1-(9′′′-(4′′′′-Methyl)-9′′′,10′′′-dihydro-2′′′H,8′′′H-chromeno[8′′′,7′′′-e][1′′′,3′′′]oxazin-2′′′-one-4′′′-methyl)-C4-(2′-deoxy-β-D-ribofuranos-1′-(2′′,4′′-dioxo-3′′,4′′-dihydropyrimidin))-1,2,3-triazole (15a).
It was obtained as a yellow solid. Yield: 0.59 g (96%). Rf = 0.25 (10% methanol in chloroform); melting point = 266 °C; IR (KBr, cm−1): 3275, 3071, 1672, 1586, 1489, 1446, 1254, 1174, 1040, 818; 1H NMR (DMSO-d6, 400 MHz) δ 11.72 (brs, 1H, N-3′H), 8.66–8.60 (m, 1H, C-6′′H), 8.60–8.52 (m, 1H, C-5H), 7.66–7.54 (m, 1H, ArH), 7.12–6.62 (m, 5H, ArH), 6.28–6.21 (m, 1H, C-1′H), 6.03–5.89 (m, 2H, CH2), 5.75–5.43 (m, 2H, CH2), 5.32 (brs, 1H, OH), 5.10–5.02 (m, 1H, ArH), 4.67–4.50 (m, 1H, C-3′H), 4.32–4.25 (m, 1H, C-4′H), 3.90–3.78 (m, 2H, CH2), 3.70–3.55 (m, 5H, C-5′H, CH3), 2.23–2.16 (m, 2H,C-2′H); 13C NMR (DMSO-d6, 100 MHz) δ 161.6 (ArC), 160.0 (ArC), 159.6 (ArC), 158.0 (ArC), 154.8 (ArC), 151.5 (ArC), 150.1 (ArC), 141.6 (ArC), 139.8 (C-4), 136.8 (C-6′′), 123.2 (ArC), 120.3 (ArC), 115.3 (ArC), 114.9 (ArC), 113.8 (ArC), 110.6 (C-5), 109.1 (ArC), 105.4 (ArC), 88.1 (C-4′), 85.2 (C-1′), 55.9 (CH2), 71.1 (C-3′), 61.8 (C-5′), 55.6 (CH3), 55.6 (CH2), 49.6 (CH2), 45.6 (C-2′), 40.5(CH2); HRMS (ESI): m/z calculated for C30H29N6O9+ [M + H]+: 617.1991, found: 617.1981.
4.3.8
N
1-(9′′′-(4′′′′-Chloro)-9′′′,10′′′-dihydro-2′′′H,8′′′H-chromeno[8′′′,7′′′-e][1′′′,3′′′]oxazin-2′′′-one-4′′′-methyl)-C4-(2′-deoxy-β-D-ribofuranos-1′-(2′′,4′′-dioxo-3′′,4′′-dihydropyrimidin))-1,2,3-triazole (15b).
It was obtained as a yellow solid. Yield: 0.61 g (99%). Rf = 0.26 (10% methanol in chloroform); melting point = 259–260 °C; IR (KBr, cm−1): 3186, 3004, 1659, 1581, 1440, 1387, 1257, 1048, 834; 1H NMR (DMSO-d6, 400 MHz) δ 8.62–8.61 (m, 1H, C-6′′H), 8.54–8.53 (m, 1H, C-5H), 7.67–7.61 (m, 1H, ArH), 7.40–7.38 (m, 1H, ArH), 7.17–7.15 (m, 1H, ArH), 7.14–7.12 (m, 1H, ArH), 6.88–6.84 (m, 1H, ArH), 6.67–6.64 (m, 1H, ArH), 6.25–6.22 (m, 1H, C-1′H), 5.96–5.94 (m, 1H, CH2), 5.92–5.90 (m, 1H, CH2), 5.56–5.53 (m, 2H, CH2), 4.75–4.74 (m, 1H, ArH), 4.29–4.22 (m, 3H, C-3′H, CH2), 3.86–3.84 (m, 1H, C-4′H), 3.60–3.59 (m, 2H, C-5′H), 2.20–2.17 (m, 2H, C-2′H); 13C NMR (DMSO-d6, 100 MHz) δ 161.2 (ArC), 160.2 (ArC), 159.5 (ArC), 157.4 (ArC), 151.0 (ArC), 150.7 (ArC), 149.7 (ArC), 146.7 (ArC), 139.4 (C-4), 136.4 (C-6′′), 131.9 (ArC), 131.2 (ArC), 123.4 (ArC), 120.0 (ArC), 114.1 (ArC), 112.9 (ArC), 110.3 (ArC), 108.7 (C-5), 106.2 (ArC), 105.0 (ArC), 87.7 (C-4′), 84.8 (C-1′), 79.3 (CH2), 70.7 (C-3′), 61.4 (C-5′), 49.1 (CH2), 44.5 (C-2′), 35.6 (CH2); HRMS (ESI): m/z calculated for C29H26ClN6O8+ [M + H]+: 621.1495, found: 621.1491.
4.3.9
N
1-(9′′′-(4′′′′-Fluoro)-9′′′,10′′′-dihydro-2′′′H,8′′′H-chromeno[8′′′,7′′′-e][1′′′,3′′′]oxazin-2′′′-one-4′′′-methyl)-C4-(2′-deoxy-β-D-ribofuranos-1′-(2′′,4′′-dioxo-3′′,4′′-dihydropyrimidin))-1,2,3-triazole (15c).
It was obtained as a yellow solid. Yield: 0.59 g (98%). Rf = 0.26 (10% methanol in chloroform); melting point = 208–210 °C; IR (KBr, cm−1): 3363, 3280, 2924, 1678, 1583, 1512, 1453, 1405, 1275, 1215, 1097, 1037, 824;1H NMR (DMSO-d6, 400 MHz) δ 11.71 (brs, 1H, NH), 8.63–8.62 (m, 1H, C-6′′H), 8.56–8.54 (m, 1H, C-5H), 7.70–7.66 (m, 1H, ArH), 7.19–7.16 (m, 1H, ArH), 7.01–6.97 (m, 1H, ArH), 6.93–6.87 (m, 2H, ArH), 6.69–6.67 (m, 1H, ArH), 6.26–6.23 (m, 1H, C-1′H), 5.97–5.94 (m, 2H, CH2), 5.72–5.66 (m, 1H, CH2), 5.57–5.48 (m, 1H, CH2), 5.34 (brs, 1H, OH), 5.07 (brs, 1H, OH), 4.76–4.69 (m, 1H, ArH), 4.30–4.28 (m, 1H, C-3′H), 4.26–4.22 (m, 2H, CH2), 3.87–3.85 (m, 1H, C-4′H), 3.62–3.59 (m, 2H, C-5′H), 2.21–2.18 (m, 2H, C-2′H); 13C NMR (DMSO-d6, 100 MHz) δ 161.7 (ArC), 160.8 (ArC), 160.4 (ArC), 155.9 (ArC), 154.0 (ArC), 151.4 (ArC), 150.1 (ArC), 146.0 (ArC), 139.8 (C-4), 136.8 (C-6′′), 125.1 (ArC), 123.9 (ArC), 120.5 (ArC), 120.4 (ArC), 116.3 (ArC), 116.0 (ArC), 115.7 (ArC), 115.5 (ArC), 113.4 (ArC), 113.3 (ArC), 113.1 (ArC), 109.7 (C-5), 109.5 (ArC), 105.4 (ArC), 88.1 (C-4′), 85.2 (C-1′), 80.6 (CH2), 71.1 (C-3′), 61.8 (C-5′), 49.7 (CH2), 45.5 (C-2′), 36.4 (CH2); HRMS (ESI): m/z calculated for C29H26FN6O8+ [M + H]+: 605.1718, found: 605.1787.
4.3.10
N
1-(9′′′,10′′′-Dihydro-2′′′H,8′′′H-chromeno[8′′′,7′′′-e][1′′′,3′′′]oxazin-2′′′-one-4′′′-methyl)-C4-(2′-deoxy-β-D-ribofuranos-1′-(2′′,4′′-dioxo-3′′,4′′-dihydropyrimidin))-1,2,3-triazole (15d).
It was obtained as a yellow solid. Yield: 0.57 g (98%). Rf = 0.27 (10% methanol in chloroform); melting point = 242 °C; IR (KBr, cm−1): 3276, 2946, 1648, 1565, 1417, 1405, 1266, 1043, 811, 524; 1H NMR (DMSO-d6, 400 MHz) δ 8.64–8.62 (m, 1H, C-6′′H), 8.56–8.55 (m, 1H, C-5H), 7.71–7.65 (m, 1H, ArH), 7.27–7.16 (m, 2H, ArH), 7.07–7.00 (m, 2H, ArH), 6.91–6.85 (m, 1H, ArH), 6.72–6.68 (m, 1H, ArH), 6.56–6.48 (m, 1H, C-1′H), 6.26–6.23 (m, 1H, CH2), 5.97–5.93 (m, 2H, CH2), 5.59–5.56 (m, 1H, CH2), 4.80–4.73 (m, 1H, ArH), 4.32–4.23 (m, 3H, C-3′H, CH2), 3.88–3.85 (m, 1H, C-4′H), 3.62–3.60 (m, 2H, C-5′H), 2.22–2.18 (m, 2H, C-2′H); 13C NMR (DMSO-d6, 100 MHz) δ 161.7 (ArC), 160.7 (ArC), 160.0 (ArC), 158.0 (ArC), 155.7 (ArC), 154.2 (ArC), 150.1 (ArC), 149.4 (ArC), 139.8 (C-4), 136.8 (C-6′′), 129.7 (ArC), 129.2 (ArC), 123.9 (ArC), 118.4 (ArC), 116.1 (ArC), 113.1 (C-5), 113.2 (ArC), 112.7 (ArC), 105.4 (ArC), 88.1 (C-4′), 85.2 (C-1′), 80.1 (CH2), 71.1 (C-3′), 61.8 (C-5′), 49.1 (C-2′, CH2), 36.0 (CH2); HRMS (ESI): m/z calculated for C29H27N6O8+ [M + H]+: 587.1885, found: 587.1869.
4.3.11
N
1-(9′′′-(4′′′′-Bromo)-9′′′,10′′′-dihydro-2′′′H,8′′′H-chromeno[8′′′,7′′′-e][1′′′,3′′′]oxazin-2′′′-one-4′′′-methyl)-C4-(2′-deoxy-β-D-ribofuranos-1′-(2′′,4′′-dioxo-3′′,4′′-dihydropyrimidin))-1,2,3-triazole (15e).
It was obtained as a yellow solid. Yield: 0.64 g (97%). Rf = 0.27 (10% methanol in chloroform); melting point = 229 °C; IR (KBr, cm−1): 3325, 3224, 2814, 1708, 1683, 1658, 1583, 1557, 1516, 1457, 1306, 1222, 1047, 820, 644, 517; 1H NMR (DMSO-d6, 400 MHz) δ 8.63–8.62 (m, 1H, C-6′′H), 8.56–8.54 (m, 1H, C-5H), 7.69–7.61 (m, 1H, ArH), 7.42–7.38 (m, 1H, ArH), 7.19–7.15 (m, 1H, ArH), 7.15–7.13 (m, 1H, ArH), 6.89–6.85 (m, 1H, ArH), 6.68–6.65 (m, 1H, ArH), 6.26–6.23 (m, 1H, C-1′H), 5.97–5.95 (m, 1H, CH2), 5.94–5.91 (m, 1H, CH2), 5.59–5.52 (m, 2H, CH2), 4.76–4.74 (m, 1H, ArH), 4.30–4.21 (m, 3H, C-3′H, CH2), 3.87–3.85 (m, 1H, C-4′H), 3.62–3.59 (m, 2H, C-5′H), 2.21–2.18 (m, 2H, C-2′H); 13C NMR (DMSO-d6, 100 MHz) δ 161.7 (ArC), 160.7 (ArC), 159.9 (ArC), 157.8 (ArC), 151.5 (ArC), 151.2 (ArC), 150.1 (ArC), 147.2 (ArC), 139.8 (C-4), 136.8 (C-6′′), 132.3 (ArC), 131.7 (ArC), 123.9 (ArC), 120.4 (ArC), 114.5 (ArC), 113.3 (ArC), 110.8 (ArC), 109.1 (C-5), 106.6 (ArC), 105.4 (ArC), 88.1 (C-4′), 85.2 (C-1′), 79.7 (CH2), 71.1 (C-3′), 61.8 (C-5′), 49.5 (CH2), 45.0 (CH2), 40.5 (C-2′), 36.0 (CH2); HRMS (ESI): m/z calculated for C29H26BrN6O8+ [M + H]+: 665.0990, found: 665.1005.
4.3.12
N
1-(9′′′-(4′′′′-Methyl)-9′′′,10′′′-dihydro-2′′′H,8′′′H-chromeno[8′′′,7′′′-e][1′′′,3′′′]oxazin-2′′′-one-4′′′-methyl)-C4-(2′-deoxy-β-D-ribofuranos-1′-(2′′,4′′-dioxo-3′′,4′′-dihydropyrimidin))-1,2,3-triazole (15f).
It was obtained as a yellow solid. Yield: 0.58 g (97%). Rf = 0.28 (10% methanol in chloroform); melting point = 202 °C; IR (KBr, cm−1): 3296, 2932, 1672, 1589, 1506, 1446, 1399, 1268, 1043, 817;1H NMR (DMSO-d6, 400 MHz) δ 11.72 (brs, 1H, NH), 8.63–8.62 (m, 1H, C-6′′H), 8.56–8.54 (m, 1H, C-5H), 7.70–7.62 (m, 1H, ArH), 7.05–7.02 (m, 2H, ArH), 6.93–6.83 (m, 2H, ArH), 6.64–6.56 (m, 1H, ArH), 6.26–6.23 (m, 1H, C-1′H), 5.97–5.92 (m, 2H, CH2), 5.73–5.64 (m, 1H, CH2), 5.55–5.52 (m, 1H, CH2), 5.34–5.32 (m, 1H, ArH), 5.08–5.05 (m, 1H, C-3′H), 4.71 (brs, 1H, OH), 4.31–4.25 (m, 2H, CH2), 3.88–3.85 (m, 1H, C-4′H), 3.63–3.59 (m, 2H, C-5′H), 2.22–2.17 (m, 4H, C-2′H, CH3), 2.14–2.13 (m, 1H, C-2′H); 13C NMR (DMSO-d6, 100 MHz) δ 161.6 (ArC), 160.5 (ArC), 160.0 (ArC), 158.0 (ArC), 154.0 (ArC), 150.1 (ArC), 145.5 (ArC), 139.8 (C-4), 136.8 (C-6′′), 130.8 (ArC), 130.1 (ArC), 129.7 (ArC), 123.9 (ArC), 118.6 (ArC), 112.9 (ArC), 110.6 (C-5), 109.2 (ArC), 105.4 (ArC), 88.1 (C-4′), 85.2 (C-1′), 80.5 (CH2), 71.1 (C-3′), 61.8 (C-5′), 49.7 (CH2), 45.1 (C-2′), 36.3 (CH2), 20.5 (CH3); HRMS (ESI): m/z calculated for C30H29N6O8+ [M + H]+: 601.2041, found: 601.2064.
4.4. Single-crystal X-ray structural analysis
High-quality crystals of compound 6f were obtained by slow evaporation of 5
:
1 methanol–chloroform solution at room temperature. The crystals were selected by careful examination under a polarizing microscope. Detailed experimental data for the X-ray crystallography of compound 6f can be found in Table S2 (refer to the ESI‡). The crystallographic data for compound 6f have been deposited with the Cambridge Crystallographic Data Centre, and a CCDC number has been assigned: 2343787 (Fig. 7).‡
 |
| Fig. 7 X-Ray crystallographic structure of compound 6f. | |
Conflicts of interest
The authors declare no competing financial interest.
Acknowledgements
We appreciate the funding provided by the Institute of Imminence (IoE), University of Delhi which has contributed to further research and development. We appreciate the assistance provided by USIC and the Department of Chemistry, University of Delhi, for recording NMR, HRMS and IR data. Aditi Arora (SRF, File No. 09/0045(11270)/2021/EMR-I) and Sumit Kumar (SRF, File No. 09/045(1798)/2020-EMR-1) are grateful to the CSIR, New Delhi for fellowships.
References
- L. P. Jordheim, D. Durantel, F. Zoulim and C. Dumontet, Nat. Rev. Drug Discovery, 2013, 12, 447 CrossRef CAS PubMed.
- N. J. Greco and Y. Tor, Tetrahedron, 2007, 63, 3515–3527 CrossRef CAS PubMed.
- Y. Saito and R. H. E. Hudson, J. Photochem. Photobiol., C, 2018, 36, 48–73 CrossRef CAS.
- D. Andreatta, S. Sen, J. L. P. Lustres, S. A. Kovalenko, N. P. Ernsting, C. J. Murphy, R. S. Coleman and M. A. Berg, J. Am. Chem. Soc., 2006, 128, 6885–6892 CrossRef CAS PubMed.
- L. Zilbershtein-Shklanovsky, M. Weitman, D. T. Major and B. Fischer, J. Org. Chem., 2013, 78, 11999–12008 CrossRef CAS PubMed.
- K. Seio, T. Kanamori and Y. Masaki, Tetrahedron Lett., 2018, 59, 1977–1985 CrossRef CAS.
- J. Zayas, M. Annoual, J. K. Das, Q. Felty, W. G. Gonzalez, J. Mikssovska, N. Sharifai, A. Chiba and S. F. Wnuk, Bioconjugate Chem., 2015, 26, 1519–1532 CrossRef CAS PubMed.
- E. Shipova and K. S. Gates, Bioorg. Med. Chem. Lett., 2005, 15, 2111–2113 CrossRef CAS PubMed.
- N. J. Greco and Y. Tor, J. Am. Chem. Soc., 2005, 127, 10784–10785 CrossRef CAS PubMed.
- S. G. Srivatsan and Y. Tor, J. Am. Chem. Soc., 2007, 129, 2044–2053 CrossRef CAS PubMed.
- C. Beauck, I. Singh, A. Bhattacharya, W. Hecker, V. S. Parmar, O. Seitz and E. Weinhold, Angew. Chem., Int. Ed., 2003, 42, 3958–3960 CrossRef PubMed.
- D. W. Dodd, K. N. Swanick, J. T. Price, A. L. Brazeau, M. J. Ferguson, N. D. Jones and R. H. E. Hudson, Org. Biomol. Chem., 2010, 8, 663 RSC.
- C. Dyrager, K. Borjesson, P. Diner, A. Elf, B. Albinsson, L. M. Wilhelmsson and M. Grotli, Eur. J. Org. Chem., 2009, 1515 CrossRef CAS.
- F. Seela and V. R. Sirivolu, Org. Biomol. Chem., 2008, 6, 1674 RSC.
- V. R. Sirivolu, P. Chittepu and F. Seela, ChemBioChem, 2008, 9, 2305 CrossRef CAS PubMed.
- M. M. Haque, H. Sun, S. Liu, Y. Wang and X. Peng, Angew. Chem., Int. Ed., 2014, 53, 7001 CrossRef CAS PubMed.
- S. S. Bag and H. Gogoi, J. Org. Chem., 2018, 83, 7606–7621 CrossRef CAS PubMed.
- A. P. Silverman and E. T. Kool, Chem. Rev., 2006, 106, 3775 CrossRef CAS PubMed.
- S. A. Azim, S. M. Al-Hazmy, E. M. Ebeid and S. A. El-Daly, Opt. Laser Technol., 2005, 37, 245–249 CrossRef CAS.
- M. Adamczyk, M. Cornwell, J. Huff, S. Rege and T. V. S. Rao, Bioorg. Med. Chem. Lett., 1997, 7, 1985–1988 CrossRef CAS.
- D. Cao, Z. Liu, P. Verwilst, S. Koo, P. Jangjili, J. S. Kim and W. Lin, Chem. Rev., 2019, 119, 10403–10519 CrossRef CAS PubMed.
- Z. Xu, G. Ding, G. Zhong, G. Xing, F. Li, W. Huang and H. Tian, Res. Chem. Intermed., 2008, 34, 249–256 CrossRef CAS.
- W. Y. Lin, L. L. Long and W. Tan, Chem. Commun., 2010, 46, 1503e5 Search PubMed.
- H. Q. Li, L. Cai, J. X. Li, Y. X. Hu, P. P. Zhou and J. M. Zhang, Dyes Pigm., 2011, 91, 309e16 CrossRef.
- M. C. Dilelio, N. P. Brites, L. A. Vieira, B. A. Iglesias, T. S. Kaufman and C. C. Silveira, Synthesis, 2019, 2965–2976 CAS.
- Y. Saito, A. Suzuki, Y. Okada, Y. Yamasaka, N. Nemoto and I. Saito, Chem. Commun., 2013, 49, 5684 RSC.
- D. Dziuba, P. Pospíšil, J. Matyašovský, D. Nachtigallová, L. Rulíšek, R. Pohl, M. Hof and M. Hocek, Chem. Sci., 2016, 7, 5775 RSC.
- A. P. Demchenko, J. Mol. Struct., 2014, 1077, 51 CrossRef CAS.
-
(a) A. Okamoto, K. Tainaka, K. I. Nishiza and I. Saito, J. Am. Chem. Soc., 2005, 127, 13128 CrossRef CAS PubMed;
(b) D. Dziuba, V. Y. Postupalenko, M. Spadafora, A. S. Klymchenko, V. Guérineau, Y. Mély, R. Benhida and A. Burger, J. Am. Chem. Soc., 2012, 134, 10209 CrossRef CAS PubMed;
(c) K. Gavvala, N. P. F. Barthes, D. Bonhomme, A. S. Dabert-Gay, D. Debayle, B. Y. Michel, A. Burger and Y. Mély, RSC Adv., 2016, 6, 87142 RSC;
(d) N. P. F. Barthes, I. A. Karpenko, D. Dziuba, M. Spadafora, J. Auffret, A. P. Demchenko, Y. Mély, R. Benhida, B. Y. Michel and A. Burger, RSC Adv., 2015, 5, 33536 RSC.
- S. Srivastava, V. K. Maikhuri, R. Kumar, K. Bohra, H. Singla, J. Maity and A. K. Prasad, Carbohydr. Res., 2018, 470, 19–25 CrossRef CAS PubMed.
- S. Kumar, S. Kumar, J. Maity, B. Kumar, S. B. Mehta and A. K. Prasad, New J. Chem., 2021, 45, 16635–16647 RSC.
- H. Singla, S. Kumar, V. K. Maikhuri, Kavita and A. K. Prasad, ChemistrySelect, 2023, 8, e202203412 CrossRef CAS.
- R. Rajaganesh, P. Ravinder, V. Subramanian and T. M. Das, Carbohydr. Res., 2011, 346, 2327–2336 CrossRef CAS PubMed.
- T. O. Olomola, R. Klein, K. A. Lobb, Y. Sayed and P. T. Kaye, Tetrahedron Lett., 2010, 51, 6325–6328 CrossRef CAS.
- S. S. Bag, S. Talukdar, S. K. Das, M. K. Pradhan and S. Mukherjee, Org. Biomol. Chem., 2016, 14, 5088–5108 RSC.
- S. Kumar, A. Arora, R. Kumar, N. N. Senapati and B. K. Singh, Carbohydr. Res., 2023, 108857 CrossRef CAS PubMed.
- W. Lin, L. Long, J. Feng, B. Wang and C. Guo, Eur. J. Org. Chem., 2007, 4301–4304 CrossRef CAS.
- Y. C. Duan, Y. C. Ma, E. Zhang, X. J. Shi, M. M. Wang, X. W. Ye and H. M. Liu, Eur. J. Med. Chem., 2013, 62, 11–19 CrossRef CAS PubMed.
- H. Mostafavi, R. Najjar and E. Maskani, Chem. Nat. Compd., 2013, 49, 3 CrossRef.
- V. Rostovtsev, L. Green, V. Fokin and B. Sharpless, Angew. Chem., Int. Ed., 2002, 41, 2596–2599 CrossRef CAS PubMed.
- K. Sivakumar, F. Xie, B. M. Cash, S. Long, H. N. Barnhill and Q. Wang, Org. Lett., 2004, 6, 4603–4606 CrossRef CAS PubMed.
- V. K. Maikhuri, K. Bohra, S. Srivastava, Kavita and A. K. Prasad, Synth. Commun., 2019, 49, 3140–3147 CrossRef CAS.
- R. Bawa, P. Gahlyan, M. Dalela, K. Jindal, P. K. Jha, M. Tomar, V. Gupta and R. Kumar, Dyes Pigm., 2019, 161, 162–171 CrossRef.
- D. H. Hilko, L. F. Bornaghi and S. A. Poulsen, Curr. Protoc. Nucleic Acid Chem., 2019, 77, 1–23 Search PubMed.
-
B. P. Mathew, Synthesis and Antimicrobial evaluation of dihydro[1,3]oxazine derivatives, 2011 Search PubMed.
- M. K. Mahadevan, H. N. Harishkumar, J. N. Masagalli and M. N. N. Kumara, SOP Trans. Org. Chem., 2014, 1, 20–30 Search PubMed.
-
M. J. Frisch, G. W. Trucks, H. B. Schlegel, G. E. Scuseria, M. A. Robb, J. R. Cheeseman, G. Scalmani, V. Barone, B. Mennucci, G. A. Petersson and H. Nakatsuji, Gaussian 09, Gaussian Inc, Wallingford, CT, 2009 Search PubMed.
-
R. Dennington, T. Keith and J. Millam, Gauss View 5.0, Semichem Inc, Shawnee, KS, 2009 Search PubMed.
- A. D. Becke, Phys. Rev. A, 1988, 38, 3098–3100 CrossRef CAS PubMed.
- C. Lee, W. Yang and R. G. Parr, Phys. Rev. B: Condens. Matter Mater. Phys., 1988, 37, 785–789 CrossRef CAS PubMed.
- L. E. Daga, B. Civalleri and L. Maschio, J. Chem. Theory Comput., 2020, 16, 2192–2201 CrossRef CAS PubMed.
- E. Zahedi, S. Shaabani and A. Shiroudi, J. Phys. Chem. A, 2017, 121, 8504–8517 CrossRef CAS PubMed.
- S. Namuangruk, S. Jungsuttiwong, N. Kungwan, V. Promarak, T. Sudyoadsuk, B. Jansang and M. Ehara, Theor. Chem. Acc., 2016, 135, 1–13 Search PubMed.
- E. Runge and E. K. U. Gross, Phys. Rev. Lett., 1984, 52, 997 CrossRef CAS.
Footnotes |
† We dedicate this article to the fond memory of our beloved Late Prof. Ashok K. Prasad. |
‡ Electronic supplementary information (ESI) available. CCDC 2343787. For ESI and crystallographic data in CIF or other electronic format see DOI: https://doi.org/10.1039/d4ob00749b |
|
This journal is © The Royal Society of Chemistry 2024 |
Click here to see how this site uses Cookies. View our privacy policy here.