DOI:
10.1039/D4PM00201F
(Review Article)
RSC Pharm., 2024,
1, 675-688
A brief overview of quality by design approach for developing pharmaceutical liposomes as nano-sized parenteral drug delivery systems
Received
9th July 2024
, Accepted 31st August 2024
First published on 5th September 2024
Abstract
Liposomes are sophisticated drug delivery vehicles that have significantly broadened the range of therapeutic agents that can be selectively delivered along with controlled release. Liposomes are small vesicles (size optimizable) composed of a lipid bilayer that encapsulates hydrophilic as well as hydrophobic drugs. This advancement has led to the creation of liposomal nano-formulations for drugs with very poor water solubility and cell membrane permeability, resulting in improved therapeutic efficacy and reduced side effects. Liposomal formulations can also be engineered with ligands or antibodies to target specific cells or tissues, ensuring site-specific drug delivery and minimizing off-target side effects. These targeted liposomal formulations have shown promising potential in treating various diseases, such as cancer, infectious diseases, and inflammatory disorders. With continuous advancements in liposomal technology, researchers are exploring new ways to further optimize the liposomal formulations for enhanced drug stability, bioavailability, and targeted delivery to specific cells or tissues. The Quality by Design (QbD) approach is a systematic and scientific method for designing and developing pharmaceutical products, ensuring quality and consistency throughout the product lifecycle. Applied to the development of pharmaceutical liposomes, QbD facilitates the optimization of liposome formulations for targeted drug delivery, improved stability, and enhanced therapeutic outcomes. The creation of novel liposomal formulations with superior drug stability, bioavailability, and targeting capabilities will undoubtedly play a crucial role in shaping the future of medicine and improving patient care. This brief review provides an overview of the development of liposomes as nanocarrier systems for parenteral drug delivery, covering aspects such as quality manufacturing attributes, structure, preparation methods, characterization, clinical applications, and regulatory considerations.
1. Introduction
Lately, nanotechnology has been widely used to address the issues of low solubility, efficacy, and poor bioavailability in the drug discovery and development processes.1 The prefix nano is derived from the Greek word ‘nanos’, meaning dwarf or small, and one nanometer is equivalent to one billionth of a meter.2,3 Several new drug delivery systems developed with various polymers (natural, synthetic, and semi-synthetic) have demonstrated significant limitations, including toxicity and the absence of acceptable production techniques.4,5 To overcome these problems, there has been a growing interest in lipid-based nanocarrier systems.6 Lipid-based nanocarriers have emerged as a promising approach and have garnered significant attention due to their desirable features, including improved biocompatibility, high drug loading efficiency, enhanced bioavailability, controlled release properties, reduced toxicity, protection against degradation in the gastrointestinal tract (GIT), ease of scale-up, sterilization, validation, and suitability for drug delivery through various routes of administration. Lipid nanocarriers can be used to deliver both hydrophilic and hydrophobic drugs. Formulation of lipid nanocarriers can improve drug solubility, which is a rate-determining step for BCS (Biopharmaceutics Classification System) Class II (low solubility, high permeability), and IV (low solubility, low permeability) drugs; similarly, lipid nanocarriers can enhance permeability, a rate-determining step for BCS Class III (high solubility, low permeability) drugs.
Lipid nanocarriers are classified as (1) Vesicular Systems: liposomes, ethosomes, and transferosomes; and (2) Nonvesicular Systems: Solid lipid nanoparticles, lipid drug conjugates, and nano-structured lipid carriers.2 Liposomes are colloidal carrier systems formed from polar lipids and water, creating self-organized particulate systems. Liposomes consist of one or more lipid bilayers that encase aqueous compartments. Among colloidal particulate structural systems, liposomes have been examined extensively, and their attractiveness lies in their optimizability, biocompatibility, and biodegradability.7,8 The word ‘liposome’ consists of two Greek words: ‘lipos’, meaning fat, and ‘soma’, meaning body.2 Liposomes are microparticulate, colloidal, concentric bilayered vesicles in which a lipid bilayer membrane, composed of natural or synthetic phospholipids, entraps an aqueous volume (Fig. 1). Liposomes are classified according to their size as small, intermediate, or large and according to lamellarity as unilamellar, oligolamellar, and multilamellar vesicles. Cargos with diverse lipophilicities can be encapsulated in liposomal drug delivery systems in a phospholipid bilayer, aqueous volume, or a bilayer interface.7 The diameter of liposomes varies from 0.02 to 10 μm.2
 |
| Fig. 1 Bilayer liposome structure with encapsulated hydrophilic drug in the aqueous core. | |
Liposomes are comprised of natural or synthetic phospholipids such as phosphatidylethanolamine, phosphatidylcholine (PC), phosphatidylglycerol (PG), and phosphatidylserine (PS). Lipids can exist in either a gel or fluid state, depending on the temperature. In their fluid state, lipids readily enable the entrapment of the aqueous phase, which can be utilized effectively. The phospholipids possess a natural flip-flop that encourages liposome leakiness;9 cholesterol is often added to liposome formulations to stabilize the lipid bilayer,10 and various levels of drug loading can be achieved by including different cholesterol concentrations.11 Additionally, vesicle size is directly proportional to the concentration of added cholesterol in the formulation.12 The clinical behavior of liposomes is influenced by their charge, which can be positive, negative, or neutral, depending on the addition of inactive ingredients and lipids.13 For example, the addition of oleic acid produces liposomes with a negative charge, while the addition of DOTAP (N-[1(2,3-dioleoyloxy)propyl]-N,N,N-trimethylammonium chloride) generates positively charged liposomes. Charged liposomes exhibit electrostatic repulsion, which leads to slower aggregation over their shelf life. Cationic liposomes show electrostatic attraction with negatively charged cell membranes, increasing cell-liposome interaction and easier cargo release.14 A research study demonstrated that the polycationic layer thickness influences liposomal membrane fusion. Fusion is favored by the ability of charge-neutralizing macromolecules to self-assemble into thin, planar arrays whereas polymers engaging in brush-heap configuration result in repulsion.15 A liposomal ciprofloxacin formulation was optimized to remodel the encapsulation and release properties by adding commonly used surfactants.16 In another study, the effect of surface hydrophilicity on charged liposomes composed of varying phospholipids was explored to achieve local delivery and peritoneal retention of an anti-cancer drug.17
This brief review will outline varied details of the vesicular drug delivery system, ‘liposomes’. In particular, it will focus on the Quality by Design approach for pharmaceutical liposome development, preparation methods, structure and critical quality attributes, liposomal drug delivery, and regulatory considerations. QbD principles cultivate the quality of the drug product by involving risk assessment strategies and sound scientific and empirical data.18 Quality can be further built into the product by enhancing understanding and improving the manufacturing process, reducing the time gap between development and industrial scale.19,20 The detailed elements of pharmaceutical QbD are presented in the subsequent sections.
2. QbD mediated development of pharmaceutical formulations
Pharmaceutical Quality by Design is a systematic, scientific, risk-based, holistic, and proactive approach to pharmaceutical formulation development. It begins with predefined objectives and emphasizes product and process understanding and process control. This approach integrates advanced scientific methods into research and development, ensuring quality is built into product processing from the early stages.21 QbD approach helps identify the quality attributes from the patient's perspective and translates them into critical drug product characteristics. It establishes a connection between formulation and manufacturing variables such as Critical Material Attributes (CMAs), Critical Process Parameters (CPPs), and Critical Quality Attributes (CQAs) to produce high-quality drug products consistently.18 A comprehensive QbD study consists of four key elements: (a) Identification of quality target product profile (QTPP) and performance of risk-assessment analysis; (b) product and manufacturing process design to meet predefined objectives; (c) identification of CQAs, CMAs, and CPPs to establish a design space; and (d) control manufacturing parameters to continually produce a quality product.21 A succinct description of these elements is detailed below.
2.1. Identification of quality target product profile and risk assessment analysis
Quality target product profile is an anticipated summary of the attributes of a drug product considering patient safety (stability and degradation products) and efficacy (bioavailability) that preferably will be attained to ensure the desired product quality.18 Hazard identification followed by risk analysis are two fundamental units of risk assessment as mentioned in the International Council for Harmonization (ICH) Q9 document.22 Both these units are critical in applying the QbD approach in pharmaceutical product development. Hazard identification deals with information identifying potential risk factors, whereas risk analysis deals with the severity and occurrence of harm. Risk assessment analysis aims to identify high-risk factors affecting the drug product's CQAs. Fig. 2 is an Ishikawa (fishbone) diagram that visually represents the various factors that could affect the critical quality attributes of a product, helping teams to identify and address potential issues systematically.
 |
| Fig. 2 Ishikawa fishbone diagram depicting factors that may significantly impact CQAs. | |
2.2. QbD guided liposome manufacturing processes
Quality by design focuses on optimizing input materials and manufacturing processes to produce superior-quality liposomal products. It also identifies and translates critical parameters and key variables into a systematic framework that ensures the production of drug products with the most desired characteristics.23,24 Liposomes should exhibit prominent entrapment efficiencies, longer shelf life, narrow size distribution, and better release profiles for superior drug delivery. Liposome formation is based on hydrophilic and/or hydrophobic interactions between lipid-lipid and lipid–water entities. Energy input drives lipid positioning through bilayered vesicles, maintaining thermodynamic equilibrium with the aqueous phase.25 The manufacturing of liposomes is further categorized into three methods: mechanical methods, methods based on replacing organic solvents, and methods based on detergent removal.26
Liposomal preparation often involves mechanical methods such as the film method, homogenization, and extrusion. The standard steps in the film method include hydration, hydration with agitation, and sizing to achieve a uniform vesicular distribution. Despite several modifications, this method yields a heterogeneous liposome distribution. Vesicle size is impacted by energy input, the nature of the aqueous phase, and lipid charge. As mentioned earlier, difficulty in scale-up is the major constraint of the method. Extrusion is the most prominent scalable method for downsizing liposomes. In this process, pre-formed vesicles are forced through a membrane under pressure to achieve size reduction. The homogenization technique reduces liposomal size and decreases the number of lamellae in multilamellar liposomes. Additionally, size reduction is achieved by causing larger vesicles to collide under pressure in a microfluidizer.27
Liposome preparation methods that replace organic solvents with aqueous media involve substituting water-miscible or immiscible solvents with an aqueous phase. Standard techniques in this category include the pro-liposome-liposome method, which involves the stepwise addition of an aqueous phase to a non-aqueous phase, and the ethanol injection method, where a non-aqueous phase is injected into an aqueous solution. Additionally, reverse-phase evaporation and double-emulsion techniques also facilitate the replacement of organic solvents with a polar phase. Liposomal preparation methods may incorporate detergents, such as bile salts or alkyl glycosides, to enhance lipid solubilization within micellar systems. Unlike lipids, detergents are soluble in both aqueous and non-aqueous phases, maintaining equilibrium between these environments. Standard procedures for detergent removal include dialysis through membrane filters, gel chromatography, and adsorption using cyclodextrins or hydrophobic resins.26,27
3. Critical quality attributes of liposomal drug delivery system
Liposomal characteristic details can aid in determining the fate of liposomes under several clinical applications, settings, and storage environments. Critical Quality Attributes of a liposomal drug delivery system are essential parameters that must be carefully controlled to ensure the product's safety, efficacy, and quality. Some of the significant attributes are as follows.
3.1. Particle size and polydispersity
Size and polydispersity (size distribution) play a crucial role in determining the physicochemical properties of the dosage form, offering insights into batch quality and manufacturing consistency. Additionally, these factors influence the biological properties and, ultimately, the clinical outcomes.28 Furthermore, they both play a critical role in parenteral routes of administration.29 Size determination can be performed by size exclusion chromatography (SEC), nuclear magnetic resonance (NMR), dynamic light scattering (DLS), and microscopic technologies, including atomic force microscopy (AFM), transmission electron microscopy (TEM), and cryogenic-TEM (Cryo-TEM).3,14
The DLS method is most widely used and is dependent on the Brownian motion of the suspended colliding particles. The incident light is scattered due to the difference in refractive index between the suspended particles and the solvent/vehicle. The DLS method uses the shifts in light scattering intensity observed over a range of time intervals. A major limitation of this method is the false determination of the particle size due to particle aggregation.14,30
Microscopic techniques such as Transmission Electron Microscopy and Cryogenic Transmission Electron Microscopy are also widely used to obtain liposomal images.31 However, a radical limitation of this technique is associated with the disturbance of the lipid chemistry since liposomes are removed from their native environment for image analysis.14 The sample preparation process and cryo-TEM analysis of colloidal systems are challenging and demand meticulous control throughout the process.32–34
3.2. Encapsulation efficiency
The entrapment efficiency of the liposomal drug delivery systems depends on the drug's solubility, molecular weight, liposome composition, dispersion medium, and liposome size, etc.35 Passive drug loading occurs during the preparation process without additional steps. In contrast, active drug loading methods offer superior entrapment efficiency due to the gradient between lipid bilayers. In this approach, liposomes are initially suspended in a buffer with a known pH, while the uncharged drug is loaded in a second buffer with a different pH. The resulting pH gradient drives the uncharged drug molecules into the lipid bilayers, where they become charged. Once charged, these molecules cannot diffuse across the lipid bilayers and remain entrapped within them.3,14,36
3.3. Zeta potential
Zeta potential (ZP) measures the surface charge of a liposome formulation. It is crucial in determining its interactions with body tissues, systemic circulation, and cellular recognition.37 A surface charge of ±30 mV is essential for maintaining formulation stability and preventing particle aggregation.38 ZP is crucial for drug loading and can be optimized through charge–charge interactions, encapsulation, covalent conjugation, and hydrophobic interactions.3 Additionally, ZP can be manipulated by biopolymer deposition on the liposomal surface,39 adjusting lipids’ pH and molar ratio during formulation optimization, among others.38,40
3.4. Sterility
Liposomal formulations usually contain phospholipids and cholesterol but may also comprise additional ligands that impart unique characteristics. All these formulation ingredients are probable sources of microbial contaminants such as bacteria, endotoxins, viruses and pyrogens.41 Sterilization of liposomes is challenging owing to their distinctive composition and unique manufacturing requirements. Sterile filtration can be achieved via membrane filtration for liposomal particles smaller than 200 nm, allowing the larger particles to retain on the filter membrane. Gamma irradiation results in peroxidation and destabilization of the phospholipid bilayer.
Further, irradiation is also attributed to free radical phospholipid fragmentation. Saturated steam sterilization eliminates the lipid peroxidation issues; however, encapsulated contents leak, hydrolysis of phospholipids, and vesicle aggregation. Due to the limitations of the methods mentioned earlier, aseptic manufacturing is a better choice and can promote the manufacturing of sterile parenteral liposomes. All solvents (organic and aqueous), lipids, and other excipients are passed through 200 nm filter membranes while the manufacturing equipment can be autoclaved. Further, prepared liposomes are assembled into containers via the aseptic filling method. The filling process is performed in Class-100 environments, designed to maintain a sterile environment during the entire process of aseptic manufacturing.14,42
3.5.
In vitro drug release
In vitro release studies are performed under dialysis conditions. Liposomal formulations are immersed in dialysis bags with appropriate molecular weight cut-offs in a dissolution medium and mixed continuously. To mimic in vivo conditions, dialysis is performed at 37 °C, and the dissolution medium consists of buffered saline at pH 7.4. Samples are withdrawn and analyzed for released pharmaceutical content at pre-determined time intervals. A release profile plot estimates the drug release from the liposomal carrier.14
4. Liposomal drug delivery systems
Liposomes are highly versatile and practical drug delivery systems, leveraging the unique properties of liposomes to enhance therapeutic efficacy. These systems consist of spherical vesicles with one or more lipid bilayers capable of encapsulating hydrophilic and hydrophobic drugs. Liposome encapsulation protects the drug from degradation, improves bioavailability, and allows for controlled release. Liposomal systems can be engineered to target specific tissues or cells, reducing systemic side effects and increasing the drug concentration at the desired site of action (Fig. 3). Liposomes’ unique properties, such as their ability to carry small molecules and macromolecules, optimize drug solubility and stability, and control drug release kinetics, make them a promising tool for drug delivery in various medical fields.14,43,44
 |
| Fig. 3 Surface modification strategies of liposomes, together with their classification. The modified carriers can contain active components, such as drugs, small molecules, proteins, and/or targeting moieties, such as antibodies, peptides, aptamers, etc., conjugated on the surface of the vehicles through different linkers, non-covalent or covalent bonds, and electrostatic interactions. Abbreviation: PL – phospholipid. Reproduced under the terms of the Creative Commons Attribution License. M. P. Nikolova, E. M. Kumar and M. S. Chavali, Updates on responsive drug delivery based on liposome vehicles for cancer treatment, Pharmaceutics, 2022, 14(10), 2195. https://doi.org/10.3390/pharmaceutics14102195. | |
4.1. Conventional versus long circulating liposomes
Targeted drug delivery and biodistribution throughout the body are two fundamental problems in drug therapy. Liposomal formulations protect encapsulated active molecules from degradation and passively target tissues/organs that do not have a continuous endothelium (spleen, liver, and bone marrow). When administered intravenously, the mononuclear phagocyte system (MPS) rapidly captures and removes liposomes from blood circulation.45 This behavior efficiently delivers antimicrobial and antiparasitic agents to treat localized infections in MPS. Even so, one of the significant disadvantages of the liposomal drug delivery system is liposome uptake and their subsequent removal from blood circulation when the site of action is beyond the MPS. The binding of serum proteins/opsonins (e.g., immunoglobulins, fibronectin, C-reactive protein, etc.) results in rapid clearance of liposomes as MPS recognizes opsonins bound to the surface of the liposomes. Another limitation of conventional liposomes is their interaction with high and low-density lipoproteins.46
Several strategies have been developed to control the rapid removal of liposomes in vivo.47 The first strategy studied was to mimic erythrocyte membrane in preparation of liposomes. The composition of liposomes was modified with sialic acid derivatives and gangliosides. The next step was to improve the hydrophilicity of liposomal surfaces with hydrophilic polymers.7 Liposomal longevity in circulation can be markedly increased via steric stabilization.48 The core principle is that polyethylene glycol, a hydrophilic polymer with a flexible chain, occupies the space next to the perisomal layer. This, in turn, prevents other macromolecules from entering this area. Thus, access to plasma proteins in the perisomal layer is hampered, reducing macrophage interaction.7 While PEG can extend the longevity of liposomes, some drawbacks are associated with this hydrophilic polymer. Immune response is triggered, and the generated antibodies (IgM) bind PEG, resulting in ABC (accelerated blood clearance) phenomenon. This can be alleviated by using non-cleavable high molecular weight PEG derivatives. Intracellular accumulation of PEG affects lysosomal density by interfering with the outflow of metabolites. To overcome the limitations associated with PEG, substitute materials such as glycopolymers, copolymers, polyglycerols, dextrans, hyaluronic acid etc. have been proposed.49
Long circulating liposomes offer a significantly advantageous delivery system for anti-cancer agents as current chemotherapy focuses on destroying rapidly dividing cells. The pitfall of this therapy is that the body's other rapidly proliferating cells, such as intestinal epithelium and hair follicles are also destroyed.1 Long-circulating liposomes often accumulate in tumor tissues due to the enhanced permeability and retention (EPR) effect. However, effective distribution within the tumor remains a significant challenge. Tumor tissue typically features high interstitial fluid pressure, irregular blood vessels, and uneven blood flow. Vasoactive agents such as bradykinin, thrombin, substance P, endotoxin, and VEGF (anti-vascular endothelial growth factor) can be administered to improve vascular permeability. These vasoactive agents generate cellular events, increasing vascular permeability.50
The chemotherapeutic activity of paclitaxel was demonstrated using a pre-clinical approach by Shi et al. VEGF is particularly cumulated in the tumor tissue, thus resulting in an overexpression of VEGF. Stable PEGylated paclitaxel liposomes conjugated with the VEGF mAb (monoclonal antibody) were produced. The anticancer activity was further analyzed in BALB/C nude mice bearing SGC-7901 xenografts. VEGF-targeted mAb-liposomes were visually observed in the tumor's interior, suggesting the chemotherapeutic agent's targeted delivery.51 The potential of another chemotherapeutic agent, doxorubicin, was explored to enhance safety and efficacy. Targeted (functionalized) liposomes offer an enhanced system for the delivery of doxorubicin. C225-immunoliposomal(IL)-DOX is a PEGylated liposome that encapsulates doxorubicin and is conjugated with the antigen-binding fragment (Fab’) of the monoclonal antibody Cetuximab. C225-immunoliposomal(IL)-DOX showed tumor growth inhibition in different mouse models and healthy adult rats.52
Drug loading of Imatinib in magnetic liposome nanocomposites showed its anti-cancer capability. In vitro evaluation illustrated the active release from magnetoliposome nanocomposites in the presence of AMF (alternative magnetic field) by altering the bilayer permeability. In vivo assessment exhibited the accumulation of liposomes at the targeted sites in a rapid and efficient manner. This multidisciplinary technique may be an effective cancer therapy.53
A ligand-mediated approach should be thoroughly explored to address liposome's rapid clearance and degradation after administration to improve intracellular drug delivery. Antibodies are widely used ligands for target-specific cargo delivery in chemotherapy. Initial attempts to utilize antibodies as ligands can be traced back to the late 1970s to early 1980s. Antibody-modified liposomes have been used to perform liposomal targeting both in vitro and in vivo.54
5-Flurouracil (5-FU) is cytotoxic and is immensely used in treating cancers such as the brain, colon, breast, head, liver, neck, and ovary. 5-FU has certain limitations, such as wide distribution, cardiac toxicity, damage to the central nervous system, short half-life, dermatitis, etc., that result in limited use of 5-FU. Folic acid was conjugated with liposomes to overcome these shortcomings and achieve targeted delivery. Folate-targeted liposomal formulations showed improved efficacy than free drugs, with no tissue abnormalities studied in vivo.55 Breast cancer is very common among women, with a mortality rate of 17.7%. Tumor cells are highly heterogeneous, thus making cancer treatment a challenge. Folic acid modified curcumin liposomes were evaluated in 2D and 3D cell culture models. The liposomes exhibited a zeta potential of −13 mV, encapsulation efficiency of 73%, and a particle size of ∼138 nm. These nanosystems illustrated cytotoxic effects owing to tissue permeability and selective recognition by folate receptors.56
4.2. Lyophilized liposomes
Liposome formulations can be administered through multiple routes, including oral, transdermal, ophthalmic, nasal, and pulmonary, but the intramuscular and intravenous routes are the most thoroughly investigated.57 Despite their benefits, these complex systems often face physico–chemical instabilities that can shorten shelf life. Key factors impacting the stability include liposome aggregation, phospholipid oxidation, poor re-dispersibility, and drug leakage. Lyophilization (freeze-drying) is commonly used to mitigate these stability risks. Liposomal integrity is a significant challenge in the lyophilization process. During freezing, ice crystals forming within the core or external aqueous phase can rupture the lipid bilayer structure.58
Reconstituting liposomes with protein, peptide, or gene cargo is particularly challenging. The lyophilization process for liposome formulations follows a procedure similar to that for small molecules, including freezing, primary drying, and secondary drying, to achieve the desired moisture content in the final dried liposomes. Preserving the bioactivity of encapsulated biological entities during lyophilization is a key challenge, often addressed by incorporating lyoprotectants into the formulations.59 These lyoprotectants help maintain the structure of biological entities through direct binding mechanisms during dehydration.60 Scale-up of the lyophilization process and the choice of excipients are critical factors. Regulatory agencies must develop comprehensive guidance to ensure the safety and efficacy of liposomal products.
A lyophilized liposomal formulation accommodating peptidomimetic-doxorubicin conjugate to achieve targeted delivery to HER2-positive cancer cells was described. The pH-sensitive lyophilized formulation showed better efficacy than it's free form. Such liposomal delivery systems exhibited long-term stability at 4 °C.61
4.3. Hybrid liposomes
Hybrid liposomes (HL) combine liposomal formulations with another delivery system through complexation or encapsulation to enhance the benefits of liposomes.62 This approach helps avoid the individual drawbacks of each component. A straightforward example of HL is the encapsulation of magnetic nanoparticles within liposomal formulations.14 Hybrid liposomes have been developed using silver and gold nanoparticles. Additionally, the long-circulating properties of PEGylated liposomes for anti-cancer applications have been investigated. Three methods for HL preparation have been discussed: (a) modified emulsification method/reverse phase evaporation; (b) one-step nanoprecipitation using microfluidic technology and (c) classical thin-film followed by sonication/extrusion.63 An integrated self-assembly of lipids and amphiphilic co-polymers is discussed in detail, along with the state-of-the-art design structure and future applications.64 Hydrophobic drugs were loaded into liposomes by encapsulating those active moieties in cyclodextrins (pre-loading), followed by active loading into liposomes.65
Recent trend is combining liposomes (including biomimetics) and exosomes to harness the advantages of both systems for more efficient drug delivery. One promising approach is through membrane fusion, where the lipid membranes of liposomes and exosomes are fused together to create hybrid vesicles containing both liposomal and exosomal components. Several techniques have been developed to facilitate the fusion of liposomes and exosomes, including chemical methods such as the introduction of fusogenic lipids or viral fusogens, as well as physical methods such as sonication or electroporation.66–68
A combination therapy of linalool and doxorubicin encapsulated liposomes was demonstrated as a potential effective treatment against epithelial ovarian cancer. The particle size of the prepared LN-NE-DOX (linalool nanoemulsion and doxorubicin)-liposomes was determined by light scattering. In vitro toxicity was assessed in HeyA8 cells by MTT Assay. Further, in vivo therapeutic efficacy and biochemical toxicity were evaluated in female BALB/C mice. This study demonstrated the efficacy of a combination therapy in a mouse model.69
Infectious diseases can be treated with DNA vaccines. However, weak immunogenicity and short half-life limit the usage of DNA vaccines in preclinical and clinical studies. Zhao et al., reported the delivery of multi-epitope self-replication DNA vaccine (pSFV-MEG) utilizing a liposome-polymer hybrid nanoparticle system. The system comprised of a biodegradable core and a hydrophilic shell made of up mPEG-PLGA and lecithin/PEG-DSPE-Mal 2000 respectively. The in vitro release profile of the prepared hybrid liposomes was monitored. Subsequently, these hybrid systems were injected in BALB/C mice intramuscularly to study the effect in vivo. In conclusion, a DNA vaccine and nano-delivery systems was developed for the treatment of infectious diseases.68
4.4. Liposomal vaccine delivery
Liposomal capacity for vaccine delivery has been highlighted, especially during the COVID-19 pandemic.70–72 Liposomal vaccine formulations consisting of encapsulated or surface-presented antigens, are engulfed by macrophages, thus triggering an immune response.73 Consequently, the antigen peptides present on the surface of the macrophages bind to T-cells. The helper T-cells generate cytokines, are associated with B-cells, and prompt the production of antibodies. Liposomal formulations serve as a good depot for steady and unbroken providers of antigens. A specific immune response can be provoked by suitable alterations in liposomal composition, charge, size and encapsulated or surface antigen.14 A research study demonstrates the critical role of formulation composition and the charge in preparing liposomes with adjuvant properties.74
Besides, virosomes (liposomes embedded with viral envelope proteins within their phospholipid bilayer),75,76 and archaesomes (liposomes derived from the polar lipids of archaea, characterized by their distinctive ether-linked lipids)77,78 have gained importance as carrier systems for vaccine delivery. Based on the chemical properties, hydrophilic antigens, such as nucleic acids, peptides, proteins, etc., are entrapped in the inner aqueous core of liposomal space. In contrast, hydrophobic antigens or adjuvants are interspersed into the lipid bilayer. A coformulation consisting of hydrophilic and hydrophobic antigens and surface adjuvants can be tailored per the need of individual therapy. Further, nucleic acid vaccines (DNA or RNA) are a suitable alternative to attenuated viral vaccines.79
The first liposome vaccine based on virosome technology, Epaxal®, was developed by Swiss Berna Crucell Biotech and launched in 1993 for hepatitis A. Inflexal® V, a virosome-assisted influenza vaccine, was developed by Swiss Berna Crucell Biotech and launched in 1997.80 Both these products were launched in Europe. Liposome-like nanoparticles (LLPs) exhibiting structural similarity to liposomes have recently illustrated their capability of vaccine delivery, which was advantageous in containing the spread of the global COVID-19 pandemic (Fig. 4). Two such mRNA vaccines utilizing lipid technology were developed jointly by Moderna, Pfizer, and German BioNTech (Comirnaty®).81
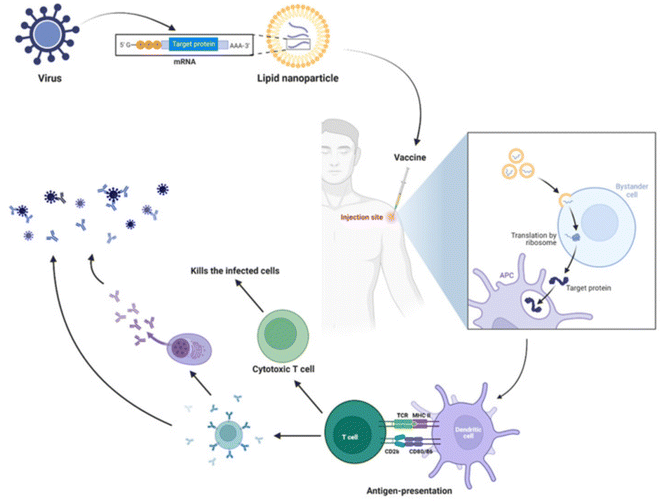 |
| Fig. 4 Immune response by mRNA vaccines. Lipid nanoparticles (LNPs) are prepared by encapsulating mRNA, which encodes the viral protein of interest. Upon injection of vaccines, muscular cells take up the LNPs following the release of mRNA into the cytosol and translation of target protein with the help of host machinery. In parallel, the danger-associated signals produced by the LNPs recruit the innate immune cells, including neutrophils, monocytes, macrophages, dendritic cells, and others. The antigen-presenting cells (APC) process and present the antigen to the T cells, further polarizing into effector T cells and helping in B cell-mediated responses. The cytotoxic T cells produced upon activation kill the infected cells, and antibodies (produced by B cells or plasma cells) neutralize the virus. K. Swetha, N. G. Kotla, L. Tunki, A. Jayaraj, S. K. Bhargava, H. Hu, S. R. Bonam and R. Kurapati, Recent advances in the lipid nanoparticle-mediated delivery of mRNA vaccines, Vaccines, 2023, 11(3), 658. https://doi.org/10.3390/vaccines11030658. | |
5. Application of QbD approach in the design of liposomal formulations
This section will present a few examples that best showcase the utilization of Quality by Design principles in developing and optimizing robust parenteral liposomal formulations. Readers are encouraged to refer to various recently published articles cited throughout this manuscript for further in-depth and exhaustive discussion on the specific areas of interest.23,82–93
Novel pharmaceutical preparatory approaches can be time-consuming, but applying Quality by Design principles from the early stages of formulation and process optimization can help bridge the time gap between development and preclinical studies. The optimization of a prototype formulation involves a comprehensive analysis of product quality attributes. Often, the identification of risk factors resulting in the failure of the product is recognized after a substantial investment of time. This results in increased testing and does not guarantee product quality. Therefore, risk assessment is valuable and can be performed using screening designs. The optimized design space and formulation can be obtained using the Design of Experiments (DOE). These experimental designs provide a larger advantage by considerably reducing the number of trials while simultaneously analyzing the effect of multiple factors. Initial in vitro, in vivo and ex vivo studies can provide a better understanding of the formulation behavior thereby providing an augmented success rate for preclinical and clinical trials.
Azacitidine-loaded nano liposomal formulations were developed using a Box-Behnken design, and in vitro evaluation was conducted with MCF-7 cells. Azacitidine, a DNA methyltransferase inhibitor, is commonly used in the treatment of breast cancer and myelodysplastic syndrome. The optimized formulation was evaluated for surface charge, particle size, polydispersity index, entrapment efficiency, drug loading, in vitro drug release, and through TEM, X-ray diffraction (XRD), and hemolytic toxicity assessments. The optimized batch exhibited desirable properties, and the study highlighted the anticancer potential of azacitidine-loaded liposomes. The formulation demonstrated suitability for preclinical and clinical studies as an effective anti-cancer drug delivery system.94 Additionally, this illustrates how applying QbD concepts can reduce development time and produce a liposomal formulation with optimal therapeutic properties ready for preclinical evaluation.
Lu et al. applied QbD concepts to analyze the effects of formulation and process variables on the quality attributes of multivesicular liposomes containing bupivacaine. Several Ishikawa diagrams were constructed to identify potential risk factors. The defined CQAs were particle size, morphology, and encapsulation efficiency. This study further details the effects of processing parameters and material attributes during liposome preparation.95
A QbD case study was performed on the liposomes containing a hydrophilic antiviral drug, Tenofovir. Ishikawa fishbone diagrams were designed to identify the risk and assess the impact on two key quality attributes, drug encapsulation efficiency and particle size. Risk identification and risk analysis were conducted simultaneously for identification of high-risk variables. Liposomes were prepared using thin film hydration method. The authors concluded that liposome preparation process has a large impact on drug encapsulation efficiency with significant variability. However, an appropriate process design can provide a homogenous system with uniform particle size distribution as well as drug encapsulation efficiency.21
In another study researchers developed liposomes loaded with (−)-epigallocatechin gallate (EGCG) using the Quality by Design approach. Risk assessment tools identified three key formulation factors (phospholipid concentration, phospholipid to cholesterol molar ratio, and EGCG concentration) likely to influence the critical quality attributes of EGCG-containing liposomes. These factors were studied using a D-optimal experimental design. Authors noted all three formulation factors significantly influenced liposome CQAs.96
6. Scientific and regulatory considerations
The lack of a clear definition for nanotechnology continues to be a major concern. Nanotechnology can be regarded as an umbrella term covering processes, products, and characteristics at micro/nanoscale. A definition cited by the US National Nanotechnology Initiative proposed in 1990 states that the size ranges from ‘about 1 to 100 nm’. This definition excludes the size ranges in micrometers and less than 1 nm, a scale widely used by nanoscientists. Guidelines from regulatory agencies about liposomal technology are ambivalent and dependent on the first-in-class product field.97,98 Liposomes comprise several formulation components, and the applicant needs to perform physicochemical stability and toxicological studies of the individual components. Further, these studies should also be conducted in combination with other elements.14,59,99
US FDA has published a guidance, ‘Liposome Drug Products: Chemistry, Manufacturing, and Controls; Human Pharmacokinetics and Bioavailability; and Labeling Documentation’ (April 2018). This document covers the following sections: (A) chemistry, manufacturing, and controls (CMC); (B) Bioavailability and clinical PK studies or, in the case of an ANDA, bioequivalence; and (C) labeling in NDAs (New Drug Applications) and ANDAs (Abbreviated New Drug Applications). The introduction of the first successful liposomal formulation to the US market was Doxil® indicated for the treatment of AIDS-related Kaposi's sarcoma and ovarian cancer. It was the first liposome product to receive regulatory approval in the nano-size range. Later, DuanoXome® and Ambiosome®, containing active ingredients daunorubicin and amphotericin B, received approvals in 1996 and 1997 for the treatment of HIV-associated Kaposi's sarcoma and fungal infections, respectively.100 The first US Amphotericin B generic liposomal products were approved in 2021 (Sun Pharma Industries LTD) and 2022 (Eugia Pharma Specialities LTD). FDA has also published product-specific guidance for liposomal products, such as Draft Guidance on Amphotericin B (Recommended Apr 2014; Revised Jan 2016, Aug 2020) and Draft Guidance for Doxorubicin Hydrochloride (Recommended Feb 2010; Revised Nov 2013, Dec 2014, Apr 2017, Sep 2018, May 2022).
These product-specific guidances represent the agency's thinking to demonstrate bioequivalence. For example, the draft guidance for the active ingredient doxorubicin hydrochloride administered via liposomal injection recommends one in vitro liposome size distribution study and one in vivo study to demonstrate bioequivalence. Details about the in vitro and in vivo studies are stated in the product-specific guidance.
Table 1 below describes some FDA-approved parenteral injectable liposomal products.
Table 1 FDA-approved parenteral injectable liposomal products
Proprietary name |
Sponsor |
Active ingredient |
Indication |
US approval year |
Doxil® |
Baxter Healthcare CORP |
Doxorubicin |
Ovarian cancer, Kaposi's sarcoma |
1995 |
Ambiosome® |
Astellas Pharma US Inc. |
Amphotericin B |
Anti-fungal |
1997 |
Marqibo® |
Acrotech Biopharma LLC |
Vincristine sulfate |
Anti-cancer |
2012 |
Abelcet® |
Leadiant Biosciences Inc. |
Amphotericin B |
Anti-fungal |
1995 |
DepoDur® |
Pacira Pharmaceuticals Inc. |
Morphine sulfate |
Pain management |
2004 |
Exparel® |
Pacira Pharmaceuticals Inc. |
Bupivacaine |
Local anesthetic |
2011 |
Onivyde® |
Ipsen Biopharmaceuticals Inc. |
Irinotecan hydrochloride |
Anti-cancer |
2015 |
Arikayce Kit® |
Insmed Inc. |
Amikacin sulfate |
Anti-cancer |
2018 |
Vyxeos® |
Celator Pharmaceuticals Inc. |
Cytarabine and daunorubicin |
Anti-cancer |
2017 |
Onpattro® |
Alnylam Pharmaceuticals Inc. |
Patisiran sodium |
Polyneuropathy of hereditary transthyretin-mediated amyloidosis |
2018 |
The FDA's ongoing efforts are crucial to align technological advancements and drug product complexity with established guidelines. Specific guidance and a Quality by Design strategy are essential for developing lyophilized liposomes and biological liposomal formulations.
7. Conclusions and future perspectives
In conclusion, the Quality by Design approach is a game-changing strategy for developing pharmaceutical liposomes as nano-sized parenteral drug delivery systems. By applying QbD principles from the initial stages of formulation and process development, researchers can achieve a more systematic and predictive method for producing robust, high-quality liposomal products. The sub-micron and nano drug delivery systems discussed in this article demonstrate significant potential for delivering a wide range of hydrophilic, lipophilic, and amphiphilic drugs, including both small and large molecules. These systems are well-established and increasingly utilized, with the parenteral route being the preferred choice in clinical settings. While QbD approaches are well-suited for liposomal development, continued efforts by regulatory authorities are essential to ensure liposomal products’ stability, safety, and efficacy.
Author contributions
Conceptualization, P.A. and S.A.A.R.; writing – original draft preparation, P.A. and S.A.A.R.; writing – review and editing, P.A. and S.A.A.R. Both P.A. and S.A.A.R contributed equally to this manuscript. All authors have read and agreed to the published version of the manuscript.
Data availability
No primary research results, software, or code has been included, and no new data were generated or analyzed as part of this review.
Conflicts of interest
The authors declare no conflict of interest.
Acknowledgements
None.
References
- S. A. Rizvi and A. M. Saleh, Applications of nanoparticle systems in drug delivery technology, Saudi Pharm. J., 2018, 26(1), 64–70 CrossRef PubMed.
-
T. Shukla, N. Upmanyu, S. Prakash Pandey and D. Gosh, Lipid nanocarriers, lipid nanocarriers for drug targeting, Elsevier, 2018, pp. 1–47 Search PubMed.
- P. Prabhakar and M. Banerjee, Nanotechnology in drug delivery system: challenges and opportunities, J. Pharm. Sci. Res., 2020, 12(4), 492–498 CAS.
-
Y. Shahzad, S. A. A. Rizvi, A. M. Yousaf and T. Hussain, Drug delivery using nanomaterials, CRC Press, 2022 Search PubMed.
- A. B. Sairam, A. Sanmugam, A. Pushparaj, G. Mahesh Kumar, N. Sundarapandian, S. Balaji, M. Nallal and K. H. Park, Toxicity of polymeric nanodrugs as drug carriers, ACS Chem. Health Saf., 2023, 30(5), 236–250 CrossRef CAS.
- C. C. Chen, T. H. Tsai, Z. R. Huang and J. Y. Fang, Effects of lipophilic emulsifiers on the oral administration of lovastatin from nanostructured lipid carriers: physicochemical characterization and pharmacokinetics, Eur. J. Pharm. Biopharm., 2010, 74(3), 474–482 CrossRef CAS.
- M. Laura Immordino, F. Dosio and L. Cattel, Stealth liposomes: review of the basic science, rationale, and clinical applications, existing and potential, Int. J. Nanomed., 2006, 1(3), 297–315 CrossRef.
- R. Jangde and D. Singh, Preparation and optimization of quercetin-loaded liposomes for wound healing, using response surface methodology, Artif. Cells, Nanomed., Biotechnol., 2016, 44(2), 635–641 CrossRef CAS PubMed.
- D. Marquardt, F. A. Heberle, T. Miti, B. Eicher, E. London, J. Katsaras and G. Pabst,
1H NMR shows slow phospholipid flip-flop in gel and fluid bilayers, Langmuir, 2017, 33(15), 3731–3741 CrossRef CAS PubMed.
- Z. Wang, W. Li, Y. Jiang, J. Park, K. M. Gonzalez, X. Wu, Q. Y. Zhang and J. Lu, Cholesterol-modified sphingomyelin chimeric lipid bilayer for improved therapeutic delivery, Nat. Commun., 2024, 15(1), 2073 CrossRef CAS PubMed.
- S. Pande, Factors affecting response variables with emphasis on drug release and loading for optimization of liposomes, Artif. Cells, Nanomed., Biotechnol., 2024, 52(1), 334–344 CrossRef CAS PubMed.
- H. Nsairat, D. Khater, U. Sayed, F. Odeh, A. Al Bawab and W. Alshaer, Liposomes: Structure, composition, types, and clinical applications, Heliyon, 2022, 8(5), e09394 CrossRef CAS.
- L. van der Koog, T. B. Gandek and A. Nagelkerke, Liposomes and extracellular vesicles as drug delivery systems: A comparison of composition, pharmacokinetics, and functionalization, Adv. Healthcare Mater., 2022, 11(5), 2100639 CrossRef CAS PubMed.
- B. S. Pattni, V. V. Chupin and V. P. Torchilin, New developments in liposomal drug delivery, Chem. Rev., 2015, 115(19), 10938–10966 CrossRef CAS.
- A. A. Yaroslavov, A. V. Sybachin, E. Kesselman, J. Schmidt, Y. Talmon, S. A. Rizvi and F. M. Menger, Liposome fusion rates depend upon the conformation of polycation catalysts, J. Am. Chem. Soc., 2011, 133(9), 2881–2883 CrossRef CAS PubMed.
- D. Cipolla, H. Wu, I. Gonda, S. Eastman, T. Redelmeier and H. K. Chan, Modifying the release properties of liposomes toward personalized medicine, J. Pharm. Sci., 2014, 103(6), 1851–1862 CrossRef CAS PubMed.
- S. Dadashzadeh, N. Mirahmadi, M. H. Babaei and A. M. Vali, Peritoneal retention of liposomes: Effects of lipid composition, PEG coating and liposome charge, J. Controlled Release, 2010, 148(2), 177–186 CrossRef CAS PubMed.
- L. X. Yu, G. Amidon, M. A. Khan, S. W. Hoag, J. Polli, G. K. Raju and J. Woodcock, Understanding pharmaceutical quality by design, AAPS J., 2014, 16, 771–783 CrossRef CAS.
- S. Swain, R. Parhi, B. R. Jena and S. M. Babu, Quality by design: concept to applications, Curr. Drug Discovery Technol., 2019, 16(3), 240–250 CrossRef CAS.
- C. C. Vieira, L. Peltonen, A. P. Karttunen and A. J. Ribeiro, Is it advantageous to use quality by design (QbD) to develop nanoparticle-based dosage forms for parenteral drug administration?, Int. J. Pharm., 2024, 124163 CrossRef.
- X. Xu, M. A. Khan and D. J. Burgess, A quality by design (QbD) case study on liposomes containing hydrophilic API: I. Formulation, processing design and risk assessment, Int. J. Pharm., 2011, 419(1–2), 52–59 CrossRef CAS.
- International Conference on Harmonisation of Technical Requirements for Registration of Pharmaceuticals for Human Use (ICH) guideline Q9 (R1) on quality risk management – Step 5 – Revision 1, European Medicines Agency, Effective: 26/07/2023.
- W. Alshaer, H. Nsairat, Z. Lafi, O. M. Hourani, A. Al-Kadash, E. Esawi and A. M. Alkilany, Quality by design approach in liposomal formulations: Robust product development, Molecules, 2022, 28(1), 10 CrossRef.
- A. Porfire, M. Achim, C. Barbalata, I. Rus, I. Tomuta and C. Cristea, Pharmaceutical Development of Liposomes Using the QbD Approach, Liposomes Adv. Perspect., 2019, 1–20 Search PubMed.
- M. R. Mozafari, Liposomes: an overview of manufacturing techniques, Cell. Mol. Biol. Lett., 2005, 10(4), 711 CAS.
- A. Wagner and K. Vorauer-Uhl, Liposome technology for industrial purposes, J. Drug Delivery, 2011, 2011(1), 591325 Search PubMed.
- D. Lombardo and M. A. Kiselev, Methods of liposomes preparation: formation and control factors of versatile nanocarriers for biomedical and nanomedicine application, Pharmaceutics, 2022, 14(3), 543 CrossRef CAS.
- L. P. Kolluru, P. Atre and S. A. Rizvi, Characterization and applications of colloidal systems as versatile drug delivery carriers for parenteral formulations, Pharmaceuticals, 2021, 14(2), 108 CrossRef CAS.
- M. R. Danaei, M. Dehghankhold, S. Ataei, F. Hasanzadeh Davarani, R. Javanmard, A. Dokhani, S. Khorasani and M. R. Mozafari, Impact of particle size and polydispersity index on the clinical applications of lipidic nanocarrier systems, Pharmaceutics, 2018, 10(2), 57 CrossRef.
- T. Provder, Challenges in particle size distribution measurement past, present and for the 21st century, Prog. Org. Coat., 1997, 32(1–4), 143–153 CrossRef CAS.
- Q. Wang and Y. Chao, Multifunctional quantum dots and liposome complexes in drug delivery, J. Biomed. Res., 2018, 32(2), 91 CrossRef CAS.
- J. Kuntsche, J. C. Horst and H. Bunjes, Cryogenic transmission electron microscopy (cryo-TEM) for studying the morphology of colloidal drug delivery systems, Int. J. Pharm., 2011, 417(1–2), 120–137 CrossRef CAS PubMed.
- T. Li, C. J. Nowell, D. Cipolla, T. Rades and B. J. Boyd, Direct comparison of standard transmission electron microscopy and cryogenic-TEM in imaging nanocrystals inside liposomes, Mol. Pharm., 2019, 16(4), 1775–1781 CrossRef CAS.
- N. A. Koifman and Y. Talmon, Cryogenic electron microscopy methodologies as analytical tools for the study of self-assembled pharmaceutics, Pharmaceutics, 2021, 13(7), 1015 CrossRef CAS.
- R. Binaymotlagh, F. Hajareh Haghighi, L. Chronopoulou and C. Palocci, Liposome–Hydrogel Composites for Controlled Drug Delivery Applications, Gels, 2024, 10(4), 284 CrossRef CAS.
- K. A. Edwards and A. J. Baeumner, Analysis of liposomes, Talanta, 2006, 28;68(5), 1432–1441 CrossRef PubMed.
- M. K. Rasmussen, J. N. Pedersen and R. Marie, Size and surface charge characterization of nanoparticles with a salt gradient, Nat. Commun., 2020, 11(1), 2337 CrossRef CAS.
- Z. Németh, I. Csóka, R. Semnani Jazani, B. Sipos, H. Haspel, G. Kozma, Z. Kónya and D. G. Dobó, Quality by design-driven zeta potential optimisation study of liposomes with charge imparting membrane additives, Pharmaceutics, 2022, 14(9), 1798 CrossRef.
- M. Qiang, X. Pang, D. Ma, C. Ma and F. Liu, Effect of Membrane Surface Modification Using Chitosan Hydrochloride and Lactoferrin on the Properties of Astaxanthin-Loaded Liposomes, Molecules, 2020, 25(3), 610 CrossRef CAS PubMed.
- M. J. Carrasco, S. Alishetty, M. G. Alameh, H. Said, L. Wright, M. Paige, O. Soliman, D. Weissman, T. E. Cleveland IV, A. Grishaev and M. D. Buschmann, Ionization and structural properties of mRNA lipid nanoparticles influence expression in intramuscular and intravascular administration, Commun. Biol., 2021, 4(1), 956 CrossRef CAS.
- K. L. Delma, A. Lechanteur, B. Evrard, R. Semdé and G. Piel, Sterilization methods of liposomes: Drawbacks of conventional methods and perspectives, Int. J. Pharm., 2021, 597, 120271 CrossRef CAS PubMed.
- M. R. Toh and G. N. Chiu, Liposomes as sterile preparations and limitations of sterilisation techniques in liposomal manufacturing, Asian J. Pharm. Sci., 2013, 8(2), 88–95 CrossRef CAS.
- S. Pande, Liposomes for drug delivery: review of vesicular composition, factors affecting drug release and drug loading in liposomes, Artif. Cells, Nanomed., Biotechnol., 2023, 51(1), 428–440 CrossRef CAS.
- P. Liu, G. Chen and J. Zhang, A review of liposomes as a drug delivery system: current status of approved products, regulatory environments, and future perspectives, Molecules, 2022, 27(4), 1372 CrossRef CAS.
- L. W. Allahou, S. Y. Madani and A. Seifalian, Investigating the application of liposomes as drug delivery systems for the diagnosis and treatment of cancer, Int. J. Biomater., 2021, 2021(1), 3041969 Search PubMed.
- S. Paramshetti, M. Angolkar, S. Talath, R. A. Osmani, A. Spandana, A. Al Fatease, U. Hani, K. V. Ramesh and E. Singh, Unravelling the in vivo dynamics of liposomes: Insights into biodistribution and cellular membrane interactions, Life Sci., 2024, 122616 CrossRef CAS.
- J. Chen, S. Hu, M. Sun, J. Shi, H. Zhang, H. Yu and Z. Yang, Recent advances and clinical translation of liposomal delivery systems in cancer therapy, Eur. J. Pharm. Sci., 2024, 106688 CrossRef CAS.
- D. C. Drummond, O. Meyer, K. Hong, D. B. Kirpotin and D. Papahadjopoulos, Optimizing liposomes for delivery of chemotherapeutic agents to solid tumors, Pharmacol. Rev., 1999, 51(4), 691–744 CAS.
- H. B. Haroon, A. C. Hunter, Z. S. Farhangrazi and S. M. Moghimi, A brief history of long circulating nanoparticles, Adv. Drug Delivery Rev., 2022, 188, 114396 CrossRef CAS.
- S. Deodhar and A. K. Dash, Long circulating liposomes: challenges and opportunities, Ther. Delivery, 2018, 9(12), 857–872 CrossRef CAS PubMed.
- C. Shi, H. Cao, W. He, F. Gao, Y. Liu and L. Yin, Novel drug delivery liposomes targeted with a fully human anti-VEGF165 monoclonal antibody show superior antitumor efficacy in vivo, Biomed. Pharmacother., 2015, 73, 48–57 CrossRef CAS.
- K. Aloss and P. Hamar, Recent preclinical and clinical progress in liposomal doxorubicin, Pharmaceutics, 2023, 15(3), 893 CrossRef CAS.
- M. Amiri, T. Gholami, O. Amiri, A. Pardakhti, M. Ahmadi, A. Akbari, A. Amanatfard and M. Salavati-Niasari, The magnetic inorganic-organic nanocomposite based on ZnFe2O4-Imatinib-liposome for biomedical applications, in vivo and in vitro study, J. Alloys Compd., 2020, 849, 156604 CrossRef CAS.
- V. Torchilin, Antibody-modified liposomes for cancer chemotherapy, Expert Opin. Drug Delivery, 2008, 5(9), 1003–1025 CrossRef CAS PubMed.
- E. Moghimipour, M. Rezaei, Z. Ramezani, M. Kouchak, M. Amini, K. A. Angali, F. A. Dorkoosh and S. Handali, Folic acid-modified liposomal drug delivery strategy for tumor targeting of 5-fluorouracil, Eur. J. Pharm. Sci., 2018, 114, 166–174 CrossRef CAS PubMed.
- M. T. Luiz, J. A. Dutra, T. de Cássia Ribeiro, G. C. Carvalho, R. M. Sábio, J. M. Marchetti and M. Chorilli, Folic acid-modified curcumin-loaded liposomes for breast cancer therapy, Colloids Surf., A, 2022, 645, 128935 CrossRef CAS.
- M. A. Farooq and N. L. Trevaskis, TPGS decorated liposomes as multifunctional nano-delivery systems, Pharm. Res., 2023, 40(1), 245–263 CrossRef CAS.
- G. F. Boafo, K. T. Magar, M. D. Ekpo, W. Qian, S. Tan and C. Chen, The role of cryoprotective agents in liposome stabilization and preservation, Int. J. Mol. Sci., 2022, 23(20), 12487 CrossRef CAS.
- Y. Wang and D. W. Grainger, Lyophilized liposome-based parenteral drug development: Reviewing complex product design strategies and current regulatory environments, Adv. Drug Delivery Rev., 2019, 151, 56–71 CrossRef.
- S. Ohtake, Y. Kita and T. Arakawa, Interactions of formulation excipients with proteins in solution and in the dried state, Adv. Drug Delivery Rev., 2011, 63(13), 1053–1073 CrossRef CAS.
- J. J. Sonju, P. Shrestha, A. Dahal, X. Gu, W. D. Johnson, D. Zhang, C. M. Muthumula, S. A. Meyer, G. Mattheolabakis and S. D. Jois, Lyophilized liposomal formulation of a peptidomimetic-Dox conjugate for HER2 positive breast and lung cancer, Int. J. Pharm., 2023, 639, 122950 CrossRef CAS PubMed.
- M. Okumura, H. Ichihara and Y. Matsumoto, Hybrid liposomes showing enhanced accumulation in tumors as theranostic agents in the orthotopic graft model mouse of colorectal cancer, Drug Delivery, 2018, 25(1), 1192–1199 CrossRef CAS PubMed.
- S. Tan, X. Li, Y. Guo and Z. Zhang, Lipid-enveloped hybrid nanoparticles for drug delivery, Nanoscale, 2013, 5(3), 860–872 RSC.
- J. F. Le Meins, C. Schatz, S. Lecommandoux and O. Sandre, Hybrid polymer/lipid vesicles: state of the art and future perspectives, Mater. Today, 2013, 16(10), 397–402 CrossRef CAS.
- S. Sur, A. C. Fries, K. W. Kinzler, S. Zhou and B. Vogelstein, Remote loading of preencapsulated drugs into stealth liposomes, Proc. Natl. Acad. Sci. U. S. A., 2014, 111(6), 2283–2288 CrossRef CAS PubMed.
- A. Liu, G. Yang, Y. Liu and T. Liu, Research progress in membrane fusion-based hybrid exosomes for drug delivery systems, Front. Bioeng. Biotechnol., 2022, 10, 939441 CrossRef.
- D. N. Moholkar, R. Kandimalla, R. C. Gupta and F. Aqil, Advances in lipid-based carriers for cancer therapeutics: Liposomes, exosomes and hybrid exosomes, Cancer Lett., 2023, 565, 216220 CrossRef CAS.
- Z. Zhao, X. Ma, R. Zhang, F. Hu, T. Zhang, Y. Liu, M. H. Han, F. You, Y. Yang and W. Zheng, A novel liposome-polymer hybrid nanoparticles delivering a multi-epitope self-replication DNA vaccine and its preliminary immune evaluation in experimental animals, Nanomedicine, 2021, 35, 102338 CrossRef CAS PubMed.
- T. I. Wi, J. E. Won, C. M. Lee, J. W. Lee, T. H. Kang, B. C. Shin, H. D. Han and Y. M. Park, Efficacy of combination therapy with linalool and doxorubicin encapsulated by liposomes as a two-in-one hybrid carrier system for epithelial ovarian carcinoma, Int. J. Nanomed., 2020, 8427–8436 CrossRef CAS.
- Y. Eygeris, M. Gupta, J. Kim and G. Sahay, Chemistry of lipid nanoparticles for RNA delivery, Acc. Chem. Res., 2021, 55(1), 2–12 CrossRef PubMed.
- B. Karunakaran, R. Gupta, P. Patel, S. Salave, A. Sharma, D. Desai, D. Benival and N. Kommineni, Emerging trends in lipid-based vaccine delivery: a special focus on developmental strategies, fabrication methods, and applications, Vaccines, 2023, 11(3), 661 CrossRef CAS.
- T. Unruh, K. Götz, C. Vogel, E. Fröhlich, A. Scheurer, L. Porcar and F. Steiniger, Mesoscopic Structure of Lipid Nanoparticle Formulations for mRNA Drug Delivery: Comirnaty and Drug-Free Dispersions, ACS Nano, 2024, 18(13), 9746–9764 CrossRef CAS.
- T. Iyoda, S. Yamasaki, M. Kawamura, M. Ueda, K. Son, Y. Ito, K. Shimizu and S. I. Fujii, Optimal therapeutic strategy using antigen–containing liposomes selectively delivered to antigen–presenting cells, Cancer Sci., 2019, 110(3), 875–887 CrossRef CAS.
- M. J. Hussain, A. Wilkinson, V. W. Bramwell, D. Christensen and Y. Perrie, Th1 immune responses can be modulated by varying dimethyldioctadecylammonium and distearoyl-sn-glycero-3-phosphocholine content in liposomal adjuvants, J. Pharm. Pharmacol., 2014, 66(3), 358–366 CrossRef CAS PubMed.
- H. Ali, M. Akbar and B. Iqbal,
et al., Virosome: An engineered virus for vaccine delivery, Saudi Pharm. J., 2023, 31(5), 752–764 CrossRef CAS PubMed.
- G. Koopman, M. Amacker and T. Stegmann,
et al., A low dose of RBD and TLR7/8 agonist displayed on influenza virosome particles protects rhesus macaque against SARS-CoV-2 challenge, Sci. Rep., 2023, 13(1), 5074 CrossRef CAS PubMed.
- P. B. Santhosh and J. Genova, Archaeosomes: New Generation of Liposomes Based on Archaeal Lipids for Drug Delivery and Biomedical Applications, ACS Omega, 2022, 8(1), 1–9 CrossRef PubMed.
- E. L. Romero and M. J. Morilla, Ether lipids from archaeas in nano-drug delivery and vaccination, Int. J. Pharm., 2023, 634, 122632 CrossRef CAS PubMed.
- R. A. Schwendener, Liposomes as vaccine delivery systems: a review of the recent advances, Ther. Adv. Vaccines, 2014, 2(6), 159–182 CrossRef CAS PubMed.
- H. Ali, M. Akbar, B. Iqbal, F. Ali, N. K. Sharma, N. Kumar, A. Najmi, M. Albratty, H. A. Alhazmi, O. A. Madkhali and K. Zoghebi, Virosome: An engineered virus for vaccine delivery, Saudi Pharm. J., 2023, 31(5), 752–764 CrossRef CAS PubMed.
- Y. He, W. Zhang, Q. Xiao, L. Fan, D. Huang, W. Chen and W. He, Liposomes and liposome-like nanoparticles: From anti-fungal infection to the COVID-19 pandemic treatment, Asian J. Pharm. Sci., 2022, 17(6), 817–837 CrossRef.
- F. Pedro, F. Veiga and F. Mascarenhas-Melo, Impact of GAMP 5, data integrity and QbD on quality assurance in the pharmaceutical industry: How obvious is it?, Drug Discovery Today, 2023, 103759 CrossRef CAS.
- P. Yogendrarajah, L. Natalis, W. Peys, M. Lenaerts, P. Lebrun, J. P. Boon and M. Hellings, Application of design space and quality by design methodologies combined with ultra high-performance liquid chromatography for the optimization of the sample preparation of complex pharmaceutical dosage forms, J. Pharm. Biomed. Anal., 2023, 227, 115149 CrossRef CAS.
- D. G. Dobó, Z. Németh, B. Sipos, M. Cseh, E. Pallagi, D. Berkesi, G. Kozma, Z. Kónya and I. Csóka, Pharmaceutical development and design of thermosensitive liposomes based on the QbD approach, Molecules, 2022, 27(5), 1536 CrossRef PubMed.
- D. Kapoor, S. Sharma, K. Verma, A. Bisht, M. Sharma, N. J. Singhai, N. Raval and R. Maheshwari, Quality-by-design-based engineered liposomal nanomedicines to treat cancer: an in-depth analysis, Nanomedicine, 2022, 17(17), 1173–1189 CrossRef CAS.
- G. Yenduri, A. P. Costa, X. Xu and D. J. Burgess, Impact of critical process parameters and critical material attributes on the critical quality attributes of liposomal formulations prepared using continuous processing, Int. J. Pharm., 2022, 619, 121700 CrossRef CAS PubMed.
- D. M. Zagalo, J. Sousa and S. Simões, Quality by design (QbD) approach in marketing authorization procedures of non-biological complex drugs: a critical evaluation, Eur. J. Pharm. Biopharm., 2022, 178, 1–24 CrossRef CAS PubMed.
- M. T. Luiz, J. S. Viegas, J. P. Abriata, F. Viegas, F. T. de Carvalho Vicentini, M. V. Bentley, M. Chorilli, J. M. Marchetti and D. R. Tapia-Blacido, Design of experiments (DoE) to develop and to optimize nanoparticles as drug delivery systems, Eur. J. Pharm. Biopharm., 2021, 165, 127–148 CrossRef.
- I. Csóka, R. Ismail, O. Jójárt-Laczkovich and E. Pallagi, Regulatory considerations, challenges and risk-based approach in nanomedicine development, Curr. Med. Chem., 2021, 28(36), 7461–7476 CrossRef PubMed.
- S. Cunha, C. P. Costa, J. N. Moreira, J. M. Lobo and A. C. Silva, Using the quality by design (QbD) approach to optimize formulations of lipid nanoparticles and nanoemulsions: A review, Nanomedicine, 2020, 28, 102206 CrossRef CAS PubMed.
- H. B. Grangeia, C. Silva, S. P. Simões and M. S. Reis, Quality by design in pharmaceutical manufacturing: A systematic review of current status, challenges and future perspectives, Eur. J. Pharm. Biopharm., 2020, 147, 19–37 CrossRef CAS PubMed.
- E. Kajiwara, H. Kamizato and M. Shikano, Impact of Quality by Design development on the review period of new drug approval and product quality in Japan, Ther. Innovation Regul. Sci., 2020, 54, 1192–1198 CrossRef PubMed.
- M. Rawal, A. Singh and M. M. Amiji, Quality-by-design concepts to improve nanotechnology-based drug development, Pharm. Res., 2019, 36(11), 153 CrossRef.
- P. Kesharwani, S. Md, N. A. Alhakamy, K. M. Hosny and A. Haque, QbD enabled azacitidine loaded liposomal nanoformulation and its in vitro evaluation, Polymers, 2021, 13(2), 250 CrossRef CAS.
- B. Lu, Q. Ma, J. Zhang, R. Liu, Z. Yue, C. Xu, Z. Li and H. Lin, Preparation and characterization of bupivacaine multivesicular liposome: A QbD study about the effects of formulation and process on critical quality attributes, Int. J. Pharm., 2021, 598, 120335 CrossRef CAS PubMed.
- C. I. Barbălată, I. Tomuţă, M. Achim, A. B. Boşca, G. Cherecheş, O. Soriţău and A. S. Porfire, Application of the QbD Approach in the Development of a Liposomal Formulation with EGCG, J. Pharm. Innovation, 2022, 17(3), 867–880 CrossRef.
- Y. Wang and D. W. Grainger, Regulatory considerations specific to liposome drug development as complex drug products, Front. Drug Delivery, 2022, 2, 901281 CrossRef.
- M. Mehta, T. A. Bui, X. Yang, Y. Aksoy, E. M. Goldys and W. Deng, Lipid-based nanoparticles for drug/gene delivery: An overview of the production techniques and difficulties encountered in their industrial development, ACS Mater. Au, 2023, 3(6), 600–619 CrossRef CAS PubMed.
- R. Bawa, FDA and nanotech: baby steps lead to regulatory uncertainty, Bio-Nanotechnol., 2013, 720–732 Search PubMed.
- U. Bulbake, S. Doppalapudi, N. Kommineni and W. Khan, Liposomal formulations in clinical use: an updated review, Pharmaceutics, 2017, 9(2), 12 CrossRef PubMed.
|
This journal is © The Royal Society of Chemistry 2024 |
Click here to see how this site uses Cookies. View our privacy policy here.