DOI:
10.1039/D4PM00210E
(Paper)
RSC Pharm., 2024,
1, 1055-1065
Effect of fluorine substituents in 4-(1-benzyl-1H-benzo[d]imidazol-2-yl)thiazole for the study of antiparasitic treatment of cysticercosis on a Taenia crassiceps model†
Received
13th July 2024
, Accepted 28th October 2024
First published on 1st November 2024
Abstract
This work details the synthesis of five N-benzylated derivatives of thiabendazoles (L1–L5), four of which were previously unreported in the literature (L2–L5). The compounds were characterised using a comprehensive array of spectroscopic (FT-IR, 1H, 13C{1H}, and 19F{1H} NMR), spectrometric (MS-EI+) and diffractometric (SC-DRX) techniques. To evaluate the effect of increased fluorine substituents in the N-benzyl fragment, we conducted a parasitotoxic activity assay, testing the compounds at various concentrations of unhatched Taenia crassiceps cysticerci. The inclusion of the N-benzyl fragment and the increase in fluorine substituents led to an enhancement in the lipophilicity of thiabendazoles.
Introduction
Benzimidazoles are bicyclic heteroaromatic compounds that have attracted the scientific community's attention since their introduction as antiparasitic agents in the 1960s.1,2 These heterocyclic pharmacophores serve as essential scaffolds in designing new molecules with biological activity or clinical application. Benzimidazoles are among the ten most used nitrogenous heterocycles in medicines approved by the U.S. Food and Drug Administration (FDA), both in human and animal medicine, due to their notable pharmacological properties.3,4 These compounds are capable of accepting or donating protons, allowing the formation of weak interactions, which promotes the union of the therapeutic targets with these heterocycles. Structure–activity relationship (SAR) analyses indicate that the placement of substituents in the N1, C2, C5 or C6 positions influences their therapeutic activity.5,6 Modifications in benzimidazoles affect their binding capacity with proteins since new weak interactions and new electronic and steric effects arise, which alter their ability to recognise specific enzymes and modify their pharmacological potential.7,8 These molecules have a structure similar to those of the nitrogenous bases known as purines (adenine and guanine), which facilitates their application in various biological activities, such as analgesic, anticancer, anticoagulant, anticonvulsant, antidiabetic, antihypertensive, antihistaminic, anti-inflammatory, antimalarial, antimicrobial, antioxidant, antipsychotic, antituberculosis, antitumor, antiulcer, antiviral, and contraceptive activities, among others.9–11
One of the best-known benzimidazoles is 4-(1H-benzo[d]imidazol-2-yl)thiazole, commonly called thiabendazole (TBZ). This compound inhibits the helminth-specific mitochondrial enzyme (fumarate reductase), which is why it is used as an anthelmintic agent in animals and humans.12,13 TBZ was the first benzimidazole-based drug approved by the FDA for treating human gastrointestinal parasites. Even so, it was later withdrawn from the market because other anthelmintic agents with better tolerance emerged.14,15 Nonetheless, this drug is still available in many countries and is mainly used in veterinary medicine. TBZ is also used as a fungicide and an anthelmintic in fruits, vegetables, and various food products.16,17 This compound generally has low toxicity; however, the U.S. Environmental Protection Agency (EPA) has classified it as a probable carcinogen at doses high enough to cause disturbances in thyroid hormone balance.18,19
Brown and co-workers (1961) reported the preparation of 4-(1-benzyl-1H-benzo[d]imidazol-2-yl)thiazole (BZ-TBZ) when searching for a new class of broad-spectrum anthelmintic agents against gastrointestinal parasites of domestic animals. For this, TBZ was functionalised in the N1 position using benzoyl iodide.20 Several studies have been reported on synthesising and characterising this TBZ derivative.21–23 Even SAR experimental studies have been reported on its antimicrobial, anti-inflammatory and antiangiogenic activities, and as a vascular disruptor and methionine aminopeptidase inhibitor.24–29
On the other hand, Taenia crassiceps is a cestode found in wild canids and rodents. This parasite presents metabolic and antigenic similarities to different species of the Taeniidae family, such as Taenia solium, which makes it useful as an experimental model in cysticercosis studies.30,31 Human cysticercosis caused by Taenia crassiceps is unusual; however, isolated cases have been reported in immunocompromised and immunocompetent patients.32,33 One of the primary drugs used in the treatment of this disease is albendazole (ABZ), a derivative of benzimidazole. Nonetheless, parasite resistance to this drug has been reported, which has motivated the search for new alternatives for treating cysticercosis.34,35 Thus, developing new benzimidazole derivatives aims to increase antiparasitic activity by slightly modifying traditional anthelmintics.36,37 New compounds have been synthesised from 2-(trifluoromethyl)-1H-benzo[d]imidazole, presenting improved antiparasitic activity. For instance, 6-chloro-5-(2,3-dichlorophenoxy)-2-(trifluoromethyl)-1H-benzo[d]imidazole (RCB15) and 6-chloro-5-(naphthalen-1-yloxy)-2-(trifluoromethyl)-1H-benzo[d]imidazole (RCB20) have been chosen as promising molecules for the treatment of this disease, Scheme 1. These fluorinated benzimidazole derivatives had a greater effect on Taenia crassiceps cysticerci than ABZ and albendazole sulfoxide (ABZ-SO).38,39 Therefore, this work reports the synthesis and characterisation of BZ-TBZ derivatives with different degrees of fluorination in the N-benzylic arm to study their effect on antiparasitic activity.
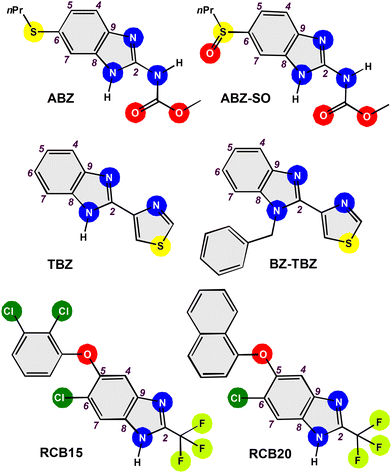 |
| Scheme 1 Benzimidazole derivatives with anticysticercosis activity. | |
Materials and methods
Experimental details
All starting materials were purchased from commercial suppliers and used without further purification. The reagents employed were thiabendazole, sodium hydride 90%, benzyl bromide 98%, 3-fluorobenzyl chloride 96%, potassium iodide 99%, 2,3,6-trifluorobenzyl bromide 97%, 2,3,4,5,6-pentafluorobenzyl bromide 99%, and 4-(trifluoromethyl)benzyl bromide 98%. Melting points were determined directly using a Fisherbrand™ digital apparatus (open capillary). Infrared spectra were obtained using an FT-IR Bruker Alpha ATR spectrophotometer. The 1H, 13C{1H} and 19F{1H} NMR experiments were carried out by using Bruker Avance III 300 MHz equipment using CDCl3 in 5 mm tubes, and subsequently, the spectra were processed using MestReNova© software. Chemical shift values (δ) are reported in parts per million (ppm) relative to the internal reference (TMS). Mass spectra were recorded using a Jeol JSM-SX102A spectrometer at 10 kV in the EI+ mode. The colourless prismatic crystals were placed in a Bruker Smart Apex II X-ray diffractometer with a molybdenum X-ray source (λ = 0.71073 Å). The structures were solved and refined using Patterson's methods with the programs SHELXS-2014/7 and SHELXL-2019/2.40 Molecular structures and supramolecular arrangements were analysed using Mercury© software. Additional information on structural data collection, resolution, and refinement can be found in the ESI.† The three structural files were deposited at the Cambridge Crystallographic Data Center (CCDC). The assigned deposit numbers for L1, L2, and L4 were 2350921, 2350919, and 2350920,† respectively. Table 1 contains the crystallographic parameters, and Tables S1–S3† contain the distances and bond angles.
Table 1 Crystal data and structure refinement for L1, L2 and L4
|
L1
|
L2
|
L4
|
Empirical formula |
C17H13N3S |
C17H12FN3S |
C17H8F5N3S |
Formula weight |
291.36 |
309.36 |
381.32 |
Temperature |
298(2) K |
298(2) K |
298(2) K |
Wavelength |
0.71073 Å |
0.71073 Å |
0.71073 Å |
Monoclinic crystal system |
Monoclinic |
Monoclinic |
Monoclinic |
Space group |
P21/n |
P21/n |
P21/c |
Unit cell dimensions |
a = 17.3372(8), α = 90° |
a = 13.1322(4) Å, α = 90° |
a = 17.173(4) Å, α = 90° |
b = 9.2800(4), β = 113.263(2)° |
b = 6.0454(2) Å, β = 107.2870(10)° |
b = 8.1616(18) Å, β = 93.871(5)° |
c = 19.3163(8), γ = 90° |
c = 19.1953(7) Å, γ = 90° |
c = 10.976(3) Å, γ = 90° |
Volume |
2855.1(2) Å3 |
1455.07(8) Å3 |
1534.8(6) Å3 |
Z
|
8 |
4 |
4 |
Density (calculated) |
1.356 Mg m−3 |
1.412 Mg m−3 |
1.650 Mg m−3 |
Absorption coefficient |
0.223 mm−1 |
0.232 mm−1 |
0.273 mm−1 |
F(000) |
1216 |
640 |
768 |
Crystal size |
0.387 × 0.243 × 0.066 mm3 |
0.462 × 0.167 × 0.090 mm3 |
0.280 × 0.180 × 0.150 mm3 |
Theta range for data collection |
2.028 to 25.328° |
1.673 to 25.390° |
2.377 to 25.472° |
Index ranges |
−20 ≤ h ≤ 20, −11 ≤ k ≤ 10, −23 ≤ l ≤ 22 |
−15 ≤ h ≤ 15, −7 ≤ k ≤ 7, −23 ≤ l ≤ 23 |
−20 ≤ h ≤ 20, −9 ≤ k ≤ 9, −13 ≤ l ≤ 13 |
Reflections collected |
25 355 |
12 341 |
13 161 |
Independent reflections |
5207 [R(int) = 0.0919] |
2674 [R(int) = 0.0421] |
2837 [R(int) = 0.0851] |
Completeness to theta |
25.242°, 99.9% |
25.242°, 100.0% |
25.242°, 100.0% |
Absorption correction |
None |
None |
None |
Refinement method |
Full-matrix least-squares on F2 |
Full-matrix least-squares on F2 |
Full-matrix least-squares on F2 |
Data/restraints/parameters |
5207/0/379 |
2674/0/199 |
2837/608/357 |
Goodness-of-fit on F2 |
0.903 |
0.967 |
0.958 |
Final R indices[I > 2sigma(I)] |
R
1 = 0.0566, wR2 = 0.1353 |
R
1 = 0.0344, wR2 = 0.0791 |
R
1 = 0.0501, wR2 = 0.1121 |
R indices (all data) |
R
1 = 0.1475, wR2 = 0.1725 |
R
1 = 0.0545, wR2 = 0.0885 |
R
1 = 0.1201, wR2 = 0.1392 |
Largest diff. peak and hole |
0.354 and −0.397 e Å−3 |
0.122 and −0.277 e Å−3 |
0.164 and −0.246 e Å−3 |
Synthesis of N-benzylated compounds
The compounds (L1–L5) were synthesised from the deprotonation of thiabendazoles (1 mmol, 0.2013 g) in the presence of sodium hydride (1 mmol, 0.0222 g). Subsequently, the product was reacted with each fluoro-substituted benzyl halide under a nitrogen atmosphere at the reflux of anhydrous tetrahydrofurans for 24 h. L1 and L3–L5 were obtained from fluoro-substituted benzyl bromides, while L2 was obtained from 3-fluorobenzyl chloride and potassium iodide. The reactions were monitored by thin-layer chromatography (TLC) in a dichloromethane–methanol (95
:
5) mixture and developed using a UV lamp. Afterwards, the reaction products were purified by column chromatography and concentrated to dryness using a rotary evaporator connected to a high vacuum. L1, L2 and L4 crystallised from the slow evaporation of a mixture of dichloromethane with acetone.
4-(1-Benzyl-1H-benzo[d]imidazol-2-yl)thiazole (L1).
It was synthesised from benzyl bromide (1 mmol, 0.1745 g). Light brown solid (0.2244 g, 77%). M.p. 146–148 °C. IR (ATR, ν cm−1): 3111 (w), 3076 (w), 2916 (w), 1602 (w), 1450 (m), 1397 (m), 1303 (m), 1283 (w), 901 (m), 880 (m), 830 (m), 734 (s). 1H NMR (300 MHz, CDCl3, δ, ppm): 8.75 (s, 1H), 8.29 (s, 1H), 7.75 (d, 1H), 7.23–7.04 (m, 6H), 5.98 (s, 2H). 13C{1H} NMR (75 MHz, CDCl3, δ, ppm): 153.07, 147.83, 146.96, 143.09, 137.11, 136.01, 128.71, 127.53, 126.74, 123.31, 122.87, 121.38, 119.79, 110.70, 48.54. MS-EI+ (m/z): 291, 214, 91.
4-(1-(3-Fluorobenzyl)-1H-benzo[d]imidazol-2-yl)thiazole (L2).
It was synthesised from 3-fluorobenzyl chloride (1 mmol, 0.1506 g) and potassium iodide (1.5 mmol, 0.2515 g). White solid (0.2413 g, 78%). M.p. 140–142 °C. IR (ATR, ν cm−1): 3122 (w), 3080 (w), 1614 (m), 1586 (m), 1480 (m), 1449 (m), 1437 (m), 1352 (w), 1295 (w), 1253 (w), 1141 (w), 939 (m), 829 (w), 746 (s). 1H NMR (300 MHz, CDCl3, δ, ppm): 8.78 (s, 1H), 8.27 (s, 1H), 7.75 (d, 1H), 7.25–7.09 (m, 4H), 6.86–6.76 (m, 3H), 5.98 (s, 2H). 19F{1H} NMR (282.77 MHz, CDCl3, δ, ppm): −112.46. MS-EI+ (m/z): 309, 308, 214, 195, 135, 109.
4-(1-(2,3,6-Trifluorobenzyl)-1H-benzo[d]imidazol-2-yl)thiazole (L3).
It was synthesised from trifluorobenzyl bromide (1 mmol, 0.2320 g). White solid (0.2590 g, 75%). M.p. 166–168 °C. IR (ATR, ν cm−1): 3130 (w), 3030 (w), 3004 (w), 1493 (s), 1306 (m), 1242 (s), 1037 (m), 879 (w), 812 (m), 733 (s). 1H NMR (300 MHz, CDCl3, δ, ppm): 8.87 (s, 1H), 8.60 (s, 1H), 7.78 (d, 1H), 7.34 (d, 1H), 7.24 (dt, 2H), 6.97 (m, 1H), 6.71 (t, 1H), 6.34 (s, 2H). 19F{1H} NMR (282.77 MHz, CDCl3, δ, ppm): −118.77, −136.53, −141.27. MS-EI+ (m/z): 345, 326, 214, 145.
4-(1-((Perfluorophenyl)methyl)-1H-benzo[d]imidazol-2-yl)thiazole (L4).
It was synthesised from 2,3,4,5,6-pentafluorobenzyl bromide (1 mmol, 0.2636 g). White solid (0.2669 g, 70%). M.p. 150–152 °C. IR (ATR, ν cm−1): 3122 (w), 3070 (w), 3002 (w), 2968 (w), 1657 (w), 1502 (s), 1400 (m), 1306 (m), 1120 (m), 948 (m), 821 (m), 732 (s). 1H NMR (300 MHz, CDCl3, δ, ppm): 8.85 (s, 1H), 8.50 (s, 1H), 7.77 (d, 1H), 7.28–7.19 (m, 3H), 6.33 (s, 2H). 19F{1H} NMR (282.77 MHz, CDCl3, δ, ppm): −141.84, −153.09, −161.02. MS-EI+ (m/z): 381, 362, 303, 214, 181.
4-(1-(4-(Trifluoromethyl)benzyl)-1H-benzo[d]imidazol-2-yl)thiazole (L5).
It was synthesised from 4-(trifluoromethyl)benzyl bromide (1 mmol, 0.2439 g). White solid (0.2803 g, 78%). M.p. 144–146 °C. IR (ATR, ν cm−1): 3119 (w), 1619 (w), 1401 (s), 1100 (s), 1063 (s), 911 (m), 817 (m), 738 (s). 1H NMR (300 MHz, CDCl3, δ, ppm): 8.78 (s, 1H), 8.41 (s, 1H), 7.79 (d, 1H), 7.44 (d, 2H), 7.25 (m, 3H), 7.19 (d, 2H), 6.08 (s, 2H). 13C{1H} NMR (75 MHz, CDCl3, δ, ppm): 153.16, 147.61, 146.79, 143.07, 141.21, 135.77, 126.94, 125.72, 123.54, 123.12, 121.56, 119.95, 110.28, 48.19. 19F{1H} NMR (282.77 MHz, CDCl3, δ, ppm): −62.55. MS-EI+ (m/z): 359, 358, 214, 159.
Parasite viability in vitro
Taenia crassiceps strain Wake Forest University (WFU) cysticerci was obtained from the peritoneum of female BALB/cAnN mice at five months of intraperitoneal infection. Cysticerci were washed with sterile phosphate-buffered saline (PBS) and used for in vitro assays. The effect of parasitotoxic activity of L1–L5 was determined on the viability and integrity of Taenia crassiceps cysticerci. To do this, seven groups of twenty parasites were incubated in microplates using Roswell Park Memorial Institute (RPMI) culture medium at 37 °C in 0.5% CO2 for 24 h and analysed at 3, 6 and 24 h. Viability was evaluated with four different concentrations of the compounds (100, 200, 300, and 500 μM) compared to the same concentrations for the reference drugs, ABZ and TBZ. Subsequently, the parasites were incubated for 1 h in pig bile diluted at a 1
:
3 ratio with RPMI to observe evagination. Viability was estimated by evagination, contractile movements and damage to the bladder wall of the cysticerci, all observed under a Nikon Eclipse TS100 inverted microscope.
Results and discussion
Synthesis and structural characterisation
The N-benzylation reaction, a crucial step in our research, was performed at the N1 position of TBZ. This stage is fundamental in enhancing the lipophilicity and bioavailability of the new compounds, a key aspect of our study. This reaction also allowed the introduction of fluorine substituents on the N-benzylic arm in four of the five compounds. Therefore, L1 and L3–L5 were prepared by one-step synthesis, which consisted of reacting TBZ with different fluorinated benzyl bromides in the presence of sodium hydride under a nitrogen atmosphere. In the case of L2, 3-fluorobenzyl chloride was used in the presence of potassium iodide under the aforementioned conditions, Scheme 2. Most of the compounds recovered as white solids (L2–L5), except for L1, which was light brown. The compounds were obtained with yields between 70 and 78% and showed good solubility in common organic solvents, such as methanol, ethanol, acetone, dichloromethane, chloroform and dimethyl sulfoxide.
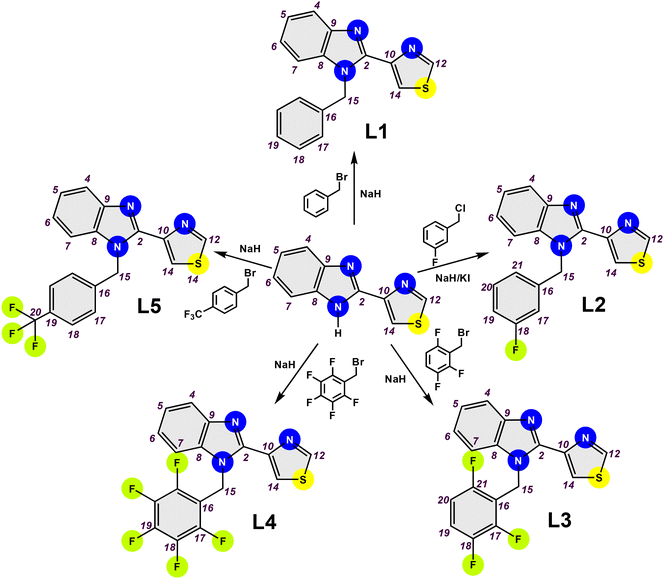 |
| Scheme 2 General procedure for the preparation of fluorinated compounds derived from TBZ. | |
IR spectra provided the first evidence of the formation of the N-benzylated compounds. The absence of the signal due to the C–Br bond (ν = 650–515 cm−1) from benzyl bromides indicated that the reaction had been completed. Besides, the absence of overtones in the spectra of each ligand (ν = 2800–2700 cm−1) is an expected behaviour due to the lack of prototropic tautomerism of benzimidazole. The predictable peaks corresponding to the C
N, C–N and C–S bonds appeared at ν = 1657–1602 cm−1, 1357–1310 cm−1 and 1141–1037 cm−1, respectively, while the signal due to the C–F bond of the fluorinated rings in L2–L4 was detected at ν = 948–911 cm−1. The trifluoromethyl group in L5 was detected at ν = 1100 cm−1. In addition, the bands due to the aromatic C–H bond were observed at ν = 3130–3000 cm−1, Fig. S1–S5.†
1H NMR spectra showed evidence of N-benzylation, with the methylene signal assigned to the range of δ = 6.34–5.98 ppm (H15). For L3 and L4, this same signal shifted to higher frequencies due to the greater number of fluorine substituents on the N-benzylic arm. The signals of thiazole rings appeared in the range of δ = 8.87–8.78 ppm (H12) and δ = 8.60–8.27 ppm (H14). The signals of the benzimidazole rings (H4–H7) and the N-benzyl fragment (H17–H21) overlapped in the aromatic region of δ = 7.80–6.68 ppm. In all cases, the H4 signal corresponds to a doublet in the range of δ = 7.80–7.74 ppm due to the absence of prototropic tautomerism in benzimidazole, Fig. S6–S10.†
In the 13C{1H} NMR spectra of L1 and L5, the methylene carbon (C15) signal was assigned at 48.5 ppm for L1 and 48.2 ppm for L5. The most displaced carbon in both compounds was the thiazole carbon (C12) due to its proximity to the nitrogen and sulphur heteroatoms (N11 and S13), at 153.1 ppm for L1 and 153.2 ppm for L5. One of the most significant changes was observed in the quaternary benzyl carbon (C16) because it is found between the nitrogen atoms (N1 and N3), shifting from 137.1 ppm for L1 to 141.2 ppm for L5. The coupling between 19F and 13C allowed the signals of the trifluoromethyl group to be assigned at 125.7 ppm (C20) and 129.6 ppm (C19), Fig. S11 and S12.†
Additionally, L2–L5 were characterised by 19F{1H} NMR. The observed signals were consistent with the molecular structures proposed for the four fluorinated compounds. L2 and L5 presented a single signal. In the case of L2, the signal was observed at −112.5 ppm and corresponded to the fluorine atom placed in the meta position of the benzyl ring. In the case of L5, the signal was observed at −62.8 ppm and corresponded to the trifluoromethyl group placed in the para position of the benzyl ring. L3 and L4 exhibited the signals corresponding to the position of the fluorine atoms around the benzyl ring. For example, at −118.7, −136.5, and −141.3 ppm for L3, which correspond to the fluorine atoms placed at C17, C18 and C21, and at −141.8, −153.1 and −161.0 ppm for L4, which correspond to the fluorine atoms placed at C17, C19 and C18, respectively, Fig. S13–S16.† In the MS-EI+ data of L1–L5, the peak corresponding to the molecular ion of the five compounds was identified at m/z = 291, 309, 345, 381, 359. In addition, the fragment corresponding to TBZ was found at m/z = 214 for all compounds, Fig. S17–S21.†
Crystallographic analysis
The molecular structures of L1, L2 and L4 with an ellipsoid representation at a 50% probability level are presented in Fig. 1. L1 presents two independent molecules in the asymmetric unit, crystallised in a monoclinic system with a P21/n space group with eight molecules in the unit cell. It is important to note that the benzimidazole ring approaches coplanarity with the thiazole ring with interplane angles of 9.68° and 14.91° between both molecules. In addition, the N-benzyl fragment has angles between planes of 83.95° and 88.01° between both molecules. On the other hand, L2 crystallised in a monoclinic system with a P21/n space group with four molecules in the unit cell. Unlike L1, this compound crystallised with one molecule in the asymmetric unit. Furthermore, the presence of a fluorine atom in the meta position of the N-benzyl fragment can be observed. Finally, L4 crystallised into a monoclinic system with a P21/c space with four molecules in the unit cell and one in the asymmetric unit. The N1 nitrogen atom bonds to the pentafluorobenzyl ring with an interplane angle of 74.20° concerning the benzimidazole ring. This compound presents disorder in the benzimidazole and thiazole rings.
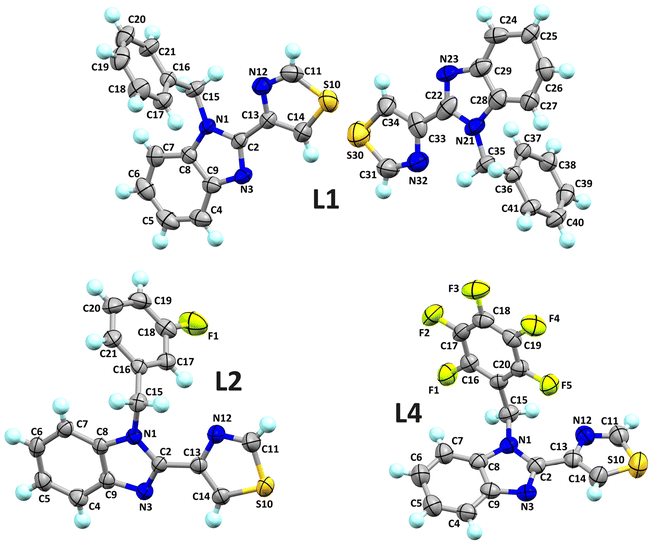 |
| Fig. 1 Ellipsoidal representation at a 50% probability level of the molecular structures of L1, L2 and L4. | |
In L1, the C20–H20⋯N3 hydrogen bond interactions stand out. These interactions can generate a 1D arrangement with an H20⋯N3 interaction distance of 2.60 Å (∑rvdW = 2.75 Å) and a bond angle ∠C20–H20–N3 of 141°. The two molecules in the asymmetric unit are related by the interactions C25–H25⋯Cg1 and C31–H31⋯Cg1, forming a significant part of the molecular structure. The distances between the hydrogen atoms and the Cg1 centroid of the C16–C21 rings are 2.84 and 2.80 Å, respectively. The C–H⋯π interactions and the C20–H20⋯N3 hydrogen bond, perpendicular to each other, form a laminar arrangement parallel to the ac plane, Fig. 2. In L2, the N3 nitrogen atom of benzimidazole forms two C–H⋯N interactions, giving rise to a 1D arrangement along the b-axis. These arrangements present a distance of 2.55 and 2.74 Å (∑rvdW = 2.75 Å) for C15–H15B⋯N3 and C14–H14⋯N3, respectively. The observed angle for ∠C15–H15B–N3 is 174°, and for the ∠C14–H14–N3 is 149°. In this same supramolecular arrangement, a C4–H4⋯S10 interaction is observed at a distance of 2.87 Å (∑rvdW = 3.00 Å) with an angle ∠C4–H4–S10 of 156°. The arrangement is complemented by a π⋯π interaction between the benzimidazole's six-membered ring and the thiazole's five-membered ring with a distance of 3.772 Å between centroids. The complexity of the molecular arrangements is showcased in the 2D and 3D arrangements, which are stabilised by C–H⋯π interactions. For instance, the C19–H19⋯Cg1 interaction, together with the C–H⋯N interactions, generates a 2D arrangement parallel to the bc plane, Fig. 3. In L4, π⋯π interactions were found between the pentafluorinated system and the thiazole ring. The distance between the centroids was 3.74 Å, and these interactions stabilise the 3D supramolecular arrangement, Fig. 4.
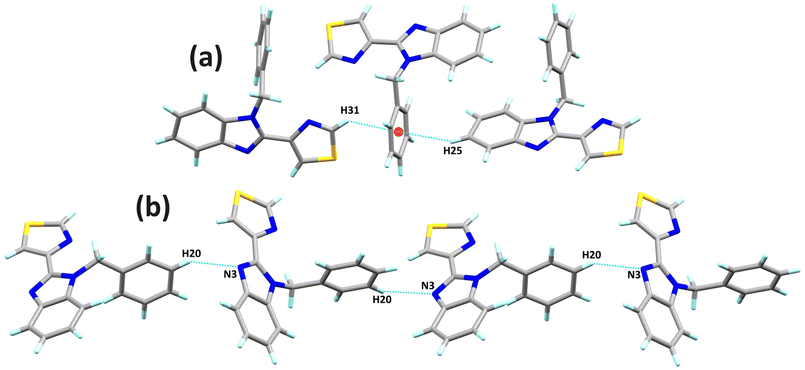 |
| Fig. 2 (a) Lamellar arrangement of L1 parallel to the ac plane, and (b) chain arrangement of L1 generated by the C–H⋯N interaction. | |
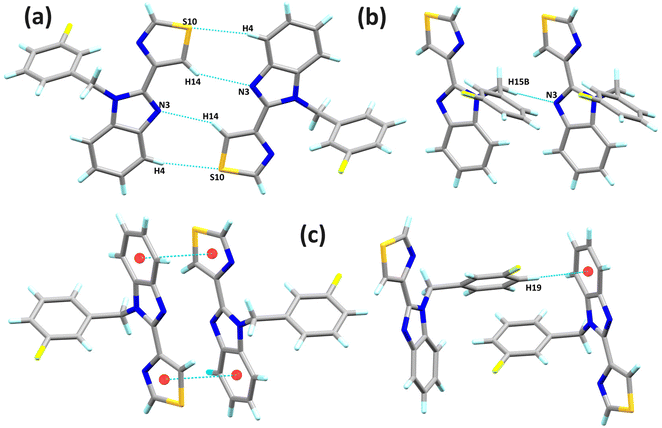 |
| Fig. 3 (a) Dimeric arrangement of L2 generated by the C–H⋯N and C–H⋯S interactions, (b) chain arrangement of L2 generated by the C–H⋯N interaction, and (c) representation of the π⋯π and C–H⋯π interactions found in L2. | |
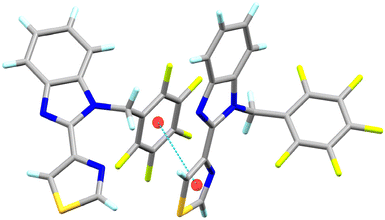 |
| Fig. 4 Representation of the π⋯π interactions found between the pentafluorobenzyl and thiazole rings in L4. | |
Antiparasitic activity
Since 1979, when treatment with mebendazole (MBZ) at a single dose (50 mg kg−1 in rats) was shown to be effective in controlling infections caused by Taenia crassiceps,41 multiple studies have been conducted to evaluate the effect of traditional drugs in the treatment of this disease. For example, it was proposed that combining praziquantel (PZQ) and ABZ-SO could improve the current treatment of cysticercosis in a murine model with Taenia crassiceps.42 This treatment was dependent on the exposure time and the concentration of both drugs.43 In addition, the best time to start treatment was 10 days after infection and at least 20 days of treatment were required.44In vitro studies of the combination of nitazoxanide (NTZ) with ABZ45 or of NTZ and flubendazole (FLB) could be useful in the treatment of infections caused by Taenia crassiceps since they induce a greater metabolic impact on cysticerci compared to the isolated exposure of each of them.46 Moreover, metabolic acidosis was greater in the group of cysticerci treated with NTZ than in the group treated with ABZ-SO, indicating that this is one of the modes of action used by this drug to induce parasite death.47 NTZ induced stress in the energetic metabolism in the tricarboxylic acid cycle, in protein catabolism and in the oxidation of fatty acids in Taenia crassiceps, so it could be used as an alternative for the treatment of cysticercosis.48 Additionally, other systems have been studied, such as curcumin oxidation products, which could act as suitable inhibitors of thioredoxin-glutathione reductase from Taenia crassiceps cysticerci.49 As well as the ternary system ABZ-βCD-PVP (albendazole, β-cyclodextrin and polyvinylpyrrolidone) due to its greater dissolution, high bioavailability and better cysticidal activity with respect to ABZ.50 Or, treatment with dehydroepiandrosterone (DHEA) which inhibits the establishment, growth and reproduction of Taenia crassiceps metacestodes.51
From the above, it is evident that most of the studies carried out for the treatment of cysticercosis in murine models with Taenia crassiceps have been carried out on drugs with a benzimidazole structure. Furthermore, it is known that evagination is a key indicator of the ability of the scolex to evaginate, a crucial process in the life cycle of Taenia crassiceps. Therefore, part of the methodology proposed by Parra-Unda and collaborators was used to evaluate the antiparasitic activity of compounds L1–L5.52 This test is particularly relevant to this study as it helps understand the compounds’ impact on the parasite's ability to develop and survive. In this context, the group incubated with ABZ at a concentration of 500 μM showed that after 24 h of incubation, 70% of evaginated cysticerci presented high mobility, but their integrity was reduced from complete to moderate. In the group incubated with TBZ, the percentage of evaginated cysticerci was 100% at the four exposure concentrations, and no reduction in integrity or mobility was observed. The cysticerci were only reduced in size by increasing the incubation time from 24 to 48 h with TBZ. In the five groups of parasites incubated with each of the five compounds, the percentage of evaginated cysticerci was 100% at any of the concentrations tested. Nonetheless, all compounds reduced the mobility of cysticerci with increasing concentration. L4 and L5 annulled mobility after 24 h of treatment at concentrations of 500 μM and 300 μM, respectively. A special case was the result obtained after 24 hours of incubation with L1 at a concentration of 500 μM, observing 100% of evaginated but wrinkled cysticerci with low mobility. This could be because N-benzylation increases the partition coefficient, promoting a species with greater bioavailability but without action on mobility. L4, with the highest amount of fluorine substituents in the N-benzyl fragment, presented greater activity at a lower concentration compared to L1–L3 but lower compared to L5. The steric volume and electron density of fluorine atoms in L4 could be a critical factor in the interaction with phenylalanine of parasitic β-tubulin. L5, with the trifluoromethyl group in the para position of the N-benzyl ring, presented a greater action at a concentration comparable to that of ABZ, which could be directly related to the cancellation of the parasites’ mobility, preventing their transfer to the hatching zone. L5 is an excellent candidate for future studies in the treatment of cysticercosis. This compound presents the trifluoromethyl group in its structure, a very important functional group in recent studies of the possible drugs RCB15 and RCB20, Table 2.
Table 2 Effect of ABZ, TBZ and L1–L5 (100, 200, 300 and 500 μM) on Taenia crassiceps cysticerci cultured in RPMI at 24 h
|
ABZ
|
TBZ
|
L1
|
L2
|
L3
|
L4
|
L5
|
Viability was determined by: [E] percentage of evaginated cysticerci: number of evaginated parasites/total number of cultured parasites. [M] Parasite mobility: high (++++), medium (+++), moderate (++), low (+), and null (−). [D] Damage to the cysticerci wall: complete (C), medium (M) and null (N). Cysticerci cultured in RPMI medium were used as a control. Additionally, cysticerci were exposed to 0.5% DMSO for the ABZ assay and 0.5% absolute ethanol for the TBZ and L1–L5 assays. |
Control |
[E] 100% |
[E] 100% |
[E] 100% |
[E] 100% |
[E] 100% |
[E] 100% |
[E] 100% |
[M] ++++ |
[M] ++++ |
[M] ++++ |
[M] ++++ |
[M] ++++ |
[M] ++++ |
[M] ++++ |
[D] C |
[D] C |
[D] C |
[D] C |
[D] C |
[D] C |
[D] C |
EtOH/DMSO (0.5%) |
[E] 100% |
[E] 100% |
[E] 100% |
[E] 100% |
[E] 100% |
[E] 100% |
[E] 100% |
[M] ++++ |
[M] ++++ |
[M] ++++ |
[M] ++++ |
[M] ++++ |
[M] ++++ |
[M] ++++ |
[D] C |
[D] C |
[D] C |
[D] C |
[D] C |
[D] C |
[D] C |
100 μM |
[E] 85% |
[E] 100% |
[E] 100% |
[E] 100% |
[E] 100% |
[E] 100% |
[E] 100% |
[M] ++++ |
[M] ++++ |
[M] ++++ |
[M] ++++ |
[M] ++++ |
[M] ++++ |
[M] ++ |
[D] C |
[D] C |
[D] C |
[D] C |
[D] C |
[D] C |
[D] C |
200 μM |
[E] 80% |
[E] 100% |
[E] 100% |
[E] 100% |
[E] 100% |
[E] 100% |
[E] 100% |
[M] ++++ |
[M] ++++ |
[M] ++++ |
[M] ++++ |
[M] ++++ |
[M] ++++ |
[M] + |
[D] C |
[D] C |
[D] C |
[D] C |
[D] C |
[D] C |
[D] C |
300 μM |
[E] 75% |
[E] 100% |
[E] 100% |
[E] 100% |
[E] 100% |
[E] 100% |
[E] 100% |
[M] ++++ |
[M] ++++ |
[M] ++++ |
[M] ++++ |
[M] ++++ |
[M] + |
[M] − |
[D] C |
[D] C |
[D] C |
[D] C |
[D] C |
[D] C |
[D] C |
500 μM |
[E] 70% |
[E] 100% |
[E] 100% |
[E] 100% |
[E] 100% |
[E] 100% |
[E] 100% |
[M] ++++ |
[M] ++++ |
[M] + |
[M] + |
[M] +++ |
[M] − |
[M] − |
[D] M |
[D] C |
[D] C |
[D] C |
[D] C |
[D] C |
[D] C |
Ethics approval
All protocols were carried out in the animal facilities of the Facultad de Medicina Universidad Nacional Autónoma de Mexico, under controlled conditions of temperature (22 °C), a relative humidity of 50–60% and 12 h dark/light cycles, in strict accordance with the Official Mexican Norm for the Production, Care, and Use of Laboratory Animals (NOM-062-ZOO-1999) and the Guide for the Care and Use of Laboratory Animals of the National Institutes of Health, U.S.A. The project was also approved by the Internal Committee for the Care and Use of Laboratory Animals (CICUAL-020-CIC-2018) de la Facultad de Medicina, UNAM, Mexico.
Conclusions
Five compounds derived from TBZ with an N-benzyl arm functionalised with fluoride substituents were synthesised and characterised. Remarkably, four of the compounds, excluding L1, have not been previously reported, adding a significant element of novelty to our research, as confirmed by our query in CAS Scifindern. Crystals of L1, L2 and L4 suitable for characterisation by SC-XRD were obtained. The supramolecular arrangements of the three structures are mainly supported by C–H⋯N3 intermolecular interactions. In addition, other weak interactions are reported, such as C–H⋯S10, C–H⋯π and π⋯π. All compounds showed activity in the mobility of Taenia crassiceps cysticerci, especially L4 and L5. The effect of ABZ on mobility, integrity and evagination was observed at different concentrations. ABZ is the drug of choice to treat neurocysticercosis, acting selectively on the cytoplasmic microtubules of the intestinal cells of the parasite. TBZ inhibits the fumarate reductase enzyme of some helminths other than Taenia solium and Taenia crassiceps. Thus, this investigation represents the first study against cysticercosis in a murine model with Taenia crassiceps using novel, non-commercial molecules.
Author contributions
M. I. Rodríguez-Mora: investigation, methodology and conceptualization. R. Colorado-Peralta: writing – original draft, visualization and formal analysis. V. Reyes-Márquez: writing – original draft, visualization and formal analysis. M. A. García-Eleno: investigation, supervision, software and validation. E. Cuevas-Yáñez: investigation, supervision, software and validation. J. R. Parra-Unda: investigation, methodology, resources and data curation. A. Landa: investigation, methodology, resources and data curation. D. Morales-Morales: conceptualization, resources, funding acquisition, project administration, and writing – review and editing.
Data availability
The data supporting this article have been included as part of the ESI.†
Conflicts of interest
There are no conflicts to declare.
Acknowledgements
The authors appreciate the collaboration of the institutions UAEM, UAS, UNAM, UNISON, and UV, and are thankful for CONAHCYT's financial support through the SNII. A. L. is thankful for the financial support from DGAPA-UNAM PAPIIT-205422. D. M-M. gratefully acknowledges the generous financial support from DGAPA-UNAM PAPIIT IN223323 and CONAHCYT A1-S-033933.
References
- M. Faheem, A. Rathaur, A. Pandey, V. K. Singh and A. K. Tiwari, A Review on the Modern Synthetic Approach of Benzimidazole Candidate, ChemistrySelect, 2020, 5(13), 3981–3994, DOI:10.1002/slct.201904832.
- C. S. W. Law and K. Y. Yeong, Benzimidazoles in Drug Discovery: A Patent Review, ChemMedChem, 2021, 16(12), 1861–1877, DOI:10.1002/cmdc.202100004.
- B. Pathare and T. Bansode, Review-biological active benzimidazole derivatives, Results Chem., 2021, 3, 100200, DOI:10.1016/j.rechem.2021.100200.
- Y. T. Lee, Y. J. Tan and C. E. Oon, Benzimidazole and its derivatives as cancer therapeutics: The potential role from traditional to precision medicine, Acta Pharm. Sin. B, 2023, 13(2), 478–497, DOI:10.1016/j.apsb.2022.09.010.
- H. E. Hashem and Y. El Bakri, An overview on novel synthetic approaches and medicinal applications of benzimidazole compounds, Arabian J. Chem., 2021, 14(11), 103418, DOI:10.1016/j.arabjc.2021.103418.
- I. Beltran-Hortelano, V. Alcolea, M. Font and S. Perez-Silanes, The role of imidazole and benzimidazole heterocycles in Chagas disease: A review, Eur. J. Med. Chem., 2020, 206, 112692, DOI:10.1016/j.ejmech.2020.112692.
- R. Veerasamy, A. Roy, R. Karunakaran and H. Rajak, Structure-activity relationship analysis of benzimidazoles as emerging anti-inflammatory agents: an overview, Pharmaceuticals, 2021, 14(7), 663, DOI:10.3390/ph14070663.
- M. Sweeney, D. Conboy, S. I. Mirallai and F. Aldabbagh, Advances in the synthesis of ring-fused benzimidazoles and imidazobenzimidazoles, Molecules, 2021, 26(9), 2684, DOI:10.3390/molecules26092684.
- K. Wu, X. Peng, M. Chen, Y. Li, G. Tang, J. Peng, Y. Peng and X. Cao, Recent progress of research on anti-tumor agents using benzimidazole as the structure unit, Chem. Biol. Drug Des., 2022, 99(5), 736–757, DOI:10.1111/cbdd.14022.
- A. C. G, R. Gondru, Y. Li and J. Banothu, Coumarin-benzimidazole hybrids: A review of developments in medicinal chemistry, Eur. J. Med. Chem., 2022, 227, 113921, DOI:10.1016/j.ejmech.2021.113921.
- D.-S. Son, E.-S. Lee and S. E. Adunyah, The Antitumor Potentials of Benzimidazole Anthelmintics as Repurposing Drugs, Immune Netw., 2020, 20(4), e29, DOI:10.4110/in.2020.20.e29.
- M. Devereux, D. O'Shea, A. Kellett, M. McCann, M. Walsh, D. Egan, C. Deegan, K. Kędziora, G. Rosair and H. Müller-Bunz, Synthesis, X-ray crystal structures and biomimetic and anticancer activities of novel copper(II) benzoate complexes incorporating 2-(4′-thiazolyl)benzimidazole (thiabendazole), 2-(2-pyridyl)benzimidazole and 1,10-phenanthroline as chelating nitrogen donor ligands, J. Inorg. Biochem., 2007, 101(6), 881–892, DOI:10.1016/j.jinorgbio.2007.02.002.
-
M. A. Alam, Chapter 1 - Thiazole, a privileged scaffold in drug discovery, in Privileged Scaffolds in Drug Discovery, ed. B. Yu, N. Li and C. Fu, Academic Press, 2023, pp. 1–19. DOI:10.1016/B978-0-443-18611-0.00027-9.
- K. El Bourakadi, M. E. M. Mekhzoum, A. E. K. Qaiss and R. Bouhfid, Recent Advances in the Synthesis and Applications of Thiabendazole Derivatives: A Short Review, Curr. Org. Chem., 2020, 24(20), 2367–2377, DOI:10.2174/1385272824999200922090947.
-
S. Kumar, M. T. Patil, R. Kataria and D. B. Salunke, 11. Thiazole: A privileged scaffold in drug discovery, in Chemical Drug Desing, ed. G. K. Gupta and K. Vinod, Boston, Berlin, 2016, pp. 243–281. DOI:10.1515/9783110368826-013.
- M. Budetić, D. Kopf, A. Dandić and M. Samardžić, Review of Characteristics and Analytical Methods for Determination of Thiabendazole, Molecules, 2023, 28(9), 3926, DOI:10.3390/molecules28093926.
- Q. Wu, Y. Li, C. Wang, Z. Liu, X. Zang, X. Zhou and Z. Wang, Dispersive liquid–liquid microextraction combined with high performance liquid chromatography–fluorescence detection for the determination of carbendazim and thiabendazole in environmental samples, Anal. Chim. Acta, 2009, 638(2), 139–145, DOI:10.1016/j.aca.2009.02.017.
- R. Igual-Adell, C. Oltra-Alcaraz, E. Soler-Company, P. Sánchez-Sánchez, J. Matogo-Oyana and D. Rodríguez-Calabuig, Efficacy and safety of ivermectin and thiabendazole in the treatment of strongyloidiasis, Expert Opin. Pharmacother., 2004, 5(12), 2615–2619, DOI:10.1517/14656566.5.12.2615.
- C. Henriquez-Camacho, E. Gotuzzo, J. Echevarria, A. C. White Jr, A. Terashima, F. Samalvides, J. A. Pérez-Molina and M. N. Plana, Ivermectin versus albendazole or thiabendazole for Strongyloides stercoralis infection, Cochrane Database Syst. Rev., 2016, 1, CD007745, DOI:10.1002/14651858.CD007745.pub3.
- H. D. Brown, A. R. Matzuk, I. R. Ilves, L. H. Peterson, S. A. Harris, L. H. Sarett, J. R. Egerton, J. J. Yakstis, W. C. Campbell and A. C. Cuckler, Antiparasitic drugs. IV. 2-(4′-Thiazolyl)benzimidazole, a new anthelmintic, J. Am. Chem. Soc., 1961, 83(7), 1764–1765, DOI:10.1021/ja01468a052.
- J. A. Maynard, I. D. Rae, D. Rash and J. M. Swan, Reaction of 2-(4-thiazolyl)benzimidazole (thiabendazole) with alkyl halides, Aust. J. Chem., 1971, 24(9), 1873–1881, DOI:10.1071/CH9711873.
- P. P. Mahulikar, N. S. Pawar, D. S. Dalat and P. P. Patil, Synthesis of N-alkyl-2-(4-thiazolyl)-1H-benzimidazole and N-acyl-2-(4-thiazolyl)-1H-benzimidazole derivatives, Org. Chem.: Indian J., 2007, 3(1), 34–36 CAS
https://www.tsijournals.com/articles/synthesis-of-biologically-active-nalkyland-nacyl-24thiazolyl-1hbenzimidazoles.pdf
.
- S.-Q. Zhu, Y.-L. Liu, H. Li, X.-H. Xu and F.-L. Qing, Direct and Regioselective C-H Oxidative Difluoromethylation of Heteroarenes, J. Am. Chem. Soc., 2018, 140(37), 11613–11617, DOI:10.1021/jacs.8b08135.
- N. S. Pawar, D. S. Dalal, S. R. Shimpi and P. P. Mahulikar, Studies of antimicrobial activity of N-alkyl and N-acyl 2-(4-thiazolyl)-1H-benzimidazoles, Eur. J. Pharm. Sci., 2004, 21(2–3), 115–118, DOI:10.1016/j.ejps.2003.09.001.
- L. Pan, N. Hang, C. Zhang, Y. Chen, S. Li, Y. Sun, Z. Li and X. Meng, Synthesis and biological evaluation of novel benzimidazole derivatives and analogs targeting the NLRP3 inflammasome, Molecules, 2017, 22(2), 213, DOI:10.3390/molecules22020213.
- C. Zhang, B. Zhong, S. Yang, L. Pan, S. Yu, Z. Li, S. Li, B. Su and X. Meng, Synthesis and biological evaluation of thiabendazole derivatives as anti-angiogenesis and vascular disrupting agents, Bioorg. Med. Chem., 2015, 23(13), 3774–3780, DOI:10.1016/j.bmc.2015.03.085.
- Z. Garkani-Nejad and F. Saneie, QSAR study of benzimidazole derivatives inhibition on escherichia coli methionine aminopeptidase, Bull. Chem. Soc. Ethiop., 2010, 24(3), 317–325, DOI:10.4314/bcse.v24i3.60661.
- R. Schiffmann, A. Neugebauer and C. D. Klein, Metal-Mediated Inhibition of Escherichia coli Methionine Aminopeptidase: Structure-Activity Relationships and Development of a Novel Scoring Function for Metal-Ligand Interactions, J. Med. Chem., 2006, 49(2), 511–522, DOI:10.1021/jm050476z.
- M. A. Altmeyer, A. Marschner, R. Schiffmann and C. D. Klein, Subtype-selectivity of metal-dependent methionine aminopeptidase inhibitors, Bioorg. Med. Chem. Lett., 2010, 20(14), 4038–4044, DOI:10.1016/j.bmcl.2010.05.093.
- A. G. Lescano and J. Zunt, Chapter 27 - Other cestodes: sparganosis, coenurosis and Taenia crassiceps cysticercosis, Handb. Clin. Neurol., 2013, 114, 335–345, DOI:10.1016/B978-0-444-53490-3.00027-3.
- K. Willms and R. Zurabian,
Taenia crassiceps: in vivo and in vitro models, Parasitology, 2010, 137(3), 335–346, DOI:10.1017/S0031182009991442.
- J. Morales-Montor and C. Larralde, The role of sex steroids in the complex physiology of the host-parasite relationship: the case of the larval cestode of Taenia crassiceps, Parasitology, 2005, 131(3), 287–294, DOI:10.1017/S0031182005007894.
- M. C. Romano, R. A. Valdéz, A. L. Cartas, Y. Gómez and C. Larralde, Steroid hormone production by parasites: the case of Taenia crassiceps and Taenia solium cysticerci, J. Steroid Biochem. Mol. Biol., 2003, 85(2–5), 221–225, DOI:10.1016/S0960-0760(03)00233-4.
- A. N. Peón, A. Espinoza-Jiménez and L. I. Terrazas, Immunoregulation by Taenia crassiceps and its antigens, BioMed Res. Int., 2013, 498583, DOI:10.1155/2013/498583.
- A. Márquez-Navarro, A. Pérez-Reyes, A. Zepeda-Rodríguez, O. Reynoso-Ducoing, A. Hernández-Campos, F. Hernández-Luis, R. Castillo, L. Yépez-Mulia and J. R. Ambrosio, RCB20, an experimental benzimidazole derivative, affects tubulin expression and induces gross anatomical changes in Taenia crassiceps cysticerci, Parasitol. Res., 2013, 112, 2215–2226, DOI:10.1007/s00436-013-3379-2.
- C. M. Fraga, T. L. da Costa, A. M. de Castro, O. Reynoso-Ducoing, J. Ambrosio, A. Hernández-Campos, R. Castillo and M. C. Vinaud, Alternative energy production pathways in Taenia crassiceps cysticerci in vitro exposed to a benzimidazole derivative (RCB20), Parasitology, 2016, 143(4), 488–493, DOI:10.1017/S0031182015001729.
- C. M. Fraga, T. Luiza da Costa, A. M. de Castro, O. Reynoso-Ducoing, J. Ambrosio, A. Hernández-Campos, R. Castillo and M. C. Vinaud, A benzimidazole derivative (RCB20) in vitro induces an activation of energetic pathways on Taenia crassiceps (ORF strain) cysticerci, Exp. Parasitol., 2017, 172, 12–17, DOI:10.1016/j.exppara.2016.11.002.
- G. de Andrade Picanço, N. Ferreira de Lima, C. M. Fraga, T. L. da Costa, E. Isac, J. Ambrosio, R. Castillo and M. C. Vinaud, A benzimidazole derivative (RCB15) in vitro induces the alternative energetic metabolism and glycolysis in Taenia crassiceps cysticerci, Acta Trop., 2017, 176, 288–292, DOI:10.1016/j.actatropica.2017.08.022.
- G. de A. Picanço, N. F. Lima, D. S. M. M. Alves, C. M. Fraga, T. L. Costa, R. de S. Lino Junior, R. Castillo, A. Hernández-Campos, J. Ambrosio and M. C. Vinaud, Partial inhibition of the tricarboxylic acid cycle in Taenia crassiceps cysticerci after the in vitro exposure to a benzimidazole derivative (RCB15), Acta Trop., 2020, 202, 105254, DOI:10.1016/j.actatropica.2019.105254.
- G. M. Sheldrick, Crystal structure refinement with SHELXL, Acta Crystallogr., Sect. C: Struct. Chem., 2015, 71(1), 3–8, DOI:10.1107/S2053229614024218.
- D. D. Heath and S. B. Lawrence, A single oral treatment with mebendazole for the control of Taenia crassiceps larval infections in rats, Int. J. Parasitol., 1979, 9(1), 73–76, DOI:10.1016/0020-7519(79)90070-5.
- F. Palomares, G. Palencia, J. R. Ambrosio, A. Ortiz and H. Jung-Cook, Evaluation of the efficacy of albendazole sulfoxide and praziquantel in combination on Taenia crassiceps cysts: in vitro studies, J. Antimicrob. Chemother., 2006, 57(3), 482–488, DOI:10.1093/jac/dki484.
- F. Palomares, G. Palencia, R. Pérez, D. González-Esquivel, N. Castro and H. Jung-Cook, In Vitro Effects of Albendazole Sulfoxide and Praziquantel against Taenia solium and Taenia crassiceps Cysts, Antimicrob, Agents Chemother., 2004, 48(6), 2302–2304, DOI:10.1128/aac.48.6.2302-2304.2004.
- F. Palomares-Alonso, G. Palencia Hernández, I. S. Rojas-Tomé, H. Jung-Cook and E. Pinzón-Estrada, Murine cysticercosis model: Influence of the infection time and the time of treatment on the cysticidal efficacy of albendazole and praziquantel, Exp. Parasitol., 2015, 149, 1–6, DOI:10.1016/j.exppara.2014.12.002.
- F. Palomares-Alonso, J. C. Piliado, G. Palencia, A. Ortiz-Plata and H. Jung-Cook, Efficacy of nitazoxanide, tizoxanide and tizoxanide/albendazole sulphoxide combination against Taenia crassiceps cysts, J. Antimicrob. Chemother., 2007, 59(2), 212–218, DOI:10.1093/jac/dkl463.
- N. Ferreira de Lima, G. de Andrade Picanço, T. L. Costa and M. C. Vinaud,
In vitro metabolic stress induced by nitazoxanide and flubendazole combination in Taenia crassiceps cysticerci, Exp. Parasitol., 2022, 238, 108265, DOI:10.1016/j.exppara.2022.108265.
- E. Isac, G. de A. Picanço, T. L. da Costa, N. F. de Lima, D. de S. M. M. Alves, C. M. Fraga, R. de S. Jr. Lino and M. C. Vinaud, Nitazoxanide induces in vitro metabolic acidosis in Taenia crassiceps cysticerci, Exp. Parasitol., 2016, 171, 17–22, DOI:10.1016/j.exppara.2016.10.012.
- E. Isac, G. A. Picanco, T. L. Costa, N. F. Lima, D. S. M. M. Alves, C. M. Fraga, R. S. Jr. Lino and M. C. Vinaud, In vitro nitazoxanide exposure affects energetic metabolism of Taenia crassiceps, flubendazole combination in Taenia crassiceps cysticerci, Exp. Parasitol., 2020, 208, 107792, DOI:10.1016/j.exppara.2019.107792.
- A. Guevara-Flores, J. J. Martínez-González, A. M. Herrera-Juárez, J. L. Rendón, M. González-Andrade, P. V. Torres Durán, R. G. Enríquez-Habib and I. P. del Arenal Mena, Effect of curcuminoids and curcumin derivate products on thioredoxin-glutathione reductase from Taenia crassiceps cysticerci, Evidence suggesting a curcumin oxidation product as a suitable inhibitor, PLoS One, 2019, 14(7), e0220098, DOI:10.1371/journal.pone.0220098.
- F. Palomares-Alonso, C. Rivas González, M. J. Bernad-Bernad, M. D. Castillo Montiel, G. Palencia Hernández, I. González-Hernández, N. Castro-Torres, E. Pinzón Estrada and H. Jung-Cook, Two novel ternary albendazole–cyclodextrin–polymer systems: Dissolution, bioavailability and efficacy against Taenia crassiceps cysts, Acta Trop., 2010, 113(1), 56–60, DOI:10.1016/j.actatropica.2009.09.006.
- J. A. Vargas-Villavicencio, C. Larralde and J. Morales-Montor, Treatment with dehydroepiandrosterone in vivo and in vitro inhibits reproduction, growth and viability of Taenia crassiceps metacestodes, Int. J. Parasitol., 2008, 38(7), 775–781, DOI:10.1016/j.ijpara.2007.10.011.
- R. Parra-Unda, F. Vaca-Paniagua, L. Jiménez and A. Landa, Cu,Zn superoxide dismutase: Cloning and analysis of the Taenia solium gene and Taenia crassiceps cDNA, Exp. Parasitol., 2012, 130, 32–38, DOI:10.1016/j.exppara.2011.10.002.
|
This journal is © The Royal Society of Chemistry 2024 |
Click here to see how this site uses Cookies. View our privacy policy here.