DOI:
10.1039/D4QO00515E
(Review Article)
Org. Chem. Front., 2024,
11, 5573-5604
Advances in organocatalytic asymmetric [3 + 3] cycloadditions: synthesis of chiral six-membered (hetero)cyclic compounds
Received
20th March 2024
, Accepted 1st May 2024
First published on 1st May 2024
Abstract
Organocatalytic asymmetric [3 + 3] cycloaddition underpins the efficient synthesis of chiral six-membered (hetero)cyclic compounds. Various elegant works were exploited to promote the prosperity of this cycloaddition-based methodology in past decades. The advantage of this unique strategy lies in great compatibility with flexible catalytic modes and multiple three-atom building blocks. This review summarizes the development of organocatalytic asymmetric [3 + 3] cycloaddition over the past twenty years. Both mechanistic studies and the activation mode of various organocatalytic systems, including (1) chiral amine catalysis, (2) chiral bifunctional catalyst catalysis, (3) chiral N-heterocyclic carbene catalysis, (4) chiral phosphoric acid catalysis, and (5) other asymmetric organocatalysis (isothioureas, phosphine and cooperative catalysis) are discussed in this review. Additionally, for each catalytic pattern, three-atom building blocks for organocatalytic asymmetric [3 + 3] cycloaddition were classified as all-carbon three-atom, aza-three-atom, oxa-three-atom building blocks and those containing other heteroatoms. This review has not only given a systematic summary of this topic, but also provided insights into the remaining challenges, which will promote the future development of this field.
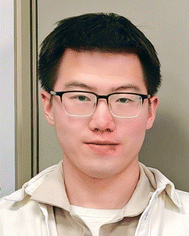 Zi-Qi Zhu | Zi-Qi Zhu received his Bachelor's degree from Jiangsu Normal University in 2015. Then, he started to pursue his M.S. degree under the supervision of Prof. Feng Shi at this university. In 2019, he began to pursue his Ph.D. degree at Nanjing University under the supervision of Prof. Zhuangzhi Shi. After receiving his Ph.D. degree in 2022, he joined Changzhou University as a lecturer and was promoted to an associate professor in 2023. His current research interests include developing novel catalytic asymmetric cycloaddition reactions and the synthesis of compounds bearing heteroatom chirality. |
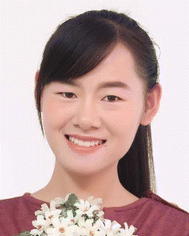 Tian-Zhen Li | Tian-Zhen Li received her Bachelor's degree from Jiangsu Normal University in 2018. Then, she joined the group of Prof. Feng Shi to pursue her M.S. degree at this university. In 2023, she continued to pursue her Ph.D. degree at Changzhou University. Her current research interests include asymmetric catalysis and photoredox catalysis for the synthesis of chiral heterocycles. |
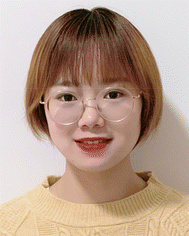 Si-Jia Liu | Si-Jia Liu received her Bachelor's degree from Jiangsu Normal University in 2019. Then, she started to pursue her M.S. degree under the supervision of Prof. Feng Shi at this university. In 2023, she continued to pursue her Ph.D. degree at Changzhou University. Her current research interests focus on the catalytic asymmetric synthesis of axially chiral indole derivatives. |
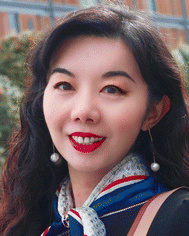 Feng Shi | Feng Shi received her Ph.D. degree from Soochow University and worked as a visiting scholar at Nanyang Technological University. She started her independent career in 2013 at Jiangsu Normal University and was promoted to a full professor in 2015. She was appointed a distinguished professor at Changzhou University in 2022. Her research interests focus on organocatalytic asymmetric reactions for the construction of chiral heterocyclic frameworks, especially chiral indole chemistry. She has published over 130 research papers as a corresponding author in peer-reviewed international journals. She is a recipient of the “National Science Fund for Distinguished Young Scholars in China”. Group website: https://m.x-mol.com/groups/Shi_Feng |
10th anniversary statement
Organic Chemistry Frontiers helped me to build my unique academic profile in “chiral indole chemistry.” For example, in Org. Chem. Front., 2018, 5, 2657 (https://doi.org/10.1039/C8QO00565F), we reported the design of 2,3-indolyldimethanols as a new class of indole-based platform molecules and established the first catalytic asymmetric substitution of 2,3-indolyldimethanols. Another example is Org. Chem. Front., 2021, 8, 212 (https://doi.org/10.1039/D0QO00699H), wherein we reported a regio- and enantioselective ring-opening reaction of vinylcyclopropanes with indoles under cooperative catalysis, and this paper was highlighted with an inside front cover which illustrated the landmark building of Jiangsu Normal University. Additionally, we published two review articles related to chiral indole chemistry, one is focusing on organocatalytic asymmetric dearomatization reactions of indole derivatives (https://doi.org/10.1039/D0QO01124J), another is concentrating on organocatalytic asymmetric reactions of vinylindoles (https://doi.org/10.1039/D0QO01643H). Based on my own experience, I believe publication with Organic Chemistry Frontiers is helpful for scholars to build their unique academic profiles because this journal has a broad reader community and the speed of on-line publication is very fast, which deserves publication of your research work or review articles with high quality.
|
1. Introduction
Chiral six-membered (hetero)cyclic compounds are widely found in many natural products and bioactive molecules (Fig. 1).1–5 Consequently, intensive interest has been focused on the construction of these frameworks in an enantioenriched fashion.6,7 In this context, catalytic asymmetric cycloadditions provide powerful methods to access enantioenriched six-membered (hetero)cyclic compounds in a single step with simple starting materials.8 In general, commonly used cycloaddition reactions for synthesizing such compounds include catalytic asymmetric [4 + 2] cycloaddition9,10 and [3 + 3] cycloaddition.11–13 Compared to traditional [4 + 2] cycloaddition, catalytic asymmetric [3 + 3] cycloaddition has recently attracted much attention from the synthetic community due to the diverse three-atom building blocks and flexible catalytic patterns of this class of reactions.
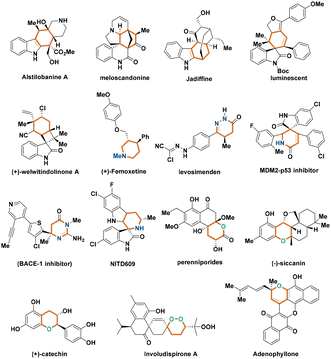 |
| Fig. 1 Selected natural products and pharmaceuticals containing a chiral six-membered ring. | |
Among catalytic asymmetric [3 + 3] cycloadditions, organocatalytic asymmetric [3 + 3] cycloadditions have developed rapidly in the past decades owing to the advantages of asymmetric organocatalysis such as mild reaction conditions, easily available organocatalysts and avoiding metal contamination of the products.14–19 As a consequence, organocatalytic asymmetric [3 + 3] cycloadditions have become important tools for synthesizing enantioenriched six-membered (hetero)cyclic compounds.
As summarized in Scheme 1, based on different catalytic modes, organocatalytic asymmetric [3 + 3] cycloadditions are mainly classified as: (1) chiral amine catalysis, (2) chiral bifunctional catalyst catalysis, (3) chiral N-heterocyclic carbene catalysis, and (4) chiral phosphoric acid catalysis. When using chiral phosphoric acid catalysis and chiral bifunctional catalyst catalysis, the enantioselectivity of the [3 + 3] cycloadditions was controlled by H-bonding interactions between the chiral catalysts and the substrates. While using chiral amine catalysis and chiral N-heterocyclic carbene catalysis, the enantioselectivity was controlled by covalent interactions between the chiral catalysts and the substrates.
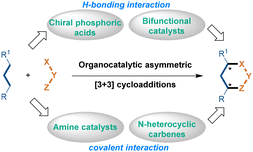 |
| Scheme 1 Different catalytic modes of organocatalytic asymmetric [3 + 3] cycloadditions. | |
The first catalytic mode is chiral amine catalysis (Scheme 2), which usually begins with the LUMO activation of an α,β-unsaturated aldehyde by condensation with a chiral amine catalyst to generate vinyl iminium intermediate I. Then, the 1,3-dinucleophile (X–Y–Z) attacks vinyl iminium I through Michael addition to give chiral intermediate II, which undergoes hydrolysis and tautomerization to release the chiral amine catalyst. Chiral intermediate III further undergoes an intramolecular 1,2-addition to accomplish the [3 + 3] cycloaddition and deliver the chiral six-membered (hetero)cyclic compound. In addition, an extra oxidation or elimination process is helpful for stabilizing the products.
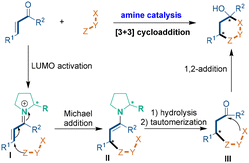 |
| Scheme 2 Catalytic mode of chiral amine-catalyzed asymmetric [3 + 3] cycloadditions. | |
The second catalytic mode is chiral bifunctional catalyst catalysis, which can activate substrates by H-bonding interactions (Scheme 3). The H-bonding donors in such chiral catalysts usually rely on urea, thiourea and squaramide groups, while the tertiary-amine moiety generally plays the role of a Brønsted base to activate the substrate. In most cases, in the reaction pathway, the chiral bifunctional catalyst activates both of the substrates to undergo an enantioselective Michael addition, thus generating chiral intermediate IV, which isomerizes into another chiral intermediate V. Then, still under activation of the chiral bifunctional catalyst, intermediate V undergoes an intramolecular addition reaction to accomplish the [3 + 3] cycloaddition.
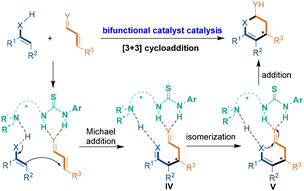 |
| Scheme 3 Catalytic mode of chiral bifunctional catalyst-catalyzed asymmetric [3 + 3] cycloadditions. | |
The third catalytic mode is chiral N-heterocyclic carbene (NHC) catalysis, which involves the formation of acyl azoliums as key intermediates. In NHC-catalyzed asymmetric [3 + 3] cycloadditions (Scheme 4), cinnamaldehyde derivatives can form a Breslow intermediate, which undergoes oxidation to give an acyl azolium intermediate (Scheme 4a). Besides, a number of reports demonstrate that the same intermediate can be directly formed from unsaturated esters and ynals under the catalysis of NHC (Scheme 4b and c). Another efficient method to form an acyl azolium intermediate relies on the elimination of a Breslow intermediate, which is generated from α-halogenated unsaturated enals and an NHC (Scheme 4d). After the formation of acyl azoliums, this kind of chiral intermediate undergoes successive 1,4-addition and 1,2-addition reactions with dinucleophiles to realize the asymmetric [3 + 3] cycloaddition and the construction of chiral six-membered rings.
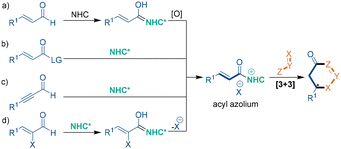 |
| Scheme 4 Catalytic mode of chiral NHC-catalyzed asymmetric [3 + 3] cycloadditions. | |
The fourth catalytic mode for asymmetric [3 + 3] cycloadditions is chiral phosphoric acid (CPA) catalysis. In most cases, indolylmethanols are utilized as suitable three-atom building blocks to undergo CPA-catalyzed asymmetric [3 + 3] cycloadditions with 1,3-dipoles such as azomethine ylides (Scheme 5). In the presence of CPA, indolylmethanols are easily transformed into vinyl iminium intermediates via dehydration. Then, CPA simultaneously activates both vinyl iminium intermediates and azomethine ylides by forming H-bonding and ion-pairing interactions, thus controlling the enantioselectivity of the [3 + 3] cycloadditions.
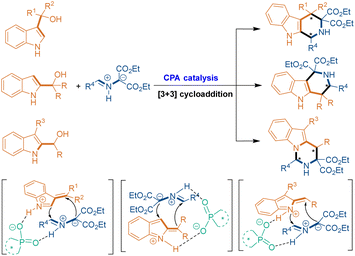 |
| Scheme 5 Catalytic mode of CPA-catalyzed asymmetric [3 + 3] cycloadditions. | |
Based on the above-mentioned four kinds of catalytic modes, excellent enantiocontrol can be achieved in organocatalytic asymmetric [3 + 3] cycloadditions. In addition, extensive studies on structurally tunable three-atom building blocks for [3 + 3] cycloadditions promoted the rapid development of this kind of reaction, thus affording chiral six-membered rings with optical purity and structural diversity. Besides, organocatalytic asymmetric [3 + 3] cycloadditions have been applied to the total synthesis of natural products and chemical probes, and the in vitro cytotoxicity of some chiral products has been examined; this demonstrates the potential of this class of cycloaddition and six-membered (hetero)cyclic compounds.
Although organocatalytic asymmetric [3 + 3] cycloadditions have flourished in the past decades, a systematic summary of this topic has not appeared. Therefore, there is an urgent need to summarize the developments in this field and give insights into the remaining challenges, thus promoting the future development of organocatalytic asymmetric [3 + 3] cycloadditions. This review is organized by different catalytic modes in organocatalytic asymmetric [3 + 3] cycloadditions as introduced above, and the subsections are divided into the different types of three-atom building blocks that are involved in organocatalytic asymmetric [3 + 3] cycloadditions.
2. Chiral amine-catalyzed asymmetric [3 + 3] cycloadditions
2.1 Enamine as carbon–carbon–nitrogen building blocks
In 2005, pioneering work by Hsung and coworkers demonstrated that chiral amine salt (S)-C1 could promote the intramolecular aza-[3 + 3] cycloaddition of enamine-type substrates 1 (Scheme 6).20 A serious of chiral quinolizidines 2 were generated through a cascade aza-1,4-addition/carbon-1,2-addition process. The chiral center was formed at the aza-1,4-addition step, and it was deduced that the nitrogen atom attacked the chiral vinyl iminium salt from the Re-face. Although only modest enantioselectivity was obtained, this study represented the first enantioselective intramolecular aza-[3 + 3] cycloaddition reaction promoted by a chiral secondary amine catalyst.
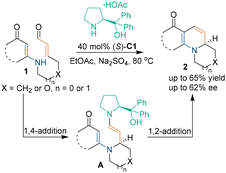 |
| Scheme 6 Chiral amine-catalyzed asymmetric intramolecular aza-[3 + 3] cycloaddition. | |
Later, in 2008, Hayashi and coworkers developed an efficient method to synthesize a chiral piperidine ring via a chiral amine-catalyzed asymmetric intermolecular aza-[3 + 3] cycloaddition.21 This aza-[3 + 3] cycloaddition of unsaturated aldehydes 4 and enecarbamates 3 was realized by using diphenylprolinol silyl ether (S)-C2 as a chiral catalyst (Scheme 7). Through this approach, products 5 with excellent enantioselectivity were obtained in high yield. In the proposed reaction pathway, unsaturated aldehydes 3 condensed with catalyst (S)-C2 to form imine cation intermediates (TS-1), which underwent an ene reaction with substrates 3 to generate acylimine intermediates B. The subsequent isomerization and hemiacetal reaction of intermediates B led to the production of enantioenriched piperidines 5.
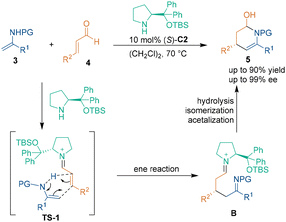 |
| Scheme 7 Chiral amine-catalyzed asymmetric aza-[3 + 3] cycloaddition. | |
At the same time, Wang's group reported a related transformation using N-Ac enamides 6 as substrates (Scheme 8a).22 Additionally, other enamine analogues such as enaminones (8, 10a–10b)23–25 and aminobenzofurans 13
26 could also serve as three-atom building blocks to react with aldehydes 4 and undergo asymmetric aza-[3 + 3] cycloadditions under this catalysis system (Scheme 8b–e).
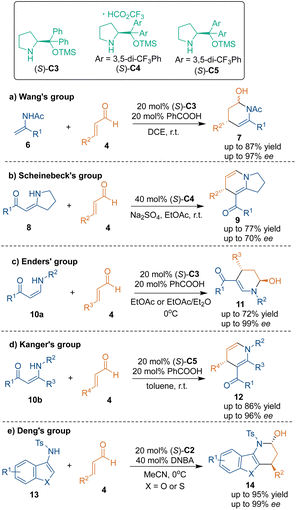 |
| Scheme 8 Other enamine-based catalytic asymmetric aza-[3 + 3] cycloadditions. | |
Meanwhile, imines can tautomerize to enamines and participate in aza-[3 + 3] cycloadditions in the presence of organic bases. In 2011, Chen's group discovered the reversed electrophilic reactivity of cyclic N-sulfonylimines 15.27 The electron-withdrawing effect of the sulfonyl group resulted in a lower pKa of the α-protons. In the presence of chiral secondary amine (S)-C3, cyclic imines 15 could undergo Michael addition with cinnamaldehydes 4, and the subsequent cyclization process was promoted by DBU in one pot, thus accomplishing the aza-[3 + 3] cycloaddition to give products 16 with high enantioselectivities (Scheme 9a). Three years later, Zhang's group upgraded this strategy by using DMAP as an additive (Scheme 9b).28 A series of biologically important piperidine derivatives 18 containing two stereocenters were obtained through such catalytic asymmetric tandem reactions with excellent enantioselectivities and diastereoselectivities. In addition, acyclic imines 19 could also be used as carbon–carbon–nitrogen building blocks to construct enantioenriched nitrogen-containing heterocyclic scaffolds 20 through Michael addition, subsequent cyclization and oxidation (Scheme 9c).29
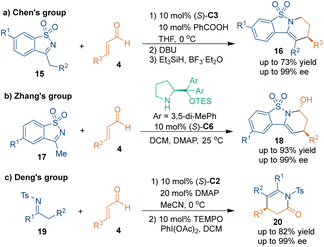 |
| Scheme 9
N-Sulfonylimine-based catalytic asymmetric aza-[3 + 3] cycloadditions. | |
2.2 Carbonyl compounds as three-carbon building blocks
In 2006, Hong's group reported an organocatalytic asymmetric [3 + 3] cycloaddition between crotonaldehydes 4 and 4′ in the presence of L-proline,30 which occurred through the Michael addition–aldol reaction pathway (Scheme 10). This cascade process afforded chiral cyclohexene adducts 21 with up to 73% yield and 95% ee. Besides, products 21 could be easily transformed into (−)-isopulegol hydrate 22 and (−)-cubebaol 23, which were applied to the synthesis of HIV-1 protease inhibitive didemnaketals.
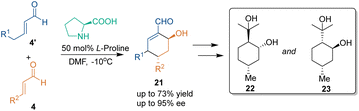 |
| Scheme 10 Crotonaldehyde-based catalytic asymmetric [3 + 3] cycloaddition. | |
Soon after, another organocatalytic asymmetric [3 + 3] cycloaddition between ketones 24 and enones 25 was realized by Tang's group (Scheme 11).31 Under the catalysis of pyrrolidine trifluoromethane-sulfonamide (S)-C7 and 4-methoxybenzoic acid, different cyclohexanone derivatives 24 and enones 25 could react smoothly to construct optically pure bicyclic [3.3.1] skeletons 26 in overall high enantioselectivities. According to the proposed reaction mechanism, ketones 24 underwent dehydration through the action of catalyst (S)-C7 to generate enamine intermediates C, which further attacked enones 25 from the Si-face under the activation of the sulfonamide functional group via H-bonding interactions. Then, intermediates D underwent tautomerization and an aldol reaction to give final products 26 and regenerate the secondary amine catalyst (S)-C7 to complete the catalytic cycle. Although 50 equivalent ketones were used, this method constructed four stereogenic centers in one step and provided efficient access to biologically active bicyclic [3.3.1] compounds.
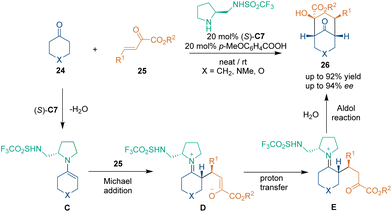 |
| Scheme 11 Catalytic asymmetric [3 + 3] cycloaddition of cyclic ketones and enones. | |
The applications of other ketones bearing an α-H as three-carbon building blocks in secondary amine-catalyzed [3 + 3] cycloadditions have been demonstrated by the groups of Hayashi,32 Alonso,33 Wang34 and so on. For example, Hayashi and coworkers reported that dimethyl 3-oxopentanedioate 27 could undergo Michael addition–Knoevenagel condensation with unsaturated aldehydes 4 in the presence of diphenylprolinol silyl ether (S)-C2 (Scheme 12a), which afforded products 28 with high enantioselectivities. Moreover, enantioselective [3 + 3] cycloaddition using ketones 29 as three-carbon building blocks could also be used for the total synthesis of natural products (Scheme 12b). In detail, dioxanone 29 and β-(hetero)aryl-α-nitro-α,β-enals 30 could take part in the [3 + 3] cycloaddition under the catalysis of chiral pyrrolidine catalyst (R)-C8, and the enantioselectivities of products 31 could reach 99% ee after crystallization. Further multi-step transformations of 31 gave (+)-pancratistatin in an overall 21.5% yield from 31.
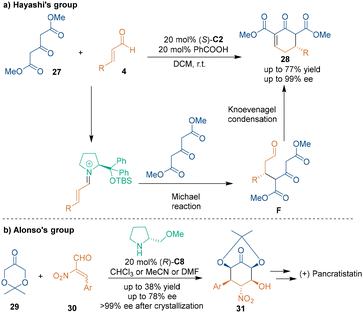 |
| Scheme 12 Catalytic asymmetric [3 + 3] cycloadditions of ketones and unsaturated aldehydes. | |
Additionally, using a potassium salt of an amino acid (L-Phe-K) as a catalyst, the catalytic asymmetric [3 + 3] cycloaddition of acetone 32 with 2-enoyl-pyridine N-oxide 33 was accomplished,35 wherein the counterion interaction between a potassium cation and pyridine N-oxide (TS-2) played an important role in the enantiocontrol of this transformation (Scheme 13).
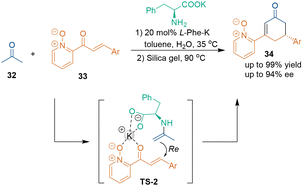 |
| Scheme 13 Catalytic asymmetric [3 + 3] cycloaddition of acetone and 2-enoyl-pyridine N-oxides. | |
Alkylidene pyrazolinones, a class of promising core structures in a variety of pharmaceuticals, have been demonstrated to be excellent electrophilic two-carbon building blocks in the synthesis of spirocyclohexane-based pyrazolones.36 Nevertheless, the acidic γ-H enabled this class of substrates to act as three-carbon building blocks in chiral amine-catalyzed [3 + 3] cycloadditions. In 2018, Li's group reported enantioselective [3 + 3] cycloadditions between alkylidene pyrazolinones 35 and cinnamaldehydes 4 (Scheme 14).37a In the suggested reaction pathway, pyrazolinones 35 attacked the iminium ions generated from aldehydes 4 and secondary amine catalyst (S)-C2 to give intermediates G. Then, the hydrolysis of intermediates G delivered intermediates H, which underwent an intramolecular aldol reaction to give enantioenriched spirocyclohexanes 36 with high yields and excellent diastereo- and enantioselectivities.
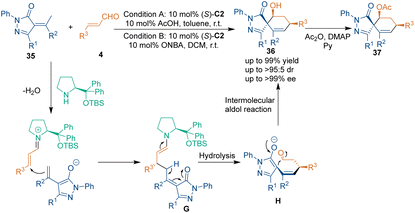 |
| Scheme 14 Catalytic asymmetric [3 + 3] cycloaddition of pyrazolinones and unsaturated aldehydes. | |
Apart from the reaction pathway of the tandem Michael–aldol reaction, other catalytic asymmetric [3 + 3] cycloadditions involving different reaction pathways were investigated. In 2007, Jørgensen's group realized an organocatalytic asymmetric [3 + 3] cycloaddition involving a Michael/Morita–Baylis–Hillman (MBH) mechanism (Scheme 15).37b Catalyzed by proline derivative (S)-C3, the asymmetric [3 + 3] cycloaddition of α,β-unsaturated aldehydes 4 with Nazarov reagents 38 generated chiral cyclohexenones 39 with high enantioselectivities. In the proposed reaction mechanism, diarylprolinol ether (S)-C3 activated aldehydes 4 to generate iminium intermediates, which further underwent a Michael addition with Nazarov reagents 37 to afford enamine intermediates I. The subsequent hydrolysis of intermediates I delivered intermediates J and recovered catalyst (S)-C3. Then, the amine catalyst (S)-C3 played a different role by attacking the carbon–carbon double bond of intermediates J to trigger the intramolecular MBH reaction and complete the cycloaddition. In-depth investigations were conducted to support this unique mechanism. For example, different catalysts were tested for the MBH reaction step, and it was discovered that several amines and PPh3 could promote this transformation. Meanwhile, the stereoselectivity of this step was induced by the stereogenic center, which was generated in the Michael addition step.
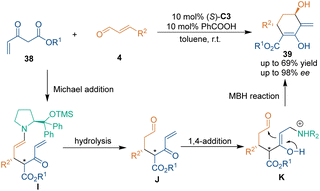 |
| Scheme 15 Catalytic asymmetric [3 + 3] cycloaddition involving a MBH reaction. | |
2.3 Carbonyl compounds as carbon–carbon–oxygen building blocks
Due to the nucleophilicity of the oxygen atom in enols, carbonyl compounds can also be used as carbon–carbon–oxygen building blocks in catalytic asymmetric [3 + 3] cycloadditions. In 2008, Rueping's group realized an organocatalytic asymmetric [3 + 3] cycloaddition by using 2-hydroxy-1,4-naphthoquinone 40 as carbon–carbon–oxygen building blocks to react with aldehydes 4 in the presence of chiral amine catalyst (S)-C5, thus achieving the enantioselective synthesis of pyranonaphthoquinones 41 (Scheme 16).38 This cascade reaction started from the condensation of catalyst (S)-C5 with aldehydes 4 to give iminium intermediates. Afterwards, the iminium intermediates were attacked by 2-hydroxy-1,4-naphthoquinone 40 to afford intermediates L after subsequent isomerization. Then, the hydrolysis of L released catalyst (S)-C5, and the intramolecular acetylization resulted in the formation of naphthoquinone products 41. Notably, the unstable hemiacetal group in products 41 could transform into the corresponding 1,2-pyranonaphthoquinones 42 by reduction without compromising the enantiopurity.
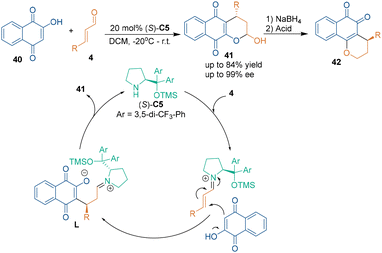 |
| Scheme 16 Catalytic asymmetric [3 + 3] cycloaddition of naphthoquinones and aldehydes. | |
Almost at the same time, Gong's group reported a related asymmetric [3 + 3] cycloaddition of Nazarov reagents 43 with aldehydes 4 under the catalysis of (S)-C3 to generate six-membered heterocycles 44 with high enantioselectivities (Scheme 17a).39 In this approach, Nazarov reagents 43 were used as nucleophiles to attack iminium salts, which were generated by the condensation of aldehydes 4 with catalyst (S)-C3.
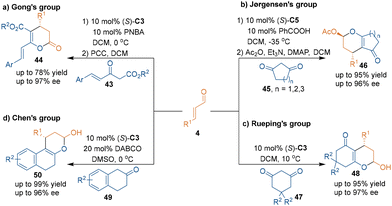 |
| Scheme 17 Catalytic asymmetric [3 + 3] cycloadditions of different ketones and aldehydes. | |
Moreover, the groups of Jørgensen,40 Rueping41 and Chen42 developed various chiral amine-catalyzed asymmetric [3 + 3] cycloadditions of cycloalkanediones (45, 47, 49) with α,β-unsaturated aldehydes 4, which constructed a variety of chiral oxygen-containing six-membered rings in high yields with excellent enantioselectivities (Scheme 17b–d). In addition, as an analogue of carbonyl compounds, naphthols could smoothly take part in amine-catalyzed asymmetric [3 + 3] cycloaddition to construct six-membered rings.43
2.4 Other compounds as three-atom building blocks
Other than the aforementioned building blocks, chemists developed other compounds as three-atom building blocks for chiral amine-catalyzed asymmetric [3 + 3] cycloadditions. In early 2009, Franzén's group developed tryptamine-derived amides 51 as three-atom building blocks.44 This class of unique substrates could undergo a cascade reaction sequence of [3 + 3] cycloaddition and electrophilic aromatic substitution with aldehydes 4 to construct chiral quinolizidine skeletons 52 under the catalysis of classic proline derivative (S)-C3 (Scheme 18). In their suggested reaction mechanism, the iminium ion generated from α,β-unsaturated aldehydes 4 and catalyst (S)-C3 was firstly attacked by substrates 51 to give intermediate M. This Michael addition adduct M spontaneously cyclized to give annular hemiacetal N. Then, the dehydration of intermediate N in the presence of an acid afforded acyliminium intermediate O, which was attacked by the C2-position of the indole ring, thus generating the final products 52. Through this method, various indolo-[2,3-a]quinolizidines 52 containing three stereogenic centers were synthesized with excellent enantioselectivities and moderate diastereoselectivities. Later, they demonstrated that the same strategy could be utilized for the enantioselective synthesis of quinolizidine derivatives involving a thiophene or a furan moiety.45
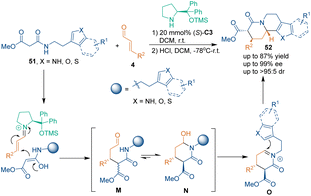 |
| Scheme 18 Catalytic asymmetric [3 + 3] cycloaddition of tryptamine derivatives and aldehydes. | |
In 2015, Ishikawa's group utilized malonamate 53 as a three-atom building block in chiral amine-catalyzed asymmetric [3 + 3] cycloaddition with aldehydes 4 for the synthesis of chiral thioenaminones 54 (Scheme 19).46 More importantly, they applied this strategy as a key step in the asymmetric total synthesis of (+)-α-skytanthine.
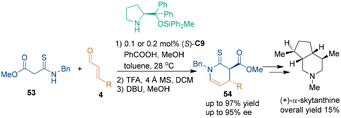 |
| Scheme 19 Catalytic asymmetric [3 + 3] cycloaddition of malonamate and aldehydes. | |
In 2010, Gong's group applied 3-(furan-2-yl)-2-nitro-1-propanols 55 as three-carbon building blocks in chiral amine-catalyzed asymmetric [3 + 3] cycloaddition with cinnamaldehydes 4 (Scheme 20a).47 Through this protocol, enantioenriched δ-lactols 56 containing a quaternary stereogenic center could be yielded and purified simply by filtration. Moreover, the catalyst and excess reagents can be recycled eight times without losing enantioselectivity. Three years later, Cid's group developed a related strategy to prepare nitrocyclohexenes 58 (Scheme 20b).48 Notably, the [3 + 3] cycloadduct could be reduced to cyclohexylamine by H2/Pd(C); this is an essential intermediate in the asymmetric total synthesis of trandolapril.
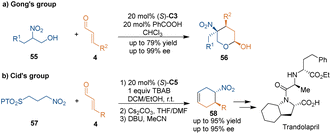 |
| Scheme 20 Catalytic asymmetric [3 + 3] cycloadditions of nitromethanes and aldehydes. | |
3. Chiral bifunctional catalyst-catalyzed asymmetric [3 + 3] cycloadditions
3.1 Nitroolefins as three-carbon building blocks
Benefiting from multiple activation sites, chiral bifunctional catalysts could act as Lewis bases, nucleophilic catalysts and H-bond donors in asymmetric catalysis.49 In 2009, Tang, Li and coworkers realized the first chiral bifunctional catalyst-catalyzed asymmetric [3 + 3] cycloaddition (Scheme 21).50 A series of 2-nitroallylic acetates 60 were used as three-carbon building blocks to undergo [3 + 3] cycloaddition with cyclic ketones 59 in the presence of chiral bifunctional catalyst (S)-C10, constructing chiral bicycle [3.3.1] skeletons 61 with four or five stereogenic centers in one step with excellent enantioselectivity. In the supposed reaction pathway and activation mode, the secondary amine group of catalyst (S)-C10 activated cyclic ketones 59 to generate enamine intermediate P, and the thiourea group of this catalyst activated the nitro group of 2-nitroallylic acetates 60 (TS-3), thus facilitating Michael addition and the subsequent elimination of an acetate to give iminium intermediate Q. Then, enamine intermediate R, which was tautomerized from Q, underwent a second Michael addition to yield the target molecule 61via hydrolysis to regenerate the chiral catalyst and finish the catalytic cycle. Density functional theory (DFT) studies indicated that two strong hydrogen bonds between the thiourea group of the catalyst and the nitro group of substrate 60 were vital for stereocontrol in the first carbon–carbon bond-forming step.
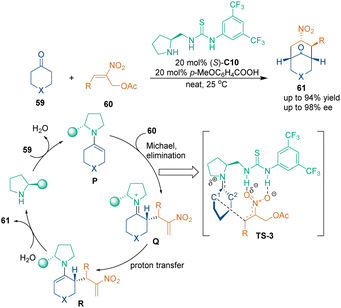 |
| Scheme 21 Catalytic asymmetric [3 + 3] cycloaddition of 2-nitroallylic acetates and ketones. | |
A similar strategy could also be used to construct a chiral tetrahydropyran scaffold (Scheme 22). In 2016, Zhou's group demonstrated that an overall SN2′ reaction and spontaneous oxa-Michael addition could occur between pyrazolin-5-one 62 and nitroalkenes 60 in the presence of tertiary amine-squaramide catalyst C11 (Scheme 22a).51 In the same year, Xu's group developed a more complex transformation,52 which involved a three-component tandem reaction of phenols 64, 2-nitro-1,3-enynes 65 and vinyl ketones 66, leading to an enantioenriched 3-nitrochromane enabled by chiral catalyst C12 (Scheme 22b). In their design, the tandem Friedel–Crafts (F–C) alkylation/oxa-addition of phenols 64 with 2-nitro-1,3-enynes 65 produced [3 + 3] cycloadducts T first. The subsequent 1,4-addition of intermediate products T to vinyl ketones 66 gave 3-nitrochromans 67 as the final products in a highly chemo-, regio-, and stereoselective manner. In the suggested reaction pathway, the bifunctional squaramide C12 activated the substrates or the intermediates by H-bonding interactions in each step.
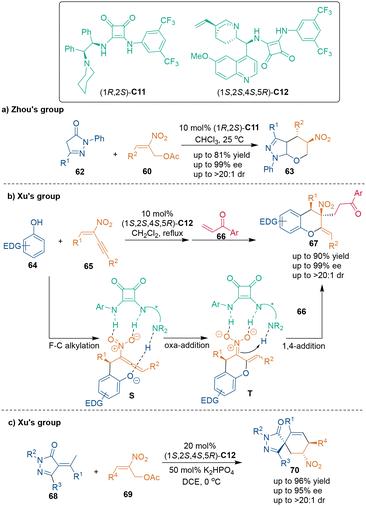 |
| Scheme 22 Catalytic asymmetric [3 + 3] cycloadditions involving nitro-enynes. | |
One year later, the same group synthesized chiral spirocyclohexene pyrazolones 70via bifunctional squaramide C12-catalyzed [3 + 3] cycloaddition between nitroolefins 69 and α-arylidene pyrazolinones 68 (Scheme 22c).53 The binucleophilicity of pyrazolinones 68 facilitated the Si-face attack and Michael addition, and the synergistic activation of the two substrates by catalyst C12 ensured excellent diastereo- and enantioselectivities of products 70. Additionally, other reports from the groups of Chen,54 Cao55 and Singh56 further demonstrated that these kinds of electron-poor alkenes 68 were suitable three-carbon building blocks in organocatalytic asymmetric [3 + 3] cycloaddition reactions.
In 2021, Ni, Song and coworkers constructed biologically intriguing aza-spirooxindole skeletons 72 utilizing branched nitroenynes 65 as dual electrophilic reagents.57 As illustrated in Scheme 23, nitroenynes 65 were attacked by the C3-position of 3-pyrrolyloxindoles 71 first, whereupon the functionalities of the tertiary amine and squaramide in catalyst C13 simultaneously activated the oxindole and nitro groups in this stereocontrol step (TS-4). Then, a 6-endo-trig Friedel–Crafts cyclization (TS-5) and subsequent isomerization occurred to produce products 72 smoothly.
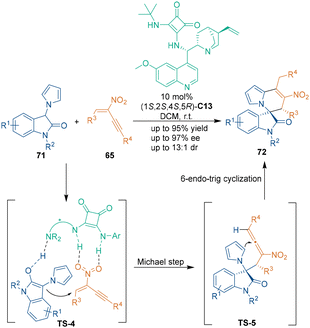 |
| Scheme 23 Catalytic asymmetric [3 + 3] cycloaddition of nitro-enynes and 3-pyrrolyloxindoles. | |
3.2 Unsaturated carbonyl compounds as three-carbon building blocks
As classic acceptors of Michael addition, unsaturated carbonyl compounds were frequently utilized as suitable three-carbon building blocks in organocatalytic [3 + 3] cycloadditions. In 2013, Wu and co-workers reported a catalytic asymmetric dearomatization annulation of 2,3-disubstituted indoles 73 with acrolein 4 (Scheme 24).58 Quinine-derived primary amine C14 activated acrolein 4 by iminium catalysis, which facilitated an enantioselective 1,4-addition of the C3-position of 2,3-disubstituted indoles 73 to the iminium ion, thus giving dearomatized intermediates U. Subsequent isomerization and cyclization of intermediates U provided tricyclic hydrocarbazoles 74 in good to excellent yields with high enantioselectivities.
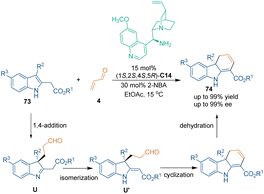 |
| Scheme 24 Catalytic asymmetric [3 + 3] cycloaddition of 2,3-disubstituted indole and acroleins. | |
Unsaturated carbonyl compounds with an additional electron-withdrawing group showed higher reactivity in [3 + 3] cycloadditions. For example, in 2014, a formal [3 + 3] spiroannulation of unsaturated ketones 76 bearing an electron-withdrawing cyano group with β-ketoamides 75 was developed by Rodriguez's group (Scheme 25).59 In this transformation, Michael addition occurred between β-ketoamides 75 and cinnamoyl cyanides 76 in the present of Takemoto's thiourea catalyst C15 (TS-6). Subsequent lactamization with a cyanide ion as a leaving group constructed optically pure azaspiro[4,5]decanones 77 efficiently.
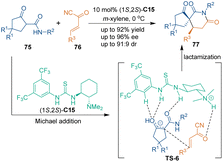 |
| Scheme 25 Catalytic asymmetric [3 + 3] cycloaddition of β-ketoamides and cinnamoyl cyanides. | |
Similar to cinnamoyl cyanides 76, β,γ-unsaturated α-ketoesters 78 could also participate in organocatalytic asymmetric [3 + 3] cycloadditions as three-carbon building blocks. From 2016 to 2020, the groups of Wang60,62 and Guo61 reported a series of organocatalytic [3 + 3] cycloadditions involving β,γ-unsaturated α-ketoesters 78 (Scheme 26). Under the catalysis of quinine-derived bifunctional tertiary amine–thiourea catalysts C16–C18, isatin-derived unsaturated α-ketoesters 78 could undergo tandem Michael addition–cyclization with carbonyl compounds (79, 81, 83, 85) as 1,3-dinucleophiles to construct a variety of chromene-based spirooxindole skeletons (80, 82, 84, 86) bearing an all-carbon six-membered ring. Notably, with this class of organocatalytic [3 + 3] cycloaddition, Guo's group developed a novel synthesis route to biologically interesting purine nucleoside analogues (Scheme 26c).61 These reactions provided efficient and stereoselective approaches toward the construction of biologically important spirooxindole scaffolds.
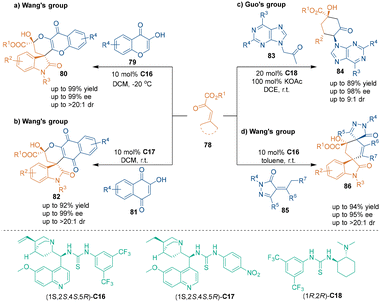 |
| Scheme 26 Catalytic asymmetric [3 + 3] cycloadditions of β,γ-unsaturated α-ketoesters and carbonyl compounds. | |
In 2020, Xu's group designed rhodanine-derived ketoesters 88 as three-carbon building blocks in organocatalytic asymmetric [3 + 3] cycloadditions with pyrazolones 87, which provided a unique approach for constructing enantioenriched spirorhodanine skeletons 89.63 The cinchona alkaloid-derived squaramide catalyst C12 exhibited excellent enantiocontrol in this [3 + 3] annulation by activating both substrates synergistically (Scheme 27). The H-bonding interactions between the amide groups of catalyst C12 and the carbonyl groups of substrates 88 enhanced the electrophilicity of 88 and facilitated control of the stereoselectivity. Additionally, the tertiary amine group of catalyst C12 captured the H atom of pyrazolones 87 to facilitate the formation of the enolized pyrazoles. Then, the Si face attack of pyrazolones 87 to ketoesters 88 gave Michael addition adducts, which further underwent an intramolecular hemiketalization to yield six-membered spirorhodanine heterocycles 89. Furthermore, the Ac group stabilized the final products by preventing the formation of hemiacetals.
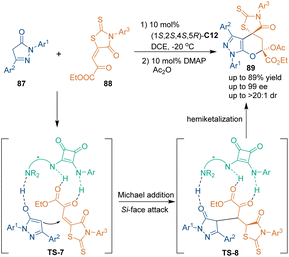 |
| Scheme 27 Catalytic asymmetric [3 + 3] cycloaddition to construct spirorhodanine skeletons. | |
Installing a leaving group (LG) on carbonyl compounds could prevent the generation of unstable hemiacetals. Based on this consideration, in 2020, Li's group constructed tetrahydro-α-carbolinone skeletons 92 by using α,β-unsaturated imides 91 bearing a LG group as three-carbon building blocks (Scheme 28).64a They found that the [3 + 3] cycloaddition between 91 and 90 proceeded smoothly when using succinimide as a leaving group. Meanwhile, it was discovered that using quinidine squaramide C12 as a chiral organocatalyst for this reaction could provide the best enantioselectivity. In the proposed reaction pathway, it was deduced that bifunctional catalyst C12 activated both substrates synergistically. The nucleophilic addition between enamines 90 and imides 91 determined the absolute configuration of products 92, and a subsequent intramolecular amidation reaction accomplished the [3 + 3] cycloaddition to construct tetrahydro-α-carbolinone frameworks with good enantioselectivities. Additionally, Xu's group also demonstrated that benzofuran-derived binucleophiles could also take part in organocatalytic asymmetric [3 + 3] cycloaddition to generate chiral polycyclic 1,4-dihydropyridines.64b
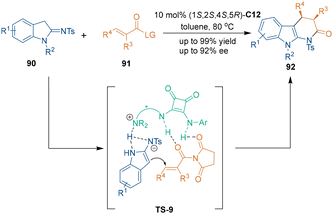 |
| Scheme 28 Catalytic asymmetric [3 + 3] cycloaddition of indolin-2-imines and unsaturated imides. | |
3.3 Unsaturated nitriles and imines as three-carbon building blocks
An organocatalytic asymmetric [3 + 3] cycloaddition involving unsaturated nitriles as three-carbon building blocks was first demonstrated in 2010 by Yuan and co-workers, who reported an enantioselective [3 + 3] cycloaddition of isatylidene malononitrile derivatives 93 and 1,3-diones (Scheme 29a).65 Biologically active spiro[4H-pyran-3,3′-oxindole] skeletons 92 were constructed in a highly enantioselective manner under the catalysis of chiral organocatalyst C19. They also explored a step-economical strategy by developing one-pot, three-component reactions. No significant changes were observed in one-pot reactions compared with the results in two-component reactions. Several years later, Shi's group established a related three-component transformation using the same catalyst, C19 (Scheme 29b).66 In this approach, biologically valuable tetrahydroquinolin-5-one-based spirooxindoles 96 were obtained through catalytic enantioselective three-component [3 + 3] cycloaddition of dimedone-derived enaminones 95 with isatins and malononitrile. As shown in Scheme 29b, the unsaturated nitriles generated from the Knoevenagel condensation of isatins and malononitrile acted as 1,3-dielectrophiles in this organocatalytic reaction. Both nucleophiles 95 and electrophiles were simultaneously activated by chiral organocatalyst C19 through hydrogen-bonding interactions in the Michael addition step. Then, the Michael addition products underwent isomerization from imine-type to enamine-type, which enabled subsequent intramolecular nucleophilic addition to construct tetrahydroquinoline skeletons 96 and regenerate the catalyst.
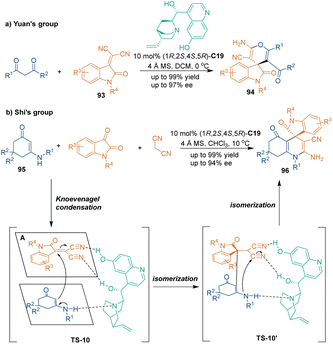 |
| Scheme 29 Catalytic asymmetric [3 + 3] cycloadditions involving isatylidene malononitrile derivatives. | |
Unsaturated nitriles could also be used as three-carbon building blocks in thio-[3 + 3] cycloadditions. In 2015, Wang's group realized an organocatalytic asymmetric thio-[3 + 3] cycloaddition of indoline-2-thiones 97 and 2-benzylidenemalononitriles 98 (Scheme 30a).67 Thiourea–tertiary amine catalyst C20 derived from a chiral 1,2-diamine showed the best enantiocontrol in this cascade reaction to afford products 99 in high yields with excellent enantioselectivities. Notably, density functional theory (DFT) calculations demonstrated that both substrates were activated by the thiourea–tertiary amine catalyst synergistically.
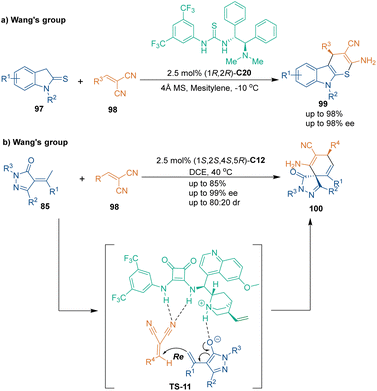 |
| Scheme 30 Catalytic asymmetric [3 + 3] cycloadditions involving 2-benzylidenemalononitriles. | |
Four years later, another [3 + 3] annulation for the synthesis of chiral spirocyclohexadiene–pyrazolones was reported by the same group (Scheme 30b).68 In this approach, α-arylidene pyrazolinones 85 underwent Michael addition/intramolecular addition with 2-benzylidenemalononitriles 98 under the catalysis of cinchona alkaloid-derived squaramide tertiary amine catalyst C12. Although products 100 were generated with only moderate diastereoselectivities, the enantioselectivities were well controlled.
Similar to unsaturated nitriles, azadienes are suitable electrophiles and can serve as three-carbon building blocks in organocatalytic asymmetric [3 + 3] cycloadditions. In 2015, the groups of Chen69 and Wang70 independently reported organocatalytic asymmetric [3 + 3] cycloadditions involving azadienes 102 with different reaction partners (Scheme 31). In Chen's approach, N-sulfonyl ketimines 102 could react with aliphatic ketones 101 to synthesize 2-benzoisothiazole-1,1-dioxides 103 in the presence of chiral catalyst C14 (Scheme 31a). While with Wang's approach, the same azadienes 102 could undergo [3 + 3] cycloaddition with indoline-2-thiones 97 to construct spiro-indole derivatives 104 bearing a spiro quaternary stereogenic center through the formation of C–N/C–S bonds, which offered a useful protocol for the construction of sulfur-containing six-membered chiral heterocycles (Scheme 31b).
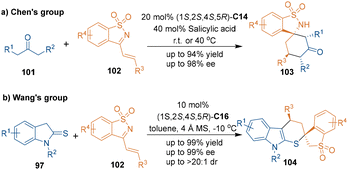 |
| Scheme 31 Catalytic asymmetric [3 + 3] cycloadditions involving azadienes. | |
3.4 Other compounds as three-carbon building blocks
Benefitting from high catalytic activity and compatibility with chiral bifunctional organocatalysts, various substrates could act as three-carbon building blocks and participate in asymmetric [3 + 3] cycloadditions through hydrogen-bonding activation.
In 2016, 4-arylidene-2-aryloxazol-5(4H)-ones 105, a kind of lactam-type compound, were utilized in catalytic asymmetric [3 + 3] cycloaddition with dimedones 47 under the catalysis of chiral bifunctional organocatalyst (S)-C21 by Shi and coworkers (Scheme 32a).71 Various hexahydrocoumarins 106 were synthesized in good enantioselectivity and excellent diastereoselectivity through a reaction pathway of Michael addition/intramolecular ester exchange. Interestingly, the enantioselectivity of most products could be improved significantly through simple recrystallization. Notably, 1H NMR experiments provided strong evidence of hydrogen-bonding activation in both substrates. For example, the 1H NMR signal of the methyl group of catalyst (S)-C21 shifted to low field, and the same phenomenon was observed for the aromatic H atom of (S)-C21. These phenomena demonstrated the existence of H-bonding interactions between the tertiary amine group of (S)-C21 and dimedones 47. A related report by Zhou and coworkers was further proof for the application of this class of lactam-type compound 105 as a three-carbon building block in organocatalytic asymmetric [3 + 3] cycloadditions (Scheme 32b).72
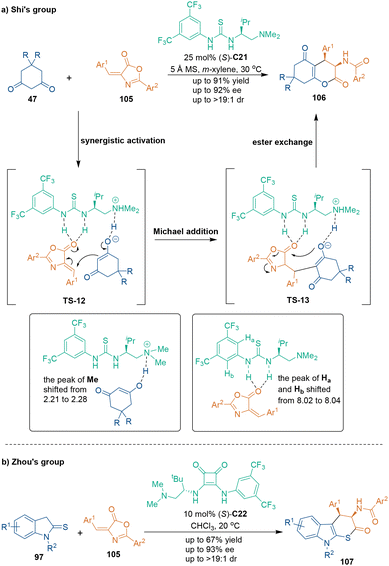 |
| Scheme 32 Catalytic asymmetric [3 + 3] cycloadditions involving 4-arylidene-2-aryloxazol-5(4H)-ones. | |
As a class of powerful building blocks, allenes show great reactivity in cycloadditions.73 In 2016, Tong's group realized asymmetric [3 + 3] cycloadditions of β′-acetoxy allenoates 109 with 3-oxo-nitriles 108 or pyrazolones 111 (Scheme 33).74 Amino acid derived tertiary amine–quinine catalyst C23 played triple catalytic roles in this transformation: (1) the tertiary amine group acted as a Lewis base to interact with allenoates 109 to form the 1,3-diene-2-aminium; (2) the amine group acted as a H-bond donor to bind with nucleophiles; (3) the tertiary amine group from the amino acid unit acted as a Brønsted base to deprotonate ketones 108 or 111. This method provided an efficient approach for constructing multiple substituted 4H-pyran skeletons in excellent yields and enantioselectivities. Downstream transformations of pyrano[2,3-c]pyrazole 112 led to compound 113, analogs of which have good fungicide activity.
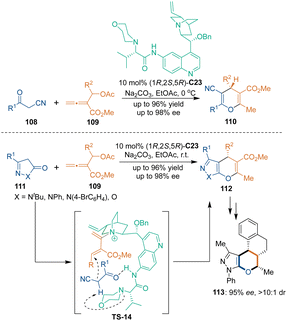 |
| Scheme 33 Catalytic asymmetric [3 + 3] cycloadditions involving β′-acetoxy allenoates. | |
When exploring δ-acetoxy allenoates 114 as bielectrophiles in catalytic asymmetric [3 + 3] cycloadditions, a similar activation mode could be applied to such transformations (Scheme 34).75 Quinine-derived catalyst C24 bearing a perfluorobenzamido group activated allenoates 114via covalent and H-bonding interactions. The first conjugate addition determined the absolute configuration of products 115, and oxa-Michael addition furnished chiral products 115 and regenerated catalyst C24. Notably, the tricyclic core of calyxin I could be constructed by applying this methodology.
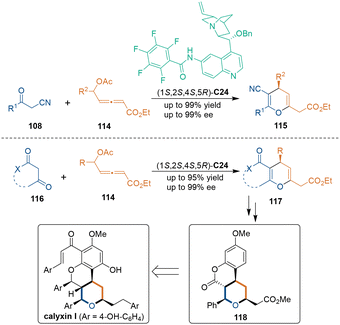 |
| Scheme 34 Catalytic asymmetric [3 + 3] cycloadditions involving δ-acetoxy allenoates. | |
Likewise, Morita–Baylis–Hillman (MBH) carbonates belong to a class of reactive substrates that can be activated by tertiary amine-derived catalysts through covalent interactions. In 2017, Guo's group reported the first catalytic asymmetric synthesis of spirocyclohexenes through [3 + 3] cycloaddition between MBH carbonates and unsaturated pyrazolones (Scheme 35).76 They suggested that catalyst C25 acted both as a Lewis base and as a hydrogen bond donor in this mechanism. Initially, the tertiary amino group of catalyst C25 attacked MBH carbonates 119 to generate intermediates V, and the deprotonation of 85 led to strong nucleophilicity at the δ-position of pyrazolones 85. Subsequent conjugate addition between intermediates W and X generated the first stereocenter. After proton transfer and enolization, the intramolecular attack of intermediates Z yielded [3 + 3] cycloadducts with three chiral centers in high diastereo- and enantioselectivities.
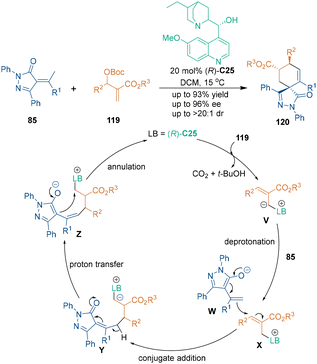 |
| Scheme 35 Catalytic asymmetric [3 + 3] cycloaddition of MBH carbonates and pyrazolones. | |
Additionally, yne–enones can act as three-carbon building blocks in organocatalytic asymmetric [3 + 3] cycloadditions. In 2016, Zhang's group disclosed an asymmetric [3 + 3] cycloaddition of 2-(1-alkynyl)-2-alken-1-ones 122 with 1,3-dicarbonyl compounds 121 in the presence of bifunctional chiral thiourea–tertiary amine catalyst C26 (Scheme 36).77 This transformation proceeded smoothly to construct various penta-carbon-substituted 4H-pyran skeletons 123 in good yields with high enantioselectivities. Bifunctional catalyst C26 activated substrates 121 and 122 simultaneously through H-bonding interactions in the 1,4-addition step (TS-15), and the resulting allene-type intermediates AC underwent 6-endo-trig cyclization to produce oxygen-containing six-membered heterocycles 123. Notably, cyclic ketones such as dimethyl barbituric acid were also compatible in this [3 + 3] cycloaddition.78
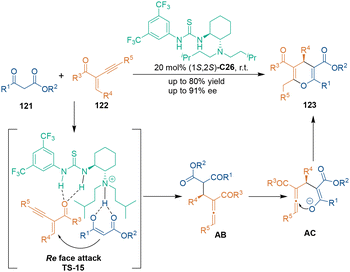 |
| Scheme 36 Catalytic asymmetric [3 + 3] cycloaddition of yne-enones and dicarbonyl compounds. | |
4. Chiral N-heterocyclic carbene-catalyzed asymmetric [3 + 3] cycloadditions
4.1 Unsaturated aldehydes as three-carbon building blocks
Chiral N-heterocyclic carbenes (NHCs) are known as powerful covalent catalysts in organocatalytic asymmetric transformations.79 Classic Breslow intermediates formed from aldehydes and chiral NHCs enabled excellent enantiocontrol in such reactions through forming covalent bonds. Chiral NHC catalysis was first utilized in asymmetric [3 + 3] cycloadditions by Bode's group in 2010 (Scheme 37).80 In this approach, they employed ynal 125 as three-carbon building blocks, which could be activated by chiral NHC catalyst C27, to undergo [3 + 3] cycloadditions with ketoester 124 and 2-naphthol 127, respectively, thus constructing six-membered rings 126 and 128 in moderate to high enantioselectivities. Notably, the reaction of ynal 125 with 2-naphthol 127 involved a process of Claisen rearrangement (TS-16).
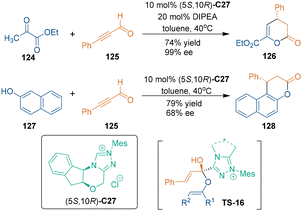 |
| Scheme 37 Catalytic asymmetric [3 + 3] cycloadditions involving ynal and enols. | |
Subsequently, in 2021, Xiao's group reported a chiral NHC-catalyzed [3 + 3] cycloaddition of unsaturated aldehydes 129 or 4 with 1,3-dicarbonyls (Scheme 38), wherein both ynals 129 and enals 4 could result in enantioenriched dihydropyranones 130.81 In the proposed reaction pathway, the reactive electrophilic unsaturated acyl azolium AD could be generated from unsaturated ynals 129 or oxidation of the Breslow intermediate derived from α,β-unsaturated enals 4. Then, two rounds of addition between 1,3-dicarbonyls and AD yielded final product 130 and released NHC catalyst (5S,10R)-C27.
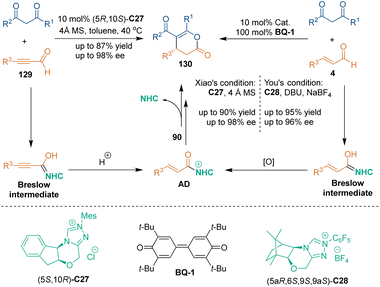 |
| Scheme 38 Catalytic asymmetric [3 + 3] cycloaddition of 1,3-dicarbonyls, ynal and enals. | |
Almost at the same time, You and coworkers realized a related reaction in the presence of a bulky triazolium salt and DBU.82 A catalytic amount of NaBF4 could improve the enantioselectivity through the interaction with the C6F5 group of C28. Cinnamaldehyde derivatives and dicarbonyl compounds could participate in this [3 + 3] cycloaddition in good yields and excellent enantioselectivities. Further investigations by the groups of Biju,83 Yao,84 Zhong,85 and Sundén86 demonstrated the compatibility of different substrates in this catalytic system.
Using cyclic N-sulfonylimines 131 bearing an α-H as nucleophiles and three-atom building blocks, chiral lactams 132 could be synthesized through chiral NHC-catalyzed [3 + 3] cycloaddition by Bode's group (Scheme 39a).87 They proposed a condensation mechanism between acyl azolium AF and enamine isomers of substrates 131. Subsequently, hemiaminal AG underwent Stork–Hickmott–Stille-type annulation via an aza-Claisen-type transition state. Subsequent isomerization from imine AH to enamine AI led to lactonization and finished the catalytic cycle. It is worth noting that high enantioselectivities were observed when both aliphatic and aromatic cyclic imines 131 were examined. Additionally, when employing trisubstituted enals as substrates, the [3 + 3] cycloaddition would afford cyclic products bearing two chiral centers. Based on this anticipated result, in 2019, Enders’ group reported that chiral pentacyclic spirooxindoles 134 were synthesized by using isatin-derived enals 133 as reactants in chiral NHC C27-catalyzed asymmetric [3 + 3] cycloaddition (Scheme 39b).88
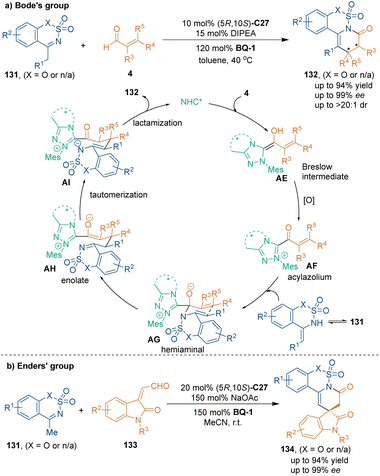 |
| Scheme 39 Catalytic asymmetric [3 + 3] cycloadditions of cyclic N-sulfonylimines and enals. | |
In 2013, Chi's group revealed that acyclic enamines 19 could also participate in chiral NHC C29-catalyzed [3 + 3] cycloaddition with α,β-unsaturated esters 135 through LUMO activation (Scheme 40a),89 which generated various six-membered lactams 136 with excellent enantioselectivities. Moreover, bulky β,β-disubstituted unsaturated esters are also compatible with this methodology, which affords lactams with a quaternary stereogenic center in high enantioselectivities. Two years later, Zhong's group explored the chiral NHC C27-catalyzed [3 + 3] cycloaddition of oxindole-derived enals 133 with acyclic imines 19a (Scheme 40b).90 Interestingly, this transformation only took one hour or less to construct bioactive spirooxindole skeletons 137 with excellent enantioselectivities.
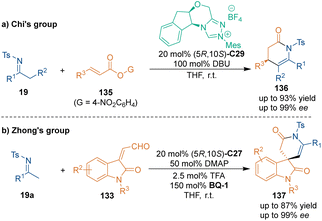 |
| Scheme 40 Catalytic asymmetric [3 + 3] cycloadditions involving acyclic enamines. | |
Later, several relevant works reported by the groups of Zhong,91 Cheng,92 Liu,93 Chen94 and Qi95 demonstrated the advantages of this method for the synthesis of multi-substituted dihydropyridinone analogues. In addition, Guin and coworkers developed NHC C29-catalyzed [3 + 3] cycloaddition of substituted malonamides 138 with enals 4, producing glutarimide derivatives 139 with excellent diastereo- and enantioselectivities (Scheme 41). Notably, they demonstrated the application of this synthesis method by the formal synthesis of (−)-paroxetine, a marketed drug.96
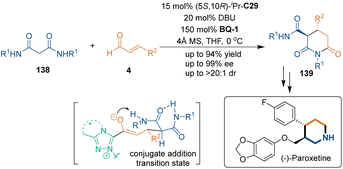 |
| Scheme 41 Catalytic asymmetric [3 + 3] cycloaddition of malonamides and enals. | |
In 2016, Biju's group constructed chiral all-carbon six-membered rings 140 through asymmetric [3 + 3] cycloaddition between α,β-unsaturated aldehydes 4 and α-arylidene pyrazolinone 35 under the catalysis of NHC C27 (Scheme 42).97 With this protocol, various spirocyclohexadienones 140 bearing an all-carbon quaternary stereocenter were synthesized with excellent enantioselectivities. In their assumption, NHC C27 activated enals 4 to form unsaturated acyl azoliums AK. Meanwhile, dienolate/enolate intermediates were generated in the presence of DBU. This cooperative interaction played an important role in the excellent enantiocontrol of this transformation.
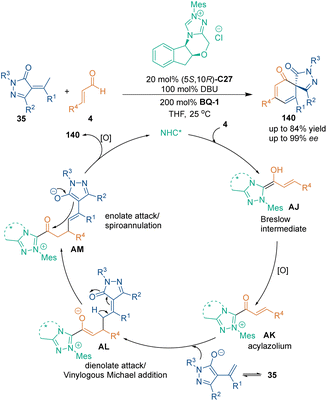 |
| Scheme 42 Catalytic asymmetric [3 + 3] cycloaddition of pyrazolinones and enals. | |
In recent years, the organocatalytic atroposelective construction of axially chiral (hetero)aryl-based scaffolds has become an emerging field due to the importance and potential applications of such scaffolds in developing chiral catalysts or ligands and discovering bioactive molecules.14,18,19,98 In this context, other than six-membered heterocycles bearing central chirality, axially chiral aryl-based scaffolds could also be constructed by chiral NHC-catalyzed [3 + 3] cycloaddition (Scheme 43). In 2018, Wang's group reported the atroposelective [3 + 3] cycloaddition of ynals 141 with dimedones 47 under the catalysis of NHC C30,99a which offered axially chiral α-pyrone-aryls 142 with high enantioselectivities (Scheme 43a). The newly synthesized axially chiral compounds 142 showed relatively high configurational stability, and the rotational barrier between the two isomers was 119.7 kJ mol−1 at 85 °C. In the suggested reaction pathway, Breslow intermediate AN was oxidized to generate alkynyl acyl azolium AO. Then, cyclic 1,3-diones 47 tautomerized to enols AP and attacked acyl azoliums in the presence of a Lewis acid, Mg(OTf)2. Then, the allenolates AQ, yielded from Michael addition, underwent proton transfer to form AR, which further underwent lactonization to give axially chiral biaryls 142 and regenerated NHC catalyst C30. An additional control experiment ruled out the possibility of chirality transfer from central-to-axial. This work demonstrated the potential of organocatalytic asymmetric [3 + 3] cycloadditions for constructing axially chiral scaffolds.
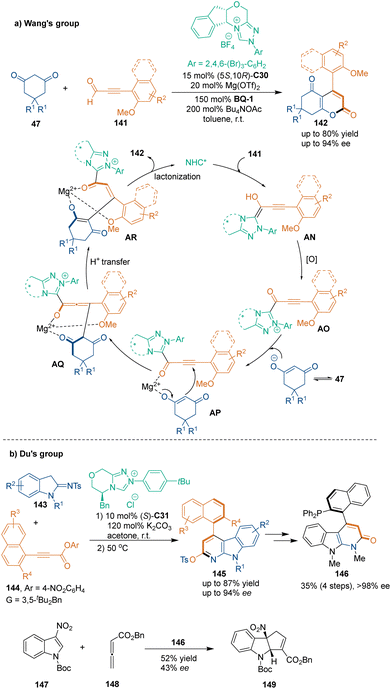 |
| Scheme 43 Catalytic asymmetric atroposelective [3 + 3] cycloadditions for constructing axially chiral scaffolds. | |
Another example for constructing axially chiral scaffolds was reported in 2021 by Du's group, who used 2-sulfonamidoindolines 143 as reaction partners to undergo an atroposelective [3 + 3] cycloaddition with substrates 144via NHC catalysis (Scheme 43b).99b By this reaction, axially chiral heterobiaryls compounds 145 were synthesized in good enantioselectivities. Notably, the modification of 4-aryl α-carboline atropisomers 145 led to axially chiral phosphine organocatalyst 146, which showed some degree of catalytic activity in Lu's [3 + 2] cycloaddition.
4.2 Halo-substituted unsaturated aldehydes as three-carbon building blocks
A pre-installed leaving group on unsaturated aldehydes could lead to α,β-unsaturated acylazolium intermediates without using stoichiometric oxidants. In the early 2010s, the groups of Ye100 and Biju101 reported chiral NHC-catalyzed asymmetric [3 + 3] cycloadditions of α-bromoenals with 1,3-dicarbonyl compounds successively. As shown in Scheme 44a, these transformations were triggered by the addition of NHC catalysts to 2-bromoenals 150. The Breslow intermediates AS could be tautomerized to bromoketones AT, which underwent elimination to yield acylazolium intermediates AU. Then, 1,3-dicarbonyl compounds 90 served as suitable binucleophiles to undergo Michael addition with intermediates AU and subsequent lactonization, which afforded the desired dihydropyranones 151 with high yields and enantioselectivities. Interestingly, Ye's group found that different substituents on the NHC catalysts C32 and C33 with the same absolute configuration would lead to products 151 with opposite configurations.
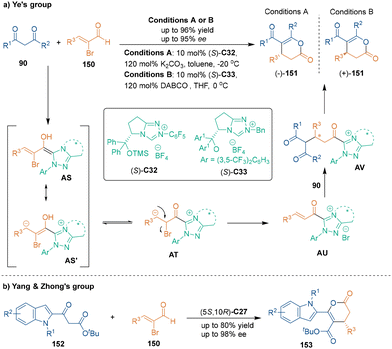 |
| Scheme 44 Catalytic asymmetric [3 + 3] cycloadditions of 2-bromoenals and dicarbonyl compounds. | |
Recently, Yang, Zhong and co-workers utilized indole-derived β-ketoesters 152 as annulation partners in NHC-catalyzed asymmetric [3 + 3] cycloaddition with 2-bromoenals 150, constructing chiral 6-(indole-2-yl)-3,4-dihydropyran-2-one skeletons 153 in good yields with excellent enantioselectivities (Scheme 44b).102 In this reaction, excellent enantiocontrol was achieved through NHC-bound vinyl acyl azolium intermediates. This work provides an efficient and enantioselective method toward the synthesis of chiral indole-based six-membered heterocycles.
In addition to highly reactive 1,3-dicarbonyl compounds, enolizable aldehydes and ketones could participate in NHC-catalyzed asymmetric [3 + 3] cycloadditions with α-bromoenals. In 2013, Biju's group utilized phenyl acetaldehyde derivatives 154 to synthesize 4,5-disubstituted dihydropyranones 155via sequential Michael addition/lactonization (Scheme 45a).103 Aminoindanol-derived triazolium salt C27 exhibited high catalytic activity for enantiocontrol in this transformation. Moreover, the groups of Enders (Scheme 45b)104 and Yao (Scheme 45c)105 demonstrated that cyclic ketones such as indolin-3-ones 156 and β-tetralones 49 were also efficient nucleophiles for reacting with α-bromoenals 150 in NHC-catalyzed [3 + 3] cycloadditions, which afforded biologically intriguing dihydropyranoindol-2-ones 157 and naphthopyrans 158, respectively.
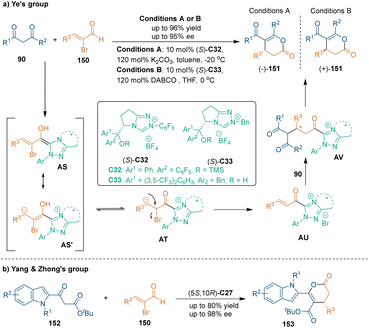 |
| Scheme 45 Catalytic asymmetric [3 + 3] cycloadditions of 2-bromoenals and carbonyl compounds. | |
As the precursors of enamines, imines bearing an α-H could undergo NHC-catalyzed [3 + 3] cycloadditions with α,β-unsaturated acylazoliums (Scheme 46). Elegant work from the groups of Ye106,107,109 and Enders108 proved the capability of both cyclic ketimines and aldimines to be competent three-atom building blocks in NHC-catalyzed [3 + 3] cycloadditions. Through these transformations, various dihydropyridinone derivatives were synthesized with excellent enantioselectivities (Scheme 46a–d). Similarly, Zhong's group discovered that hydrazones could serve as suitable substrates for constructing chiral dihydropyridazone skeletons (Scheme 46e).110
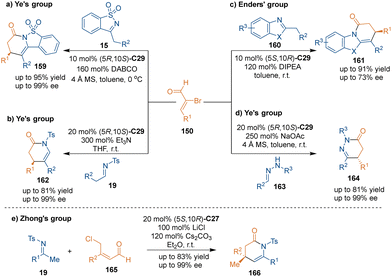 |
| Scheme 46 Catalytic asymmetric [3 + 3] cycloadditions involving imines and hydrazones. | |
Substituted dihydropyridones bearing two stereogenic centers could be constructed through dynamic kinetic resolution (DKR). In 2018, Ye's group reported an NHC-catalyzed [3 + 3] cycloaddition of α-bromoenals 150 and enamines 167 (Scheme 47).111 Both cyclic and acyclic enamines 167 could tautomerize to racemic isomers in the presence of NHC catalyst C34 and Et3N. The higher reactivity of (S)-AAB in the Michael addition step facilitated the dynamic kinetic resolution of 167. Aminoindanol-derived tetracyclic triazolium C34 provided excellent enantio- and diastereocontrol in the formation of dihydropyridinones 168. Meanwhile, NHC catalysis also exhibited high efficiency in the kinetic resolution (KR) of α,α-disubstituted imines rac-169. Excellent enantioselectivities were observed in both dihydropyridinone products 170 and the recovered substrates 169, and the selectivity factor (S) was up to 83.
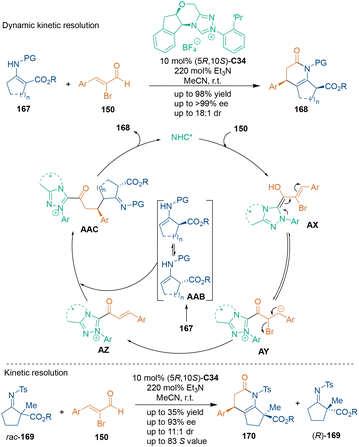 |
| Scheme 47 Catalytic asymmetric [3 + 3] cycloadditions through DKR and KR of enamines. | |
In 2020, Wang's group disclosed a weak nucleophile-based [3 + 3] cycloaddition using 2-aminobenzimidazoles 171 as N-nucleophiles to react with α-bromoenals 150 (Scheme 48).112 Morpholinone-derived sterically hindered triazolium catalyst C35 could catalyze the reaction to afford dihydropyrimido[1,2-a]benzimidazole-2(1H)-ones 172 with excellent enantioselectivities. An additional biological investigation revealed the moderate cytotoxic activity of several cycloadducts against human/murine tumor cells. A similar strategy involving amidines 173 also realized dual C–N bond formation in one reaction.113
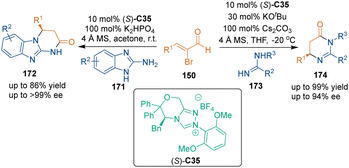 |
| Scheme 48 Catalytic asymmetric [3 + 3] cycloadditions of imidazole derivatives and 2-bromoenals. | |
Other nitrogen-containing nucleophilic substrates could also serve as suitable building blocks in NHC-catalyzed asymmetric [3 + 3] cycloadditions, which demonstrated the effectiveness of NHC catalysts in controlling the stereoselectivity. For example, enantioenriched δ-carbolinones could be synthesized from 3-aminoindoles and 2-bromoenal according to Du's report.114 Moreover, Biju's group utilized racemic N-aryl aminomaleimides rac-175 as three-atom building blocks in NHC-catalyzed asymmetric [3 + 3] cycloaddition with α-bromoenals 150via a kinetic resolution process, which simultaneously afforded chiral products 177 and recovered substrates 175 bearing C–N axial chirality (Scheme 49).115 Notably, the [3 + 3] cycloaddition produced dihydropyridinones 177 bearing both C–N axial chirality and central chirality with moderate diastereoselectivities and good enantioselectivities. An additional investigation demonstrated that the [3 + 3] cycloadducts 177 had a higher C–N rotational barrier than maleimide 175.
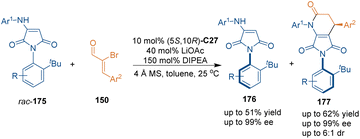 |
| Scheme 49 Catalytic asymmetric [3 + 3] cycloaddition of maleimides and 2-bromoenals. | |
Apart from nitrogen-containing heterocycles, sulfur-containing six-membered heterocycles could be synthesized via organocatalytic asymmetric [3 + 3] cycloadditions. In 2019, Biju's group reported that thioamides 178 could take part in asymmetric [3 + 3] cycloaddition with bromoenals 150 under the catalysis of Nitro-substituted C35 (Scheme 50a).116 They proposed a tentative mechanism for this cycloaddition, which involved the generation of a classic unsaturated acylazolium through elimination of bromide from Breslow intermediate AAD. Then, thioamides 178 underwent conjugate addition to form intermediate AAE. Subsequent proton transfer and intramolecular lactamization yielded final products 179. Another possible pathway involved 1,2-addition from a hard nitrogen center and subsequent [3,3] sigmatropic rearrangement to give the same intermediate AAF to continue the catalytic cycle. Three years later, the same group developed another efficient method towards the construction of chiral sulfur-containing six-membered heterocycles. In this approach, they used β-oxodithioesters 180 as nucleophilic building blocks in NHC-catalyzed asymmetric [3 + 3] cycloaddition with bromoenals 150, which constructed chiral dihydrothiopyranone skeletons 181 with good enantioselectivity and regioselectivity (Scheme 50b).117
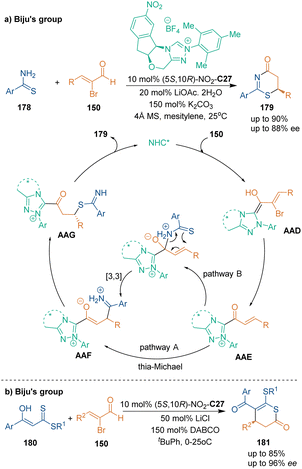 |
| Scheme 50 Catalytic asymmetric [3 + 3] cycloadditions to construct sulfur-containing heterocycles. | |
4.3 Saturated aldehyde as three-carbon building blocks
Direct β-functionalization of saturated aldehydes was less explored in NHC-catalyzed reactions. In 2013, Chi's group developed a [3 + 3] cycloaddition using saturated aldehydes 182 as pre-Michael addition acceptors (Scheme 51).118 They designed a unique process of double oxidation to generate an α,β-unsaturated acyl azolium. Namely, the first oxidation occurred on Breslow intermediates AAH, while the second oxidation yielded Michael addition acceptors AAK, which could react with 1,3-dicarbonyl compounds 90 in a highly selective manner. Furthermore, an electron-pair-transfer pathway was proposed for quinone-based oxidation, i.e., the oxa-Michael addition gave intermediates AAM and the capture of acidic β-C–H provided the key intermediate of unsaturated acyl azolium AAK.
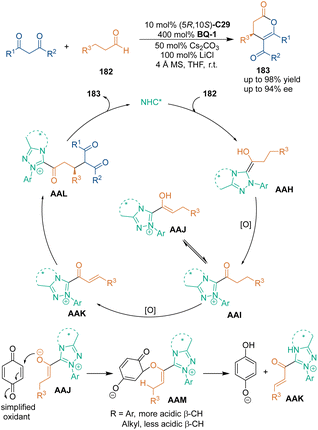 |
| Scheme 51 Catalytic asymmetric [3 + 3] cycloaddition of saturated aldehyde and 1,3-diketones. | |
5. Chiral phosphoric acid-catalyzed asymmetric [3 + 3] cycloadditions
5.1 Indolylmethanols as three-carbon building blocks
Chiral phosphoric acids (CPAs) have been regarded as powerful chiral organocatalysts in asymmetric catalysis and synthesis119 since the pioneering works of Akiyama119a and Terada119b in 2004. This unique class of Brønsted acids could activate substrates through hydrogen-bonding or ion-pairing interactions. The dual catalysis model enabled wide applications of CPAs in catalytic asymmetric transformations like Friedel–Crafts reactions, Diels–Alder reactions and dearomatization reactions.119 The CPA-catalyzed [3 + 3] cycloaddition was first explored by Gong's group in 2013 (Scheme 52a).120 In this approach, they used 3-indolylmethanols 186 as three-carbon building blocks to undergo CPA-catalyzed [3 + 3] cycloaddition with azomethine ylides, which were generated in situ from aromatic aldehyde 184 and amino esters 185, thus synthesizing various tetrahydro-β-carbolines 187via a three-component one-pot reaction. In the proposed reaction pathway, aryl 3-indolylmethanols 186 underwent protonation and dehydration in an acidic catalytic environment. The resulting carbocation intermediates could tautomerize to reactive vinyliminium intermediates, which could be attacked by azomethine ylides. Afterwards, the intramolecular Pictet–Spengler reaction finished the catalytic cycle and yielded the annulation products 187 with good enantioselectivities and excellent diastereoselectivities.
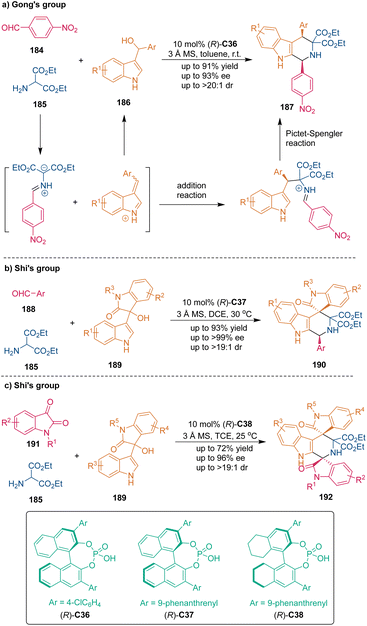 |
| Scheme 52 Catalytic asymmetric [3 + 3] cycloadditions involving 3-indolylmethanols. | |
Shortly after, in 2014, related work by Shi's group realized the construction of tetrahydro-β-carboline-based spirooxindole skeletons 190 with two stereogenic centers using isatin-derived indolylmethanols 189 as reactants in CPA-catalyzed asymmetric [3 + 3] cycloaddition (Scheme 52b).121 Through this protocol, pharmaceutically attractive spiro[indoline-3,4′-pyridoindoles] 190 containing an all-carbon quaternary stereogenic center were obtained with moderate diastereoselectivities and excellent enantioselectivities.
To construct structurally more complex and challenging bispirooxindole scaffolds, the same group utilized isatins 191 as reactants in this class of CPA-catalyzed asymmetric [3 + 3] cycloadditions with isatin-derived indolylmethanols 189 in the presence of CPA C38 (Scheme 52c).122 Structurally more rigid H8-BINOL-based CPA C38 exhibited high diastereocontrol in this reaction, affording enantioenriched bispirooxindole derivatives 192 containing a tetrahydro-β-carboline core with excellent diastereo- and enantioselectivities. Moreover, considering the potential bioactivity of both oxindoles and tetrahydro-β-carbolines, this methodology provided an efficient route to access such bioactive chiral molecules.
Continuous investigations from Shi's group shed some light on CPA-catalyzed asymmetric [3 + 3] cycloadditions involving 2-indolylmethanols, which were discovered to display the unique property of C3-electrophilicity in substitution reactions123a–c and cycloaddition reactions.123d In 2016, the first catalytic enantioselective and regioselective [3 + 3] cycloaddition using 2-indolylmethanols 193 as three-carbon building blocks was established by Shi's group (Scheme 53).124 In the presence of CPA (R)-C39, 2-indolylmethanols 193 could be transformed into carbocations, vinyliminiums and delocalized cations. Meanwhile, azomethine ylides were generated from aldehydes 188 and amino esters 185 with the aid of CPA (R)-C39. According to their hypothesis, CPA C39 activated both substrates synchronously via dual hydrogen-bonding interactions because blocking the N–H group of 193 thwarted this transformation. It is suggested that the steric hindrance between bulky aryl groups (Ar1 and Ar2) and the two ester groups might be responsible for the observed regioselectivity in the [3 + 3] cycloaddition.
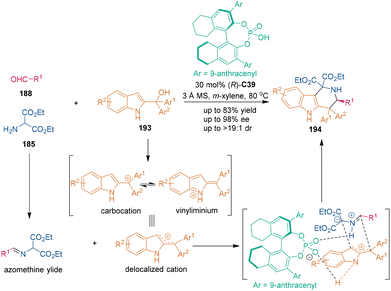 |
| Scheme 53 Catalytic asymmetric [3 + 3] cycloaddition of 2-indolylmethanols and azomethine ylides. | |
Several years later, in 2021, the C3-nucleophilicity of 2-indolymethanols was also utilized by Shi's group in CPA-catalyzed asymmetric [3 + 3] cycloaddition of nitrones 195 with 2-indolylmethanols 193 for the construction of optically pure oxacarboline frameworks 196 in an unusual regioselective manner (Scheme 54).125 A cooperative catalytic system of CPA (R)-C38 with hexafluoroisopropanol (HFIP) was established to achieve excellent control of the reactivity and the enantioselectivity of this [3 + 3] cycloaddition. As illustrated, CPA (R)-C38 activated 2-indolymethanols 193 through H-bonding interactions, while HFIP could form multiple hydrogen bonds with both the substrates and the chiral catalyst (R)-C38 to facilitate the first step of an enantioselective nucleophilic addition. DFT calculations demonstrated that HFIP played an important role during the whole reaction pathway. Namely, C–H⋯F hydrogen-bonding interactions were observed between fluorine atoms of HFIP and phenyl C–H groups of both substrates (TS-17). Additionally, apart from interactions with CPA, the OH group of HFIP formed another hydrogen bond with prochiral CH of the protonated nitrone. So, the pincer-like interactions led to a lower energy barrier of TS-17 than that of TS-17′ in the absence of HFIP, which explained the observed excellent enantiocontrol of the reaction by the cooperative catalytic system. Notably, the enantioenriched oxacarbolines 196 exhibited high cytotoxicity against the PC-3 cell line, and thus, they might find applications in medicinal chemistry.
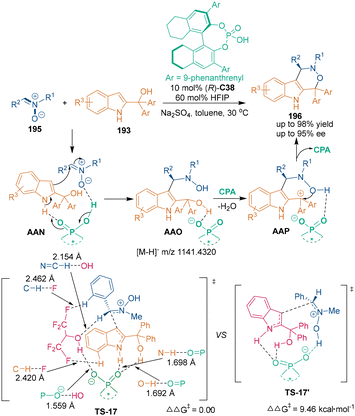 |
| Scheme 54 Catalytic asymmetric [3 + 3] cycloaddition of 2-indolylmethanols and nitrones. | |
Another application of the C3-nucleophilicity of 2-indolymethanols in [3 + 3] cycloadditions was reported in 2023 by Shi's group,126a who designed novel m-hydroxybenzyl alcohols as suitable three-atom building blocks in [3 + 3] cycloadditions with 2-indolymethanols to construct indole-fused six-membered rings in high yields with moderate enantioselectivities. Very recently, the same group established a CPA-catalyzed asymmetric [3 + 3] cycloaddition between different 2-indolylmethanols using the C3-nucleophilicity of 2-indolymethanols.126b Through this approach, a series of chiral indole-fused six-membered heterocycles were constructed in high yields with excellent enantioselectivities, which displayed promising antitumor activities. Notably, DFT calculations were performed to give an in-depth understanding of the reaction pathway and activation mode of this [3 + 3] cycloaddition.
5.2 Indolylmethanol as carbon–carbon–nitrogen building blocks
Installing a blocking group on the C3-position of 2-indolymethanols makes C3-substituted 2-indolymethanols 197 act as carbon–carbon–nitrogen building blocks in organocatalytic cycloadditions.127 In 2017, Shi's group realized a CPA-catalyzed asymmetric [3 + 3] cycloaddition between C3-substituted 2-indolylmethanols 197 and azomethine ylides, which were generated in situ from amino-ester 185 and aldehydes 188 (Scheme 55).128 Under the catalysis of H8-BINOL-derived CPA (R)-C37, chiral tetrahydropyrimido[1,6-a]indoles 198 were synthesized with good enantioselectivities and excellent diastereoselectivities. In the proposed reaction pathway, the first C–C bond formation step was the intermolecular nucleophilic addition between azomethine ylides and vinyliminium, which was formed from C3-substituted 2-indolymethanols 197via dehydration. In this step, hydrogen-bonding and ion-pairing interactions between CPA and the substrates realized enantiocontrol of the first stereogenic center. Then, the NH group of the indole ring performed an intramolecular addition with the imine group to accomplish the [3 + 3] cycloaddition. Similarly, the spirooxindole skeleton could be constructed when using isatin-derived azomethine ylides in the [3 + 3] cycloaddition.129
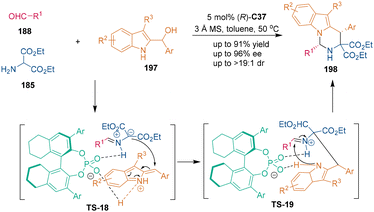 |
| Scheme 55 Catalytic asymmetric [3 + 3] cycloaddition involving 3-substituted 2-indolylmethanols. | |
5.3 Other compounds as three-atom building blocks
In addition to indolylmethanols as three-atom building blocks under CPA catalysis, some new building blocks were developed to synthesize chiral six-membered heterocycles under chiral Brønsted acid catalysis. In 2021, Peng's group reported a chiral imidodiphosphoric acid (IDP) C40-catalyzed asymmetric [3 + 3] cycloaddition of α-haloketones 199 with α-diazomethylphosphonates 200 (Scheme 56).130 Relying on ion pair interactions between chiral IDP and oxyallyl zwitterions, excellent diastereo- and enantioselectivities were achieved in this transformation. This [3 + 3] cycloaddition was supposed to undergo a stepwise pathway. Namely, the α-position of α-haloketones 199 attacked the W-shaped oxyallyl zwitterion to form two stereocenters in one step, and subsequent cyclization and isomerization afforded chiral phosphorylpyridazin-4(1H)-one products 201.
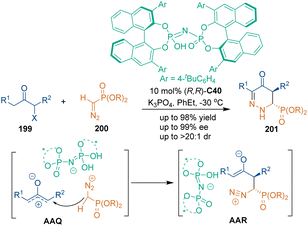 |
| Scheme 56 Catalytic asymmetric [3 + 3] cycloaddition of haloketones and diazomethylphosphonates. | |
In 2022, a catalytic asymmetric [3 + 3] cycloaddition was reported by Lin's group for the preparation of trifluoromethylated pyrazoles 204 bearing adjacent chiral centers (Scheme 57).131 As electron-rich heterocycles, N-aryl-5-aminopyrazoles 202 could undergo Friedel–Crafts alkylation with 3-trifluoroethylideneoxindole 203via a synergistic catalysis of CPA C41 with MgSO4, which was the key step to determine the enantioselectivity and the diastereoselectivity. Subsequent intramolecular transamidation gave the final pyrazolo[3,4-b]pyridin-6-one products 204. In this reaction, MgSO4 may work as a Lewis acid to coordinate with the carbonyl group of the intermediates, thus enhancing the electrophilicity during the transformation.
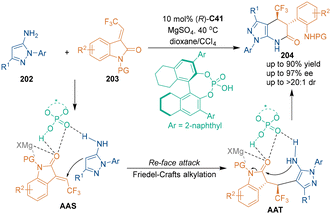 |
| Scheme 57 Catalytic asymmetric [3 + 3] cycloaddition of trifluoroethylideneoxindoles and pyrazoles. | |
6. Other organocatalytic asymmetric [3 + 3] cycloadditions
6.1 Chiral isothiourea-catalyzed asymmetric [3 + 3] cycloadditions
Chiral isothioureas were commonly employed as Lewis base catalysts in asymmetric organocatalysis.132 Under the catalysis of chiral isothioureas, good catalytic efficiency and selectivity control were achieved through chiral N-carboxy intermediates. In 2013, Smith's group discovered the novel reactivity of α,β-unsaturated acyl ammonium species, and designed a chiral isothiourea-catalyzed asymmetric [3 + 3] cycloaddition of unsaturated anhydrides 206 with ethyl benzoylacetate 205 (Scheme 58).133 In this reaction, chiral isothiourea C42 attacked unsaturated anhydrides 206 to form acyl ammonium species AAU, and subsequent Michael addition and lactonization led to the generation of dihydropyranone products 207 with high enantioselectivities.
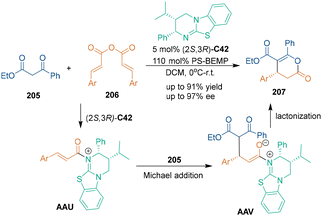 |
| Scheme 58 Catalytic asymmetric [3 + 3] cycloaddition of ethyl benzoylacetate and anhydrides. | |
Interestingly, different selectivity was observed when azaaryl ketones 208 were explored as nucleophiles in chiral isothiourea C42-catalyzed asymmetric [3 + 3] cycloaddition with unsaturated anhydrides 206 (Scheme 59).134 It was proposed that non-bonding 1,5-S–O interactions might affect the chemo- and enantioselectivity of the reaction. Both aryl and alkyl substituted acylbenzazoles 208 and 210 could undergo N-cyclization to give lactams 209 and 211 with good enantioselectivity, while 2-phenacylbenzoxazole 212 produced O-cyclization adducts 213 with excellent chemo- and enantioselectivity. In the suggested reaction pathway, chiral isothiourea C42 attacked unsaturated anhydrides 206 to yield α,β-unsaturated acyl ammonium intermediates AAW, which formed pre-cyclization intermediates AAX through subsequent 1,4-addition. Then, lactamization occurred when using benzothiazoles 208 or 210 as nucleophiles, and the non-bonding S–O interactions led to the observed high chemoselectivity. On the other hand, lactonization of benzoxazole 212 gave dihydropyranone products 213. A further investigation by the same group explored using CF3-substituted α,β-unsaturated esters as dielectrophiles in chiral isothiourea-catalyzed asymmetric [3 + 3] cycloaddition, which enabled the synthesis of polyfluorinated heterocycles.135
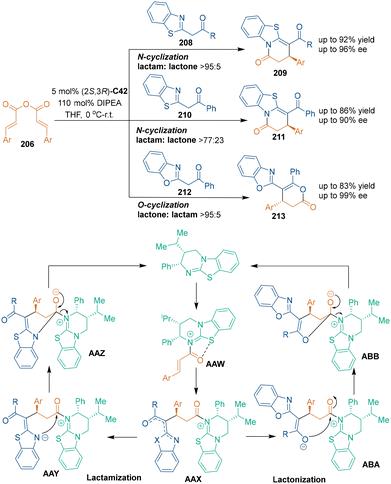 |
| Scheme 59 Catalytic asymmetric [3 + 3] cycloadditions of azaaryl ketones and anhydrides. | |
In 2020, Smith's group developed another [3 + 3] cycloaddition of trifluoromethyl enones 215 with 2-(pyrrol-1-yl)acetic acid 214 (Scheme 60).136 In the presence of chiral isothiourea catalyst C42, enantioenriched tetrahydroindolizines 216 bearing three stereogenic centers were synthesized through tandem cyclization. In the reaction pathway, a Michael addition–lactonization process afforded conventional O-cyclization products. Afterwards, ring opening triggered by nucleophiles generated 1,5-diketone intermediates ABD, following Friedel–Crafts cyclization yielded [3 + 3] cycloadducts with a new stereogenic center.
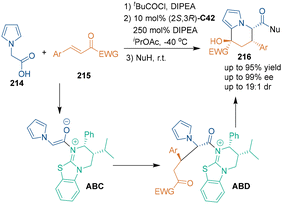 |
| Scheme 60 Catalytic asymmetric [3 + 3] cycloaddition involving trifluoromethyl enones. | |
6.2 Chiral phosphine-catalyzed asymmetric [3 + 3] cycloadditions
In the past decades, nucleophilic phosphine catalysts have had a significant impact on the synthesis of heterocyclic compounds.137 This kind of catalyst could attack electron-deficient alkenes, allenes, alkynes, or Morita–Baylis–Hillman (MBH) carbonates to form reactive phosphonium enolate zwitterions. In 2015, Guo's group developed the first chiral phosphine-catalyzed enantioselective [3 + 3] cycloaddition of MBH carbonates 218 with cyclic azomethine imines 217 (Scheme 61a),138 which offered six-membered fused heterocycles 219 in excellent enantioselectivity in the presence of chiral spirophosphine C43. This transformation has potential applications in synthesizing biologically active chiral tetrahydroisoquinolines and pyridazines.
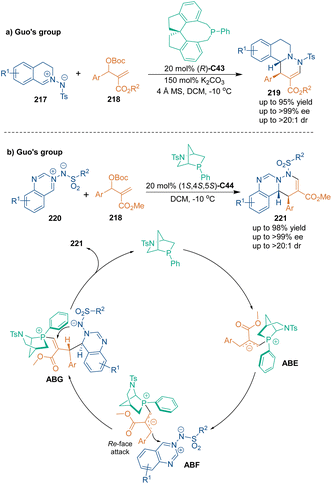 |
| Scheme 61 Chiral phosphine-catalyzed asymmetric [3 + 3] cycloadditions. | |
Two years later, enantioenriched quinazoline-fused heterocycles 221 were synthesized through chiral phosphine C44-catalyzed asymmetric [3 + 3] cycloaddition of MBH carbonates 218 with 1,3-dipoles 220 by the same group (Scheme 61b).139 The [3 + 3] cycloaddition proceeded through the formation of allylic phosphorus ylide intermediates ABE, which were generated from the nucleophilic addition of catalyst C44 to MBH carbonates 218. After isomerization, a Re-face attack occurred between ABE and 1,3-dipoles 220 to give intermediates ABG. Then, ABG underwent aza-Michael addition and subsequent elimination to construct chiral quinazoline scaffolds 221 in good yields with excellent enantioselectivities.
6.3 Cooperative catalytic asymmetric [3 + 3] cycloadditions
In the last decade, cooperative catalysis has emerged as a powerful tool in catalytic asymmetric transformations.140 This catalytic mode is helpful for developing innovative reactions that are difficult to achieve under the catalysis of a single catalyst. In 2019, Gong's group developed a copper salt and chiral NHC (S)-C45 co-catalyzed asymmetric [3 + 3] cycloaddition of isatin-derived enals 133 with ethynylethylene carbonates 222, thus constructing optically pure spirooxindole skeletons 223 with excellent diastereo- and enantioselectivities (Scheme 62).141 In the presence of a copper catalyst, ethynylethylene carbonates 222 underwent sequential deprotonation and decarboxylation to generate copper–allenylidene intermediates ABJ. Meanwhile, chiral NHC catalyst (S)-C45 activated isatin-derived enals 133 to form nucleophilic enolate intermediates ABL, which could intercept ABJ to give ABM. Subsequent lactonization and protonation yielded final spirooxindole 223 with excellent stereoselectivity. Notably, NHC catalyst (S)-C45 served as both an organocatalyst and ligand in the copper catalytic cycle.
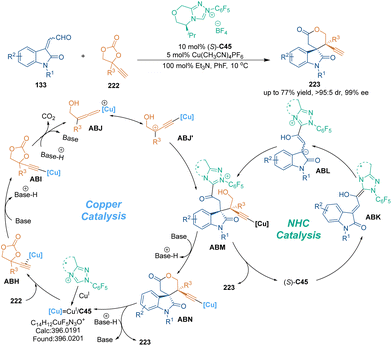 |
| Scheme 62 Copper and NHC co-catalyzed asymmetric [3 + 3] cycloaddition. | |
One year later, another asymmetric [3 + 3] cycloaddition between α,β-unsaturated 2,4,6-trichlorophenyl esters 225 and enamines 224 was realized by the same group under the cooperative catalysis of chiral isothiourea C46 and a Brønsted acid (Scheme 63),142 generating various 3,4-dihydropyridin-2-ones 226 with excellent enantioselectivities. A remarkable increase in the yield was observed when diphenylphosphinic acid was utilized as the cocatalyst. Mechanistically, unsaturated acyl ammoniums ABO would undergo anion exchange with a weaker base (Ph2PO2−), and the generated intermediates ABP could be attacked by enamines 224 to give 1,4-addition adducts ABQ. In this step, it was suggested that the H-bonding interaction between phosphate counterions and enamines might accelerate the Michael addition. Subsequent tautomerization and lactamization produced final dihydropyridinone products 226 and regenerated both catalysts.
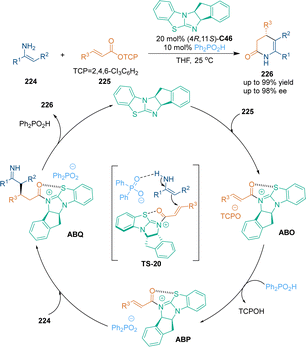 |
| Scheme 63 Chiral isothiourea and Brønsted acid co-catalyzed asymmetric [3 + 3] cycloaddition. | |
Another cooperative catalysis, merging organocatalysis and transition-metal catalysis, was developed by Deng's group.143 A combination of chiral N-heterocyclic carbene (NHC)/iridium catalysis was employed to catalyze the regiodivergent asymmetric [3 + 2] and [3 + 3] cycloadditions of 2-indolyl allyl carbonates 227 with enals 4 (Scheme 64). In the [3 + 3] cycloaddition, chiral NHC (S)-C47 attacked enals 4 to afford homoenolates ABS, followed by branch-selective allylation with allyliridium species. Both the Feringa ligand and NHC catalyst dominated the diastereo- and enantiocontrol in this step. Final lactamization of intermediates ABU generated pyridine[1,2-a]indoles 229 in a highly regioselective manner.
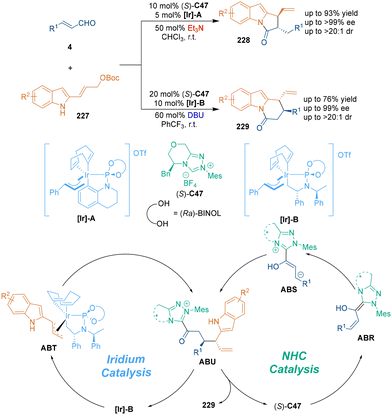 |
| Scheme 64 Iridium and chiral NHC co-catalyzed asymmetric [3 + 3] cycloaddition. | |
Recently, Gong's group designed a desymmetric [3 + 3] cycloaddition of oxabicyclic alkenes 230 with isatin-derived enals 133 under the cooperative catalysis of rhodium and chiral NHC catalyst (S)-C47 (Scheme 65), constructing chiral spirooxindole-based six-membered heterocycles 231 in high yields with excellent stereoselectivities.144 In the possible reaction pathway, desymmetric oxidative addition of the Rh catalyst to substrates 230 gave intermediates ABV. Meanwhile, chiral NHC (S)-C47 attacked enals 133 to form Breslow intermediates, which could tautomerize to intermediates ABX smoothly. Afterwards, sequential nucleophilic addition–lactonization between intermediates ABV and azolium homoenolate species yielded the final spirooxindole products 231. Moreover, the biological evaluation revealed that these chiral spirooxindoles could arrest mammalian cells in mitosis and displayed potent antiproliferative activities against HeLa cells, which demonstrated the promising applications of this class of chiral spirooxindoles in medicinal chemistry.
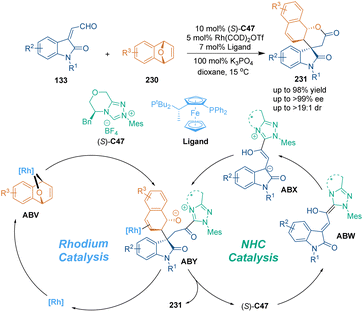 |
| Scheme 65 Rhodium and chiral NHC co-catalyzed asymmetric [3 + 3] cycloaddition. | |
7. Conclusions and outlook
Organocatalytic asymmetric [3 + 3] cycloadditions have flourished in the past twenty years, and a number of related research studies appeared during the preparation and publication of this manuscript.145 Various efficient methodologies and catalytic modes have been established to construct enantioenriched six-membered (hetero)cyclic frameworks, which constitute the core structures of many natural products and bioactive molecules. This review summarized different activation modes in diverse organocatalytic systems and elaborated strategies involving three-atom building blocks in organocatalytic asymmetric [3 + 3] cycloadditions, which aimed to shed some light on the future development of this field.
In spite of the rapid developments in this area, there are still some challenging issues to be solved in organocatalytic asymmetric [3 + 3] cycloadditions, mainly including: (i) most of the three-atom reaction partners in [3 + 3] cycloadditions are limited to building blocks containing C, N, O atoms. In contrast, three-atom building blocks containing B, Si, P, S atoms have scarcely been utilized in organocatalytic asymmetric [3 + 3] cycloadditions. Nevertheless, designing and developing three-atom building blocks containing B, Si, P, S atoms and their organocatalytic asymmetric [3 + 3] cycloadditions will provide more opportunities for constructing more intriguing heterocycles, particularly those bearing hetero-atom chirality. (ii) Visible-light photoredox catalysis and electrocatalysis, as clear and efficient catalytic systems, have rarely been combined with asymmetric organocatalysis to realize enantioselective [3 + 3] cycloadditions. Although challenges in controlling the enantioselectivity and finding compatible substrates have thwarted the application of these powerful tools, it is highly attractive to merge photoredox catalysis and electrocatalysis with organocatalysis in asymmetric [3 + 3] cycloadditions, thus realizing some unconventional catalytic asymmetric [3 + 3] cycloadditions, especially those involving radical species. (iii) Six-membered rings containing multiple stereocenters are enormously desired in the synthesis of natural products and bioactive molecules. However, mono-catalysis can hardly realize the stereodivergent construction of these molecules. In contrast, cooperative catalysis or relay catalysis may be suitable solutions to realize the stereodivergent synthesis of different stereoisomers of chiral six-membered heterocycles bearing multiple stereocenters. So, it is highly desired to develop stereodivergent asymmetric [3 + 3] cycloadditions under cooperative catalysis or relay catalysis of organocatalysts and metal catalysts. (iv) Six-membered rings are fundamental frameworks in natural products, drugs and advanced materials. Unfortunately, the applications of organocatalytic asymmetric [3 + 3] cycloadditions in the total synthesis of natural products or the synthesis of pharmaceuticals are rather rare and require exquisite design and great synthetic endeavors. Besides, the biological and pharmacological activities of the synthesized chiral six-membered heterocycles have not been investigated systematically; this requires joint efforts from synthetic chemists and medicinal chemists. We hope this review will inspire chemists to design more innovative strategies for solving the above-mentioned challenges in organocatalytic asymmetric [3 + 3] cycloadditions, and we believe this research field will develop to a higher level and find more and more important applications in the future.
Conflicts of interest
There are no conflicts to declare.
Acknowledgements
We are grateful for financial support from the National Natural Science Foundation of China (22125104) and the Natural Science Foundation of Jiangsu Province (BK20230624).
References
-
J. A. Joule and K. Mills, Heterocyclic Chemistry, John Wiley & Sons, Oxford, 5th edn, 2010, p. 689 Search PubMed.
-
D. Lednicer, Six-Membered Heterocycles, in The Organic Chemistry of Drug Synthesis, John Wiley & Sons, Hoboken, 2007, p. 115 Search PubMed.
- A. Moyano and R. Rios, Asymmetric Organocatalytic Cyclization and Cycloaddition Reactions, Chem. Rev., 2011, 111, 4703 CrossRef CAS.
-
J. J. Li, Heterocyclic Chemistry in Drug Discovery, John Wiley & Sons, Hoboken, 2013, pp. xxi–697 Search PubMed.
- Y. Yang and B. Yu, Recent Advances in the Chemical Synthesis of C-Glycosides, Chem. Rev., 2017, 117, 12281 CrossRef CAS PubMed.
-
N. Toyooka, Asymmetric Synthesis of Six-Membered Ring Heterocycles, in Asymmetric Synthesis of Nitrogen Heterocycles, ed. J. Royer, Wiley-VCH, Weinheim, 2009, p. 95 Search PubMed.
- M. G. Núñez, P. García, R. F. Moro and D. Díez, Asymmetric organocatalytic synthesis of six-membered oxygenated heterocycles, Tetrahedron, 2010, 66, 2089 CrossRef.
-
(a) N. Brodt and J. Niemeyer, Chiral organophosphates as ligands in asymmetric metal catalysis, Org. Chem. Front., 2023, 10, 3109 RSC;
(b) D.-F. Chen and L.-Z. Gong, Feng chiral N,N′-dioxide ligands: uniqueness and impacts, Org. Chem. Front., 2023, 10, 3676 RSC.
-
(a) J.-L. Li, T.-Y. Liu and Y.-C. Chen, Aminocatalytic Asymmetric Diels-Alder Reactions via HOMO Activation, Chem. Rev., 2013, 113, 5515 CrossRef;
(b) G. Masson, C. Lalli, M. Benohoud and G. Dagouseset, Catalytic enantioselective [4+2]-cycloaddition: a strategy to access aza-hexacycles, Chem. Soc. Rev., 2013, 42, 902 RSC.
- X. Jiang and R. Wang, Recent Developments in Catalytic Asymmetric Inverse-Electron-Demand Diels-Alder Reaction, Chem. Rev., 2013, 113, 5515 CrossRef CAS PubMed.
- J. P. A. Harrity and O. Provoost, [3+3] Cycloadditions and Related Strategies in Alkaloid Natural Product Synthesis, Org. Biomol. Chem., 2005, 3, 1349 RSC.
- Y. Deng, Q.-Q. Cheng and M. Doyle, Asymmetric [3+3] Cycloaddition for Heterocycle Synthesis, Synlett, 2017, 1695 CAS.
-
(a) Y. Zhu and Y. Huang, Organocatalyzed [3+3] Annulations for the Construction of Heterocycles, Synthesis, 2020, 1181 CAS;
(b) S.-S. Dai, X.-J. Yang, R. Fang, A. M. Kirillov and L. Yang, A DFT study of Ni-catalyzed (3 + 3)-annulation between donor–acceptor cyclopropanes and diaziridines, Org. Chem. Front., 2023, 10, 1948 RSC.
- Y.-B. Wang and B. Tan, Construction of Axially Chiral Compounds via Asymmetric Organocatalysis, Acc. Chem. Res., 2018, 51, 534 CrossRef CAS PubMed.
- Y.-C. Zhang, F. Jiang and F. Shi, Organocatalytic Asymmetric Synthesis of Indole-Based Chiral Heterocycles: Strategies, Reactions, and Outreach, Acc. Chem. Res., 2020, 53, 425 CrossRef CAS PubMed.
- B. Han, X.-H. He, Y.-Q. Liu, G. He, C. Peng and J.-L. Li, Asymmetric Organocatalysis: An Enabling Technology for Medicinal Chemistry, Chem. Soc. Rev., 2021, 50, 1522 RSC.
- N. M. Rezayee, J. N. Lamhauge and K. A. Jørgensen, Organocatalyzed Cross-Nucleophile Couplings: Umpolung of Catalytic Enamines, Acc. Chem. Res., 2022, 55, 1703 CrossRef CAS.
- H.-H. Zhang and F. Shi, Organocatalytic Atroposelective Synthesis of Indole Derivatives Bearing Axial Chirality: Strategies and Applications, Acc. Chem. Res., 2022, 55, 2562 CrossRef CAS PubMed.
- J. K. Cheng, S.-H. Xiang and B. Tan, Organocatalytic Enantioselective Synthesis of Axially Chiral Molecules: Development of Strategies and Skeletons, Acc. Chem. Res., 2022, 55, 2920 CrossRef CAS.
- A. I. Gerasyuto, R. P. Hsung, N. Sydorenko and B. A. Slafer, Formal [3+3] Cycloaddition Reaction. 5. An Enantioselective Intramolecular Formal Aza-[3+3] Cycloaddition Reaction Promoted by Chiral Amine Salts, J. Org. Chem., 2005, 70, 4248 CrossRef CAS.
- Y. Hayashi, H. Gotoh, R. Masui and H. Ishikawa, Diphenylprolinol Silyl Ether as a Catalyst in an Enantioselective, Catalytic, Formal Aza [3+3] Cycloaddition Reaction for the Formation of Enantioenriched Piperidines, Angew. Chem., Int. Ed., 2008, 47, 4012 CrossRef CAS PubMed.
- L. Zu, H. Xie, H. Li, J. Wang, X. Yu and W. Wang, Chiral Amine–Catalyzed Enantioselective Cascade Aza–Ene–Type Cyclization Reactions, Chem. – Eur. J., 2008, 14, 6333 CrossRef CAS PubMed.
- G. S. Buchanan, H. Dai, R. P. Hsung, A. I. Gerasyuto and C. M. Scheinebeck, Asymmetric Aza-[3+3] Annulation in the Synthesis of Indolizidines: An Unexpected Reversal of Regiochemistry, Org. Lett., 2011, 13, 4402 CrossRef CAS.
- J.-P. Wan, C. C. J. Loh, F. Pan and D. Enders, Enantioselective Organocatalytic Domino Synthesis of Tetrahydropyridin-2-Ols, Chem. Commun., 2012, 48, 10049 RSC.
- A. Noole, M. Borissova, M. Lopp and T. Kanger, Enantioselective Organocatalytic Aza-Ene-Type Domino Reaction Leading to 1,4-Dihydropyridines, J. Org. Chem., 2011, 76, 1538 CrossRef CAS PubMed.
- X. Ding, R. Su, W. Yang and W. Deng, Organocatalytic Asymmetric Formal Aza-[3+3] Cyclo-additions of 3-Aminobenzofuran with α,β-Unsaturated Aldehydes, Adv. Synth. Catal., 2018, 360, 4168 CrossRef CAS.
- X. Xiong, H. Zhang, J. Peng and Y. Chen, Direct Asymmetric Michael Addition of Cyclic N-Sulfonylimines to α,β-Unsaturated Aldehydes., Chem. – Eur. J., 2011, 17, 2358 CrossRef CAS PubMed.
- Q. An, J. Li, J. Shen, N. Butt, D. Liu, Y. Liu and W. Zhang, Asymmetric Tandem Reactions of N-Sulfonylimines and α,β-Unsaturated Aldehydes: An Alternative Reaction Pathway to That of Using Saturated Aldehydes, Chem. Commun., 2015, 51, 885 RSC.
- R.-H. Su, X.-F. Ding, S.-X. Wu, J.-H. Zhao and W.-P. Deng, Secondary Amine-Catalyzed Asymmetric Formal Aza [3+3] Cycloaddition to Construct Enantioenriched Piperidines Derivatives, Tetrahedron, 2017, 73, 6031 CrossRef CAS.
- B.-C. Hong, M.-F. Wu, H.-C. Tseng and J.-H. Liao, Enantioselective Organocatalytic Formal [3+3]-Cycloaddition of α,β-Unsaturated Aldehydes and Application to the Asymmetric Synthesis of (−)-Isopulegol Hydrate and (−)-Cubebaol, Org. Lett., 2006, 8, 2217 CrossRef CAS PubMed.
- C.-L. Cao, X.-L. Sun, Y.-B. Kang and Y. Tang, Enantioselective Formal [3+3] Annulation for the Direct Construction of Bicyclic Skeletons with Four Stereogenic Centers, Org. Lett., 2007, 9, 4151 CrossRef CAS.
- Y. Hayashi, M. Toyoshima, H. Gotoh and H. Ishikawa, Diphenylprolinol Silyl Ether Catalysis in an Asymmetric Formal Carbo [3+3] Cycloaddition Reaction via a Domino Michael/Knoevenagel Condensation, Org. Lett., 2009, 11, 45 CrossRef CAS.
- F. Cagide-Fagín, O. Nieto-García, H. Lago-Santomé and R. Alonso, Enantioselective Synthesis of Protected Nitrocyclohexitols with Five Stereocenters. Total Synthesis of (+)-Pancratistatin, J. Org. Chem., 2012, 77, 11377 CrossRef.
- S. Wang, Y. Zhang, G. Dong, S. Wu, S. Zhu, Z. Miao, J. Yao, H. Li, J. Li, W. Zhang, C. Sheng and W. Wang, Asymmetric Synthesis of Chiral Dihydrothiopyrans via an Organocatalytic Enantioselective Formal Thio [3+3] Cycloaddition Reaction with Binucleophilic Bisketone Thioethers, Org. Lett., 2013, 15, 5570 CrossRef CAS PubMed.
- Y. Xu, S. Zhang, L. Li, Y. Wang, Z. Zha and Z. Wang,
L -Phenylalanine Potassium Catalyzed Asymmetric Formal [3+3] Annulation of 2-Enoyl-Pyridine N -Oxides with Acetone, Org. Chem. Front., 2018, 5, 376 RSC.
-
(a) A. Zea, A.-N. R. Alba, A. Mazzanti, A. Moyano and R. Rios, Highly Enantioselective Cascade Synthesis of Spiropyrazolones, Org. Biomol. Chem., 2011, 9, 6519 RSC;
(b) J. Zhang, N. Li, Z. Liu, X. Huang, Z. Geng and X. Wang, Enantioselective Synthesis of Unsymmetrical Diaryl–Substituted Spirocyclohexanonepyrazolones through a Cascade [4+2] Double Michael Addition, Adv. Synth. Catal., 2013, 355, 797 CrossRef CAS;
(c) B. Han, W. Huang, W. Ren, G. He, J. Wang and C. Peng, Asymmetric Synthesis of Cyclohexane–Fused Drug–Like Spirocyclic Scaffolds Containing Six Contiguous Stereogenic Centers via Organocatalytic Cascade Reactions, Adv. Synth. Catal., 2015, 357, 561 CrossRef CAS.
-
(a) H. Leng, Q. Li, R. Zeng, Q. Dai, H. Zhu, Y. Liu, W. Huang, B. Han and J. Li, Asymmetric Construction of Spiropyrazolone Skeletons via Amine–Catalyzed [3+3] Annulation, Adv. Synth. Catal., 2018, 360, 229 CrossRef CAS;
(b) S. Cabrera, J. Aleman, P. Bolze, S. Bertelsen and K. A. Jørgensen, An Unexpected Organocatalytic Asymmetric Tandem Michael/Morita–Baylis–Hillman Reaction., Angew. Chem., Int. Ed., 2008, 47, 121 CrossRef CAS PubMed.
- M. Rueping, E. Sugiono and E. Merino, Asymmetric Iminium Ion Catalysis: An Efficient Enantioselective Synthesis of Pyranonaphthoquinones and β–Lapachones, Angew. Chem., Int. Ed., 2008, 47, 3046 CrossRef CAS.
- M.-K. Zhu, Q. Wei and L.-Z. Gong, Organocatalytic Asymmetric Formal [3+3] Cycloaddition Reactions of α,β-Unsaturated Aldehydes with Nazarov Reagents, Adv. Synth. Catal., 2008, 350, 1281 CrossRef CAS.
- P. T. Franke, B. Richter and K. A. Jørgensen, Organocatalytic Asymmetric Synthesis of Functionalized 3,4-Dihydropyran Derivatives, Chem. – Eur. J., 2008, 14, 6317 CrossRef CAS PubMed.
- M. Rueping, E. Sugiono and E. Merino, Asymmetric Organocatalysis: An Efficient Enantioselective Access to Benzopyranes and Chromenes, Chem. – Eur. J., 2008, 14, 6329 CrossRef CAS PubMed.
- J.-H. Chen, C. Chang, H.-J. Chang and K. Chen, Synthesis of 2,3,5,6-Tetrahydro-1-Alkyl/Aryl-1H-Benzo[f]Chromen-3-Ol Derivatives from β-Tetralones and α,β-Unsaturated Aldehydes, Org. Biomol. Chem., 2011, 9, 7510 RSC.
- L. Hong, L. Wang, W. Sun, K. Wong and R. Wang, Organocatalytic Asymmetric Friedel−Crafts Alkylation/Cyclization Cascade Reaction of 1-Naphthols and α,β-Unsaturated Aldehydes: An Enantioselective Synthesis of Chromanes and Dihydrobenzopyranes, J. Org. Chem., 2009, 74, 6881 CrossRef CAS PubMed.
- J. Franzén and A. Fisher, Asymmetric Alkaloid Synthesis: A One–Pot Organocatalytic Reaction to Quinolizidine Derivatives, Angew. Chem., Int. Ed., 2009, 48, 787 CrossRef.
- W. Zhang and J. Franzén, Diverse Asymmetric Quinolizidine Synthesis: A Stereodivergent One–Pot Approach, Adv. Synth. Catal., 2010, 352, 499 CrossRef CAS.
- S. Shiomi, E. Sugahara and H. Ishikawa, Efficient Organocatalytic Construction of C4−Alkyl Substituted Piperidines and Their Application to the Synthesis of (+)-α-Skytanthine, Chem. – Eur. J., 2015, 21, 14758 CrossRef CAS PubMed.
- F. Zhang, M. Wei, J. Dong, Y. Zhou, D. Lu, Y. Gong and X. Yang, A Recyclable Organocascade Reaction System: Stereoselective Precipitation of Optically Active Cis-δ-Lactols with Quaternary Stereocenters during the Michael–Hemiacetalization Reaction, Adv. Synth. Catal., 2010, 352, 2875 CrossRef CAS.
- E. Rodrigo, J. L. G. Ruano and M. B. Cid, Organocatalytic Michael Addition/Intramolecular Julia−Kocienski Olefination for the Preparation of Nitrocyclohexenes, J. Org. Chem., 2013, 78, 10737 CrossRef CAS.
-
(a) S.-K. Tian, Y. Chen, J. Hang, L. Tang, P. McDaid and L. Deng, Asymmetric Organic Catalysis with Modified Cinchona Alkaloids, Acc. Chem. Res., 2004, 37, 621 CrossRef CAS;
(b) L. Jiang and Y.-C. Chen, Recent Advances in Asymmetric Catalysis with Cinchona Alkaloid-Based Primary Amines, Catal. Sci. Technol., 2011, 1, 354 RSC;
(c) L. Stegbauer, F. Sladojevich and D. J. Dixon, Bifunctional Organo/Metal Cooperative Catalysis with Cinchona Alkaloid Scaffolds, Chem. Sci., 2012, 3, 942 RSC.
- C. Cao, Y. Zhou, J. Zhou, X. Sun, Y. Tang, Y. Li, G. Li and J. Sun, An Organocatalytic Asymmetric Tandem Reaction for the Construction of Bicyclic Skeletons, Chem. – Eur. J., 2009, 15, 11384 CrossRef CAS.
- Y. Zheng, L. Cui, Y. Wang and Z. Zhou, Stereocontrolled Construction of Tetrahydropyrano[2,3-c]pyrazole Scaffold via an Organocatalyzed Formal [3+3] Annulation, J. Org. Chem., 2016, 81, 4340 CrossRef CAS.
- Y. Xiao, J.-B. Lin, Y.-N. Zhao, J.-Y. Liu and P.-F. Xu, Exploring the Reactivity of Nitro-Activated 1,3-Enynes in an Organocatalytic One-Pot, Three-Component Coupling Reaction: A Tandem Catalytic Approach to a Novel 3-Nitrochroman Scaffold, Org. Lett., 2016, 18, 6276 CrossRef CAS.
- J.-Y. Liu, J. Zhao, J.-L. Zhang and P.-F. Xu, Quaternary Carbon Center Forming Formal [3+3] Cycloaddition Reaction via Bifunctional Catalysis: Asymmetric Synthesis of Spirocyclohexene Pyrazolones, Org. Lett., 2017, 19, 1846 CrossRef CAS.
- R. Gurubrahamam, B.-F. Gao, Y. M. Chen, Y.-T. Chan, M.-K. Tsai and K. Chen, Organocascade Synthesis of Annulated (Z,)-2-Methylenepyrans: Nucleophilic Conjugate Addition of Hydroxycoumarins and Pyranone to Branched Nitro Enynes via Allene Formation/Oxa-Michael Cyclization/Alkene Isomerization Sequence, Org. Lett., 2016, 18, 3098 CrossRef CAS PubMed.
- Z. Xing, L. Song, F. Li, N. Xu, L. Wang, Z. Shi and X. Cao, Organocatalytic Enantioselective Tandem Michael Addition and Cyclization Reaction of Trisubstituted 2-Nitro-1,3-enynes with Cyclic 1,3−Diketones, Adv. Synth. Catal., 2017, 359, 1892 CrossRef CAS.
- N. K. Rana, R. K. Jha, H. Joshi and V. K. Singh, Enantioselective Access to Tetrahydropyrano[2,3-c]Pyrazoles via an Organocatalytic Domino Michael-Hydroalkoxylation Reaction, Tetrahedron Lett., 2017, 58, 2135 CrossRef CAS.
- Q. Ni, X. Wang, D. Zeng, Q. Wu and X. Song, Organocatalytic Asymmetric Synthesis of Aza-Spirooxindoles via Michael/Friedel-Crafts Cascade Reaction of 1,3-Nitroenynes and 3-Pyrrolyloxindoles, Org. Lett., 2021, 23, 2273 CrossRef CAS.
- J. Huang, L. Zhao, Y. Liu, W. Cao and X. Wu, Enantioselective Intermolecular Formal [3+3] Cycloaddition of 2,3-Disubstituted Indoles with Acrolein, Org. Lett., 2013, 15, 4338 CrossRef CAS.
- S. Goudedranche, X. Bugaut, T. Constantieux, D. Bonne and J. Rodriguez, α,β–Unsaturated Acyl Cyanides as New Bis–Electrophiles for Enantioselective Organocatalyzed Formal [3+3]Spiroannulation, Chem. – Eur. J., 2014, 20, 410 CrossRef CAS PubMed.
- S.-J. Yin, S.-Y. Zhang, J.-Q. Zhang, B.-B. Sun, W.-T. Fan, B. Wu and X.-W. Wang, Organocatalytic Tandem Enantioselective Michael-Cyclization of Isatin-Derived β,γ-Unsaturated α-Ketoesters with 3-Hydroxy-4H-Chromen-4-One or 2-Hydroxy-1,4-Naphthoquinone Derivatives, RSC Adv., 2016, 6, 84248 RSC.
- K.-X. Huang, M.-S. Xie, Q.-Y. Zhang, H.-Y. Niu, G.-R. Qu and H.-M. Guo, Synthesis of Chiral Six-Membered Carbocyclic Purine Nucleosides via Organocatalytic Enantioselective [3+3] Annulation, Org. Lett., 2018, 20, 5398 CrossRef CAS PubMed.
- B.-B. Sun, J.-B. Chen, J.-Q. Zhang, X.-P. Yang, H.-P. Lv, Z. Wang and X.-W. Wang, Organo-Catalyzed Asymmetric Cascade Annulation Reaction for the Construction of Bi-Spirocyclic Pyrazolone and Oxindole Derivatives, Org. Chem. Front., 2020, 7, 796 RSC.
- D.-S. Ji, Y.-C. Luo, X.-Q. Hu and P.-F. Xu, Enantioselective Synthesis of Spirorhodanine-Pyran Derivatives via Organocatalytic [3+3] Annulation Reactions between Pyrazolones and Rhodanine-Derived Ketoesters, Org. Lett., 2020, 22, 1028 CrossRef CAS.
-
(a) L. Chen, X. Zhang, K.-J. Shi, H.-J. Leng, Q.-Z. Li, Y. Liu, J.-H. Li, Q.-W. Wang and J.-L. Li, Bifunctional Brønsted Base Catalyzed [3+3] Annulations of Indolin-2-imines and α,β-Unsaturated Imides: An Enantioselective
Approach to α-Carbolinones, J. Org. Chem., 2020, 85, 9454 CrossRef CAS PubMed;
(b) Z. Li, H. Zhou and J. Xu, Access to Chiral Polycyclic 1,4-Dihydropyridines via Organocatalytic Formal [3+3] Annulation of 2-(1-Alkynyl)-2-alken-1-ones with 3-Aminobenzofurans, Org. Lett., 2021, 23, 6391 CrossRef CAS PubMed.
- W.-B. Chen, Z.-J. Wu, Q.-L. Pei, L.-F. Cun, X.-M. Zhang and W.-C. Yuan, Highly Enantioselective Construction of Spiro[4H -Pyran-3,3′-Oxindoles] Through a Domino Knoevenagel/Michael/Cyclization Sequence Catalyzed by Cupreine, Org. Lett., 2010, 12, 3132 CrossRef CAS PubMed.
- Q.-N. Zhu, Y.-C. Zhang, M.-M. Xu, X.-X. Sun, X. Yang and F. Shi, Enantioselective Construction of Tetrahydroquinolin-5-one-Based Spirooxindole Scaffold via an Organocatalytic Asymmetric Multicomponent [3+3] Cyclization, J. Org. Chem., 2016, 81, 7898 CrossRef CAS PubMed.
- X. Chen, Z.-H. Qi, S.-Y. Zhang, L.-P. Kong, Y. Wang and X.-W. Wang, Enantioselective Construction of Functionalized Thiopyrano-Indole Annulated Heterocycles via a Formal Thio [3+3]-Cyclization, Org. Lett., 2015, 17, 42 CrossRef CAS PubMed.
- B.-B. Sun, J.-Q. Zhang, J.-B. Chen, W.-T. Fan, J.-Q. Yu, J.-M. Hu and X.-W. Wang, Stereoselective Synthesis of Spirocyclohexadiene-Pyrazolones via Organic Base and/or Hydrogen Bonding Assisted [3+3] Annulation Reactions, Org. Chem. Front., 2019, 6, 1842 RSC.
- X.-L. He, Y.-C. Xiao, W. Du and Y.-C. Chen, Enantioselective Formal [3+3] Cycloadditions of Ketones and Cyclic 1−Azadienes by Cascade Enamine–Enamine Catalysis, Chem. – Eur. J., 2015, 21, 3443 CrossRef CAS.
- X. Chen, J.-Q. Zhang, S.-J. Yin, H.-Y. Li, W.-Q. Zhou and X.-W. Wang, Asymmetric Construction of Spiro[thiopyranoindole-benzoisothiazole] Scaffold via a Formal [3+3] Spiroannulation, Org. Lett., 2015, 17, 4188 CrossRef CAS PubMed.
- X. Yang, Y.-C. Zhang, Q.-N. Zhu, M.-S. Tu and F. Shi, Diastereo- and Enantioselective Construction of the Hexahydrocoumarin Scaffold via an Organocatalytic Asymmetric [3+3] Cyclization, J. Org. Chem., 2016, 81, 5056 CrossRef CAS PubMed.
- L.-L. Wu, Y. Zheng, Y.-M. Wang and Z.-H. Zhou, Organocatalyzed Enantioselective [3+3] Annulation for the Direct Synthesis of Conformationally Constrained Cyclic Tryptophan Derivatives, RSC Adv., 2016, 6, 11602 RSC.
-
(a) S. Ma, Transition Metal-Catalyzed/Mediated Reaction of Allenes with a Nucleophilic Functionality Connected to the α-Carbon Atom, Acc. Chem. Res., 2003, 36, 701 CrossRef CAS;
(b) S. Ma, Some Typical Advances in the Synthetic Applications of Allenes, Chem. Rev., 2005, 105, 2829 CrossRef PubMed;
(c) J. M. Alonso and P. Almendros, Deciphering the Chameleonic Chemistry of Allenols: Breaking the Taboo of a Onetime Esoteric Functionality, Chem. Rev., 2021, 121, 4193 CrossRef CAS PubMed.
- C. Ni and X. Tong, Amine-Catalyzed Asymmetric [3+3] Annulations of β′-Acetoxy Allenoates: Enantioselective Synthesis of 4H-Pyrans, J. Am. Chem. Soc., 2016, 138, 7872 CrossRef CAS.
- W. Zhou, C. Ni, J. Chen, D. Wang and X. Tong, Enantioselective Synthesis of 4H-Pyran via Amine-Catalyzed Formal [3+3] Annulation of δ-Acetoxy Allenoate, Org. Lett., 2017, 19, 1890 CrossRef CAS.
- W. Yang, W. Sun, C. Zhang, Q. Wang, Z. Guo, B. Mao, J. Liao and H. Guo, Lewis-Base-Catalyzed Asymmetric [3+3] Annulation Reaction of Morita−Baylis−Hillman Carbonates: Enantioselective Synthesis of Spirocyclohexenes, ACS Catal., 2017, 7, 3142 CrossRef CAS.
- Z. Yue, W. Li, L. Liu, C. Wang and J. Zhang, Enantioselective Synthesis of 4H-Pyrans Through Organocatalytic Asymmetric Formal [3+3] Cycloadditions of 2-(1-Alkynyl)-2-alken-1-ones with β-Keto Esters, Adv. Synth. Catal., 2016, 358, 3015 CrossRef CAS.
- Y. Zhu, Z. Wang, J. Zhang, J. Yu, L. Yan, Y. Li, L. Chen and X. Yan, An Organocatalytic Synthesis of Chiral Pyrano[2,3-d]Pyrimidines
through [3+3] Annulation of 1,3-Dimethyl-barbituric Acid with 2-(1-Alkynyl)-2-alken-1-ones, Eur. J. Org. Chem., 2018, 347 CrossRef CAS.
-
(a) D. Enders and T. Balensiefer, Nucleophilic Carbenes in Asymmetric Organocatalysis, Acc. Chem. Res., 2004, 37, 534 CrossRef CAS;
(b) X. Bugaut and F. Glorius, Organocatalytic Umpolung: N-Heterocyclic Carbenes and Beyond, Chem. Soc. Rev., 2012, 41, 3511 RSC;
(c) D. M. Flanigan, F. Romanov-Michailidis, N. A. White and T. Rovis, Organocatalytic Reactions Enabled by N-Heterocyclic Carbenes, Chem. Rev., 2015, 115, 9307 CrossRef CAS PubMed.
- J. Kaeobamrung, J. Mahatthananchai, P. Zheng and J. W. Bode, An Enantioselective Claisen Rearrangement Catalyzed by N-Heterocyclic Carbenes, J. Am. Chem. Soc., 2010, 132, 8810 CrossRef CAS.
- Z.-Q. Zhu, X.-L. Zheng, N.-F. Jiang, X. Wan and J.-C. Xiao, Chiral N-Heterocyclic Carbene Catalyzed Annulation of α,β-Unsaturated Aldehydes with 1,3-Dicarbonyls, Chem. Commun., 2011, 47, 8670 RSC.
- Z.-Q. Rong, M.-Q. Jia and S.-L. You, Enantioselective N-Heterocyclic Carbene-Catalyzed Michael Addition to α,β-Unsaturated Aldehydes by Redox Oxidation, Org. Lett., 2011, 13, 4080 CrossRef CAS PubMed.
- S. R. Yetra, S. Mondal, E. Suresh and A. T. Biju, Enantioselective Synthesis of Functionalized Pyrazoles by NHC-Catalyzed Reaction of Pyrazolones with α,β-Unsaturated Aldehydes, Org. Lett., 2015, 17, 1417 CrossRef CAS.
- Y. Que, Y. Lu, W. Wang, Y. Wang, H. Wang, C. Yu, T. Li, X. Wang, S. Shen and C. Yao, An Enantioselective Assembly of Dihydropyranones through an NHC/LiCl–Mediated in Situ Activation of α,β–Unsaturated Carboxylic Acids, Chem. – Asian J., 2016, 11, 678 CrossRef CAS PubMed.
- D. Xie, D. Shen, Q. Chen, J. Zhou, X. Zeng and G. Zhong, N-Heterocyclic Carbene/Lewis Acid Catalyzed Enantioselective Aerobic Annulation of α,β-Unsaturated Aldehydes with 1,3- Dicarbonyl Compounds, J. Org. Chem., 2016, 81, 6136 CrossRef CAS PubMed.
- A. Axelsson, E. Hammarvid, L. Ta and H. Sundén, Asymmetric Aerobic Oxidative NHC-Catalysed Synthesis of Dihydropyranones Utilising a System of Electron Transfer Mediators, Chem. Commun., 2016, 52, 11571 RSC.
- A. G. Kravina, J. Mahatthananchai and J. W. Bode, Enantioselective, NHC–Catalyzed Annulations of Trisubstituted Enals and Cyclic N–Sulfonylimines via α,β–Unsaturated Acyl Azoliums, Angew. Chem., Int. Ed., 2012, 51, 9433 CrossRef CAS.
- Q. Liu, X.-Y. Chen, S. Li, K. Rissanen and D. Enders, N–Heterocyclic Carbene Catalyzed Asymmetric Synthesis of Pentacyclic Spirooxindoles via [3+3] Annulations of Isatin–Derived Enals and Cyclic N–Sulfonyl Ketimines, Adv. Synth. Catal., 2019, 361, 1991 CrossRef CAS.
- J. Cheng, Z. Huang and Y. R. Chi, NHC Organocatalytic Formal LUMO Activation of α,β–Unsaturated Esters for Reaction with Enamides, Angew. Chem., Int. Ed., 2013, 52, 8592 CrossRef CAS PubMed.
- D. Xie, L. Yang, Y. Lin, Z. Zhang, D. Chen, X. Zeng and G. Zhong, Rapid Access to Spirocylic Oxindoles: Application of Asymmetric N-Heterocyclic Carbene-Catalyzed [3+3] Cycloaddition of Imines to Oxindole-Derived Enals, Org. Lett., 2015, 17, 2318 CrossRef CAS PubMed.
- Z. Zhang, X. Zeng, D. Xie, D. Chen, L. Ding, A. Wang, L. Yang and G. Zhong, N-Heterocyclic Carbene-Catalyzed Activation of Esters of N-Hydroxyphthalimide: A Highly Enantioselective Route to Chiral Dihydropyridinones Bearing an All Carbon Quaternary Stereogenic Center, Org. Lett., 2015, 17, 5052 CrossRef CAS PubMed.
- J.-H. Mao, Z.-T. Wang, Z.-Y. Wang and Y. Cheng, N-Heterocyclic Carbene-Catalyzed Oxidative Annulations of α,β-Unsaturated Aldehydes with Hydrazones: Selective Synthesis of Optically Active 4,5-Dihydropyridazin-3-ones and Pyridazin-3-ones, J. Org. Chem., 2015, 80, 6350 CrossRef CAS.
- W. Xia, H. Yao, D. Liu, L. Zhao, Y. Zhou and H. Liu, Enantioselective N–Heterocyclic Carbene–Catalyzed [3+3] Annulation of α,β–Unsaturated Esters with Methyl Ketoimine, Adv. Synth. Catal., 2016, 358, 1864 CrossRef CAS.
- C. He, Y. Zhou, Z. Li, J. Xu and X. Chen, N-Heterocyclic Carbene Catalyzed Asymmetric [3+3] Cycloaddtion of β,β-Disubstituted, α,β-Unsaturated Carboxylic Esters with 3-Aminobenzofurans, Org. Chem. Front., 2021, 8, 1569 RSC.
- M. Dong, X.-Y. Duan, Y. Li, B. Liu and J. Qi, Highly Enantioselective δ-Protonation and Formal [3+3] Annulation Promoted by N-Heterocyclic Carbene, Org. Chem. Front., 2022, 9, 3039 RSC.
- A. Porey, S. Santra and J. Guin, Highly Enantioselective Synthesis of Functionalized Glutarimide Using Oxidative N-Heterocyclic Carbene Catalysis: A Formal Synthesis of (−)-Paroxetine, J. Org. Chem., 2019, 84, 5313 CrossRef CAS PubMed.
- S. R. Yetra, S. Mondal, S. Mukherjee, R. G. Gonnade and A. T. Biju, Enantioselective Synthesis of Spirocyclohexadienones by NHC–Catalyzed Formal [3+3] Annulation Reaction of Enals, Angew. Chem., Int. Ed., 2016, 55, 268 CrossRef CAS PubMed.
- For books:
(a)
C. Mazet, in Privileged Chiral Ligands and Catalysts, ed. Q.-L. Zhou, Wiley-VCH, Weinheim, 2012, vol. 51, p. 305 Search PubMed;
(b)
B. Tan, Axially Chiral Compounds: Asymmetric Synthesis and Applications, VCH, Weinheim, 2021 CrossRef;
(c) For recent examples: F.-T. Sheng, S. Yang, S.-F. Wu, Y.-C. Zhang and F. Shi, Catalytic Asymmetric Synthesis of Axially Chiral 3,3′-Bisindoles by Direct Coupling of Indole Rings, Chin. J. Chem., 2022, 40, 2151 CrossRef CAS;
(d) S.-J. Liu, Z.-H. Chen, J.-Y. Chen, S.-F. Ni, Y.-C. Zhang and F. Shi, Rational Design of Axially Chiral Styrene-Based Organocatalysts and Their Application in Catalytic Asymmetric [2+4] Cyclizations, Angew. Chem., Int. Ed., 2022, 61, e202112226 CrossRef CAS PubMed;
(e) Q.-Q. Hang, S.-F. Wu, S. Yang, X. Wang, Z. Zhong, Y.-C. Zhang and F. Shi, Design and catalytic atroposelective synthesis of axially chiral isochromenone-indoles, Sci. China: Chem., 2022, 65, 1929 CrossRef CAS;
(f) P. Wu, L. Yu, C.-H. Gao, Q. Cheng, S. Deng, Y. Jiao, W. Tan and F. Shi, Design and synthesis of axially chiral aryl-pyrroloindoles via the strategy of organocatalytic asymmetric (2+3) cyclization, Fundam. Res., 2023, 3, 237 CrossRef CAS PubMed;
(g) Z.-H. Chen, T.-Z. Li, N.-Y. Wang, X.-F. Ma, S.-F. Ni, Y.-C. Zhang and F. Shi, Organocatalytic Enantioselective Synthesis of Axially Chiral N,N′-Bisindoles, Angew. Chem., Int. Ed., 2023, 62, e202300419 CrossRef CAS.
-
(a) C. Zhao, D. Guo, K. Munkerup, K.-W. Huang, F. Li and J. Wang, Enantioselective [3+3] Atroposelective Annulation Catalyzed by N-Heterocyclic Carbenes, Nat. Commun., 2018, 9, 611 CrossRef PubMed;
(b) R. Ma, X. Wang, Q. Zhang, L. Chen, J. Gao, J. Feng, D. Wei and D. Du, Atroposelective Synthesis of Axially Chiral 4−Aryl α–Carbolines via N-Heterocyclic Carbene Catalysis, Org. Lett., 2021, 23, 4267 CrossRef CAS.
- F.-G. Sun, L.-H. Sun and S. Ye, N–Heterocyclic Carbene–Catalyzed Enantioselective Annulation of Bromoenal and 1,3−Dicarbonyl Compounds, Adv. Synth. Catal., 2011, 353, 3134 CrossRef CAS.
- S. R. Yetra, A. Bhunia, A. Patra, M. V. Mane, K. Vanka and A. T. Biju, Enantioselective N–Heterocyclic Carbene–Catalyzed Annulations of 2−Bromoenals with 1,3−Dicarbonyl Compounds and Enamines via Chiral α,β–Unsaturated Acylazoliums, Adv. Synth. Catal., 2013, 355, 1089 CrossRef CAS.
- G. He, X. Chen, S. Xia, G. Zhong and L. Yang, Enantioselective Synthesis of 6-(Indole-2-yl)-3,4-Dihydropyran-2-One
Skeletons by N-Heterocyclic Carbene-Catalyzed Asymmetric [3+3] Cycloaddition of α-Bromocinnamaldehyde, Chem. Synth., 2023, 3, 35 CAS.
- S. R. Yetra, T. Kaicharla, S. S. Kunte, R. G. Gonnade and A. T. Biju, Asymmetric N-Heterocyclic Carbene (NHC)-Catalyzed Annulation of Modified Enals with Enolizable Aldehydes, Org. Lett., 2013, 15, 5202 CrossRef CAS.
- Q. Ni, X. Song, G. Raabe and D. Enders, N–Heterocyclic Carbene–Catalyzed Enantioselective Annulation of Indolin–3−ones with Bromoenals, Chem. – Asian J., 2014, 9, 1535 CrossRef CAS PubMed.
- S. Li, Y. Yao, Z. Tang, B. Sun, C. Yu, T. Li and C. Yao, An Enantioselective Assembly of Naphthopyran via NHC-Catalyzed [3+3] Annulation of Bromoenal with β-Tetralone., Org. Biomol. Chem., 2019, 17, 268 RSC.
- H.-M. Zhang, W.-Q. Jia, Z.-Q. Liang and S. Ye, N–Heterocyclic Carbene–Catalyzed [3+3] Cyclocondensation of Bromoenals and Ketimines: Highly Enantioselective Synthesis of Dihydropyridinones, Asian J. Org. Chem., 2014, 3, 462 CrossRef CAS.
- Z.-H. Gao, X.-Y. Chen, H.-M. Zhang and S. Ye, N-Heterocyclic Carbene-Catalyzed [3+3] Cyclocondensation of Bromoenals with Aldimines: Highly Enantioselective Synthesis of Dihydropyridinones, Chem. Commun., 2015, 51, 12040 RSC.
- Q. Ni, J. Xiong, X. Song, G. Raabe and D. Enders, N-Heterocyclic Carbene Catalyzed Enantioselective Annulation of Benzothiazolyl Ethyl Acetates with 2-Bromoenals, Synlett, 2015, 1465 CAS.
- C.-L. Zhang, D.-L. Wang, K.-Q. Chen and S. Ye, N-Heterocyclic Carbene-Catalyzed [3+3] Cyclocondensation of Bromoenals with Hydrazones: Highly Enantioselective Synthesis of Dihydropyridazones, Org. Biomol. Chem., 2015, 13, 11255 RSC.
- J. Yan, Z. Song, C. Zhao, K. Shi, L. Yang and G. Zhong, Highly Chemoselective and Enantioselective Synthesis of 3,4-2H-Pyrindin-2-Ones by an NHC-Catalyzed [3+3] Cyclization, Org. Lett., 2020, 22, 3329 CrossRef CAS.
- K.-Q. Chen, Z.-H. Gao and S. Ye, (Dynamic) Kinetic Resolution of Enamines/Imines: Enantioselective N–Heterocyclic Carbene Catalyzed [3+3] Annulation of Bromoenals and Enamines/Imines, Angew. Chem., Int. Ed., 2019, 58, 1183 CrossRef CAS.
- Y. Xie, L. Li, S. Sun, Z. Wu, M. Lang, D. Jiang and J. Wang, Enantioselective NHC-Catalyzed [3+3] Annulation of α-Bromoenals with 2-Aminobenzimidazoles, Org. Lett., 2020, 22, 391 CrossRef CAS.
- D. Meng, Y. Xie, Q. Peng and J. Wang, NHC-Catalyzed Enantioselective [3+3] Annulation to Construct 5,6-Dihydropyrimidin-4-Ones, Org. Lett., 2020, 22, 7635 CrossRef CAS PubMed.
- S. Zhang, C. Lin, C. Liu and D. Du, Enantioselective Synthesis of δ-Carbolinones via N-Heterocyclic Carbene Catalysis, J. Org. Chem., 2022, 87, 10441 CrossRef CAS PubMed.
- S. Barik, R. C. Das, K. Balanna and A. T. Biju, Kinetic Resolution Approach to the Synthesis of C–N Axially Chiral N-Aryl Aminomaleimides via NHC-Catalyzed [3+3] Annulation, Org. Lett., 2022, 24, 5456 CrossRef CAS PubMed.
- A. Ghosh, S. Barik and A. T. Biju, NHC-Catalyzed [3 + 3] Annulation of Thioamides and Modified Enals for the Enantioselective Synthesis of Functionalized Thiazinones, Org. Lett., 2019, 21, 8598 CrossRef CAS PubMed.
- S. Barik, S. Shee, R. G. Gonnade and A. T. Biju, Enantioselective Synthesis of Dihydrothiopyranones via NHC-Catalyzed [3 + 3] Annulation of 2-Bromoenals with β-Oxodithioesters, Org. Lett., 2022, 24, 8848 CrossRef CAS PubMed.
- J. Mo, L. Shen and Y. R. Chi, Direct β-Activation of Saturated Aldehydes to Formal Michael Acceptors through Oxidative NHC Catalysis, Angew. Chem., Int. Ed., 2013, 52, 8588 CrossRef CAS PubMed.
-
(a) T. Akiyama, J. Itoh, K. Yokota and K. Fuchibe, Enantioselective Mannich–Type Reaction Catalyzed by a Chiral Brønsted Acid, Angew. Chem., Int. Ed., 2004, 43, 1566 CrossRef CAS;
(b) D. Uraguchi and M. Terada, Chiral Brønsted Acid-Catalyzed Direct Mannich Reactions via Electrophilic Activation, J. Am. Chem. Soc., 2004, 126, 5356 CrossRef CAS PubMed;
(c) T. Akiyama, Stronger Brønsted Acids, Chem. Rev., 2007, 107, 5744 CrossRef CAS;
(d) D. Parmar, E. Sugiono, S. Raja and M. Rueping, Complete Field Guide to Asymmetric BINOL-Phosphate Derived Brønsted Acid and Metal Catalysis: History and Classification by Mode of Activation; Brønsted Acidity, Hydrogen Bonding, Ion Pairing, and Metal Phosphates, Chem. Rev., 2014, 114, 9047 CrossRef CAS;
(e) H. Wu, Y.-P. He and F. Shi, Recent Advances in Chiral Phosphoric Acid Catalyzed Asymmetric Reactions for the Synthesis of Enantiopure Indole Derivatives, Synthesis, 2015, 1990 CrossRef CAS;
(f) Z.-L. Xia, Q.-F. Xu-Xu, C. Zheng and S.-L. You, Chiral Phosphoric Acid-Catalyzed Asymmetric Dearomatization Reactions, Chem. Soc. Rev., 2020, 49, 286 RSC.
- J. Huang, S. Luo and L. Gong, Chiral Brønsted Acid Catalyzed Stereoselective [3+3] Cycloaddition Reaction of Azomethine Ylide to Vinylimine Intermediates: Constructing Fully Functionalized Chiral Tetrahydro-β-Carboline, Acta Chim. Sin., 2013, 71, 879 CrossRef CAS.
- F. Shi, R.-Y. Zhu, W. Dai, C.-S. Wang and S.-J. Tu, Catalytic Asymmetric Formal [3+3] Cycloaddition of an Azomethine Ylide with 3−Indolylmethanol: Enantioselective Construction of a Six–Membered Piperidine Framework, Chem. – Eur. J., 2014, 20, 2597 CrossRef CAS PubMed.
- W. Dai, H. Lu, X. Li, F. Shi and S.-J. Tu, Diastereo– and Enantioselective Construction of a Bispirooxindole Scaffold Containing a Tetrahydro–β–carboline Moiety through an Organocatalytic Asymmetric Cascade Reaction, Chem. – Eur. J., 2014, 20, 11382 CrossRef CAS.
- For a review:
(a) H.-H. Zhang and F. Shi, Advances in Catalytic Asymmetric Reactions Using 2-Indolylmethanols as Platform Molecules, Chin. J. Chem., 2022, 42, 3351 CAS;
(b) For some examples: H.-H. Zhang, C.-S. Wang, C. Li, G.-J. Mei, Y. Li and F. Shi, Design and Enantioselective Construction of Axially Chiral Naphthyl–Indole Skeletons, Angew. Chem., Int. Ed., 2017, 56, 116 CrossRef CAS;
(c) Z.-Q. Zhu, Y. Shen, J.-X. Liu, J.-Y. Tao and F. Shi, Enantioselective Direct α-Arylation of Pyrazol-5-ones with 2-Indolylmethanols via Organo-Metal Cooperative Catalysis, Org. Lett., 2017, 19, 1542 CrossRef CAS PubMed;
(d) Z.-Q. Zhu, L. Yu, M. Sun, G.-J. Mei and F. Shi, Regioselective [3+3] Cyclization of 2−Indolymethanols with Vinylcyclopropanes via Metal Catalysis, Adv. Synth. Catal., 2018, 360, 3109 CrossRef CAS.
- X.-X. Sun, H.-H. Zhang, G.-H. Li, Y.-Y. He and F. Shi, Catalytic Enantioselective and Regioselective [3+3] Cycloadditions Using 2−Indolylmethanols as 3 C Building Blocks, Chem. – Eur. J., 2016, 22, 17526 CrossRef CAS.
- T.-Z. Li, S.-J. Liu, Y.-W. Sun, S. Deng, W. Tan, Y. Jiao, Y.-C. Zhang and F. Shi, Regio- and Enantioselective [3+3] Cycloaddition of Nitrones with 2-Indolylmethanols Enabled by Cooperative Organocatalysis, Angew. Chem., Int. Ed., 2021, 60, 2355 CrossRef CAS PubMed.
-
(a) Y.-C. Shi, X.-Y. Yan, P. Wu, S. Jiang, R. Xu, W. Tan and F. Shi, Design and Application of m-Hydroxybenzyl Alcohols in Regioselective [3+3] Cycloadditions of 2−Indolymethanols, Chin. J. Chem., 2023, 41, 27 CrossRef CAS;
(b) T.-Z. Li, S.-J. Liu, S.-F. Wu, Q. Cheng, Q. Chen, Y. Jiao, Y.-C. Zhang and F. Shi, Catalytic Asymmetric [3+3] Cycloaddition between Different 2-Indolylmethanols, Sci. China: Chem., 2024 DOI:10.1007/s11426-023-1927-3.
-
(a) K. Bera and C. Schneider, Brønsted Acid Catalyzed [3+2]–Cycloaddition of 2−Vinylindoles with In Situ Generated 2−Methide–2H–indoles: Highly Enantioselective Synthesis of Pyrrolo[1,2−a]Indoles, Chem. – Eur. J., 2016, 22, 7074 CrossRef CAS PubMed;
(b) K. Bera and C. Schneider, Brønsted Acid Catalyzed [3+2]-Cycloaddition
of Cyclic Enamides with in Situ Generated 2-Methide-2H-Indoles: Enantioselective Synthesis of Indolo[1,2-a]Indoles, Org. Lett., 2016, 18, 5660 CrossRef CAS.
- X.-X. Sun, C. Li, Y.-Y. He, Z.-Q. Zhu, G.-J. Mei and F. Shi, Catalytic Asymmetric [3+3] Cycloaddition of Azomethine Ylides with C3−Substituted 2−Indolylmethanols, Adv. Synth. Catal., 2017, 359, 2660 CrossRef CAS.
- C. Li, H. Lu, X.-X. Sun, G.-J. Mei and F. Shi, Diastereo- and Enantioselective Construction of Spirooxindole Scaffolds through a Catalytic Asymmetric [3+3] Cycloaddition, Org. Biomol. Chem., 2017, 15, 4794 RSC.
- Y. Liu, X. Peng, R. She, X. Zhou and Y. Peng, Catalytic Asymmetric (3 + 3) Cycloaddition of Oxyallyl Zwitterions with α-Diazomethylphosphonates, Org. Lett., 2021, 23, 7295 CrossRef CAS PubMed.
- A. G. Woldegiorgis, Z. Han and X. Lin, Asymmetric [3+3] Annulation to Construct Trifluoromethylated Pyrazolo[3,4-b]Pyridin-6-Ones via Chiral Phosphoric Acid and MgSO4 Synergistic Catalysis, Org. Lett., 2022, 24, 4058 CrossRef CAS.
- For some examples:
(a) V. B. Birman and L. Guo, Kinetic Resolution of Propargylic Alcohols Catalyzed by Benzotetramisole, Org. Lett., 2006, 8, 4859 CrossRef CAS;
(b) X. Yang, G. Lu and V. B. Birman, Benzotetramisole-Catalyzed Dynamic Kinetic Resolution of Azlactones, Org. Lett., 2010, 12, 892 CrossRef CAS;
(c) C. Joannesse, C. P. Johnston, C. Concellón, C. Simal, D. Philp and A. D. Smith, Isothiourea–Catalyzed Enantioselective Carboxy Group Transfer, Angew. Chem., Int. Ed., 2009, 48, 8914 CrossRef CAS PubMed;
(d) Q. Peng, D. Guo, B. Zhang, L. Liu and J. Wang, Benzotetramisole catalyzed kinetic resolution of 2H-azirines, Chem. Commun., 2020, 56, 12427 RSC;
(e) For a review: J. Merad, J.-M. Pons, O. Chuzel and C. Bressy, Enantioselective Catalysis by Chiral Isothioureas, Eur. J. Org. Chem., 2016, 5589 CrossRef CAS.
- E. R. T. Robinson, C. Fallan, C. Simal, A. M. Z. Slawin and A. D. Smith, Anhydrides as α,β-Unsaturated Acyl Ammonium Precursors: Isothiourea-Promoted Catalytic Asymmetric Annulation Processes, Chem. Sci., 2013, 4, 2193 RSC.
- E. R. T. Robinson, D. M. Walden, C. Fallan, M. D. Greenhalgh, P. H.-Y. Cheong and A. D. Smith, Non-Bonding 1,5-S⋯O Interactions Govern Chemo- and Enantioselectivity in Isothiourea-Catalyzed Annulations of Benzazoles, Chem. Sci., 2016, 7, 6919 RSC.
- M. D. Greenhalgh, S. Qu, A. M. Z. Slawin and A. D. Smith, Multiple Roles of Aryloxide Leaving Groups in Enantioselective Annulations Employing α,β-Unsaturated Acyl Ammonium Catalysis, Chem. Sci., 2018, 9, 4909 RSC.
- S. Zhang, M. D. Greenhalgh, A. M. Z. Slawin and A. D. Smith, Tandem Sequential Catalytic Enantioselective Synthesis of Highly-Functionalised Tetrahydroindolizine Derivatives, Chem. Sci., 2020, 11, 3885 RSC.
-
(a) X. Lu, C. Zhang and Z. Xu, Reactions of Electron-Deficient Alkynes and Allenes under Phosphine Catalysis, Acc. Chem. Res., 2001, 34, 535 CrossRef CAS;
(b) L.-W. Ye, J. Zhou and Y. Tang, Phosphine-Triggered Synthesis of Functionalized Cyclic Compounds, Chem. Soc. Rev., 2008, 37, 1140 RSC;
(c) S.-X. Wang, X. Han, F. Zhong, Y. Wang and Y. Lu, Novel Amino Acid Based Bifunctional Chiral Phosphines, Synlett, 2011, 2766 CAS.
- L. Zhang, H. Liu, G. Qiao, Z. Hou, Y. Liu, Y. Xiao and H. Guo, Phosphine-Catalyzed Highly Enantioselective [3+3] Cycloaddition of Morita−Baylis−Hillman Carbonates with C,N-Cyclic Azomethine Imines, J. Am. Chem. Soc., 2015, 137, 4316 CrossRef CAS PubMed.
- L. Zhou, C. Yuan, C. Zhang, L. Zhang, Z. Gao, C. Wang, H. Liu, Y. Wu and H. Guo, Enantioselective Synthesis of Quinazoline–Based Heterocycles through Phosphine–Catalyzed Asymmetric [3+3] Annulation of Morita−Baylis−Hillman Carbonates with Azomethine Imines, Adv. Synth. Catal., 2017, 359, 2316 CrossRef CAS.
-
(a) Z. Shao and H. Zhang, Combining Transition Metal Catalysis and Organocatalysis: A Broad New Concept for Catalysis, Chem. Soc. Rev., 2009, 38, 2745 RSC;
(b) A. E. Allen and D. W. C. MacMillan, Synergistic Catalysis: A Powerful Synthetic Strategy for New Reaction Development, Chem. Sci., 2012, 3, 633 RSC;
(c) D.-F. Chen, Z.-Y. Han, X.-L. Zhou and L.-Z. Gong, Asymmetric Organocatalysis Combined with Metal Catalysis: Concept, Proof of Concept, and Beyond, Acc. Chem. Res., 2014, 47, 2365 CrossRef CAS PubMed.
- Z.-J. Zhang, L. Zhang, R.-L. Geng, J. Song, X.-H. Chen and L.-Z. Gong, N-Heterocyclic Carbene/Copper Cooperative Catalysis for the Asymmetric Synthesis of Spirooxindoles, Angew. Chem., Int. Ed., 2019, 58, 12190 CrossRef CAS.
- Y.-C. Zhang, R.-L. Geng, J. Song and L.-Z. Gong, Isothiourea and Brønsted Acid Cooperative Catalysis: Enantioselective Construction of Dihydropyridinones, Org. Lett., 2020, 22, 2261 CrossRef CAS PubMed.
- J. Zhang, Y.-S. Gao, B.-M. Gu, W.-L. Yang, B.-X. Tian and W.-P. Deng, Cooperative N-Heterocyclic Carbene and Iridium Catalysis Enables Stereoselective and Regiodivergent [3 + 2] and [3 + 3] Annulation Reactions, ACS Catal., 2021, 11, 3810 CrossRef CAS.
- Y.-H. Wen, F. Yang, S. Li, X. Yao, J. Song and L.-Z. Gong, Diastereodivergent Desymmetric Annulation to Access Spirooxindoles: Chemical Probes for Mitosis, J. Am. Chem. Soc., 2023, 145, 4199 CrossRef CAS.
-
(a) A. Hazra, A. Ghosh, N. Yadava and P. Banerjee, Organocatalytic (3+3)-cycloaddition of ortho-substituted phenyl nitrones with aryl cyclopropane carbaldehydes: a facile access to enantioenriched 1,2-oxazinanes, Chem. Commun., 2023, 59, 11133 RSC;
(b) A. Hazra, R. Dey, A. Kushwaha, T. J. D. Kumar and P. Banerjee, Organocatalytic Activation of Donor–Acceptor Cyclopropanes: A Tandem (3 + 3)-Cycloaddition/Aryl Migration toward the Synthesis of Enantioenriched Tetrahydropyridazines, Org. Lett., 2023, 25, 5470 CrossRef CAS PubMed;
(c) X. Song, W. Qin, X. Wang, G. Luo and Q. Ni, Bifunctional Squaramide-Catalyzed Asymmetric Cascade Reaction of Benzothiazoles with 2-Nitroallylic Acetates or Nitroenynes, Org. Lett., 2023, 25, 9164 CrossRef CAS;
(d) S.-J. Wang, X. Wang, X. Xin, S. Zhang, H. Yang, M. W. Wong and S. Lu, Organocatalytic diastereo- and atroposelective construction of N–N axially chiral pyrroles and indoles, Nat. Commun., 2024, 15, 518 CrossRef CAS;
(e) S. S. Ranganathappa, B. S. Dehury, G. K. Singh, S. Shee and A. T. Biju, Atroposelective Synthesis of N−N Axially Chiral Indoles and Pyrroles via NHC-Catalyzed Diastereoselective (3 + 3) Annulation Strategy, ACS Catal., 2024, 14, 6965 CrossRef CAS.
|
This journal is © the Partner Organisations 2024 |
Click here to see how this site uses Cookies. View our privacy policy here.