DOI:
10.1039/D4QO01334D
(Review Article)
Org. Chem. Front., 2024, Advance Article
Synthesis and applications of fluorinated, polyfluoroalkyl- and polyfluoroaryl-substituted 1,2,3-triazoles
Received
19th July 2024
, Accepted 22nd August 2024
First published on 28th August 2024
Abstract
1,2,3-Triazoles and organofluorine substitutents are both important structural and functional motifs used in a range of applications. This review summarises the confluence of these two important moities and provides a comprehensive account of the methods for synthesising and applying fluorine-, polyfluoroalkyl- and polyfluoroaryl-substituted 1,2,3-triazoles. Focus has been devoted to recent examples and applications where the properties of both the 1,2,3-triazole and organofluorine substituent are proposed to be important in enhancing the compound's functionality.
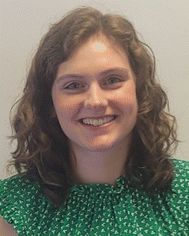 Frances E. Bugden | Frances Bugden obtained her integrated MChem in Chemistry with Drug Discovery from the University of Strathclyde, Glasgow in 2020 which included a year in the pharmaceutical industry. She then moved to the University of Warwick to carry out her PhD studies under the supervision of Dr Mark Greenhalgh in the area of chalcogen bonding catalysis using Se- and Te-functionalised triazoles. Since completing her PhD in 2024, she has transitioned from academia into the pharmaceutical industry. |
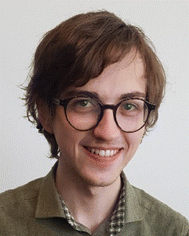 Jason L. Westwood | Jason L. Westwood was born in Birmingham and studied chemistry at the University of Warwick, obtaining a Bachelor of Science degree in 2022. He then completed a Master of Science by Research at the same institution, under the supervision of Dr Mark Greenhalgh. Currently, he is continuing his research for a PhD in organic chemistry in Dr Mark Greenhalgh's group at the University of Warwick. |
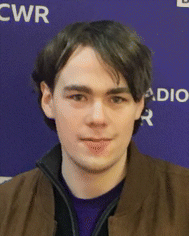 Henry Stone | Henry Stone is originally from Oxfordshire and obtained an integrated MChem degree from the University of Warwick in 2022. Henry completed his final year Masters research project under the supervision of Dr Stefan Roesner and Prof. Mike Shipman on the stereoselective synthesis of 1,2-diazetidine-3-carboxylic acid derivatives for use in peptidomimetics. Currently, Henry is working as a PhD student in the Greenhalgh group on a project sponsored by GoldenKeys® High-tech Materials Co., Ltd. |
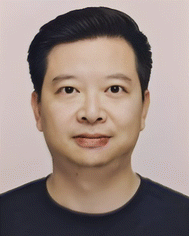 Yingjian Xu | Prof. Dr Yingjian Xu CCHEM FRSC is the CEO of GoldenKeys® High-tech Materials Co., Ltd, and an Honorary Professor at the University of Warwick. After his BSc degree at Huaqiao University, he obtained a PhD in organic chemistry at University of Warwick under the supervision of Prof. Martin Wills, during which he developed of a series of patented XuPHOS catalysts. After a postdoctoral position with Prof. John Brown FRS (Oxford), he joined PhosphonicS. In late 2016, he started his own company, which specialises in organometallic catalysts, metal-ligating functionalised materials and molecular purification technologies for pharmaceutical and fine chemical industries. |
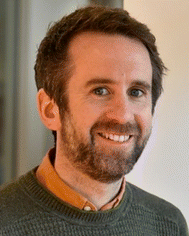 Mark Greenhalgh | Mark completed his Undergraduate degree at the University of Sheffield before moving to Bristol, and then Edinburgh, to complete a PhD with Stephen Thomas on iron-catalysed hydrofunctionalisation of alkenes and alkynes. Mark then moved to the University of St Andrews to conduct postdoctoral work with Andrew Smith on the development and mechanistic understanding of organocatalytic enantioselective methodologies. In April 2020, Mark moved to the University of Warwick as an Assistant Professor to start his independent research group and was promoted to Associate Professor in 2024. His group work on utilising unconventional non-covalent interactions for catalysis and drug discovery. |
1. Introduction
1,2,3-Triazoles are incredibly important motifs used in a range of fields due to their high thermal, chemical and biological stability. They possess two lone pairs available for bonding and have a relatively high dipole moment of >4 D,1 leading to triazoles being both good hydrogen bond acceptors/transition metal ligands and good hydrogen bond donors. Based on these various properties, 1,2,3-triazole derivatives have found applications in drug discovery,2 bioconjugation,2a,3 carbohydrate,4 peptide,5 and DNA chemistry,6 supramolecular chemistry,7 polymer science8 and sensing applications,9 amongst others.10
The widespread application of 1,2,3-triazoles is also due to their ease of synthesis. The thermal azide–alkyne cycloaddition (AAC), originally reported by Michael11 and later formalised and popularised by Huisgen,12 provides simple access to 1,2,3-triazoles, however often as a mixture of regioisomers (Scheme 1A). In 2002, the groups of Meldal and Sharpless independently reported the use of a copper(I) catalyst that promotes the AAC reaction between azides and terminal alkynes at room temperature, and provides only the 1,4-regioisomer of triazole product (Scheme 1B).13 Following this seminal work, a wide range of transition metal catalysts (Ru, Ir, Rh, Ag, Ni, Zn, Au) have been reported for AAC reactions.14 Depending on the catalyst, selective access to either the 1,4- or 1,5-regioisomer of triazole is now possible with both terminal and internal alkynes (Scheme 1C). The use of strained alkynes has also been used to accelerate the metal-free AAC reaction through strain release,3b,c with this approach particularly suited to bioconjugation strategies (Scheme 1D). Beyond AAC reactions, organocatalytic and base-mediated approaches to 1,2,3-triazoles have been reported from ketones and azides (Scheme 1E).14e,15 Finally, a range of azide-free approaches have been developed, which typically use hydrazine, hydrazone or diazo derivatives as the nitrogen-rich substrate.14e,15b,c,16
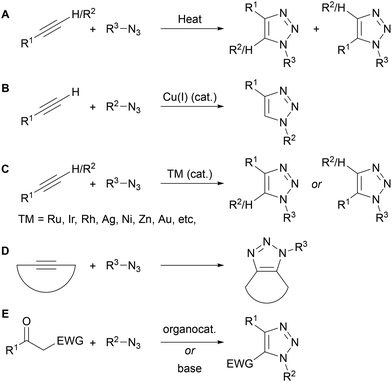 |
| Scheme 1 Synthetic approaches to 1,2,3-triazoles. | |
Organofluorine compounds have also attracted significant interest in a range of fields due to their unique physicochemical, electrochemical and biological properties, which are often distinct from their non-fluorinated analogues.17 Many of these differences originate from the high electronegativity of fluorine which results in high dipole moments, low polarisability, small size and strong C–F bonds.18 The main area of application is within medicinal chemistry,19 where the introduction of fluorine impacts various pharmacological properties of the drug candidate, including membrane permeability, metabolic stability, lipophilicity, and binding affinity. Beyond medicinal chemistry, the unique properties of organofluorine compounds have also resulted in their application in agrochemicals20 and functional materials.17,21
Combining the unique structural, chemical and biological properties of 1,2,3-triazoles and fluorine therefore provides great potential for applications across a range of fields.
Fluorine may be incorporated directly on the triazole ring at the C(4) (1) or C(5) (2) position, or alternatively polyfluoroakyl/aryl substituents may be incorporated at the N(1) (3), C(4) (4) or C(5) (5) position (Fig. 1). This review will provide a thorough summary of recent advances in the synthesis of these classes of triazoles, in addition to an overview of their applications. Focus has been devoted to applications where the properties of both the 1,2,3-triazole and organofluorine substituent are proposed to play essential roles in the compound's functionality. Several specialised reviews have been released on aspects of the synthesis or applications of fluorinated triazoles,22–24 however to the best of our knowledge no comprehensive review on the subject currently exists.
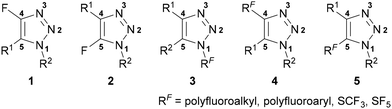 |
| Fig. 1 Fluorinated triazole structures covered in this review. | |
2. Synthesis of C(4) and C(5) fluorinated 1,2,3-triazoles
2.1. Introduction
The introduction of fluorine at the C(4) or C(5) position of a 1,2,3-triazole would ideally be achieved using a fluoroalkyne, however, these substrates are relatively difficult to synthesise and are typically unstable.25 The earliest preparation of fluoroacetylene 8 was reported by Middleton and Sharkey in 1959 through the pyrolysis of monofluoro-maleic anhydride at high temperature (650 °C) and low pressure (5–7 mmHg).26 In 1982, Sauvêtre reported that lithiation of 1,1-difluoroethylene 6 and subsequent β-elimination of LiF gave fluoroacetylene 8 (Scheme 2A). However, both fluoroacetylene 6 and the lithiated intermediate 7 are unstable and explosive.26,27 In the same year Taylor28 synthesised perfluoropropyne 10 through the debromination of dibromotetrafluoropropene 9 (Scheme 2B). Perfluoropropyne 10 was obtained by condensation at −196 °C. Due to the challenges of synthesising and handling fluoroalkynes, approaches to access 4- and 5-fluorotriazoles that avoid the use of a fluoroalkyne have been pursued.
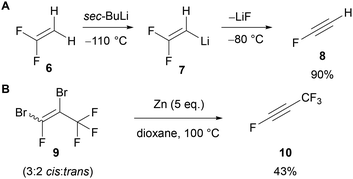 |
| Scheme 2 Preparation of fluoroalkynes. | |
Some traction has been gained in the use of fluoroalkyne surrogates to circumvent the use of unstable fluoroalkynes.29 In general, these ‘surrogates’ are fluoroalkenes bearing an electron-withdrawing substituent which serves two purposes; (i) as an activating group to promote the initial reaction with an azide, and (ii) as a leaving group to provide the triazole in the final step (Scheme 3).
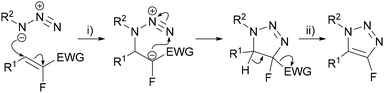 |
| Scheme 3 Fluoroalkyne ‘surrogate’ approach. | |
The first example of this ‘surrogate’ approach was reported by Reck in 2005,29a using α-fluorovinylsulfone 12 (Scheme 4). A thermal AAC reaction with oxazolidinone-functionalised azide 11 at 110 °C gave a 1
:
7 mixture of the 1,4- and 1,5-regioisomers 13 and 14 in a combined yield of 28%.
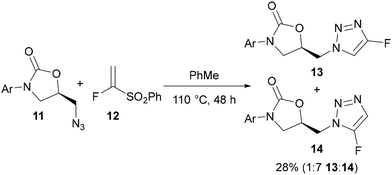 |
| Scheme 4 Cycloaddition-elimination using α-fluorovinylsulfone fluoroalkyne surrogate 12.29a | |
2.2. 4-Fluoro-1,2,3-triazoles
Building on Reck's initial work, Roy29b and Nenajdenko29c showed the efficiency and regioselectivity of these reactions can be improved by using an α-fluoronitroalkene 16 as a fluoroalkyne surrogate in combination with a Brønsted acid catalyst (Schemes 6 and 7). The preparation of α-fluoronitroalkenes 16 was achieved in a ‘one-pot’ process from a benzaldehyde derivative 15 via an α-fluorobromoalkene intermediate (Scheme 5A).29b,30 It is worth noting that this procedure is only applicable for the synthesis of β-aryl α-fluoronitroalkenes 16 as conversion of the α-fluorobromoalkene intermediate to the final nitroalkene product requires stabilisation of a radical intermediate by the aryl substituent. However, Beier has reported an alternative Horner–Wadsworth–Emmons approach using diethyl fluoronitromethylphosphonate 18 (Scheme 5B), which allows access to both β-alkyl- and β-aryl α-fluoronitroalkenes.31 However, to the best of our knowledge these β-alkyl α-fluoronitroalkenes have not yet been applied for triazole synthesis.
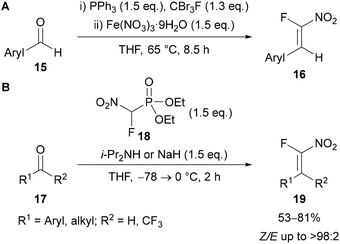 |
| Scheme 5 Preparation of α-fluoronitroalkenes (A) via an α-fluorobromoalkene intermediate; (B) through a Horner–Wadsworth–Emmons approach. | |
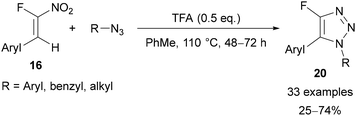 |
| Scheme 6 Synthesis of 1,5-disubstituted-4-fluoro-triazoles 20 from α-fluoronitroalkenes 16. | |
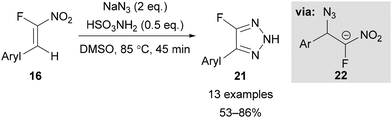 |
| Scheme 7 Synthesis of 4-fluoro-NH-1,2,3-triazoles 21.29c | |
Roy utilised β-aryl α-fluoronitroalkenes 16 for the regioselective preparation of 1,5-disubstituted-4-fluoro-1,2,3-triazoles 20 in good yields (Scheme 6).29b Through screening a range of Lewis and Brønsted acids, it was shown that regioselectivity and yield was significantly enhanced by using trifluoroacetic acid (TFA). This can be explained by the mechanism given in Scheme 3, where protonation of the electron-withdrawing nitro group can further promote regioselective product formation. A range of aryl- and alkyl-substituted azides were tolerated, however aryl azides bearing electron-withdrawing groups gave lower yields.29b α-Fluoronitroalkenes bearing β-aryl groups with para- or meta-substitution were tolerated, with those bearing electron-donating groups providing the highest yields.
Nenadjenko showed the addition of sodium azide to β-aryl α-fluoronitroalkene 16 in the presence of sulfamic acid led to the formation of 4-fluoro-1,2,3-NH-triazoles 21 (Scheme 7).29c Low nitroalkene concentration was necessary for a successful reaction, with this requirement suggested to minimise the polymerisation of proposed intermediate 22 (Scheme 7). In addition to activating the α-fluoronitroalkene to nucleophilic addition, sulfamic acid was also suggested to promote protonation of intermediate 22 to favour triazole formation over polymerisation. The NH-triazoles were isolated in good yields, and the reaction is tolerant of a variety of aryl substituents, except for those bearing electron-withdrawing groups, which were liable to undergo competitive anionic polymerisation.
Inspired by the work of Sauvêtre (Scheme 2A),27 Ichikawa reported difluorovinylzinc N,N,N′,N′-tetramethylethylenediamine (TMEDA) complex 24 as a thermally stable fluoroacetylene equivalent (Scheme 8).32 Lithiation of 1,1-difluoroethylene 23, followed by addition of zinc chloride and TMEDA gives difluorovinylzinc TMEDA complex 24 in excellent yield. The reagent was reported to be thermally stable, allowing it to be stored and used as a solution in THF/Et2O. Ichikawa initially used this reagent in palladium-32 and copper-catalysed32,33 cross-coupling reactions with aryl, alkenyl, alkynyl, allyl, and benzyl coupling partners, before applying it in the formation of 4-fluoro-1,2,3-triazoles in 2020.34
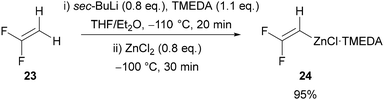 |
| Scheme 8 Preparation of difluorovinylzinc complex 24. | |
In the presence of CuCl and 1,10-phenanthraline, Ichikawa demonstrated that difluorovinylzinc TMEDA complex 24 could be applied in the copper-catalysed synthesis of a variety of 4-fluoro-triazoles 25 in good yields (Scheme 9).34 A range of substituted benzylic azides were tolerated; however, the application of aryl and allylic azides required the use of 2.5 eq. of 24 to achieve good yields.
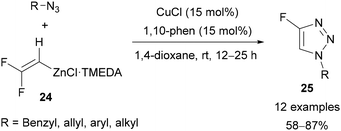 |
| Scheme 9 Copper-catalysed synthesis of 4-fluoro-1,2,3-triazoles 25 from difluorovinylzinc TMEDA complex 24. | |
A mechanism for this reaction was proposed to begin with transmetallation of 24 to give Cu–fluoroalkene 26, followed by co-ordination of CuCl (Scheme 10). This allows for oxidative cyclisation to 6-membered metallocycle 28 followed by reductive elimination and β-fluoride elimination to give the triazole product 25. The proposal of one σ-bonded Cu and one π-complexed Cu is in line with the mechanism described by Fokin for copper-catalysed alkyne–azide cycloadditions.35 An alternative pathway through initial β-fluoride elimination to give in situ fluoroacetylene was considered but discounted through preliminary mechanistic experiments.34
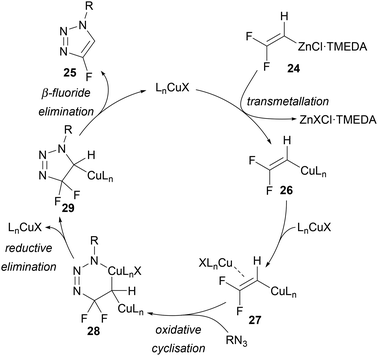 |
| Scheme 10 Ichikawa's proposed mechanism for the copper-catalysed synthesis of 4-fluoro-1,2,3-triazoles 25 from difluorovinylzinc TMEDA complex 24. | |
Building on previous work on the reaction of β-ketophosphonates and azides to give 1,5-disubstituted triazoles,36 Chou demonstrated that a range of 1,4,5-trisubstituted triazoles could be produced in the base-mediated reaction of α-substituted-β-ketophosphonates and azides.37 Of the 35 examples reported, two demonstrated the introduction of a fluorine at the C(4) position of the triazole 31, by using the appropriate α-fluoro-β-ketophosphonate 30 (Scheme 11).
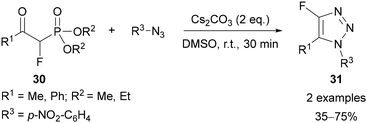 |
| Scheme 11 Base-mediated synthesis of 4-fluoro-1,2,3-triazoles 31 from α-fluoro-β-ketophosphonates 30 and azides. | |
2.3. 5-Fluoro-1,2,3-triazoles
The synthesis of 5-fluoro-1,2,3-triazoles 33 has been exclusively achieved from 5-iodo-1,2,3-triazoles 32 through a so-called “halogen exchange” (Halex) reaction.38 This approach was first reported by Fokin in 2012 using KF at 180 °C under microwave irradiation (Scheme 12A).38 The reaction is tolerant of various functional groups in both the N(1) and C(4) positions, except for alkyl chains at C(4), which led to a complex mixture of products. Additionally, the high temperature required for this reaction led to decomposition over long reaction times. The work was extended to include amide, ketone, nitrile and N-p-toluenesulfonyl functional groups by using the acidic KHF2 reagent in place of KF (Scheme 12B).38
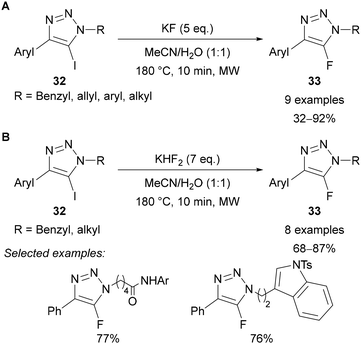 |
| Scheme 12 Halex reaction reported by Fokin38 using (A) KF; (B) KHF2. | |
Neither of the other regioisomers of iodotriazole (34 or 35) could be applied in this methodology, with >98% of the starting iodotriazole recovered in each case (Scheme 13).38
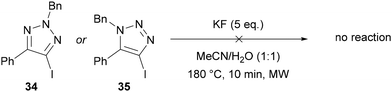 |
| Scheme 13 Alternative iodotriazole regioisomers unreactive under Halex reaction conditions. | |
Since 5-iodotriazole 32 can undergo a Dimroth-like fragmentation through ring-chain isomerisation,39 it was proposed the reaction may proceed via imidoyl iodide 36, which could undergo iodide–fluoride exchange and ring-chain isomerisation to give 5-fluorotriazole 33 (Scheme 14A). This proposal is consistent with neither of the other regioisomers of iodotriazole being applicable in this reaction (Scheme 13) and is supported by the known reaction of imidoyl halides to give fluoride analogues under similar conditions.40 Although this mechanism was favoured by the authors, they could not rule out the possibility of an SNAr-type pathway (Scheme 14B).
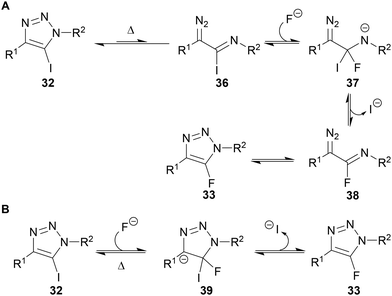 |
| Scheme 14 (A) Fokin's proposed mechanism for Halex reaction; (B) alternative SNAr mechanism.38 | |
In 2015, Chu further developed the Halex reaction by demonstrating that AgF could be used as the fluoride source in the presence of TMEDA as a solubilising agent in toluene at reflux (Scheme 15).41 This method was applicable to a wider substrate scope, in particular triazoles bearing C(4) alkyl substituents, which were not tolerated in Fokin's method using KF.38
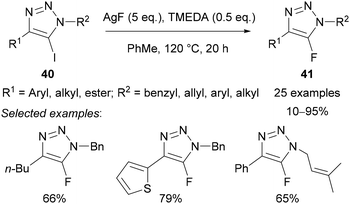 |
| Scheme 15 Alternative Halex reaction conditions reported by Chu using silver fluoride. | |
Protodehalogenated triazole side-products were also observed, which prompted mechanistic studies using deuterated solvents. Using d6-DMSO as solvent provided 63% of the deuterodehalogenated triazole 45 (Scheme 16), whilst the use of D2O in DMF or MeCN led to 32% and 25% of triazole 45, respectively. These studies indicated that the protodehalogenated side-product may form by intercepting an organometallic intermediate, hence, the need for strict exclusion of water in this transformation.
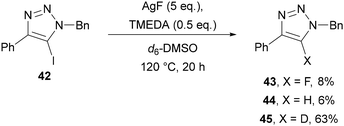 |
| Scheme 16 Deuteration studies. | |
Replacing AgF with KF or CsF provided no conversion, indicating that silver is essential for this reaction. It was therefore proposed that, in contrast to Fokin's method using KF, AgF is not simply providing a nucleophilic source of fluoride. Based on a related mechanism proposed by Ritter for the fluorination of stannanes,42 Chu suggested that oxidative addition of silver into the carbon–iodine bond of 40 gives a bimetallic silver(II) fluoride intermediate 46, which undergoes reductive elimination to give the triazole product 41 (Scheme 17).
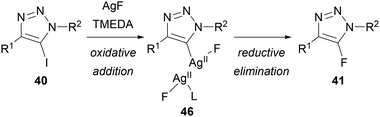 |
| Scheme 17 Mechanistic proposal for Chu's Halex reaction.41 | |
3. Synthesis of C(4) and C(5) polyfluoroalkyl- and polyfluoroaryl-1,2,3-triazoles
3.1. Introduction
The preparation of C(4) and C(5)polyfluoroalkyl-1,2,3-triazoles would be most easily attempted through the use of polyfluoroalkyl-substituted alkynes, however simple analogues are gaseous and potentially unstable species. In the 1960s, Norris43 and then Moore44 used the zinc mediated reduction of dichloroalkenes 47 and 48 for the preparation of 3,3,3-trifluoropropyne 49 and hexafluorobut-2-yne 50, respectively (Scheme 18A). Hamper45 also showed it was possible to prepare α-acetylenic esters through the vacuum pyrolysis of α-acylmethylenephosphorane 51 at 150–200 °C and 1–2 torr (Scheme 18B). This procedure specified the need for slow heating to ensure the unstable alkyne product 52 is not formed too quickly. The issues of thermal instability and difficult handling due to their gaseous nature are akin to those encountered with fluoroacetylene derivatives. Despite the challenges, Hamper's method is still used for the preparation of perfluoroalkyl-substituted α-acetylenic esters.46
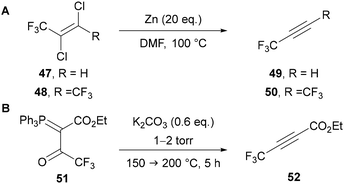 |
| Scheme 18 Preparation of perfluoroalkyl-alkynes. | |
Shibata has reported the use of fluoroform as a source of difluorocarbene for the preparation of difluoromethyl-substituted alkynes 54 from phenylacetylene derivatives 53 (Scheme 19).47 Under basic reaction conditions, fluoroform can be used as a source of the −CF3 carbanion, or as the more stable difluorocarbene and a fluoride anion.48 A range of difluoromethyl-alkynes can be synthesised by this method, including those bearing electron-rich and -poor arenes and heteroarenes.
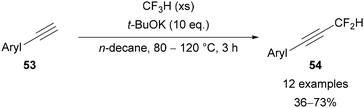 |
| Scheme 19 Synthesis of CF2H-substituted phenylacetylene derivatives. | |
In contrast to polyfluoroalkyl-substituted alkynes, polyfluoroaryl-alkynes are generally more stable and easier to prepare and handle, thus simplifying the synthesis of the corresponding polyfluoroaryl-1,2,3-triazoles. In 1991, Zanardi49 showed that polyfluoroaryl-alkynes bearing a CF3-substituent 56 could be synthesised by base-mediated elimination of HCl from the corresponding chloroalkene 55 (Scheme 20A). Adopting a similar approach to Hamper, Rao showed that β-oxo-alkylidenetriphenylphosphoranes 57 could be transformed to the corresponding polyfluoroaryl-alkynes 58 following just 5 minutes of microwave irradiation (Scheme 20B).50 Finally, polyfluoroaryl-substituted alkynes, such as 59, can be prepared from the corresponding polyfluoroaryliodide through a Sonogashira cross-coupling reaction (Scheme 20C).51
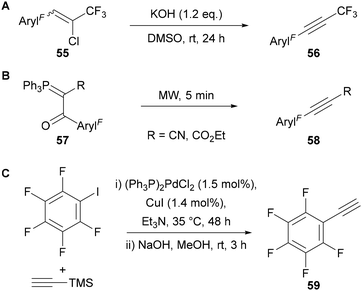 |
| Scheme 20 Preparation of polyfluoroaryl-alkynes. ArylF = polyfluoroaryl. | |
Due to the relative ease with which polyfluoroaryl-substituted alkynes can be accessed, the corresponding triazoles are generally synthesised using well-established metal-catalysed azide–alkyne cycloaddition reactions. As such, these synthetic methods have not been explicitly discussed in this review and the following sections will focus on the synthesis of polyfluoroalkyl-substituted triazoles. Examples of thermal AAC reactions have been reported for the synthesis of polyfluoroalkyl-substituted triazoles,23a,b,44,46b,49,52 often giving mixtures of regioisomers. The following sections will therefore focus on a diverse range of specialised approaches that selectively provide access to a single regioisomer of triazole product.
3.2. 4-Polyfluoroalkyl-1,2,3-triazoles
In 2015, Ma reported the silver-catalysed synthesis of 4-CF3-1,2,3-triazoles 62 from isocyanides 60 and 2,2,2-trifluorodiazoethane 61 (Scheme 21).52,53 It should be noted that low molecular weight diazo compounds are potentially explosive,54 and previous explosions have been reported with 2,2,2-trifluorodiazoethane.55 The approach proved to be regioselective and applicable to a variety of aryl isocyanides, bearing electron-donating and -withdrawing substituents in the ortho-, meta-, and para-positions. Adamantyl isocyanide was also tolerated, although cyclohexyl and t-butyl isocyanides gave only modest conversion to the triazole products.
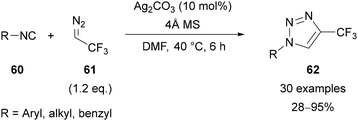 |
| Scheme 21 Synthesis of 4-trifluoromethyl-triazoles from isocyanides and 2,2,2-trifluorodiazoethane. | |
Interestingly, it was reported that if two or more equivalents of the diazo reagent were used, 1,4,5-trisubstituted triazole 63 was isolated in up to 12% yield (Scheme 22). Treatment of the triazole product 62 with 2,2,2-trifluorodiazoethane 61 under the reaction conditions did not lead to the formation of 1,4,5-trisubstituted triazole 63, indicating that this side-product is most likely formed prior to the formation of triazole 62. Based on in situ 13C NMR and IR monitoring, it was suggested that the isocyanide co-ordinates to the silver catalyst to give a silver(I) isocyanide complex,56 which undergoes cycloaddition with 2,2,2-trifluorodiazoethane 61. This provides a Ag-intermediate, which following protonation gives the product 62. Alternatively, reaction of this Ag-intermediate with 2,2,2-trifluorodiazoethane 61 gives side-product 63.
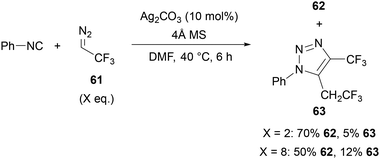 |
| Scheme 22 Formation of side-product 63 in the presence of excess 2,2,2-trifluorodiazoethane 61. | |
In 2020, Panja reported an alternative approach to prepare 4-trifluoromethyl-triazoles 65 through the copper-mediated trifluoromethylation of 4-iodotriazoles 64 (Scheme 23A).57 The use of CuI, methyl-2,2-difluoro-2-(fluorosulfonyl) acetate (MDFA, also known as Chen's reagent),58 and tetrabutylammonium iodide (TBAI) gave the desired trifluoromethyl triazole products 65 in moderate yields. The reaction was proposed to proceed through demethylation of MDFA by iodide to give copper carboxylate complex 66 (Scheme 23B). Decarboxylation results in the loss of CO2 and SO2 to give difluorocarbene59 and a fluoride ion, which can combine to form Cu–CF3 intermediate 67. Finally, this intermediate mediates the cross-coupling with iodotriazole 64 to give the trifluoromethylated triazole product 65.58,60
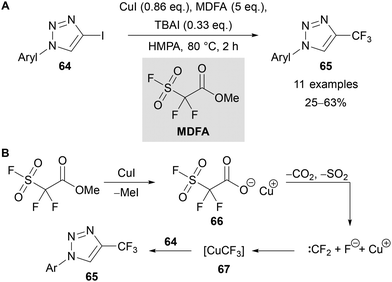 |
| Scheme 23 Synthesis and proposed mechanism for the copper-mediated formation of 4-trifluoromethyltriazoles 65. | |
Topczewski reported an interrupted Banert cascade reaction61 for the preparation of α-fluoro-NH-1,2,3-triazoles 69 (Scheme 24).62 Heating propargylic azide 68 at 60 °C was proposed to promote a [3 + 3]-sigmatropic rearrangement to give allene intermediate 70, which undergoes electrocyclisation to triazafulvene 71. Finally, nucleophilic addition of fluoride provides the α-fluoro-NH-1,2,3-triazole product 69 (Scheme 24).62,63
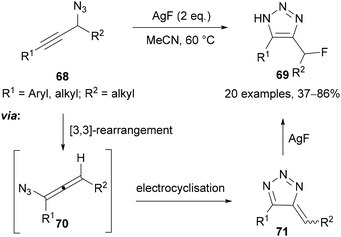 |
| Scheme 24 Banert cascade approach to α-fluoro-NH-1,2,3-triazoles 69. | |
Zajc reported the synthesis of fluorinated 1,4-disubtituted-1,2,3-triazoles 73 from novel fluorinated benzothiazole sulfones 72 (Scheme 25).64 This transformation involves silyl-deprotection followed by a copper-catalysed AAC reaction with aryl or alkyl azides. The benzothiazole sulfone-substituted triazoles 73 were subsequently applied in Julia–Kocienski olefination reactions (see section 6.2).64
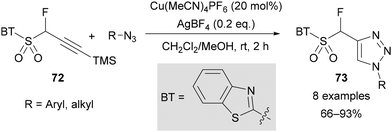 |
| Scheme 25 Copper-catalysed synthesis of α-fluoro-benzothiazole sulfone-substituted triazoles. | |
In 2018, both Moses65a and Fokin65b used 1-bromoethenesulfonyl fluoride 76 (known as BESF or Br–ESF) as a synthetic equivalent for acetylenesulfonyl fluoride for the preparation of 4-sulfonyl fluoride-substituted 1,2,3-triazoles 75. Sulfonyl fluorides are commonly applied reagents in SuFEx click reactions, used in a variety of fields and notably as biological probes.66 Moses showed this reagent could be formed in situ by reacting 1,2-dibromoethane-1-sulfonyl fluoride 74 (DESF) with Et3N for 5 minutes. Addition of an azide and heating to 90 °C, in the absence of any catalyst, provides the 4-sulfonyl fluoride-substituted triazole product 75 as a single regioisomer (Scheme 26A).65a Aryl azides bearing electron-donating and withdrawing groups were tolerated, however, no examples using alkyl azides were demonstrated. It was proposed that following in situ formation of BESF 76, [3 + 2]-cycloaddition of the azide provides triazoline 77, which following elimination of HBr gives triazole 75 (Scheme 26B). Moses also demonstrated the use of in situ generated BESF for the synthesis of isoxazoles and β-sulfams.
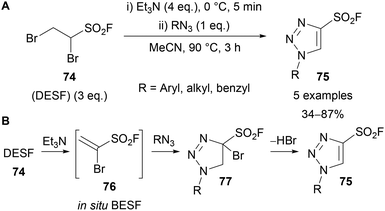 |
| Scheme 26 Preparation and proposed mechanism for the formation of 4-sulfonyl fluoride-substituted 1,2,3-triazoles. | |
Fokin showed it was possible to synthesise and isolate BESF 76 on a 68 g scale by elimination of HBr from 1,2-dibromoethane-1-sulfonyl fluoride at −78 °C (Scheme 27A).65a,67 In the absence of a catalyst, the reaction of BESF 76 with both aryl and alkyl azides gave a wide range of 4-sulfonyl fluoride-substituted 1,2,3-triazoles 78 in good yields (Scheme 27B).
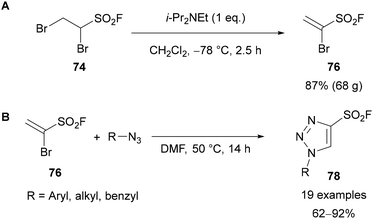 |
| Scheme 27 (A) Large-scale synthesis and isolation of BESF 76; (B) preparation of 4-sulfonyl fluoride-substituted triazoles 78 using BESF 76. | |
In 2020, Moses extended and improved on this approach with the development of alkynyl sulfonyl fluoride reagents 79 that were applied for the synthesis of 12 different classes of heterocycle.68 Within this broad scope, the synthesis of 4-sulfonyl fluoride-substituted triazoles 80 was demonstrated through a Rh-catalysed AAC reaction (Scheme 28).
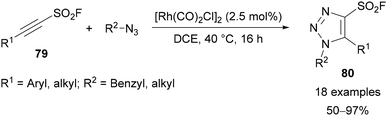 |
| Scheme 28 Synthesis of alkynyl sulfonyl fluoride reagents 79 and application for synthesis of 4-sulfonyl fluoride-substituted triazoles 80. | |
3.3. 5-Polyfluoroalkyl-1,2,3-triazoles
Beyond the use of thermal AAC reactions, efforts have been made to selectively install trifluoromethyl groups in the C(5) position of 1,2,3-triazoles, resulting in various methods published in recent years. An approach that is comparable to Panja's work57 above was reported by Cao in 2013.69 The copper-mediated transformation of 1,4-diaryl-5-iodo-1,2,3-triazole derivatives 81 to the trifluoromethylated analogue 82 was achieved using TMSCF3 (Ruppert–Prakash reagent)70 as the trifluoromethyl source (Scheme 29). The method provides triazoles 82 in good yields and was demonstrated with both electron-neutral and -rich aromatics at the C(4) position and a range of N-benzylic and N-alkyl substituents.
 |
| Scheme 29 Copper-mediated synthesis of 5-trifluoromethyl-substituted 1,2,3-triazoles 82. | |
Tsui published a related interrupted copper-catalysed AAC reaction to prepare 5-trifluoromethyl-1,2,3-triazoles 85 (Scheme 30).71a The cycloaddition of a range of alkynes with azides was achieved using a combination of CuI, 1,10-phenanthroline, TMSCF3, Ag2CO3, and Et3N. Alkynes bearing a range of aryl and ester substituents were tolerated, however only the use of benzylic azides was demonstrated. Improved yields were obtained when TMSCF3 was added at 0 °C before warming the reaction to room temperature, with this approach proposed to slow down the decomposition of the reagent.
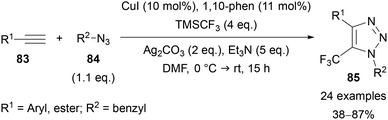 |
| Scheme 30 Interrupted copper-catalysed azide–alkyne cycloaddition for the synthesis of 5-trifluoromethyl-substituted 1,2,3-triazoles 85. | |
A catalytic cycle was proposed based on literature72 with control reactions showing that formation of a CF3-substituted internal alkyne 89 or the protonated triazole 91 prior to CF3 substitution were not relevant for the productive catalytic cycle (Scheme 31). Following formation of copper acetylide 87, in the absence of azide, formation of both diyne 88 and CF3-alkyne 89 can occur. However, when an azide is present, cycloaddition with the copper acetylide gives cuprated triazole 90, which can be protonated by water or protic solvent to form protonated triazole 91 as a side-product. Alternatively, reaction of cuprated triazole intermediate 90 with TMSCF3 in combination with Ag2CO3-mediated oxidation, gives proposed copper(III) intermediate 92. This copper(III) species can undergo reductive elimination to form the product and regenerate catalyst 86.71a Zhu and Li reported a related process, where the silver oxidant used by Tsui was omitted, and the reaction was simply run in the presence of air.71b Of note, a specific glycinamide bis-triazole ligand was required to obtain good selectivity for the trifluoromethylated triazole product over the protonated triazole side-product. The method was also demonstrated with longer chain perfluoroalkylsilanes and applied to a wider range of azides and alkynes than reported by Tsui.
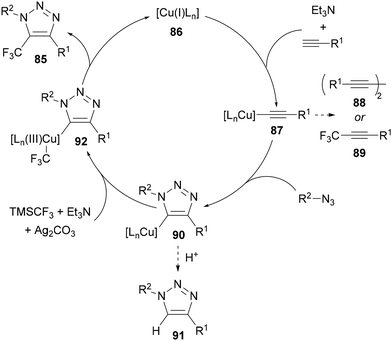 |
| Scheme 31 Proposed catalytic cycle of interrupted CuAAC reaction. | |
Building on their previous work,71a Tsui published an interrupted copper-mediated AAC reaction for the preparation of 5-C2F5-substituted triazoles 95 (Scheme 32A).71c This method requires the preparation of [CuC2F5] from gaseous HCF2CF3, CuCl, t-BuOK and Et3N·HF in a glovebox, however once the fluorinating agent has been prepared it can be used under aerobic conditions. Following a series of control experiments, it was proposed that the reaction proceeds in a similar manner to other interrupted copper-catalysed AAC reactions through formation of a 5-cuprated-triazole intermediate, which undergoes reductive elimination to install the C2F5 group. Interestingly, applying Et3SiC2F5 under the conditions reported in their previous methodology for the synthesis of 5-trifluoromethyl-substituted 1,2,3-triazoles (see Scheme 30)71a led to only 16% yield of the C2F5-substituted triazole 96, demonstrating the advantage of using CuC2F5 in this approach (Scheme 32B).
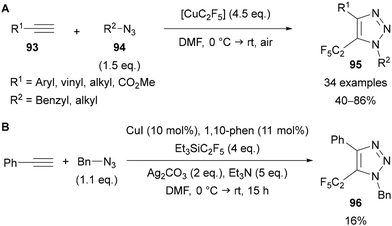 |
| Scheme 32 Copper-mediated synthesis of 5-C2F5-substituted triazoles 95. | |
Beyond the use of copper-mediated couplings and cycloadditions, Zhu reported the formal [3 + 2] cycloaddition between tertiary enamines 97 and azides 98
15 to give 5-polyfluoroalkyl substituted triazoles 99 (Scheme 33A).73a High temperatures and long reaction times were required, and the reactions were generally performed neat, with an excess of azide used as solvent. When benzyl azides were used the addition of sodium carbonate was also required. In a related base-mediated approach,15 Bakulev showed that a range of 5-trifluoromethyl substituted triazoles 102 could be access from trifluoromethyl 1,3-diketones and β-ketoesters 100 in the presence of triethylamine as a Brønsted base (Scheme 33B).73b A range of 1,3-dicarbonyls were applicable, however only azides bearing electron-withdrawing groups gave good yields (for example p-MeOC6H4N3: 12%; BnN3: 9%).
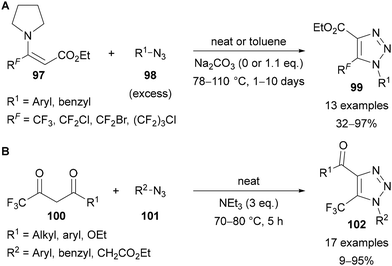 |
| Scheme 33 Enamine and base-mediated synthesis of C(5)-polyfluoroalkyl-substituted triazoles. | |
In 2013, Cao reported a combined copper- and piperidine-catalysed reaction of (hetero)aryl boronic acids 104, ketones 103 and sodium azide for the preparation of 1,4,5-trisubstituted-1,2,3-triazoles 105 (Scheme 34).73c In this method, the aryl boronic acids 102 are converted to the corresponding aryl azides in situ via a copper-catalysed Chan–Lam coupling with sodium azide. Triazole synthesis can then take place through enamine catalysis.15,74 The ketones used all required an anion-stabilising substituent in the β-position, such as another ketone, an ester or a nitrile. Of relevance to this review, trifluoromethyl and difluoromethyl ketones were applied to give CF3 and CF2H substituents in the C(5) position, in addition to fluorinated aryl N(1) substituents.73c
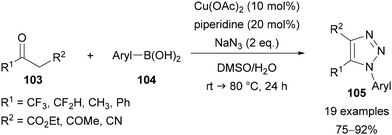 |
| Scheme 34 Copper- and piperidine-catalysed synthesis of triazoles, through Chan–Lam coupling followed by CuAAC. | |
Tverdekhlebov has also demonstrated the preparation of 1-alkyl-5-trifluoromethyl-1,2,3-triazoles 107 through the reaction of secondary enamines 106 with mesyl azide in the presence of DBU (Scheme 35A).75 Calculation of frontier orbital energies by Kascheres in 1993,76 coupled with experimental data led to the following proposed mechanism (Scheme 35B). The nucleophilic enamine 106 reacts with electrophilic mesyl azide, which, following tautomerisation, gives intermediate triazine 108. The electron-withdrawing methanesulfonyl group is necessary to lower the LUMO of the azide and promote this initial reaction.76 An ‘anti-Baldwin’ 5-endo-trig cyclisation of the enamine nitrogen onto the triazine gives intermediate 109, which following DBU-mediated elimination of methanesulfonamide provides triazole product 107.
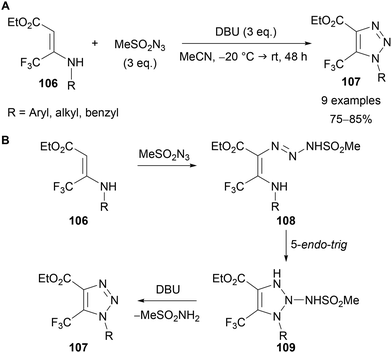 |
| Scheme 35 Use of enamines 106 and mesyl azide for triazole synthesis (A) and proposed mechanism (B). | |
In 2021, Bi reported an alternative route to 5-polyfluoroalkyl triazoles 112 through the reaction of perfluoroalkyl N-mesylhydrazones 110 with amines 111 (Scheme 36).77 This reaction was proposed to proceed by defluorinative condensation of the amine 111 with the perfluoroalkyl N-mesylhydrazone 110, followed by cyclisation and elimination of methanesulfinic acid. A very large scope was reported, with triazoles 112 generally obtained in excellent yields. In addition to simple aryl and alkyl amines, complex natural products and bioactive molecules containing primary amines were also applied. The perfluoroalkyl N-mesylhydrazone substrates 110 were reported to be bench-stable solids that can be synthesised through the condensation of methanesulfonohydrazide and perfluoroalkyl aldehyde hydrates.
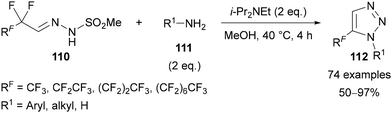 |
| Scheme 36 Defluorinative [4 + 1] annulation of perfluoroalkyl N-mesylhydrazones 110 with primary amines 111. | |
Wu has also reported an alternative approach to C(5)-polyfluoroalkylated triazoles 115 using diazo compounds 113 and polyfluoroalkyl-imidoyl chlorides 114 (Scheme 37).78 A wide range of different polyfluoroalkyl substituents on the imidoyl chloride were demonstrated. While the N-substituent was generally aromatic, an example of an N-alkyl-substituted imidoyl chloride was also successfully applied. Ethyl diazoacetate was generally used, however other stabilised diazo compounds were demonstrated, including those bearing aryl ketones, phosphonates and a trifluoromethyl group. Although some diazo compounds are relatively unstable, this method provides access to triazoles that would be difficult to access through other routes, such as the example with CF3 groups in both the C(4) and C(5) positions.
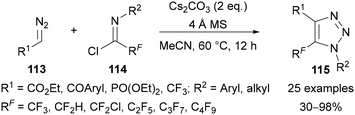 |
| Scheme 37 Synthesis of C(5)-polyfluoroalkylated triazoles 115 from diazo compounds 113 and polyfluoroalkyl-imidoyl chlorides 114. | |
Interrupted copper-mediated AAC reactions have also been used for the synthesis of (5)-SCF3-substituted triazoles 118 (Scheme 38).79 A combination of Cu(I) iodide, elemental sulfur, AgCO3 and TMSCF3 was proposed to generate a CuSCF3 reagent in situ, which can intercept the copper–triazole intermediate formed through the CuAAC reaction. The use of a range of both aryl and alkyl azides and alkynes was demonstrated to provide triazoles 118 in good yields.
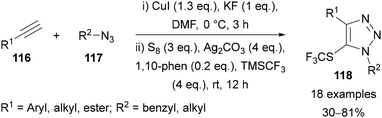 |
| Scheme 38 Copper-mediated synthesis of 5-SCF3-1,2,3-triazoles 118. | |
Xu reported an interrupted CuAAC that allowed for the isolation of stable 5-stannyl-1,2,3-triazoles 119, which could be reacted with a range of electrophiles and applied in cross-coupling reactions.80 The reaction of these 5-stannyl-1,2,3-triazoles 119 with Togni reagent II 120 or N-trifluoromethylthiosaccharin 122 provided access to a range of 5-CF3 and 5-SCF3 triazoles 121 and 122, respectively (Scheme 39).80
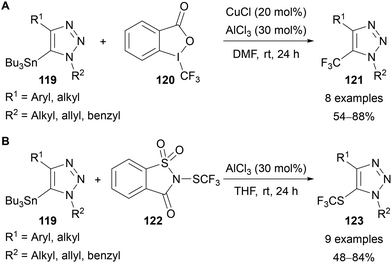 |
| Scheme 39 Electrophilic fluorination of 5-stannyl-triazoles 119 with (A) Togni reagent II 120; (B) N-trifluoromethylthiosaccharin 122. | |
Billard reported an alternative approach to the synthesis of 5-SCF3-triazoles 125 through the direct reaction of NaN3 with a SCF3-substituted alkyne 124 (Scheme 40).81 A one-pot two-step cycloaddition–alkylation process was also demonstrated to give ring-fused triazole 127.
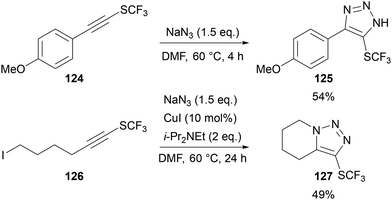 |
| Scheme 40 Synthesis of 5-SCF3-triazoles utilising NaN3. | |
More general access to 5-SCF3-triazoles from SCF3-substituted alkynes has been reported by Guo and Song using ruthenium and rhodium catalysis, respectively (Scheme 41).82 Guo reported the use of both trifluoroethyl- and (trifluoromethyl)thiosubstituted alkynes (128 and 129) for the synthesis of 1,2,3-triazoles bearing a CH2CF3 or SCF3 substituent in the C(5) position (Scheme 41A).82a The majority of examples focused on the use of trifluoroethyl-substituted alkynes 128, with a range of both aryl and alkyl azides and aryl alkynes demonstrated. A significantly lower yield was obtained using an enyne substrate and no examples of alkyl-substituted alkynes were reported. Three examples of 5-SCF3-triazoles 132 were also synthesised under analogous conditions with good yields obtained. The regioselectivity of the 5-CF3CH2-triazoles 131 was confirmed for several products by X-ray crystallography.
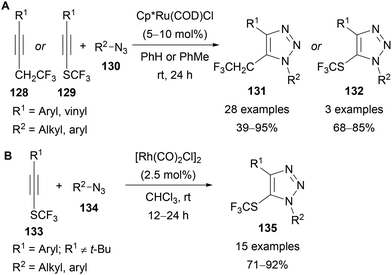 |
| Scheme 41 Preparation of 5-CH2CF3 and 5-SCF3-1,2,3-triazoles. | |
Song reported a complementary rhodium-catalysed AAC reaction of SCF3-substituted alkynes 133, where once again a range of alkyl and aryl azides were utilised, but only aryl-substituted alkynes were demonstrated (Scheme 41B).82b The only alkyl-substituted alkyne attempted (R1 = t-Bu) gave no reaction, however Song also reported an extended scope of thio- and seleno-alkynes bearing alkyl and aryl substituents (i.e. not CF3) where examples of alkynes bearing linear alkyl chains were also successfully demonstrated.
4. Synthesis of N-polyfluoroalkyl- and -aryl-1,2,3-triazoles
4.1. Introduction
Whilst N-fluorinated 1,2,3-triazoles are known, their low stability means few syntheses or applications have been reported.23a The synthesis of N-polyfluoroalkyl- and aryl triazoles is much more common and is usually achieved through transition metal-catalysed AAC reactions (Scheme 42),13,14 however other methods will also be discussed in this section. The main challenge therefore often lies in the synthesis of the polyfluoroalkyl- and aryl azides required for use in these AAC reactions.
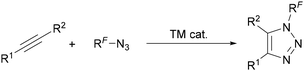 |
| Scheme 42 Common approach to synthesise N-polyfluoroalkyl- and aryl-substituted 1,2,3-triazoles. TM = transition metal. | |
4.2. Synthesis of polyfluoroaryl and -alkyl azides
Polyfluoroaryl azides 136 are readily accessed in several ways,83 including from polyfluoroaryl amines 137 (via the diazonium salt 138),84 boronic acids 139 (through Cu(II)-catalysed Chan Lam coupling)85 and halides 140 (through either SNAr86 or Cu(I)-catalysed Ullmann-type coupling)87 (Scheme 43).
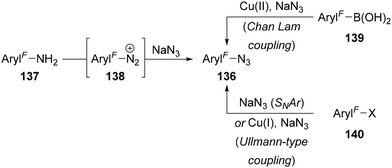 |
| Scheme 43 General procedure for synthesis of polyfluoroaryl (ArylF) azides 136. | |
The synthesis of polyfluoroalkyl azides is significantly more challenging.88 Alkyl azides are most conveniently synthesised by SN2 substitution of a (pseudo)halide leaving group by azide,83,89 however introduction of fluorine substituents on the alkyl chain severely inhibits nucleophilic substitution. Dolbier Jr has studied in detail the effect of fluorine substitution on the nucleophilic substitution of alkyl halides by sodium azide (Scheme 44A).90 Comparing a simple alkyl bromide to γ- and β-fluorinated alkyl bromides revealed a significant deceleration in SN2 rate upon introduction of fluorine close to the reaction centre [relative rates: n-C7H15Br (1); n-RFCH2CH2Br (0.14); n-RFCH2Br (0.00002)]. Whilst introduction of single α-fluorine substituent on an alkyl chain is tolerated [relative rates: n-C7H15Br (1); n-C8H17CHFBr (0.14)], α,α-difluorinated substrates do not undergo substitution in MeOH and only undergo E2 elimination in DMSO. The reduction in the rate of substitution was proposed to originate from two main effects: (1) the inductive electron-withdrawing effect of fluorine destabilising the developing partial positive charge in the SN2 transition state; and (2) electrostatic repulsion of the approaching nucleophile due to the lone pairs/electron density on fluorine.
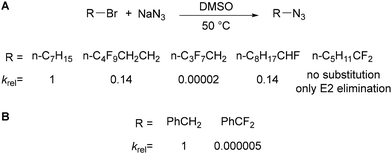 |
| Scheme 44 Kinetic studies on the nucleophilic substitution of fluorinated alkyl bromides by azide. | |
In line with Dolbier Jr's kinetic studies, the synthesis of monofluoromethyl azide 142 is relatively simple to achieve from bromofluoromethane 141 and sodium azide in NMP at room temperature (Scheme 45).91 The azide was obtained in quantitative yields and could be isolated by co-distillation with THF in 82%.
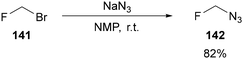 |
| Scheme 45 Synthesis of monofluoromethyl azide 142. | |
The preparation of 2-fluoroethyl azide 144 is also relatively simple through displacement of the respective tosylate 143 with sodium azide (Scheme 46).92 Alternatively these azides can be accessed through displacement of a β-tosylated azide 145 by fluoride. This approach has been widely exploited for the synthesis of 18F-labelled azide [18F]-144 using 18F isotopically enriched KF (Scheme 46).92,93
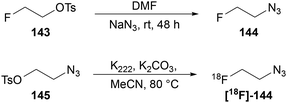 |
| Scheme 46 Synthesis of isotopic 2-fluoroethyl azide. | |
As shown in Dolbier Jr's kinetic studies, the synthesis of β,β-difluorinated alkyl azides is considerably more difficult, with high temperatures and long reaction times often required. Wu and Chen have shown the synthesis of long chain polyfluoroalkyl azides 147 can be synthesised from the reaction of the corresponding alkyl mesylate 146 and sodium azide for 20 h at 110 °C in the presence of 18-crown-6 (Scheme 47A).94 Koroniak-Szejn subsequently investigated the use of both alkyl mesylates and tosylates 148, with the latter providing higher yields (Scheme 47B).95 Shorter reaction times and reaction temperatures of 85 or 120 °C were achieved by using sodium azide in HMPA. Notably, the tosylate of hexafluoroisopropanol (HFIP) was applicable under these conditions to give a secondary alkyl azide. Koroniak-Szejn also reported a smaller range of alkyl iodides 150 were applicable when using sodium azide and Aliquot 336 (phase transfer catalyst) in a water/Et2O mixture in a sealed tube at 90–100 °C (Scheme 47C).95 The yields obtained were generally lower, suggesting the methods from mesylate of tosylate substrates are the best option for the synthesis of these polyfluorinated azides.
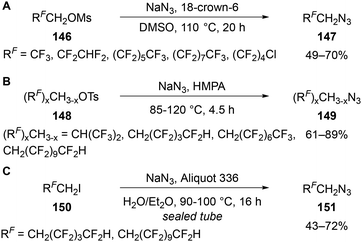 |
| Scheme 47 General procedure for synthesis of 1,1-dihydro-fluroalkyl azides. | |
The only examples of α,α-difluorinated azides that can be synthesised through nucleophilic substitution are those bearing other activating groups, such as benzylic and α-carbonyl substrates (Scheme 48).90,96 In these examples it is possible they may operate through a radical rather than SN2 mechanism. Dolbier Jr reported the kinetics for the substitution of α,α-difluorobenzyl bromide with azide, with the relative rates for PhCH2Br and PhCF2Br measured as 1 and 0.000005, respectively (Scheme 44B).90 As such, high reaction temperatures and times are required for this reaction (Scheme 48A).96a The original synthesis of ethyl 2-azido-2,2-difluoroacetate 155 (R = Et) was first reported at 50 °C in DMSO,96b however it has since been noted that these conditions can lead to an alternative substitution pathway to give ethyl azide and sodium bromodifluoroacetate.96c,f Alternative conditions in DMF or MeCN have also been reported,96g,h without the side-reaction being noted (Scheme 48B). Although yields have been reported, the α-azido ester is often simply used as a crude product in the next step of the synthesis. The use of sodium azide in DMSO at 50 °C has however been successfully used for the synthesis of the tert-butyl ester and amide analogues, where the same dealkylation side-reaction is not possible (Scheme 48B and C).96c,e,f α-Azido-α,α-difluoro amides 157 have also been synthesised by amidation of the corresponding ethyl or methyl ester.96e,97 Finally, two examples of α-azido-α,α-difluoroketones 159 have been reported, in both cases starting from the corresponding α-chloroacetophenone derivative 158 (Scheme 48D).98
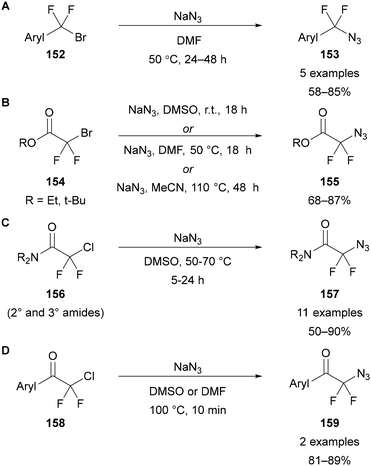 |
| Scheme 48 Preparation of α,α-difluorinated benzylic and α-carbonyl azides. | |
The apparent nucleophilic substitution of vicinal dibromo- and diiodofluoroalkanes (160 and 162) by sodium azide was reported by Knunyants in 1986 (Scheme 49A).99 Based on previous work,100 an ionic chain mechanism was proposed, in which the reaction is initiated by the attack of azide on bromine to form BrN3, elimination of bromide and formation of alkene intermediate 164 (Scheme 49B). Addition of azide to alkene 164 forms anionic intermediate 165, which can abstract another bromine from the substrate to propagate the chain mechanism.101 Evidence for this mechanism was provided by similar yields being achieved through the reaction of one of the proposed alkene intermediates 166 with sodium azide and bromine, giving addition product 167 in 70% yield (Scheme 49C). This provides a general approach to the synthesis of fluorinated alkyl azides 169 from polyfluoroalkenes 168 by the addition of sodium azide in the presence of an electrophile (Scheme 50). This method has been applied using a range of electrophiles, including sodium dihydrogen phosphate (acid),102 ICl,103 aryl thiocyanates,104 carbon dioxide105 and perfluoroalkanoates106 for the synthesis of diversely functionalised polyfluoroalkyl azides (Scheme 50).
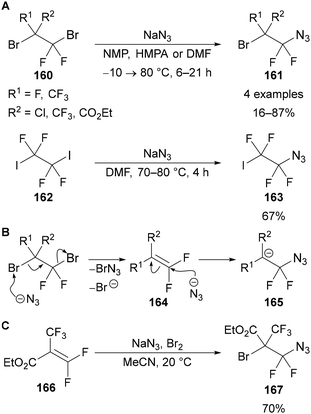 |
| Scheme 49 Synthesis of β-bromo- and β-iodo-fluoroalkylazides through an ionic chain mechanism. | |
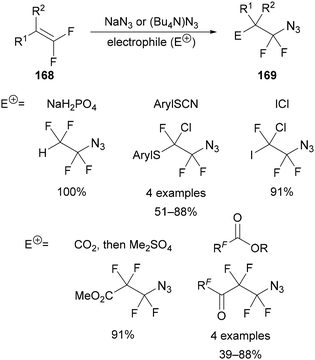 |
| Scheme 50 General approach for the synthesis of β-functionalised fluoroalkylazides. | |
In addition to fluorinated alkenes, difluorocarbene is a suitable electrophile for reaction with sodium azide (Scheme 51). Originally described in a 1973 patent,107 and again in 1985 by Bock,108 base mediated decomposition of chlorodifluoromethane 170 in the presence of sodium azide provides difluoromethyl azide 171 in 54% yield (Scheme 51A). More recently, Beier has shown that difluoromethyl azide 171 can also be accessed in a similar way via quaternary salt Bu3N+(HCF2)Cl− 172 (Scheme 51B).109
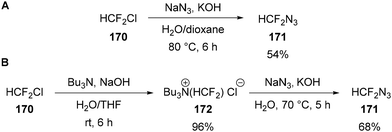 |
| Scheme 51 Methods for the synthesis of difluoromethyl azide 171. | |
Beier has reported a series of relatively simple methods for the synthesis of fluoroalkyl azides 174 through the nucleophilic fluoroalkylation of electrophilic azide sources. Treatment of the commercially available Ruppert–Prakash reagent 173 (RF = CF3) with caesium fluoride in DMF in the presence of tosyl azide provides trifluoromethyl azide 174 (RF = CF3) in high yield (Scheme 52).110 The crude reaction product mixture has been applied in subsequent reactions, or the azide can be co-distilled with THF to produce a THF solution of the fluoroalkylazide product 174. Trifluoromethyl azide is significantly more thermally stable than methyl azide, with explosive decomposition only reported over 330 °C.111 Longer chain perfluoroalkyl azides could be prepared in a similar way, however in some cases the more electrophilic nonaflyl azide (F9C4SO2N3) was required as the azide source (Scheme 52).110,112
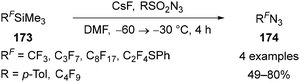 |
| Scheme 52 Synthesis of azidoperfluoroalkanes 174 through nucleophilic fluoroalkylation of sulfonyl azides. | |
As an alternative to using trimethylsilyl-substituted polyfluoroalkanes 173, polyfluoroalkyl azides can also be synthesised by formation of a polyfluoroalkyl carbanion equivalent through deprotonation (Scheme 53A and B),110,113 or magnesium–halogen exchange (Scheme 53C),112 followed by reaction with tosyl or nonaflyl azide.
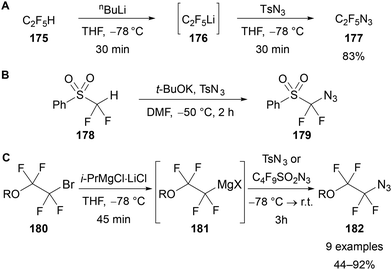 |
| Scheme 53 Synthesis of azidoperfluoroalkanes 177, 179 and 182 through nucleophilic fluoroalkylation of sulfonyl azides. | |
4.3. Synthesis of N-polyfluoroaryl-1,2,3-triazoles
N-Polyfluoroaryl triazoles are most often synthesised through a thermal or transition metal catalysed azide–alkyne cycloadditions (Scheme 54). Whilst thermal methods usually give mixtures of regioisomers, high regioselectivity can be achieved for one regioisomer by using a transition metal catalyst.12–14 When using a terminal alkyne 183, the vast majority of examples in the literature involving polyfluoroaryl azides 184 focus on the copper-catalysed synthesis of the 1,4-regioisomer 185;13 however there are also reports on using silver114 and ruthenium115 catalysts giving 1,4- and 1,5-regioselectivity (185 and 186), respectively (Scheme 54A). The use of internal alkynes 187 provides access to trisubstituted triazoles 188, with examples of polyfluoroaryl azides 184 being used in copper (specifically for iodo- and telluro-alkynes),116 rhodium,117 ruthenium82a,118 and iridium119 catalysed AAC reactions (Scheme 54B).
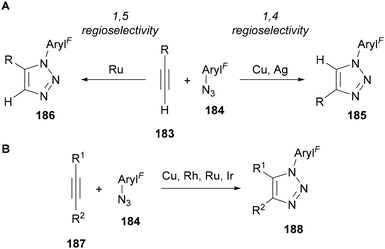 |
| Scheme 54 Transition metal-catalysed azide–alkyne cycloaddition approaches for the synthesis of N-polyfluoroaryl triazoles. | |
As previously outlined, aryl azides can be synthesised from aryl boronic acids and aryl iodides through copper-catalysed Chan Lam and Ullmann-type couplings, respectively (Scheme 43).85,87 The telescoped copper-catalysed synthesis of triazoles through in situ generation of aryl azides from aryl boronic acids 190 or iodides 191 and subsequent CuAAC has therefore been developed,85a,120 with a range of examples including polyfluorinated aryl groups (Scheme 55).121,122 This approach is convenient to avoid the synthesis and isolation of the aryl azide and is particularly suited to library synthesis where a large range of aryl boronic acids and iodides are commercially available.
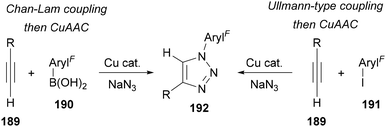 |
| Scheme 55 Telescoped copper-catalysed synthesis of N-polyfluoroaryl triazoles 192 from aryl boronic acids 190 and iodides 191. | |
Polyfluoroaryl azides 194 have also been applied in base- and secondary amine-catalysed synthesis of triazoles from 1,3-diketones, β-keto esters, amides and nitriles 193 (Scheme 56A).73a,74,123 These reactions proceed via an enolate or enamine (in the case of secondary amines) intermediate that reacts with the electrophilic azide, followed by ring closing and elimination of water.15 The electron-withdrawing nature of the polyfluoroaromatic group can therefore have a beneficial effect by increasing the electrophilicity of the azide. The ester-functionalised products 196 are particularly useful as hydrolysis followed by decarboxylation provides access to the less usual 1,5-disubstituted 197 triazole regioisomer (Scheme 56B).
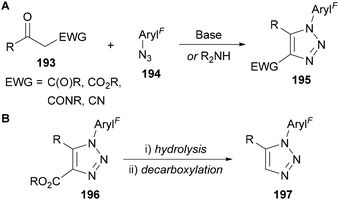 |
| Scheme 56 Base- and amine-catalysed synthesis of triazoles 195 from α-activated ketone derivatives 193. | |
Other α-substituted ketones, where the α-substituent provides an acidifying effect can also be applied in these reactions, such as α-aryl and α-thioalkyl/aryl ketones 198 (Scheme 57A).123h,124 The 4-thio-substituted triazoles 201 can also be converted into 1,5-disubstituted triazoles 202 through desulfurisation using RANEY® nickel (Scheme 57B).124a
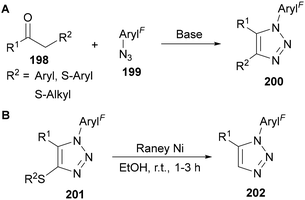 |
| Scheme 57 Base-catalysed synthesis of triazoles 200 from α-substituted ketones 198. | |
Another approach to access 1,5-disubstituted triazoles 206 is through the addition of in situ-generated magnesium acetylides to azides (Scheme 58).125–127 The azide acts as an electrophile in this reaction and therefore the use of azides bearing electron-withdrawing groups (such as polyfluorinated azides 204) accelerate the reaction and provides high yields of triazole product. This is evidenced by the reaction with azides bearing electron-withdrawing groups being complete within an hour at room temperature, whereas azides bearing neutral or electron donating groups required 24–48 h to reach completion. This method also allows for the magnesiated triazole intermediate 205 to be trapped by a range of electrophiles to provide access to 1,4,5-trisubstituted triazoles 207.
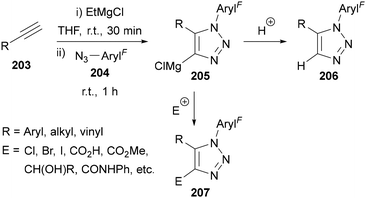 |
| Scheme 58 Synthesis of 1,5-di- and 1,4,5-trisubstituted triazoles 206 and 207 from magnesium acetylides. | |
The magnesiated triazole intermediate 205 is apparently stable at high temperatures for long reaction times, however metalated triazoles of the other triazole regioisomer are known to be unstable when bearing electron-withdrawing N-substituents. 1,4-Disubstituted triazoles bearing N-polyfluoroaryl groups 208 were shown to undergo rapid deprotonation with lithium diisopropylamide (LDA) at −78 °C, with the lithiated intermediate 209 subsequently trapped at −78 °C with a variety of electrophiles (Scheme 59A).128 However if the lithiated intermediate 209 was left for extended times or allowed to warm above −78 °C, rapid decomposition led to over 15 compounds, including amidine 211. Based on related literature on N-phenyl- and N-sulfonyl triazoles,129 it was believed that the electron-withdrawing N-substituent accelerates nitrogen extrusion through stabilisation of the intermediate acetylenamide 212 (Scheme 59B). Protonation, followed by nucleophilic attack by LDA provides the amidine product 211 upon protonation during reaction work-up.
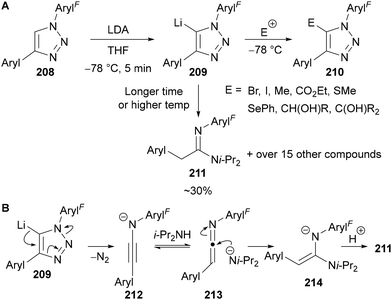 |
| Scheme 59 Formation, trapping and decomposition of lithiated triazoles 209 bearing N-polyfluoroaryl groups. | |
Avoiding the use of azides,16 the cyclocondensation of α-diazoketones and amines provides access to triazoles.130 This approach is sometimes referred to as the Wolff triazole synthesis.131 Typically, an α-electron-withdrawing group and a Lewis or Brønsted acid is required to promote condensation of the amine and ketone. In 2019, Krasavin reported an example of this approach using sulfonyl-substituted α-diazoketones 215 and anilines 216, in which examples using fluorinated aniline derivatives were demonstrated (Scheme 60).132 Presumably fluorinated aniline derivatives could also be applied in other Wolff triazole syntheses,130 however to the best of our knowledge this has not been reported.
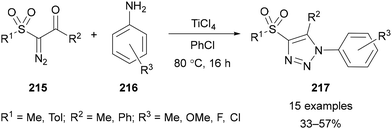 |
| Scheme 60 Triazole synthesis via Wolff cyclocondensation. | |
4.4. Synthesis of N-polyfluoroalkyl-1,2,3-triazoles
Thermal azide–alkyne cycloaddition (AAC) reactions between polyfluoroalkyl azides and terminal and internal alkynes have been reported at 90–140 °C to give N-polyfluoroalkyl triazoles.133 In the case of terminal alkynes, low regioselectivity was generally reported. The most common method to synthesise N-polyfluoroalkyl triazoles is through CuAAC reactions, with nearly all examples in the literature synthesised this way. There is also a single example of a RuAAC reaction between thioalkynes and polyfluoroalkyl azides,134 demonstrating that this metal/fluorinated azide combination is tolerated.
Beier has demonstrated that perfluoroethyl azide reacts twice as fast as ethyl azide with a terminal alkyne in a CuAAC reaction.110 As previously mentioned, short-chain perfluoroalkyl azides can be co-distilled with THF to allow isolation as a THF solution, however Beier also demonstrated that the crude perfluoroalkyl azide can be directly applied in a CuAAC reaction (Scheme 61).110 This method significantly simplifies their application for the synthesis of N-polyfluoroalkyl triazoles 218 and removes any operational concerns about azide distillation; however in some cases the regioselectivity of triazole formation was slightly lower than when using the purified azide.
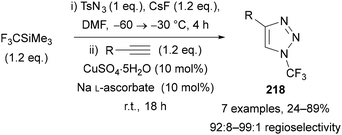 |
| Scheme 61 One-pot synthesis of N-trifluoromethyl triazoles 218. | |
In addition to applications in CuAAC reactions,91,101,102,109,110,112,113,135 Beier has demonstrated the use of polyfluoroalkyl azides 220 in an enamine-catalysed synthesis of triazoles 221 from 1,3-diketones and β-keto esters, sulfones, phosphonates, nitriles and α-nitroketones 219 (Scheme 62A).136 The hydrolysis and decarboxylation of a select number of 4-ester-substituted products 222 was demonstrated to provide access to 1,5-disubstituted triazoles 223 (Scheme 62B). This approach is therefore complementary to the 1,4-disubstituted products obtained through CuAAC reactions.
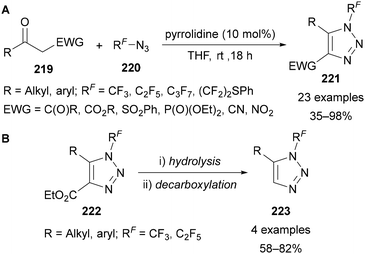 |
| Scheme 62 Preparation of 1,4,5-trisubstituted N-polyfluoroalkyl triazoles 221 and subsequent decarboxylation to access 1,5-trisubstituted N-polyfluoroalkyl triazoles 223. | |
The direct fluoroalkylation of triazoles and benzotriazoles has been reported through a range of approaches.23b The difluoromethylation of triazole and benzotriazole derivatives has been reported using a range of difluorocarbene precursors.137 Although the alkylation of benzotriazole 224 is regioselective (Scheme 63A), the alkylation of triazole derivatives 226 generally provides a mixture of the 1- and 2-difluoromethylated products 227 and 228 (Scheme 63B).
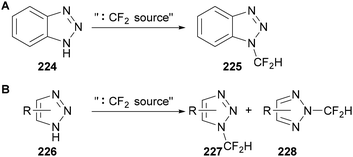 |
| Scheme 63 Difluoromethylation of triazoles using difluorocarbene precursors. | |
N-Trifluoromethylation of a range of azoles has been reported by Togni using Togni reagent I 229.138 Examples of benzotriazole and triazole N-trifluoromethylation was included in the reaction scope (Scheme 64). The in situ N-silylation of the azole substrate was achieved using hexamethyldisilazane (HMDS) and catalytic silica-supported sulfuric acid (SSA) at reflux. Following removal of excess HMDS, the addition of Togni reagent I 229 and sub-stoichiometric HNTf2 and LiNTf2 gave high N(1) regioselectivity for the trifluoromethylation of benzotriazole 224 (Scheme 64A), however when using triazoles 231 a mixture of both the N(1)- and N(2)-trifluoromethylated triazoles 232 and 232 was obtained (Scheme 64B).
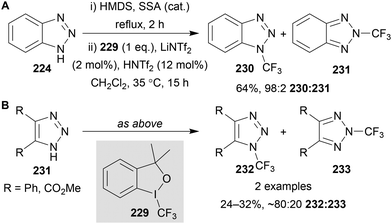 |
| Scheme 64 N-Trifluoromethylation of triazoles using Togni reagent I 229. | |
Wang has also reported the use of hypervalent iodine-mediated trifluoromethylation for the synthesis of N–CF3 azoles.139 Inspired by earlier work from Togni,140 Wang showed that trifluoromethylation of nitriles 234 could be efficiently achieved using PhICF3Cl 235,141 with the nitrilium intermediates trapped and isolated as DMAP salts 236.139 Wang has subsequently applied this strategy in a range of applications,142 including formal [3 + 2] cycloadditions with diazo compounds 237 to give a range of N–CF3 triazole products 238 (Scheme 65).139
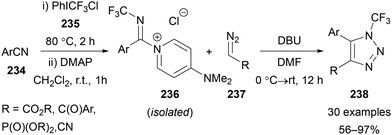 |
| Scheme 65 Synthesis of N-CF3 triazoles 238 from DMAP-stabilised nitrilium salts 236 and diazo compounds 237. | |
5. Applications of C(4) and C(5) fluorinated 1,2,3-triazoles
5.1. Pharmaceutical applications
Two recent patents have been published concerning the use of 5-fluoro-1,2,3-triazoles within drugs for cancer treatment. In 2017, Gilead Sciences prepared compounds such as 239 (Fig. 2)143 to test against the cancer Osaka thyroid (COT) protein, which is involved in the formation of tumour necrosis factor α: a cytokine also involved in inflammatory diseases such as diabetes, sepsis and multiple sclerosis. Interestingly, the triazole C(5) substituent was shown to be important, as changing from a proton to either an iodine or fluorine atom improved the IC50 value by a factor of ∼104. The C(5)-fluorinated triazole was synthesised from the iodine analogue using Fokin's Halex methodology.38
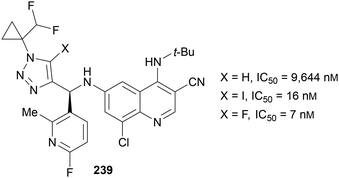 |
| Fig. 2 5-Fluoro-triazole-containing COT treatments. | |
In 2019, Idorsia Pharmaceuticals prepared drug candidates 240 to inhibit two key dioxygenases (Fig. 3),144 which are involved in catalysing the kynurenine pathway causing tryptophan degradation. This pathway is prevalent in various cancers to create an immunosuppressed environment which is key to their growth. In this example, Chu's Halex reaction conditions41 were used to prepare the fluorinated derivative, and whilst the addition of a C(5)-fluorine did not reduce the IC50 value compared to the iodinated or protonated analogues, the value is in the low nanomolar range and thus still represents a good candidate for the inhibition of these enzymes.
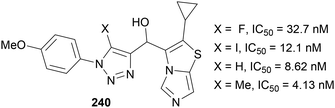 |
| Fig. 3 5-Fluoro-triazole-containing dioxygenase inhibitors. | |
A patent from Dogma therapeutics was released in 2020 using fluorinated-1,2,3-triazoles in compounds targeting proprotein convertase subtilisin/kexin 9 (PCSK9), which is important in cholesterol metabolism.145 Inhibiting PCSK9 is important in treating conditions such as cardiovascular disease and sepsis/septic shock. Triazole containing candidate 241 (Fig. 4) was one of the promising compound scaffolds, giving good KD values for the C(5) protonated and fluorinated analogues. Again, Fokin's Halex reaction38 was used for late-stage fluorination of the iodinated analogue.
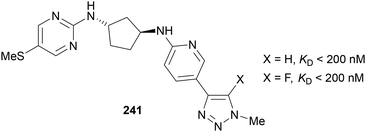 |
| Fig. 4 5-Fluoro-triazole-containing PCSK9 inhibitors. | |
In 2018, Abell reported the use of 5-fluoro-1,2,3-triazoles for the inhibition of biotin protein ligases (BPL) present in Staphylococcus aureus bacteria.146 It was found that the presence of a fluorine atom in inhibitor compound 242 decreased the minimum inhibitory concentration (MIC) drastically to within clinical levels (>100 μM to 16 μM, Fig. 5). Further cell studies determined the likely reason for this was increased uptake of the compound into the cell meaning a higher concentration was present allowing for greater inhibition of BPL across the whole cell. Importantly, this did not affect the cytotoxicity against healthy HepG2 liver cells indicating this compound would be an ideal drug candidate. Abell also demonstrated that N-benzyl-5-fluoro-1,2,3-triazoles 243 were also effective against S. aureus whilst not being cytotoxic to human cells.
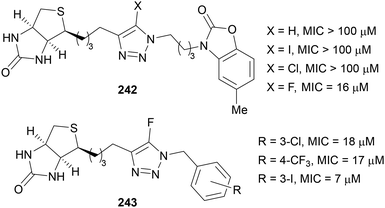 |
| Fig. 5 5-Fluoro-triazole-containing BPL inhibitors. | |
Fluorinated triazole motifs have also been incorporated into anaesthetics by Chengdu MFS Pharma Co. as alternatives to the more well-known imidazole-based Etomidate and cyclopropyl–methoxycarbonate metomidate (CPMM) (Fig. 6).147 In the examples shown below, the ED50 and therapeutic index (TI) values are improved with both C(4)–H and C(4)–F triazole derivates 244. A higher TI value indicates better efficacy of the drug.
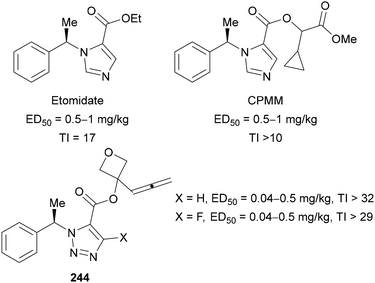 |
| Fig. 6 4-Fluoro-triazole-containing anaesthetics. | |
As well as their use in pharmaceuticals, fluorinated triazoles have also been applied in other medicinal applications. Crousse reported that incorporating 5-fluoro-1,2,3-triazoles into peptides 245 altered their electronic properties (Fig. 7).148 It was shown through X-ray crystallography and a series of calculations that the fluorine atom within model compound 246 could act as a hydrogen bond acceptor with N–H and C–H contacts from nearby amino acid residues. Furthermore, the dipole moment induced by the fluorine caused a negative potential surface on one side of the peptide, resulting in a folded conformation in solution. The properties gained from the presence of fluorine include improved stability, hydrophobicity, and acidity which may be important for biological applications. A patent released in 2023 also showed the use of 5-fluoro-triazoles in polymeric materials for biological implant devices, for use in the slow release of a pharmaceutical agents.149
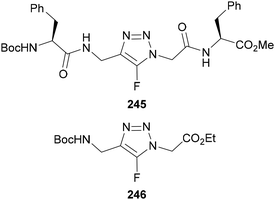 |
| Fig. 7 5-Fluoro-triazoles in peptides. | |
Zhang demonstrated in 2021 a modification of Fokin's Halex conditions38 in the preparation of 18F labelled-1,2,3-triazoles 248 for use in positron emission tomography (PET) imaging.150 The method was developed without the need to dry [18F]-HF, which, in combination with K2CO3 was presumed to form the required [18F]-KF in situ (Scheme 66). A probe targeting thiamine-dependant enzymes 249 was prepared using this method in 10–16% radiochemical yield (RCY).
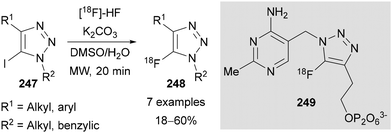 |
| Scheme 66 Radiolabelled synthesis of 5-18F-triazoles and use in thiamine-dependant enzyme inhibitor 249. | |
5.2. Use as synthetic intermediates
Regioselective alkylation of NH-triazoles in the N(2) position is typically difficult to achieve, requiring the use of directing151 or bulky152 groups to form the N(2) alkylated product as the major regioisomer. Nenajdenko demonstrated that a C(4)–fluorine substituent allows for complete N(2) regioselectivity when using primary and secondary alkyl halides (Scheme 67A).30 X-ray crystallography and DFT showed the 2H tautomer of 251 is energetically favoured the over the 1H and 3H tautomers.30 In the case of methyl iodide, ∼80% selectivity for the 2-methylated product was obtained, with small amounts of the 1- and 3-methylated products also observed. N(2)-Selective functionalisation, including arylation, acylation, tosylation, hydroxymethylation and Michael addition reactions were used to demonstrate the versatility of this method (Scheme 67B).
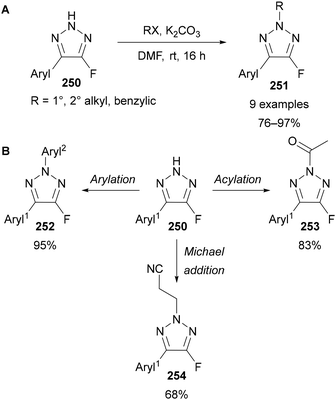 |
| Scheme 67 Selected examples of N(2) substitutions of 4-fluoro-triazoles 250. (A) Alkylations; (B) arylation: ArylB(OH)2, Cu(OAc)2 (10 mol%), O2, DMSO, 100 °C, 2 h; or (SNAr) aryl–F, K2CO3, DMSO, 70 °C, 1 h; acylation: Ac2O, Et3N, CH2Cl2, rt, 3 h; Michael addition: CH2 CHCN, Et3N, DMF, rt, 20 h.30 | |
Nenajdenko noted that the 2,5-diaryl-4-fluoro-triazoles possessed fluorescence properties, with their synthesis and application further expanded by Nenajdenko and Tabolin in 2018 (Scheme 68A).153 All triazoles 256 were found to absorb light in the near-middle UV range with maxima at 300–325 nm. Emission was recorded in near-UV to violet/blue range, demonstrating the compounds to be blue light emitting fluorophores. Incorporation of a p-methoxybenzene substituent in N(2) position (Aryl2) led to a red shift in the emission spectrum, whilst electron-withdrawing p-cyanobenzene caused a red shift in the absorption and emission spectra. Furthermore, a p-bromobenzene derivative diminished nearly all activity due to the heavy atom effect. When comparing the C(4) fluorinated triazole 258 with proto- (257) other halo-triazoles (259, 260), the fluorinated derivative 258 was shown to most significantly increase the emission maximum (359 nm, Scheme 68B). These fluorinated-triazoles may therefore have applications in medicine or materials.
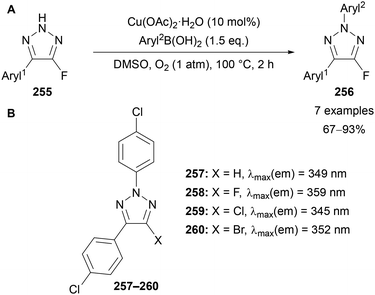 |
| Scheme 68 Synthesis and fluorescence properties of 2,5-diaryl-4-fluoro-triazoles. | |
Following the development of the Halex reaction, Fokin demonstrated the use of 5-fluoro-triazoles 261 as substrates for SNAr reactions (Scheme 69).38 This worked well for N-, O-, and S-based nucleophiles in the presence of sodium hydride. The methodology was also applicable to relatively complex examples including stereocentres with no epimerisation observed during either the Halex reaction or the subsequent SNAr reaction.
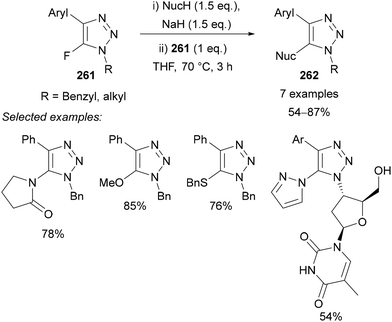 |
| Scheme 69 SNAr of 5-fluoro-triazoles 261 using N-, O-, and S-based nucleophiles. | |
Beer utilised this approach for the synthesis of C(5)-tellurated bis-triazole 264 from the corresponding C(5)-fluoro bis-triazole 263 using in situ formed MeTeLi as a nucleophile (Scheme 70).154 The tellurated bis-triazole compound 264 was used as a heteroditopic receptor for binding sodium halide salts. NMR, X-ray crystallography, and DFT calculations indicated the crown ether substituents bound the sodium ion, whilst the tellurated triazoles acted as Lewis acidic chalcogen bond donors to bind the halide anion.
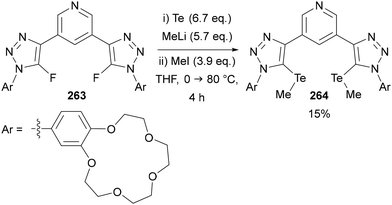 |
| Scheme 70 SNAr of 5-fluoro-triazoles 263 for the synthesis of chalcogen bond donor 5-telluro-triazoles 264. | |
Finally, Ichikawa used 4-fluoro-triazoles 265 and 266 in rhodium catalysed C–H activation-[4 + 2] annulations155 with diarylalkynes 267 to give tricyclic systems 268 and 269 (Scheme 71).34 The triazoles were proposed to act as directing groups for the C–H activation.
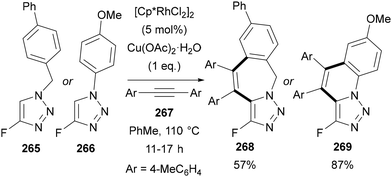 |
| Scheme 71 Rhodium-catalysed C–H activation-[4 + 2] annulation using 4-fluoro-triazoles 265 and 266 and diarylalkynes 267. | |
6. Applications of N(1), C(4) and C(5) polyfluoroalkyl- and polyfluoroaryl-1,2,3-triazoles
6.1. Pharmaceutical applications
In 2011, Chen studied the efficacy of triazole-containing inhibitors 270 of the dipeptidyl peptidase IV enzyme, which is implicated in type 2 diabetes. The introduction of CF3 and CF2H substituents at the C(4) position of the triazole was shown to significantly increase the potency of the inhibitor in comparison to C(4)–H and C(4)–Me analogues (Fig. 8).156 It was assumed this effect was due to the electronegativity of the fluorinated groups as other aromatics bearing electron-withdrawing groups also improved the IC50 values to <50 nM. Out of 25 derivatives tested, the C(4)–CF3-susbtituted triazole showed the best pharmacokinetic profile and in vivo efficacy and hence was selected for further studies.
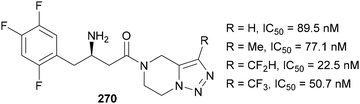 |
| Fig. 8 4-Polyfluoroalkyl-triazole-containing dipeptidyl peptidase IV inhibitors. | |
Also targeting type 2 diabetes, Al-Harrasi reported compounds containing 4-fluorophenyl-triazoles 271 as α-glucosidase inhibitors (Fig. 9).157 The triazoles were installed in the penultimate step of the synthesis via standard CuAAC conditions from 1-ethynyl-4-fluorobenzene and the appropriate azide. The IC50 values show these compounds are all potent inhibitors of this enzyme, outperforming the marketed oligosaccharide-based drug Acarbose (IC50 = 942 μM). Through structure–activity relationship docking studies, it was suggested that the triazole could form a hydrogen bond to an asparagine residue in the active site.
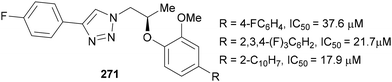 |
| Fig. 9 4-Fluorophenyl-triazole containing α-glucosidase inhibitors. | |
In 2022, Maze therapeutics released a patent targeting the glycogen synthase 1 enzyme with compounds containing fluorinated 1,2,3-triazoles 272 (Fig. 10).158 This enzyme is responsible for the conversion of glucose to glycogen; hence inhibition of this enzyme can be used to treat diseases related to glycogen accumulation. The installation of a CF3 group on the triazole dramatically reduced the IC50 value, with similar trends also observed with other derivatives of this scaffold.
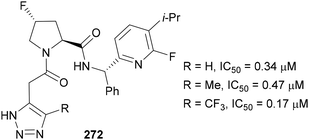 |
| Fig. 10 CF3-substituted triazole-based glycogen synthase 1 inhibitors. | |
Tang and Zheng reported the use of fluorinated-1,2,3-triazoles in a scaffold for use as kinase inhibitors to treat cancer in 2016.159 SAR studies determined that the electronegativity of the fluorinated groups was key in increasing the potency of the inhibitor structure 273 (Fig. 11). Substituting CH3 for CF3 had the largest impact, with a decrease in electron-density within the triazole ring proposed to have a significant effect on inhibitory ability. Based on docking studies, it was also proposed that CF3 group can act as a hydrogen bond acceptor to provide additional hydrogen bonding contacts in the binding site. The addition of an electron-withdrawing group in the R2 position further increasing potency to bring the IC50 value down to 1.68 nM, below that of Foretinib (IC50 = 1.92 nM), a kinase inhibitor that progressed to Phase II clinical trials.160
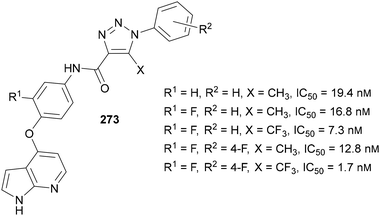 |
| Fig. 11 CF3-substituted triazole-based kinase inhibitors. | |
Wu reported the use of 4-trifluoroacetyl-1,2,3-triazoles as potential anticancer agents in 2018.161 A solvent- and catalyst-free synthesis of these triazoles was reported by the thermal [3 + 2]-cycloaddition of trifluoroacetyl-substituted alkynes 274 and azides 275 (Scheme 72). The 4-trifluoroacetyl regioisomer 276 was favoured over the 5-fluoroacetyl regioisomer 277 in all cases (≥83
:
17 C4
:
C5). These compounds were tested against six cancer cell lines, with some showing comparable or improved activity relative to Erianin, a natural product with anticancer properties. Triazole 278 had the greatest potency against HepG2 cells, with an IC50 value of 0.03 μM.
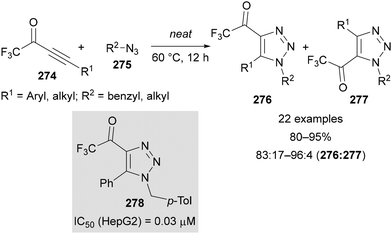 |
| Scheme 72 4-Trifluoroacetyl-triazole anticancer agents. | |
Teixeira reported the ability of fluorinated-triazole compounds to act as anti-fungal agents in 2022,162 for the treatment of papaya crops, which are prone to fungal growth. Derivatives of compound 279 (Fig. 12) were prepared and tested against the Colletotrichum pathogen which is responsible for this fungus. The EC90 values were shown to be between 1 and 5 mg mL−1, with the effect of the fluorinated aryl derivatives having a clear effect in reducing this value. Unfortunately, these were not as effective as eugenol, the fungicide used currently (<0.05 mg mL−1), however, this eugenol is prone to degradation via heat, light and oxygen, hence further modifications on these triazole compounds could provide a solution.
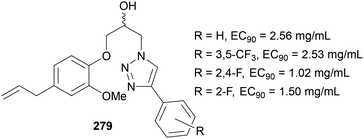 |
| Fig. 12 C(4)-(Poly)fluoroaryl-triazole fungicide candidates. | |
Fluorinated benzylic groups are the most commonly used N-polyfluoroalkyl substituents in medicinal chemistry applications. The phenyl ring is often modified with differing numbers of fluorine or trifluoromethyl groups. A notable example of this is the anticonvulsant Rufinamide 280, where the introduction of one or two fluorines on the N-benzyl substituent was shown to improve activity (Fig. 13).163
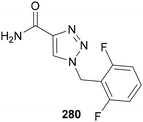 |
| Fig. 13 Structure of Rufinamide 280. | |
Another anticonvulsant bearing the 1,2,3-triazole moiety was reported by Kelley.164 The authors examined fused pyridine-1,2,3-triazoles, where pyridine is fused at the C(4) and C(5) positions of the triazole ring (Fig. 14). The o-fluorobenzyl analogue 281 showed strong anticonvulsant effects but also caused nausea. By modifying the structure to 282, the authors maintained a similar oral administration MES ED50, while eliminating the nausea side-effect.
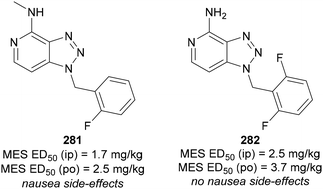 |
| Fig. 14 Anticonvulsants 281 and 282. ip = intraperitoneal injection; po = oral administration. | |
N-Polyfluoroalkyl-1,2,3-triazoles 283 have also been investigated for use as anti-inflammatory drug candidates. Xu studied triazole-based inhibitors of the NLRP3 inflammasome:165 an important target for treating inflammatory diseases.166 Generally however, the addition of substituents on the benzyl group reduced the potency of the drug candidates, demonstrating that adding fluorine substituents to triazoles is not always beneficial to activity (Fig. 15).
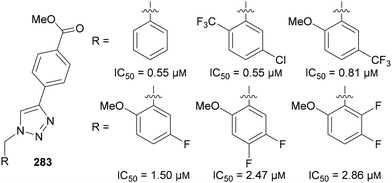 |
| Fig. 15 Fluorinated benzyl derivatives of a NLRP3 inhibitor and their IC50 values. | |
Fluorinated triazoles are often incorporated into the structures of known drugs to produce drug hybrids. For example, 28 triazole hybrids 284 derived from the antiretroviral drug Cabotegravir were recently explored by Mao, Li and Wang (Fig. 16).167 Some of the synthesised 1,2,3-triazole hybrids were effective against the human cancer cell lines tested, with fluorinated compounds 286 and 287 reported to be the most active. 287 was active against all tested cell lines, while 286 was strongly active against two cell lines. By comparison, the non-fluorinated analogue 285, in addition to the other 25 derivatives tested, displayed significantly lower activity.
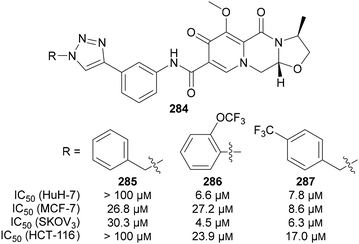 |
| Fig. 16 IC50 values for activity of 1,2,3-triazole-cabotegravir hybrids 284 against several cancer cell lines. HuH-7 = human hepatocellular carcinoma; MCF-7 = human breast cancer; SKOV3 = human ovarian cancer; HCT-116 = human colon cancer. | |
Fluorinated 1,2,3-triazole hybrids have also been investigated as anticancer drug candidates by Liu, who reported a series of novel 1,2,3-triazole-containing dithiocarbamates 289. These were evaluated against human esophageal, cervical, prostate, lung and breast cancer cell lines.168 Fluorinated triazole-containing dithiocarbamates 289 compared favourably to dithiocarbamate 288 hybrids reported previously by Liu (Fig. 17).169
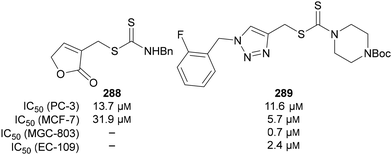 |
| Fig. 17 IC50 values for activity of fluorinated 1,2,3-triazole dithiocarbamate 289, and previously reported dithiocarbamate hydrid 288, against several cancer cell lines. | |
In 1988, Kadaba reported an early example of N-polyfluorophenyl 1,2,3-triazoles in medicinal chemistry.170 The authors described promising anti-convulsant properties of aryl- and pyridyl-functionalised triazolines and 1,2,3-triazoles. Of the triazoles tested, chlorinated and fluorinated derivatives showed promising activity in combination with relatively high toxic doses compared to the non-halogenated analogues.
The antibacterial activity of 1,2,3-triazoles-functionalised tetrahydroisoquinolinium-salts 290 has been reported by Ung.85d Screening a range of N-aryl triazoles revealed that the incorporation of hydrophobic groups resulted in increased activity against both S. aureus and B. subtilis (Fig. 18). p-tert-Butyl substitution of the aryl ring resulted in a large increase in activity, and whilst substitution with CF3 groups resulted in a smaller increase in activity, the combination of a p-OBn-substituent and either an o- or m-fluorine resulted in the same effect as tert-butyl substitution. This was suggested to reflect the combination of hydrophobicity of the OBn group, as well as synergistic electron donation from OBn and withdrawal by fluorine.
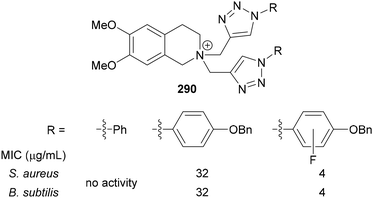 |
| Fig. 18 Select examples of 1,2,3-triazoles-functionalised tetrahydroisoquinolinium-salts 290 investigated for antibacterial activity. | |
6.2. Use as synthetic intermediates
The α-fluorosulfone-substituted triazoles 291 synthesised by Zajc (section 2.3, Scheme 25),64 were used as Julia–Kocienski olefination reagents for the stereoselective formation of fluoro-alkenes 293.64 By varying the reaction conditions, formation of either the E- or Z-fluoro-alkene could be favoured (Scheme 73).64b Both aliphatic and aryl aldehydes 292 bearing electron-withdrawing or -donating substituents were successfully applied, with higher yields and stereoselectivities typically achieved for the synthesis of the Z-alkene products (Z)-293. Five examples involving symmetric and unsymmetric ketones were also demonstrated for the synthesis of tetra-substituted alkenes.64b
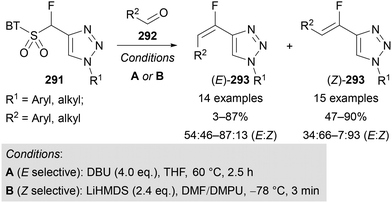 |
| Scheme 73 Use of α-fluorosulfone-substituted triazoles 291 as Julia–Kocienski olefination reagents. BT = benzo[d]thiazole. | |
The applicability of 4-sulfonyl fluoride substituted triazoles 294 in SuFEx click reactions was demonstrated by Fokin and Moses (Scheme 74).65 Reaction with phenolates 295 or amines 297 provided access to sulfonates 296 (Scheme 74A) and sulfonamides 298 (Scheme 74B) in moderate to high yields, including biologically relevant examples, including amino acids, steroids and N-heterocycles. Furthermore, base hydrolysis of 299 provided sodium sulfonate 300 in high yield (Scheme 74C). The direct sulfonation of triazoles is not possible using standard sulfonation conditions, thus this route provides a useful alternative approach to access these potentially useful compounds.
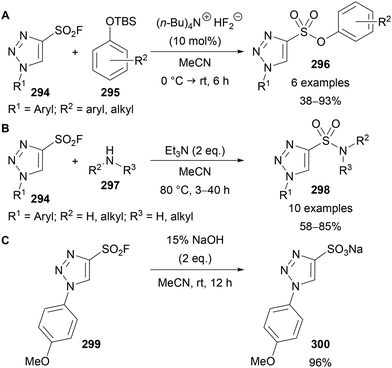 |
| Scheme 74 Example SuFEx applications of 4-sulfonyl fluoride substituted triazoles. | |
N-Polyfluoroalkyl-1,2,3-triazoles have also been used as synthetic intermediates and reagents for a range of transformations. For example, in 1997, Katritzky reported the use of (trifluoroacetyl)benzo-1,2,3-triazole 302 to trifluoroacetylate amines 301 and alcohols 304 in good to excellent yields (Scheme 75).171 Previously reported reagents for trifluoroacetylation were unstable or produced difficult to remove byproducts, however this reagent was both simple to prepare (from benzotriazole and triflic anhydride), bench-stable and convenient to use. Katritzky extended this methodology for the polyfluoroacetylation of primary and secondary amines by using polyfluoroacetylated benzo-1,2,3-triazoles.172
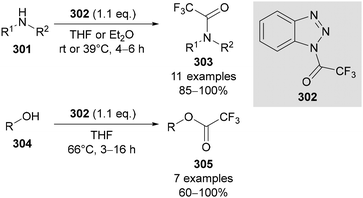 |
| Scheme 75 Trifluoroacetylation of amines and alcohols. | |
More recently, Beier has reported a range of synthetic applications of N-polyfluoroalkyl triazoles 306,23c which all take advantage of the electron-withdrawing nature of the N-polyfluoroalkyl group. Taking inspiration from the formation of rhodium–carbenoids from N-sulfonyl-triazoles with concomitant extrusion of N2,173 Beier has demonstrated analogous reactivity can be achieved from N-polyfluoroalkyl-substituted triazoles 306 (Scheme 76). Heating the triazole substrates 306 with a Rh(II) catalyst provides access to the key Rh–carbenoid intermediate 307, which has been exploited for the synthesis of imidazoles, pyrroles, azepines, oxazoles and indoles (Scheme 76).101,174
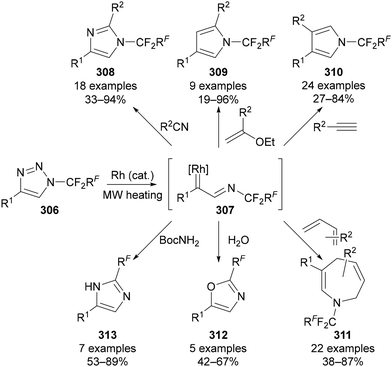 |
| Scheme 76 Synthetic use of the Rh-carbenoid intermediates generated from N-polyfluoroalkyl triazoles. | |
The reaction of N-polyfluoroalkylated triazoles 314 with both Brønsted and Lewis acids has also been investigated (Scheme 77). In these reactions, loss of N2 was proposed to generate a vinyl cation intermediate 315, which, depending on the identity of the Brønsted or Lewis acid could, following hydrolysis, result in the formation of (Z)-vinyl triflate enamides 316,101,175 β-(Z)-enamido fluorides 317,176 and β-(Z) imidoyl chlorides, bromides and iodides 318 (Scheme 77).135b Beier also demonstrated the use of these products in subsequent transformations, such as cross-coupling and displacement reactions.
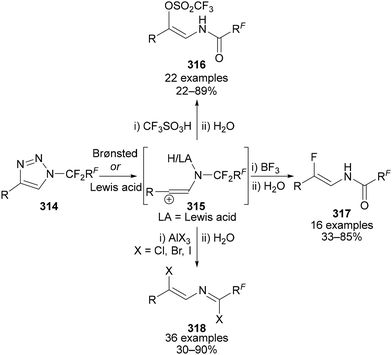 |
| Scheme 77 Treatment of N-polyfluoroalkyl-triazoles 314 with Brønsted or Lewis acids followed by hydrolysis leads to β-(Z)-enamido triflates 316, fluorides 217 or β-(Z) imidoyl halides 318. | |
Extending this reactivity, Beier demonstrated that the vinyl cation intermediate could be trapped through an intramolecular alkene insertion when N-polyfluoroalkylated triazoles bearing a C(5) allylic substituent 319 were used (Scheme 78).135a The cyclopentenyl imidoyl halides 320 formed could be further transformed into a range of products, including tetrazoles 321, isoquinolines 322, N-alkenyl amides 323, amidines 324 and cyclopentenones 325 (Scheme 78).
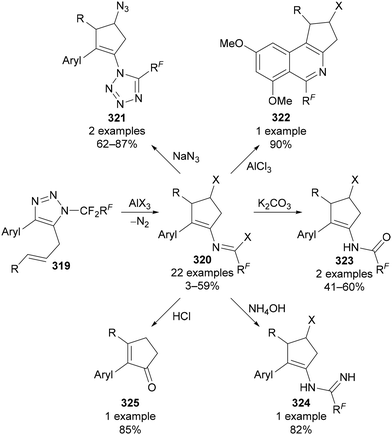 |
| Scheme 78 Formation and synthetic versatility of cyclopentenyl imidoyl halides 320. | |
Beier has also reported the formation of ketenimine intermediates 327 from the thermal aza-Wolff rearrangement of N-polyfluoroalkyl-triazoles 326 (Scheme 79).177 Treatment of the ketenimine intermediate 327 with oxygen-, sulfur- and nitrogen-based nucleophiles provides access to N-polyfluoroalkylated imidates, thioimidates and amidines 328, whilst treatment with alkenes or alkynes results in iminocyclobutanes and -butenes 329, respectively.
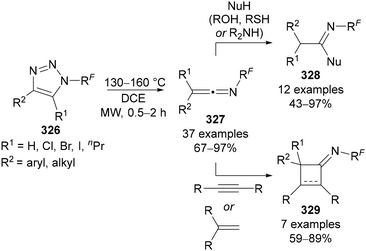 |
| Scheme 79 Formation and synthetic applications of ketenimine intermediate 327 from the aza-Wolff rearrangement of N-polyfluoroalkyl-triazoles 326. | |
N-Fluoroalkyl-triazoles have been applied for the formation of foldamers (Scheme 80). The conformation of peptides is essential to their biological function, and whilst peptides can be highly active pharmaceuticals their poor in vivo stability can be an obstacle to their application. The introduction of triazoles into peptides increases their stability, and the fluorinated triazole–peptide hybrid 330 was reported by Crousse in 2017 (Scheme 80).96e DFT studies of the peptide indicated the presence of hydrogen bonding between the amide and fluorine as well as non-classical hydrogen bonding from the methylene group to fluorine. The authors concluded that these interactions involving the CF2 group could help the structure adopt a single, rigid conformation. Ongeri reported the synthesis of triazole–peptide hybrid 331 with stereogenic CF3 substituents on the backbone (Scheme 80).96h These two triazole–peptide hybrids were found to adopt unique conformations, with the more rigid 330 forming long β-strands, whilst 331 formed short multistrand β-sheets.96h Olsen has also reported a selection of triazole–peptide dipeptide mimics that utilise an N–CF2 functionalised triazole 332. The authors synthesised Fmoc- and Boc-protected dipeptide mimics, which were used for the synthesis of Leu-enkephalin analogues (Scheme 80).96f
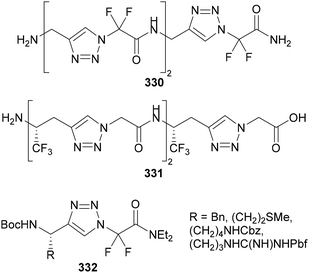 |
| Scheme 80 Fluorinated triazole–peptide hybrid foldamers. Pbf = 2,2,4,6,7-pentamethyldihydrobenzofuran-5-sulfonyl. | |
6.3. Materials applications
Shreeve has reported the use of 4-CF3 and SF5-triazoles 335 as energetic materials,178 where the fluorinated group is proposed to impart increased thermal stability, chemical resistance, electronegativity, and hydrophobicity (Scheme 81). The preparation of these triazoles is procedurally challenging due to the use of gaseous, relatively unstable acetylene substrates 334, which must be condensed into the reaction mixture at −195 °C. A range of triazole products 335 were obtained however in good yields and as single regioisomers. These compounds were tested for their density, detonation pressure (P), and detonation velocity (D) which were found to be comparable to TNT. Furthermore, the SF5-contained triazoles were found to have higher densities and more favourable detonation properties relative to the CF3 analogues. Due to these detonation properties, extreme caution should be taken if preparing these triazoles.
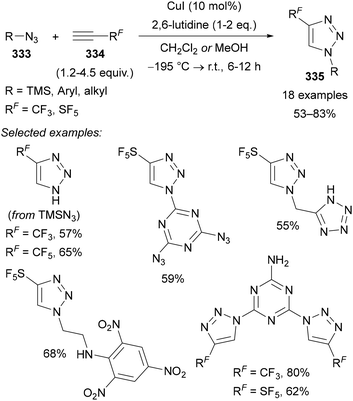 |
| Scheme 81 Synthesis and application of 4-CF3 and SF5-triazoles 335 as energetic materials. | |
6.4. Anion binding and catalysis
N-Polyfluoroaryl 1,2,3-triazoles have seen considerable application within anion binding and catalysis due to the numerous non-covalent interactions these motifs can engage in. For example, the triazole and N-aryl substituents can engage in π-π stacking; the triazole proton can act as a hydrogen bond donor (where this ability may be enhanced by the electron withdrawing nature of the N-polyfluorophenyl group); and the fluorines on the N-polyfluorophenyl group can act as hydrogen bond acceptors.
Several groups have reported the use of polyfluorinated N-aryl triazoles within anion binding, as either anion binding catalysts or as anion transporters. The earliest of these studies was performed by Mancheño where the chloride ion binding ability of a range of bis-triazoles 336a–c was evaluated (Scheme 82A).179
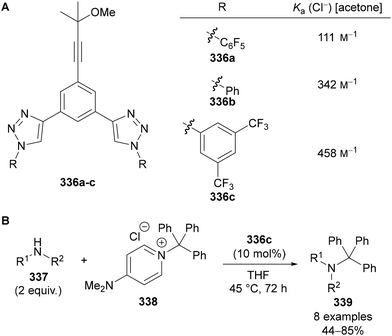 |
| Scheme 82 Mancheño's first generation 1,2,3-triazole chloride anion binding catalyst 336c. | |
The chloride binding association constant for bis-triazole 336c, bearing N-3,5-CF3-phenyl groups, was significantly improved relative to the use of a simple N-phenyl substituent 336b (Ka = 458 M−1 vs. Ka = 342 M−1). In contrast, the use of an N-pentafluorophenyl substituent 336a led to a decrease in chloride binding (Ka = 111 M−1). It was suggested that the stronger chloride binding observed for the bis-CF3-phenyl triazole 336c was due to the ortho-protons present on the bis-CF3-phenyl ring, which provide an additional non-classical hydrogen bond to the chloride anion. DFT studies suggested that the bis-CF3-phenyl ring of 336c sits co-planar with the triazole, thus facilitating the formation of this additional non-classical hydrogen bond. In contrast, the pentafluorophenyl group of 336a sits at an angle due to its inability to engage in any additional non-covalent interactions with the chloride anion. The bis-triazoles synthesised in this work (two additional structures in addition to those in Scheme 82) were applied as catalysts for the tritylation of amines 337 (Scheme 82B). The trend in kinetic performance of the bis-triazole catalysts matched the trend of chloride binding ability, hence demonstrating a useful proof-of-concept study.
Mancheño applied the knowledge from this fundamental study to design chiral analogues, by incorporating a trans-1,2-cyclohexane linkage in place of a phenyl bis CF3 group in order to obtain a chiral tetra-triazole anion binder 340 (Scheme 83A).180 Due to the relatively rigid structure of 340, it is proposed to form a chiral helix and thus was employed as an enantioselective catalyst for anion abstraction reactions through asymmetric counterion-directed catalysis.181 Initially reported for the asymmetric dearomatisation of quinolines 341 (Scheme 83B),180 the catalyst has since been applied for further studies into the dearomatisation of quinolines, isoquinolines, pyridines, diazarenes and pyryliums.182 In addition, investigations into the spatial folding of the catalyst183 and in-depth NMR studies on the effects of changing the anion have been reported.184
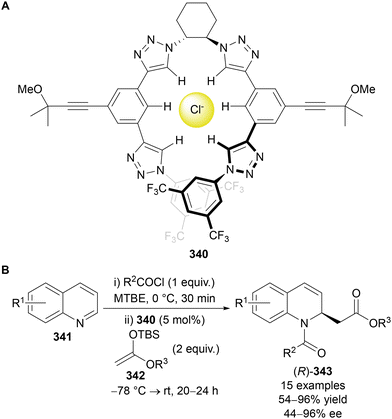 |
| Scheme 83 Mancheño's point, and helically chiral tetratriazole anion binding catalyst 340. | |
Based on Mancheño's work, Feringa developed an asymmetric anion-binding catalyst 344 which incorporated a molecular motor functionality (Scheme 84A).185 The authors described a unidirectional motor which, upon irradiation with light, transitions from a racemic trans-form of the catalyst into a helical pseudochiral cis-form, from which the configuration can be switched by heating. The catalyst was applied for chloride abstraction to promote the addition of a silyl ketene acetal 346 to 1-chloroisochromene 345 (Scheme 84B). The (M,M)- and (P,P)-pseudoenantiomers of the catalyst provided the (R)- and (S)-product 347, respectively, thus stereodivergent access to both enantiomers was achieved with the same catalyst.
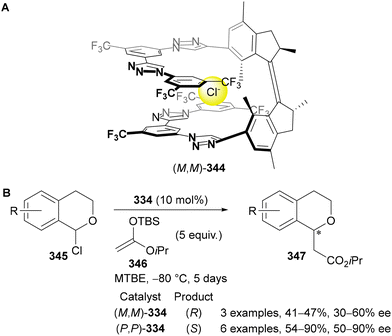 |
| Scheme 84 Molecular motor-based anion binding catalysis. | |
Beyond the use of polyfluoroaryl-substituted triazoles in hydrogen bonding applications, more recent interest has focused on their use in halogen and chalcogen bonding applications. Mancheño showed that modification of their helically-chiral tetra-triazole 340 by substitution of the C(5)–proton of the triazole with iodine created chiral halogen bond donors 348 (Scheme 85A).116c,k Chiral halogen bond donor 348a was found to bind halide and acetate anions more strongly than the hydrogen bond donor analogue 340 (for example, 348a: Ka (Cl−) = 1125 M−1; 340: Ka (Cl−) = 99 M−1). Conversely, lower association constants were observed for the binding between halogen bond donor 348a and bisulphate, hydrogen phosphate and nitrate, relative to the hydrogen bonding donor analogue 340. Whilst no rationale was provided for this change in selectivity, similar observations have previously been reported for multi-dentate triazole-based halogen bond donors.186 Interestingly, the halogen and hydrogen bond donor analogues 340 and 348a displayed opposite selectivities for binding mandelic acid salts, with halogen bond donor 348a binding more strongly with (R)-mandelic acid (Ka(R)/Ka(S) = 1.41) and hydrogen bond donor 340 binding more strongly with (S)-mandelic acid (Ka(R)/Ka(S) = 0.78). This divergence in enantiomeric preference was also observed when halogen bond donor 348a was applied as a catalyst. Using the asymmetric dearomatisation of quinoline as a benchmark reaction, the use of halogen bond donor 348a as a catalyst provided the (S)-enantiomer of product with moderate enantioselectivity (65
:
35 er (R
:
S)) (Scheme 85B). Under analogous conditions, hydrogen bond donor 340 provided the (R)-enantiomer of product with higher enantioinduction (78
:
22 er (S
:
R)). This intriguing switch in enantioselectivity between the seemingly analogous halogen and hydrogen bond donors 340 and 348a was investigated through DFT studies and was suggested to originate from distortion of the helicity of 348a due to the additional steric hindrance imposed by the large iodine groups. Mancheño subsequently demonstrated that through slight modulation of the catalyst and substrate structures, enantioselectivities of up to 76% ee were achieved for the dearomatisation of quinolines 351 (Scheme 85C), and up to 90% ee for the dearomatisation of isoquinolines.116k
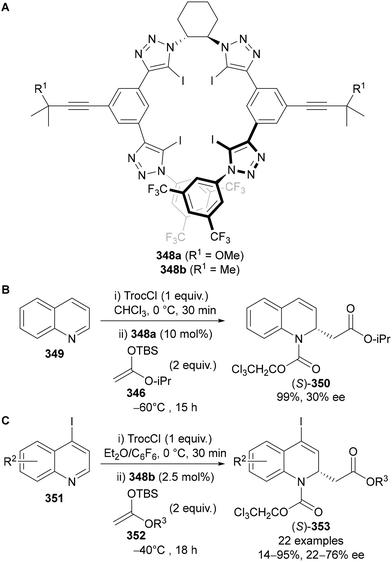 |
| Scheme 85 Helically chiral halogen bond catalyst 348. | |
In 2017, Philp reported the design of bifunctional phosphine oxide–iodotriazole compounds 354, which were shown to undergo halogen bond-induced dimerisation in solution (Scheme 86).116a An N-polyfluorinated substituent was used to enhance the strength of halogen bonding, with a long-chain para-ester substituent required to increase solubility.
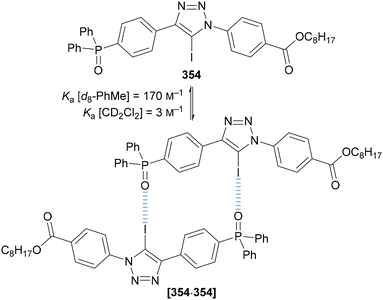 |
| Scheme 86 Halogen bond-induced dimerisation of 354. | |
Beer has reported a series of studies on the use of N-polyfluorophenyl-1,2,3-triazoles for applications in anion binding and transport. As part of a study into the utility of halogen bonding to template [2]rotaxane synthesis, Beer investigated the anion binding ability of a range of pyridinium bis-iodotriazole halogen bond donors 355a–d (Fig. 19).187 Modulating the triazole N-substituent revealed that the polyfluorophenyl derivate 355d provided significantly enhanced anion binding for chloride in particular. Based on this finding, a related cyclodextrin-capped ‘thread’ was synthesised to investigate chloride anion-templated [2]rotaxane synthesis. The importance of chloride anion binding for [2]rotaxane templating was demonstrated with the optimal halogen bond donor providing a 91% yield of a [2]rotaxane, whilst a non-fluorinated analogue gave the [2]rotaxane in only 45%.
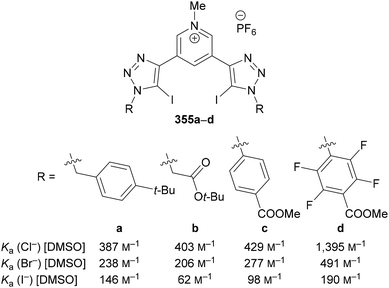 |
| Fig. 19 Anion association constants for pyridinium bis-iodotriazole halogen bond donors 355a–d. | |
Extending this work, Beer reported an in-depth study into the use of neutral halogen and chalcogen bond donors based on a 3,5-bis-triazole pyridine scaffold for anion binding (Fig. 20).116b Systematic variation of the electron-withdrawing nature of the aryl substituents on both the nitrogen of the triazole and the Te substituent of the chalcogen bond donors allowed modulation in the Lewis acidity of the halogen and chalcogen bond donors, which was assessed through halide anion binding. Increasing the degree of fluorination of N-aryl substituents resulted in a systematic increase in the anion binding ability of the chalcogen bond donor 356 (Fig. 20A). Introduction of an N-pentafluorophenyl substituent provided an association constant ∼30 times greater than the analogous chalcogen bond donor bearing a simple N-phenyl substituent (18
500 M−1 vs. 590 M−1). Similarly, modulation of the Lewis acidity of the chalcogen bond donor through the introduction of polyfluorinated aryl groups on Te led to a similar magnitude increase in anion binding for chalcogen bond donor 357 (Fig. 20B). Davis and Beer built on this work to show that the incorporation of a ferrocene substituent on Te provided redox-switchable chalcogen-bond donors, where oxidation to the ferrocenium derivative resulted in three orders of magnitude stronger anion binding.116g
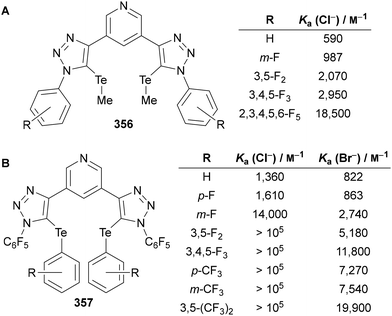 |
| Fig. 20 Binding affinity (Ka) for halide anions with chalcogen bond donors 356 and 357. | |
Beer and Langton applied related neutral bis-triazoles, incorporating the optimal N-pentafluorophenyl substituent for anion binding and transport (Fig. 21).116f N-Polyfluorophenyl-1,2,3-triazoles 358a–c were synthesised with halogen, chalcogen and hydrogen bond donor groups at the C(5) position, and were assessed for their chloride binding affinity and lipid bilayer transport selectivity. The halogen bond donor 358a had the strongest binding affinity toward chloride (>105 M−1), followed by chalcogen bond donor 358b (18
540 M−1), and finally the hydrogen bond donor 358c (1010 M−1). The anion transport capability of anionophores 358a–c was assessed using large unilamellar vesicles. The chloride vs. hydroxide transport selectivity was assessed using an adapted pH-gradient dissipation assay using N-methyl-D-glucamine chloride (NMDG–Cl) as the chloride source and incorporation of proton channel gramicidin D to investigate the relative rates of Cl− and H+/−OH transport.188 Whilst hydrogen bond donor 358c displayed minimal selectivity for chloride (S = 1.6), halogen bond donor 358a showed good selectivity (S = 5) and however chalcogen bond donor 358b showed excellent selectivity (S = 70). Based on the strong anion binding obtained with the neutral N-pentafluorophenyl substituted bis-iodotriazole 358a, Beer subsequently reported the use a related halogen bonding motif for chloride anion templated [2]rotaxane synthesis.116h The [2]rotaxane products exhibited enhanced binding affinities for hydrophilic Cl− and Br− and diminished affinity for hydrophobic I−, relative to the non-interlocked axle counterparts. This binding selectivity opposes Hofmeister bias and was proposed to originate from the effect of the mechanical bond in the [2]rotaxane.
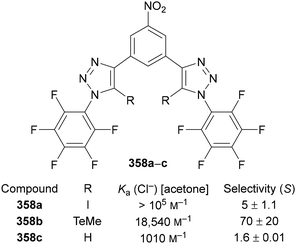 |
| Fig. 21 Chloride anion binding and transport in lipid bilayer vesicles. Selectivity (S) is defined as ECNMDG50/ECGramicidin50. S values >1 indicate chloride transport is faster than H+/−OH transport. | |
Langton built on this work to develop stimuli-responsive anion transporters based on N-pentafluorophenyl substituted bis-iodotriazole halogen bond donors attached to a photo-cleavable linker 359 (Fig. 22A).116j The incorporation of long alkyl chains was used to anchor the halogen bond donor within the lipid bilayer.189 Irradiation with 365 nm light promoted photo-triggered cleavage of the ortho-nitrobenzyl motif to release the mobile ion carrier and switch on the anion-transport ability of the halogen bond donor. In an alternative approach, Langton reported a halogen bonding ion relay in which transport is facilitated by the exchange of ions between lipid-anchored receptors 360 on opposite sides of the membrane (Fig. 22B).116m Once again, an N-pentafluorophenyl substituted bis-iodotriazole halogen bond donor was chosen for this application. The inclusion of polyfluorinated phenyl groups was proposed to serve two purposes: (i) to enhance halogen bond donor ability; and (ii) to make the receptor more lipophilic. In line with earlier work, high chloride vs. hydroxide transport selectivity was observed using the halogen bond donor.
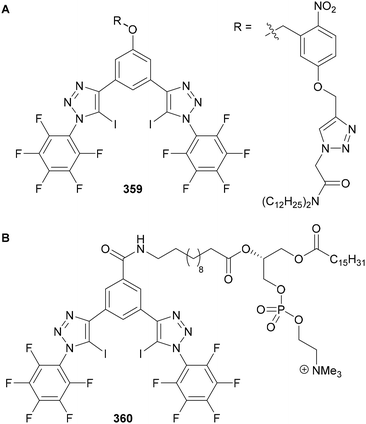 |
| Fig. 22 Lipid bilayer anion transporters based on N-pentafluorophenyl substituted bis-iodotriazole halogen bond donors. | |
Building on the exceptional chloride binding discovered from N-pentafluorophenyl substituted chalcogen bond donors, Beer has demonstrated that incorporation of 15-crown-5 motifs has allowed realisation of a selective KCl receptor 361 (Fig. 23).116i The binding of the potassium cation to the crown ether was proposed to enhance the chalcogen bond donor ability of the tellurium substituents. The chalcogen bond donor 361 was highly selective toward KCl over lighter alkali metal chlorides, however some binding was also observed for RbCl and CsCl. Preliminary studies also demonstrated the ability of 361 to promote extraction of KCl under solid–liquid and liquid–liquid extraction conditions, in addition to selective transmembrane transport of KCl over NaCl. Beer and Félix subsequently reported a related approach to potassium halide binding, using a fullerene-supported heteroditopic ion-pair receptor in which a crown ether and bis-iodotriazole halogen bond donor were spatially separated at opposite sides of the fullerene scaffold.116l In this example, an N-3,5-bis(trifluoromethyl)phenyl substituent was used on the triazole.
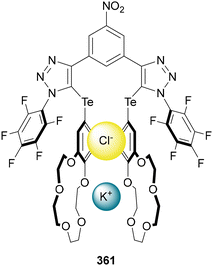 |
| Fig. 23 Chalcogen bond donor KCl receptor 361. | |
Beer and Langton also demonstrated the use of tetraphenylethene-based iodotriazole halogen bond donors for anion sensing and as photoswitchable halogen bond donor anion receptors (Fig. 24).116e Tetrakis-iodotriazole 362 was shown to undergo anion-induced aggregation, driven by halogen bond-mediated anion binding. The nanoscale aggregates displayed luminescent properties, providing a sensory mechanism for different anions. Of the anions tested chloride provided by far the largest response, followed by sulfate, nitrite, bromide, iodide, nitrate and acetate. An N-phenyl analogue of 362 displayed essentially no aggregation or luminescence, highlighting the importance of the polyfluorinated N-aryl substituent for promoting halogen bonding. Bis-iodotriazole 363 was synthesised as both the (E)- and (Z)-isomer, with the (Z)-isomer displaying a significantly higher chloride association constant [(E) = 4970 M−1; (Z) = 23
200 M−1]. When irradiated with 405 nm light, (E)-363 isomerises to give a photostationary state of 48
:
52 (E)
:
(Z). Repeating the photoisomerism in the presence of 10 equivalents of chloride however resulted in a photostationary state of 32
:
68 (E)
:
(Z), indicating that the position of equilibrium is biased by the greater chloride binding ability of (Z)-363.
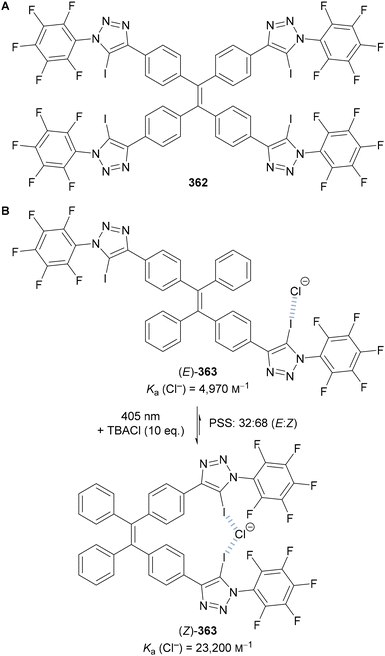 |
| Fig. 24 Tetraphenylethene-based tetrakis-iodotriazole halogen bond donor fluorescent chloride anion sensor 362 (A) and bis-iodotriazole halogen bond donor photoswitch 363 (B). | |
Han and Zhang exploited the controlled (E)/(Z)-photoisomerisation of azobenzene190 to design photoswitchable chalcogen bond donors, containing polyfluoroaryltellurium-functionalised triazoles (Scheme 87).191 Whilst the (E)-isomer (E)-364 displayed a relatively small association constant for chloride binding (807 M−1), isomerisation to (Z)-364 resulted in a significant increase in chloride affinity (3769 M−1; Ka(Z)/Ka(E) ≈ 5) (Scheme 87A). This effect was proposed to originate from the (Z)-isomer's ability to bind chloride in a bidentate fashion as a result of the two chalcogen bond donors being in closer proximity to each other. The cationic analogue 365 (Scheme 87B), provided larger association constants, as would be expected for a charge-assisted chalcogen bond donor,7h,i with the (Z)-isomer (Z)-365 once again providing significantly higher anion binding affinities than the (E)-isomer (E)-365. The neutral and cationic chalcogen bond donors were then applied as stoichiometric activators for chloride abstraction from benzhydryl chloride 367. Only the cationic chalcogen bond donor (E)/(Z)-365 displayed appreciable activity, with (Z)-365 providing significantly better activity than (E)-365 (58% vs. 9% conversion after 24 h), in line with the higher chloride anion affinity of (Z)-365 (Scheme 87B).
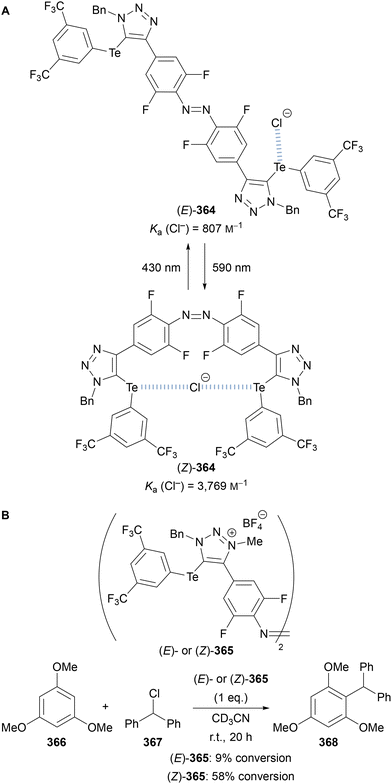 |
| Scheme 87 (E)/(Z) Photo-switchable chalcogen bond donors (A) chloride anion binding affinities for neutral chalcogen bond donors (E)- and (Z)-364; (B) anion abstraction using cationic chalcogen bond donors (E)- and (Z)-365. | |
Langton reported a similar approach for the design of photoswitchable halogen bond donors (Scheme 88).192 In this case, an electron-withdrawing 3,5-bis(trifluoromethyl)aryl group was included at the C(4) position of the triazole to enhance the Lewis acidity, and hence anion binding ability, of the iodine-based halogen bond donor. In line with Han and Zhang's work, the (Z)-isomer (Z)-369 provided a higher chloride anion binding association constant, however in this case the difference in binding for the (E)- and (Z)-isomer was even more pronounced [(E)-369: 590 M−1; (Z)-369: 31
600 M−1; Ka(Z)/Ka(E) ≈ 54] (Scheme 88A), suggesting a more optimal relative arrangement of the two Lewis acidic halogen bond donor sites. Once again, the cationic analogue (E)/(Z)-370 displayed higher chloride anion binding affinity, however in this case the differentiation between (E)- and (Z)-370 was significantly diminished [Ka(Z)/Ka(E) ≈ 3]. Both isomers of the cationic halogen bond donor were effective stoichiometric activators for chloride and bromide anion abstract, with the (Z)-isomer (Z)-370 providing ∼2-fold rate enhancement, relative to the (E)-isomer (Scheme 88B). The cationic halogen bond donor was also applied as a catalyst for a Mukaiyama aldol reaction (0.5 mol% catalyst loading) and the Michael addition of 1-methylindole to an enone (10 mol% catalyst loading). The (Z)-isomer (Z)-370 provided approximately twice as much of the aldol product 373 as the (E)-isomer (E)-370, whilst both isomers provided similar activity for the Michael addition. This last result was proposed to indicate that both isomers operate as mono-dentate halogen bond donors in this case.
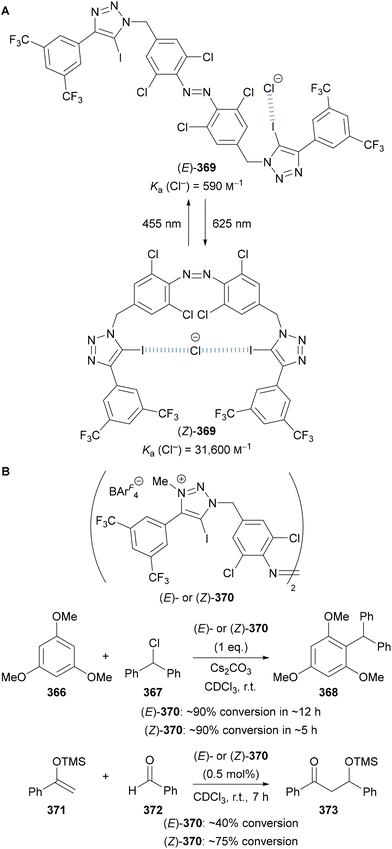 |
| Scheme 88 (E)/(Z) Photo-switchable halogen bond donors (A) chloride anion binding affinities for neutral halogen bond donors (E)- and (Z)-369; (B) anion abstraction and catalysis using cationic halogen bond donors (E)- and (Z)-370. | |
Fukuzawa reported the use of 1,3,4-triaryl-5-iodotriazolium iodides 376 as halogen bond donor catalysts (Scheme 89).193 The triazoliums were prepared via a previously reported method by Bertrand and Grubbs194 from the corresponding triazene and terminal alkyne, followed by iodination of the triazolium ion through deprotonation with t-BuOK and electrophilic trapping with I2. Varying the electronics of the C(4)–aryl substituent of the triazolium catalyst 376 revealed that an electron-withdrawing p-CF3 group provided the highest yield for the cyanosilylation of p-chlorobenzaldehyde 374 over a standard 1 h reaction time (Scheme 89). It was proposed this effect could be attributed to the enhanced Lewis acidity of the halogen bond donor. The halogen bonding catalyst was also applied for the formation of cyclic carbonates from carbon dioxide and epoxides.193
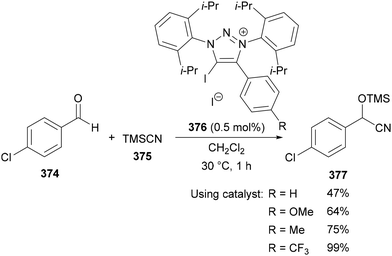 |
| Scheme 89 Effect of different C(4)–aryl substituents within triazole-based halogen bonding catalyst 376 for the cyanosilylation of aldehydes. | |
7. Conclusions
This review has highlighted the diversity of fluorinated 1,2,3-triazoles present in the literature, and has briefly described their synthesis and application.
Methods to access N(1), C(4) and C(5) polyfluoroalkyl- and aryl-substituted 1,2,3-triazoles have been summarised, in addition to methods to access 4- and 5-fluoro-1,2,3-triazoles. Although many of these triazoles can be synthesised through transition metal-catalysed azide–alkyne cycloaddition (AAC) reactions, a wide variety of other transition metal-catalysed, organocatalytic and stoichiometric approaches have been utilised. Generally, polyfluoroaryl-substituted 1,2,3-triazoles are the most straightforward to synthesise due to simple access to polyfluoroaryl-substituted azides, alkynes and other required substrates. Polyfluoroalkyl-substituted azides and alkynes are more challenging to access, however Beier has made significant recent progress in the synthesis of short-chain polyfluoroalkyl azides. In contrast, there are significantly fewer methods to synthesise 4- and 5-fluoro-1,2,3-triazoles. 5-Fluoro-1,2,3-triazoles are generally only synthesised through the so-called Halex reaction from the corresponding 5-iodo triazole;38,41 whilst 4-fluoro-1,2,3-triazoles have recently been synthesised through a small selection of alternative routes. All current approaches have limitations and thus represents an area where more advances could be made.
Fluorinated 1,2,3-triazoles have also found extremely widespread applications in drug discovery, materials chemistry, anion binding and catalysis and as use as synthetic intermediates. In many of these applications the unique structural, chemical and biological properties of both the 1,2,3-triazole and organofluorine group have been exploited.
Overall, this review has showcased the versatility and potential of fluorinated 1,2,3-triazoles in many fields of research. We believe that this summary will assist both academic and industrial practitioners in finding appropriate methods and provide inspiration for future advances in both the synthesis and application of fluorinated 1,2,3-triazoles.
Data availability
As this is a review we have not generated any new data.
Conflicts of interest
There are no conflicts to declare.
Acknowledgements
This research was funded in part by the EPSRC, grant number EP/R513374/1 (FEB), EP/W524645/1 (JLW). We thank GoldenKeys High-Tech Materials Co., Ltd (91520900MA6DL1ER7N) and the University of Warwick for funding (HS). We also thank Mr Songlin Liu from Wengfu Co., Ltd for providing basic industrial knowledge of nitrogen- and fluorine-based bulk chemicals.
References
-
(a) W. Adam and A. Grimison, Sigma-polarization in 5-membered heterocyclic ring systems, Theor. Chim. Acta, 1967, 7, 342–351 CrossRef CAS;
(b) M. Begtrup, C. L. Nielsen, L. Nygaard, S. Samdal, C. E. Sjøgren and G. O. Sørensen, The Molecular Structure and Tautomer Equilibrium of Gaseous 1,2,3-Triazole Studied by Microwave Spectroscopy, Electron Diffraction and Ab Initio Calculations, Acta Chem. Scand., Ser. A, 1988, 42, 500–514 CrossRef;
(c) K. Jug, S. Chiodo, P. Calaminici, A. Avramopoulos and M. G. Papadopoulos, Electronic and Vibrational Polarizabilities and Hyperpolarizabilities of Azoles: A Comparative Study of the Structure-Polarization Relationship, J. Phys. Chem. A, 2003, 107, 4172–4183 CrossRef CAS.
-
(a) P. Thirumurugan, D. Matosiuk and K. Jozwiak, Click Chemistry for Drug Development and Diverse Chemical–Biology Applications, Chem. Rev., 2013, 113, 4905–4979 CrossRef CAS PubMed;
(b) B. Gopalan and K. K. Balasubramanian, in Click Reactions in Organic Synthesis, ed. S. Chandrasekaran, Wiley-VCH, Weinheim, 2016, ch. 2, pp. 25–76 Search PubMed;
(c) E. Bonandi, M. S. Christodoulou, G. Fumagalli, D. Perdicchia, G. Rastelli and D. Passarella, The 1,2,3-triazole ring as a bioisostere in medicinal chemistry, Drug Discovery Today, 2017, 22, 1572–1581 CrossRef CAS PubMed;
(d) K. Bozorov, J. Zhao and H. A. Aisa, 1,2,3-Triazole-containing hybrids as leads in medicinal chemistry: A recent overview, Bioorg. Med. Chem., 2019, 27, 3511–3531 CrossRef CAS PubMed;
(e) A. Rani, G. Singh, A. Singh, U. Maqbool, G. Kaur and J. Singh, CuAAC-ensembled 1,2,3-triazole-linked isosteres as pharmacophores in drug discovery: review, RSC Adv., 2020, 10, 5610–5635 RSC;
(f) K. Lal and P. Yadav, Recent Advancements in 1,4-Disubstituted 1H-1,2,3-Triazoles as Potential Anticancer Agents, Anti-Cancer Agents Med. Chem., 2018, 18, 21–37 CrossRef CAS PubMed;
(g) M. M. Alam, 1,2,3-Triazole hybrids as anticancer agents: A review, Arch. Pharm., 2022, 355, 2100158 CrossRef CAS PubMed;
(h) I. A. Graciano, A. S. de Carvalho, F. de Carvalho da Silva and V. F. Ferreira, 1,2,3-Triazole- and Quinoline-based Hybrids with Potent Antiplasmodial Activity, Med. Chem., 2022, 18, 521–535 CrossRef CAS PubMed;
(i) S. M. A. Rahman, J. S. Bhatti, S. Thareja and V. Monga, Current development of 1,2,3-triazole derived potential antimalarial scaffolds: Structure- activity relationship (SAR) and bioactive compounds, Eur. J. Med. Chem., 2023, 259, 115699 CrossRef PubMed;
(j) N. Poonia, A. Kumar, V. Kumar, M. Yadav and K. Lal, Recent Progress in 1H-1,2,3-triazoles as Potential Antifungal Agents, Curr. Top. Med. Chem., 2021, 21, 2109–2133 CrossRef CAS PubMed;
(k) M. Marzi, M. Farjam, Z. Kazeminejad, A. Shiroudi, A. Kouhpayeh and E. Zarenezhad, A Recent Overview of 1,2,3-Triazole-Containing Hybrids as Novel Antifungal Agents: Focusing on Synthesis, Mechanism of Action, and Structure-Activity Relationship (SAR), J. Chem., 2022, 7884316 CAS.
-
(a) J.-F. Lutz and Z. Zarafshani, Efficient construction of therapeutics, bioconjugates, biomaterials and bioactive surfaces using azide–alkyne “click” chemistry, Adv. Drug Delivery Rev., 2008, 60, 958–970 CrossRef CAS PubMed;
(b) J. C. Jewetta and C. R. Bertozzi, Cu-free click cycloaddition reactions in chemical biology, Chem. Soc. Rev., 2010, 39, 1272–1279 RSC;
(c) E. Kim and H. Koo, Biomedical applications of copper-free click chemistry: in vitro, in vivo, and ex vivo, Chem. Sci., 2019, 10, 7835–7851 RSC;
(d) J. Kaur, M. Saxena and N. Rishi, An Overview of Recent Advances in Biomedical Applications of Click Chemistry, Bioconjugate Chem., 2021, 32, 1455–1471 CrossRef CAS PubMed.
-
(a) V. K. Tiwari, B. B. Mishra, K. B. Mishra, N. Mishra, A. S. Singh and X. Chen, Cu-Catalyzed Click Reaction in Carbohydrate Chemistry, Chem. Rev., 2016, 116, 3086–3240 CrossRef CAS PubMed;
(b) A. K. Agrahari, P. Bose, M. K. Jaiswal, S. Rajkhowa, A. S. Singh, S. Hotha, N. Mishra and V. K. Tiwari, Cu(I)-Catalyzed Click Chemistry in Glycoscience and Their Diverse Applications, Chem. Rev., 2021, 121, 7638–7956 CrossRef CAS PubMed.
-
(a) J. M. Holuba and K. Kirshenbaum, Tricks with clicks: modification of peptidomimetic oligomers via copper-catalyzed azide-alkyne [3 + 2] cycloaddition, Chem. Soc. Rev., 2010, 39, 1325–1337 RSC;
(b) F. A. B. M. Hoogstede and F. P. J. T. Rutjes, in Click Reactions in Organic Synthesis, ed. S. Chandrasekaran, Wiley-VCH, Weinheim, 2016, ch. 5, pp. 141–160 Search PubMed;
(c) N. Agouram, E. M. El Hadrami and A. Bentama, 1,2,3-Triazoles as Biomimetics in Peptide Science, Molecules, 2021, 26, 2937 CrossRef CAS PubMed;
(d) L.-M. Rečnik, W. Kandioller and T. L. Mindt, 1,4-Disubstituted 1,2,3-Triazoles as Amide Bond Surrogates for the Stabilisation of Linear Peptides with Biological Activity, Molecules, 2020, 25, 3576 CrossRef PubMed;
(e) A. Staśkiewicz, P. Ledwoń, P. Rovero, A. M. Papini and R. Latajka, Triazole-Modified Peptidomimetics: An Opportunity for Drug Discovery and Development, Front. Chem., 2021, 9, 674705 CrossRef PubMed.
-
(a) F. Amblard, J. H. Cho and R. F. Schinazi, Cu(I)-Catalyzed Huisgen Azide–Alkyne 1,3-Dipolar Cycloaddition Reaction in Nucleoside, Nucleotide, and Oligonucleotide Chemistry, Chem. Rev., 2009, 109, 4207–4220 CrossRef CAS PubMed;
(b) A. H. El-Sagheer and T. Brown, Click chemistry with DNA, Chem. Soc. Rev., 2010, 39, 1388–1405 RSC;
(c) F. Amblard, O. Sari, S. Boucle, A. Khalil and R. F. Schinazi, in Click Reactions in Organic Synthesis, ed. S. Chandrasekaran, Wiley-VCH, Weinheim, 2016, ch. 10, pp. 309–336 Search PubMed.
-
(a) K. D. Hännia and D. A. Leigh, The application of CuAAC ‘click’ chemistry to catenane and rotaxane synthesis, Chem. Soc. Rev., 2010, 39, 1240–1251 RSC;
(b) Y. Hua and A. H. Flood, Click chemistry generates privileged CH hydrogen-bonding triazoles: the latest addition to anion supramolecular chemistry, Chem. Soc. Rev., 2010, 39, 1262–1271 RSC;
(c) M. Juricek, P. H. J. Kouwer and A. E. Rowan, Triazole: a unique building block for the construction of functional materials, Chem. Commun., 2011, 47, 8740–8749 RSC;
(d) B. Schulze and U. S. Schubert, Beyond click chemistry – supramolecular interactions of 1,2,3-triazoles, Chem. Soc. Rev., 2014, 43, 2522–2571 RSC;
(e) D. Pasini, in Click Reactions in Organic Synthesis, ed. S. Chandrasekaran, Wiley-VCH, Weinheim, 2016, ch. 9, pp. 287–308 Search PubMed;
(f) M. Zurro and O. G. Mancheño, 1,2,3-Triazole-Based Catalysts: From Metal- to Supramolecular Organic Catalysis, Chem. Rec., 2017, 17, 485–498 CrossRef CAS PubMed;
(g) M. S. Shad, P. V. Santhini and W. Dehaen, 1,2,3-Triazolium macrocycles in supramolecular chemistry, Beilstein J. Org. Chem., 2019, 15, 2142–2155 CrossRef CAS PubMed;
(h) M. S. Taylor, Anion recognition based on halogen, chalcogen, pnictogen and tetrel bonding, Coord. Chem. Rev., 2020, 413, 213270 CrossRef CAS;
(i) J. Pancholi and P. D. Beer, Halogen bonding motifs for anion recognition, Coord. Chem. Rev., 2020, 416, 213281 CrossRef CAS;
(j) A. C. Keuper and O. G. Mancheño, in Supramolecular Catalysis: New Directions and Developments, ed. P. W. N. M. van Leeuwen and M. Raynal, Wiley-VCH, Weinheim, 2022, ch. 26, pp. 387–400 Search PubMed;
(k) K. Ohmatsu and T. Ooi, in Anion–Binding Catalysis, ed. O. G. Mancheño, Wiley-VCH, Weinheim, 2022, ch. 7, pp. 221–247 Search PubMed.
-
(a) P. L. Golas and K. Matyjaszewski, Marrying click chemistry with polymerization: expanding the scope of polymeric materials, Chem. Soc. Rev., 2010, 39, 1338–1354 RSC;
(b) M. Arseneault, C. Wafer and J.-F. Morin, Recent Advances in Click Chemistry Applied to Dendrimer Synthesis, Molecules, 2015, 20, 9263–9294 CrossRef CAS PubMed;
(c) J. Mandal and S. Ramakrishnan, in Click Reactions in Organic Synthesis, ed. S. Chandrasekaran, Wiley-VCH, Weinheim, 2016, ch. 7, pp. 203–254 Search PubMed;
(d) X. Wang, X. Zhang and S. Ding, 1,2,3-Triazole-based sequence-defined oligomers and polymers, Polym. Chem., 2021, 12, 2668–2688 RSC;
(e) K. Sykam, S. Donempudi and P. Basak, 1,2,3-Triazole rich polymers for flame retardant application: A review, J. Appl. Polym. Sci., 2022, e52771 CrossRef CAS.
-
(a) Y. Chen, Y. Xianyu, J. Wu, B. Yin and X. Jiang, Click chemistry-mediated nanosensors for biochemical assays, Theranostics, 2016, 6, 969–985 CrossRef CAS PubMed;
(b) F. Ahmed and H. Xiong, Recent developments in 1,2,3-triazole-based chemosensors, Dyes Pigm., 2021, 185, 108905 CrossRef CAS.
- N. M. M. Moura and A. C. Tomé, in Comprehensive Heterocyclic Chemistry IV, ed. D. StC Black, J. Cossy and C. V. Stevens, Elsevier, Amsterdam, 2022, ch. 5.01, vol. 5, pp. 1–77 Search PubMed.
- A. Michael, Ueber die Einwirkung von Diazobenzolimid auf Acetylendicarbonsäuremethylester, J. Prakt. Chem., 1893, 48, 94–95 CrossRef.
-
(a) R. Huisgen, 1,3-Dipolar Cycloadditions. Past and Future, Angew. Chem., Int. Ed. Engl., 1963, 2, 565–598 CrossRef;
(b) R. Huisgen, G. Szeimies and L. Möbius, 1.3-Dipolare Cycloadditionen, XXXII. Kinetik der Additionen organischer Azide an CC-Mehrfachbindungen, Chem. Ber., 1967, 100, 2494–2507 CrossRef CAS;
(c) M. Breugst and H.-U. Reissig, The Huisgen Reaction: Milestones of the 1,3-Dipolar Cycloaddition, Angew. Chem., Int. Ed., 2020, 59, 12293–12307 CrossRef CAS PubMed.
-
(a) C. W. Tornøe, C. Christensen and M. Meldal, Peptidotriazoles on Solid Phase: [1,2,3]-Triazoles by Regiospecific Copper(I)-Catalyzed 1,3-Dipolar Cycloadditions of Terminal Alkynes to Azides, J. Org. Chem., 2002, 67, 3057–3064 CrossRef PubMed;
(b) V. V. Rostovtsev, L. G. Green, V. V. Fokin and B. K. Sharpless, A Stepwise Huisgen Cycloaddition Process: Copper(I)-Catalyzed Regioselective “Ligation” of Azides and Terminal Alkynes, Angew. Chem., Int. Ed., 2002, 41, 2596–2599 CrossRef CAS;
(c) C. W. Tornøe and M. Meldal, Cu-Catalyzed Azide–Alkyne Cycloaddition, Chem. Rev., 2008, 108, 2952–3015 CrossRef PubMed;
(d) J. E. Hein and V. V. Fokin, Copper-catalyzed azide–alkyne cycloaddition (CuAAC) and beyond: new reactivity of copper(I) acetylides, Chem. Soc. Rev., 2010, 39, 1302–1315 RSC;
(e) L. Ackermann and H. K. Potukuchi, Regioselective syntheses of fully-substituted 1,2,3-triazoles: the CuAAC/C–H bond functionalization nexus, Org. Biomol. Chem., 2010, 8, 4503–4513 RSC;
(f) L. Liang and D. Astruc, The copper(I)-catalyzed alkyne-azide cycloaddition (CuAAC) “click” reaction and its applications. An overview, Coord. Chem. Rev., 2011, 255, 2933–2945 CrossRef CAS;
(g) E. Haldón, M. C. Nicasio and P. J. Pérez, Copper-catalysed azide–alkyne cycloadditions (CuAAC): an update, Org. Biomol. Chem., 2015, 13, 9528–9550 RSC;
(h) Z. Chen, Z. Liu, G. Cao, H. Li and H. Ren, Recent Advances in Multicomponent Synthesis of 1,4,5-Trisubstituted 1,2,3-Triazoles, Adv. Synth. Catal., 2017, 359, 202–224 CrossRef CAS.
-
(a) J. R. Johansson, T. Beke-Somfai, A. S. Stålsmeden and N. Kann, Ruthenium-Catalyzed Azide Alkyne Cycloaddition Reaction: Scope, Mechanism, and Applications, Chem. Rev., 2016, 116, 14726–14768 CrossRef CAS PubMed;
(b) C. Wanga, D. Ikhlef, S. Kahlalb, J.-Y. Saillard and D. Astruca, Metal-catalyzed azide-alkyne “click” reactions: Mechanistic overview and recent trends, Coord. Chem. Rev., 2016, 316, 1–20 CrossRef;
(c) R. S. Gomes, G. A. M. Jardim, R. L. de Carvalho, M. H. Araujo and E. N. da Silva Júnior, Beyond copper-catalyzed azide-alkyne 1,3-dipolar cycloaddition: Synthesis and mechanism insights, Tetrahedron, 2019, 75, 3697–3712 CrossRef CAS;
(d) J. Ma and S. Ding, Transition Metal-Catalyzed Cycloaddition of Azides with Internal Alkynes, Asian J. Org. Chem., 2020, 9, 1872–1888 CrossRef CAS;
(e) D. P. Vala, R. M. Vala and H. M. Patel, Versatile Synthetic Platform for 1,2,3-Triazole Chemistry, ACS Omega, 2022, 7, 36945–36987 CrossRef CAS PubMed;
(f) F. Ferrara, T. Beke-Somfai and N. Kann, Recent Developments in the Ruthenium-Catalyzed Azide Alkyne Cycloaddition (RuAAC) Reaction, Eur. J. Org. Chem., 2024, e202400113 CrossRef CAS.
-
(a) J. John, J. Thomas and W. Dehaen, Organocatalytic routes toward substituted 1,2,3-triazoles, Chem. Commun., 2015, 51, 10797–10806 RSC;
(b) K. Anebouselvy and D. B. Ramachary, in Click Reactions in Organic Synthesis, ed. S. Chandrasekaran, Wiley-VCH, Weinheim, 2016, ch. 4, pp. 99–140 Search PubMed;
(c) H. B. Jalani, A. Ç. Karagöz and S. B. Tsogoeva, Synthesis of Substituted 1,2,3-Triazoles via Metal-Free Click Cycloaddition Reactions and Alternative Cyclization Methods, Synthesis, 2017, 29–41 CAS;
(d) V. A. Bakulev, T. Beryozkina, J. Thomas and W. Dehaen, The Rich Chemistry Resulting from the 1,3-Dipolar Cycloaddition Reactions of Enamines and Azides, Eur. J. Org. Chem., 2018, 262–294 CrossRef CAS.
- Z. Chen, G. Cao, J. Song and H. Ren, Recent Developments in Azide-Free Synthesis of 1,2,3-Triazoles, Chin. J. Chem., 2017, 35, 1797–1807 CrossRef CAS.
-
(a) K. Uneyama, Organofluorine Chemistry, Blackwell, Oxford, 2006 CrossRef;
(b) P. Kirsch, Modern Fluoroorganic Chemistry Wiley-VCH, Weinheim, 2nd edn, 2013 CrossRef;
(c) A. Harsanyi and G. Sandford, Organofluorine chemistry: applications, sources and sustainability, Green Chem., 2015, 17, 2081–2086 RSC.
- D. O'Hagan, Understanding organofluorine chemistry. An introduction to the C–F bond, Chem. Soc. Rev., 2008, 37, 308–319 RSC.
-
(a) C. Isanbor and D. O'Hagan, Fluorine in medicinal chemistry: A review of anti-cancer agents, J. Fluorine Chem., 2006, 127, 303–319 CrossRef CAS;
(b) S. Purser, P. R. Moore, S. Swallow and V. Gouverneur, Fluorine in medicinal chemistry, Chem. Soc. Rev., 2008, 37, 320–330 RSC;
(c) D. O'Hagan, Fluorine in health care: Organofluorine containing blockbuster drugs, J. Fluorine Chem., 2010, 131, 1071–1081 CrossRef;
(d) V. Gouverneur and K. Müller, Fluorine in Pharmaceutical and Medicinal Chemistry, Imperial College Press, London, 2012 CrossRef;
(e) J. Wang, M. Sánchez-Roselló, J. L. Aceña, C. Del Pozo, A. E. Sorochinsky, S. Fustero, V. A. Soloshonok and H. Liu, Fluorine in Pharmaceutical Industry: Fluorine-Containing Drugs Introduced to the Market in the Last Decade (2001–2011), Chem. Rev., 2014, 114, 2432–2506 CrossRef CAS PubMed;
(f) V. P. Reddy, Organofluorine Compounds in Biology and Medicine, Elsevier, Amsterdam, 2015 Search PubMed;
(g) Y. Zhou, J. Wang, Z. Gu, S. Wang, W. Zhu, J. L. Aceña, V. A. Soloshonok, K. Izawa and H. Liu, Next Generation of Fluorine-Containing Pharmaceuticals, Compounds Currently in Phase II–III Clinical Trials of Major Pharmaceutical Companies: New Structural Trends and Therapeutic Areas, Chem. Rev., 2016, 116, 422–518 CrossRef CAS PubMed;
(h) N. A. Meanwell, Fluorine and Fluorinated Motifs in the Design and Application of Bioisosteres for Drug Design, J. Med. Chem., 2018, 61, 5822–5880 CrossRef CAS PubMed.
-
(a) T. Fujiwara and D. O'Hagan, Successful fluorine-containing herbicide agrochemicals, J. Fluor. Chem., 2014, 167, 16–29 CrossRef CAS;
(b) Y. Ogawa, E. Tokunaga, O. Kobayashi, K. Hirai and N. Shibata, Current Contributions of Organofluorine Compounds to the Agrochemical Industry, iScience, 2020, 23, 101467 CrossRef CAS PubMed;
(c) Q. Wang, H. Song and Q. Wang, Fluorine-containing agrochemicals in the last decade and approaches for fluorine incorporation, Chin. Chem. Lett., 2022, 33, 626–642 CrossRef CAS.
-
(a) M. G. Dhara and S. Banerjee, Fluorinated high-performance polymers: Poly(arylene ether)s and aromatic polyimides containing trifluoromethyl groups, Prog. Polym. Sci., 2010, 35, 1022–1077 CrossRef CAS;
(b) R. Ragni, A. Punzi, F. Babudri and G. M. Farinola, Organic and Organometallic Fluorinated Materials for Electronics and Optoelectronics: A Survey on Recent Research, Eur. J. Org. Chem., 2018, 3500–3519 CrossRef CAS;
(c) V. F. Cardoso, D. M. Correia, C. Ribeiro, M. M. Fernandes and S. Lanceros-Méndez, Fluorinated Polymers as Smart Materials for Advanced Biomedical Applications, Polymers, 2018, 10, 161 CrossRef PubMed.
- For reviews on C(4/5)-fluorinated triazoles see:
(a) J. L. Arenas and B. Crousse, An Overview of 4- and 5-Halo-1,2,3-triazoles from Cycloaddition Reactions, Eur. J. Org. Chem., 2021, 2665–2679 CrossRef CAS;
(b) N. Pokhodylo, K. Levchenko and M. Obushak, Fluorinated 1,2,3-Triazoles: Terra Incognita in 1,2,3-Triazoles Chemistry, ChemistrySelect, 2024, 9, e202302753 CrossRef CAS;
(c) D. Coelho, Y. Colas, M. Etheve-Quelquejeu, E. Braud and L. Iannazzo, Halo-1,2,3-triazoles: Valuable Compounds to Access Biologically Relevant Molecules, ChemBioChem, 2024, 25, e202400150 CrossRef CAS PubMed.
- For reviews on polyfluoroalkyl-substituted triazoles see:
(a) V. A. Ostrovskii and R. E. Trifonov, in Fluorine in Heterocyclic Chemistry, ed. V. Nenajdenko, Springer, Cham, 2014, vol. 1, pp. 459–513 Search PubMed;
(b) B. I. Usachev, Chemistry of fluoroalkyl-substituted 1,2,3-triazoles, J. Fluor. Chem., 2018, 210, 6–45 CrossRef CAS;
(c) A. Markos, V. Matoušek and P. Beier, Fluoroalkyl Azides and Triazoles: Unlocking a Novel Chemical Space, Aldrichimica Acta, 2022, 55, 37–44 CAS.
- For medicinal chemistry applications see: I. Ullah, M. Ilyas, M. Omer, M. Alamzeb, Adnan and M. Sohail, Fluorinated triazoles as privileged potential candidates in drug development—focusing on their biological and pharmaceutical properties, Front. Chem., 2022, 10, 926723 CrossRef CAS PubMed.
- Z. K. Yao and Z. X. Yu, Mechanisms of the Thermal Cyclotrimerizations of Fluoro- and Chloroacetylenes: Density Functional Theory Investigation and Intermediate Trapping Experiments, J. Am. Chem. Soc., 2011, 133, 10864–10877 CrossRef CAS PubMed.
- W. J. Middleton and W. H. Sharkey, Fluoroacetylene, J. Am. Chem. Soc., 1959, 81, 803–804 CrossRef CAS.
- R. Sauvêtre and J. F. Normant, Une nouvelle preparation du fluoroacetylene - sa reaction avec les organometalliques. Synthese d'alcynes et d'enynes divers, Tetrahedron Lett., 1982, 23, 4325–4328 CrossRef.
- R. E. Banks, M. G. Barlow, W. D. Davies, R. N. Haszeldine and D. R. Taylor, Polyhalogenoallenes. Part VIII. Routes to tetrafluoroallene and tetra-fluoropropyne, J. Chem. Soc. C, 1969, 1104–1107 RSC.
-
(a) F. Reck, F. Zhou, M. Girardot, G. Kern, C. J. Eyermann, N. J. Hales, R. R. Ramsay and M. B. Gravestock, Identification of 4-Substituted 1,2,3-Triazoles as Novel Oxazolidinone Antibacterial Agents with Reduced Activity against Monoamine Oxidase A, J. Med. Chem., 2005, 48, 499–506 CrossRef CAS PubMed;
(b) S. Jana, S. Adhikari, M. R. Cox and S. Roy, Regioselective synthesis of 4-fluoro-1,5-disubstituted-1,2,3-triazoles from synthetic surrogates of α-fluoroalkynes, Chem. Commun., 2020, 56, 1871–1874 RSC;
(c) V. A. Motornov, A. A. Tabolin, R. A. Novikov, Y. V. Nelyubina, S. L. Ioffe, I. V. Smolyar and V. G. Nenajdenko, Synthesis and Regioselective N-2 Functionalization of 4-Fluoro-5-aryl-1,2,3-NH-triazoles, Eur. J. Org. Chem., 2017, 6851–6860 CrossRef CAS.
- V. A. Motornov, V. M. Muzalevskiy, A. A. Tabolin, R. A. Novikov, Yu. V. Nelyubina, V. G. Nenajdenko and S. L. Ioffe, Radical Nitration-Debromination of α-Bromo-α-fluoroalkenes as a Stereoselective Route to Aromatic α-Fluoronitroalkenes-Functionalized Fluorinated Building Blocks for Organic Synthesis, J. Org. Chem., 2017, 82, 5274–5284 CrossRef CAS PubMed.
- S. Opekar, R. Pohl, P. Beran, L. Rulisek and P. Beier, Diethyl Fluoronitromethylphosphonate: Synthesis and Application in Nucleophilic Fluoroalkyl Additions, Chem. – Eur. J., 2014, 20, 1453–1458 CrossRef CAS PubMed.
- T. Fujita, T. Ichitsuka, K. Fuchibe and J. Ichikawa, Facile Synthesis of β,β-Difluorostyrenes via the Negishi Coupling of Thermally Stable 2,2-Difluorovinyl Zinc–TMEDA Complex, Chem. Lett., 2011, 40, 986–988 CrossRef CAS.
- T. Ichitsuka, T. Takanohashi, T. Fujita and J. Ichikawa, A versatile difluorovinylation method: Cross-coupling reactions of the 2,2-difluorovinylzinc–TMEDA complex with alkenyl, alkynyl, allyl, and benzyl halides, J. Fluor. Chem., 2015, 170, 29–37 CrossRef CAS.
- T. Fujita, M. Takeishi and J. Ichikawa, Copper-Catalyzed [3 + 2] Annulation of Azides with a (Difluorovinyl)zinc Complex, Fluoroacetylene Equivalent, Org. Lett., 2020, 22, 9253–9257 CrossRef CAS PubMed.
- B. T. Worrell, J. A. Malik and V. V. Fokin, Direct Evidence of a Dinuclear Copper Intermediate in Cu(I)-Catalyzed Azide-Alkyne Cycloadditions, Science, 2013, 340, 457–460 CrossRef CAS PubMed.
-
(a) D. González-Calderón, A. Fuentes-Benítes, E. Díaz-Torres, C. A. González-González and C. González-Romero, Azide–Enolate 1,3-Dipolar Cycloaddition as an Efficient Approach for the Synthesis of 1,5-Disubstituted 1,2,3-Triazoles from Alkyl/Aryl Azides and β-Ketophosphonates, Eur. J. Org. Chem., 2016, 668–672 CrossRef;
(b) N. T. Pokhodylo, O. Ya. Shyyka, E. A. Goreshnik and M. D. Obushak, 4-Phosphonated or 4-Free 1,2,3-Triazoles: What Controls the Dimroth Reaction of Arylazides with 2-Oxopropylphosphonates?, ChemistrySelect, 2020, 5, 260–264 CrossRef CAS.
- T.-C. Chi, P.-C. Yang, S.-K. Hung, H.-W. Wu, H.-C. Wang, H.-K. Liu, L.-W. Liu and H.-H. Chou, Synthesis of Multisubstituted 1,2,3-Triazoles: Regioselective Formation and Reaction Mechanism, J. Org. Chem., 2024, 89, 5401–5408 CrossRef CAS PubMed.
- B. T. Worrell, J. E. Hein and V. V. Fokin, Halogen Exchange (Halex) Reaction of 5-Iodo-1,2,3-triazoles: Synthesis and Applications of 5-Fluorotriazoles, Angew. Chem., Int. Ed., 2012, 51, 11791–11794 CrossRef CAS PubMed.
- N. Grimster, L. Zhang and V. V. Fokin, Synthesis and Reactivity of Rhodium(II) N-Triflyl Azavinyl Carbenes, J. Am. Chem. Soc., 2010, 132, 2510–2511 CrossRef CAS PubMed.
- J. E. Rowe, K. Lee, D. D. Dolliver and J. E. Johnson, The Preparation of Imidoyl Fluorides, Aust. J. Chem., 1999, 52, 807–812 CrossRef CAS.
- D. Wang, W. Sun and T. Chu, Synthesis of 5-Fluorotriazoles by Silver-Mediated Fluorination of 5-Iodotriazoles, Eur. J. Org. Chem., 2015, 4114–4118 CrossRef CAS.
- T. Furuya, A. E. Strom and T. Ritter, Silver-Mediated Fluorination of Functionalized Aryl Stannanes, J. Am. Chem. Soc., 2009, 131, 1662–1663 CrossRef CAS PubMed.
- W. G. Finnegan and W. P. Norris, Improved Synthesis of 3,3,3-Trifluoropropyne, J. Org. Chem., 1963, 28, 1139–1140 CrossRef CAS.
- W. Carpenter, A. Haymaker and D. W. Moore, Fluorinated 1,2,3-Triazolines, J. Org. Chem., 1966, 31, 789–792 CrossRef CAS.
- B. C. Hamper, α-Acetylenic Esters From α-Acylmethylenephosphoranes: Ethyl 4,4,4-Trifluorotetrolate, Org. Synth., 1992, 70, 246 CrossRef CAS.
-
(a) B. Gold, N. E. Shevchenko, N. Bonus, G. B. Dudley and I. V. Alabugin, Selective Transition State Stabilization via Hyperconjugative and Conjugative Assistance: Stereoelectronic Concept for Copper-Free Click Chemistry, J. Org. Chem., 2012, 77, 75–89 CrossRef CAS PubMed;
(b) J. Wei, J. Chen, J. Xu, L. Cao, H. Deng, W. Sheng, H. Zhang and W. Cao, Scope and regioselectivity of the 1,3-dipolar cycloaddition of azides with methyl 2-perfluoroalkynoates for an easy, metal-free route to perfluoroalkylated 1,2,3-triazoles, J. Fluor. Chem., 2012, 133, 146–154 CrossRef CAS.
- S. Okusu, E. Tokunaga and N. Shibata, Org. Lett., 2015, 17, 3802–3805 CrossRef CAS PubMed.
-
(a) G. K. S. Prakash, P. V. Jog, R. T. D. Batamack and G. A. Olah, Taming of Fluoroform: Direct Nucleophilic Trifluoromethylation of Si, B, S, and C Centers, Science, 2012, 338, 1324–1327 CrossRef CAS PubMed;
(b) S. Barata-Vallejo, M. R. Torviso, B. Lantaño, S. M. Bonesi and A. Postigo, Nucleophilic non-metal assisted trifluoromethylation and perfluoroalkylation reactions of organic substrates, J. Fluor. Chem., 2014, 161, 134–141 CrossRef CAS;
(c) W. C. Fu, P. M. Macqueen and T. F. Jamison, Continuous flow strategies for using fluorinated greenhouse gases in fluoroalkylations, Chem. Soc. Rev., 2021, 50, 7378–7394 RSC.
- G. Meazza and G. Zanardi, Aryl trifluoromethyl-1,2,3-triazoles, J. Fluor. Chem., 1991, 55, 199–206 CrossRef CAS.
- V. V. V. N. S. R. Rao, S. Ravikanth, G. V. Reddy, D. Maitraie, R. Yadla and P. S. Rao, Microwave Assisted Intramolecular Wittig Reaction: A Facile Method for the Synthesis of Conjugated Acetylenes, Synth. Commun., 2003, 33, 1523–1529 CrossRef CAS.
- Y. Zhang and J. Wen, A Convenient Synthesis of Bis(polyfluorophenyl)butadiyne Monomers, Synthesis, 1990, 727–728 CrossRef CAS.
-
(a) Y. Kobayashi, T. Yamashita, K. Takahashi, H. Kuroda and I. Kumadaki, Studies on Organic Fluorine Compounds. XLII. Synthesis and Reactions of Phenyltrifluoromethylacetylenes, Chem. Pharm. Bull., 1984, 32, 4402–4409 CrossRef CAS;
(b) Y. Shen, J. Zheng, Y. Xin, Y. Lin and M. Qi, Synthesis of perfluoroalkylated heterocyclic phosphonates, J. Chem. Soc., Perkin Trans. 1, 1995, 997–999 RSC;
(c) S. I. Coats, J. S. Link, D. Gauthier and D. J. Hlasta, Trimethylsilyl-Directed 1,3-Dipolar Cycloaddition Reactions in the Solid-Phase Synthesis of 1,2,3-Triazoles, Org. Lett., 2015, 7, 1469–1472 CrossRef PubMed;
(d) Z. Xiong, X.-L. Qiu, Y. Huang and F.-L. Qing, Regioselective synthesis of 5-trifluoromethyl-1,2,3-triazole nucleoside analogues via TBS-directed 1,3-dipolar cycloaddition reaction, J. Fluorine Chem., 2011, 132, 166–174 CrossRef CAS.
- S. Wang, L. J. Yang, J. L. Zeng, Y. Zheng and J. A. Ma, Silver-catalyzed [3 + 2] cycloaddition of isocyanides with diazo compounds: new regioselective access to 1,4-disubstituted-1,2,3-triazoles, Org. Chem. Front., 2015, 2, 1468–1474 RSC.
- S. P. Green, K. M. Wheelhouse, A. D. Payne, J. P. Hallett, P. W. Miller and J. A. Bull, Thermal Stability and Explosive Hazard Assessment of Diazo Compounds and Diazo Transfer Reagents, Org. Process Res. Dev., 2020, 24, 67–84 CrossRef CAS PubMed.
- R. Fields and J. P. Tomlinson, Preparation of trifluoromethyl-pyrazoles and -pyrazolines by the reaction of 2,2,2-trifluorodiazoethane with carbon-carbon multiple bonds, J. Fluor. Chem., 1979, 13, 147–158 CrossRef CAS.
- A. Bell and D. A. Edwards, Silver(I) isocyanide complexes: spectroscopic features and reactions with neutral ligands, J. Chem. Soc., Dalton Trans., 1984, 1317–1321 RSC.
- C. Panja, J. V. Puttaramu, T. K. Chandran, R. Y. Nimje, H. Kumar, A. Gupta, P. N. Arunachalam, J. R. Corte and A. Mathur, Methyl-2,2-difluoro-2-(fluorosulfonyl) acetate (MDFA)/copper(I) iodide mediated and tetrabutylammonium iodide promoted trifluoromethylation of 1-aryl-4-iodo-1,2,3-triazoles, J. Fluor. Chem., 2020, 236, 109516 CrossRef CAS.
- Q. Chen and S. Wu, Methyl fluorosulphonyldifluoroacetate;
a new trifluoromethylating agent, J. Chem. Soc., Chem. Commun., 1989, 705–706 RSC.
- S. Eusterwiemann, H. Martinez and W. R. Dolbier Jr, Methyl 2,2-Difluoro-2-(fluorosulfonyl)acetate, a Difluorocarbene Reagent with Reactivity Comparable to That of Trimethylsilyl 2,2-Difluoro-2-(fluorosulfonyl)acetate (TFDA), J. Org. Chem., 2012, 77, 5461–5464 CrossRef CAS PubMed.
- G. Zhao, H. Wu, Z. Xiao, Q. Y. Chen and C. Liu, Trifluoromethylation of haloarenes with a new trifluoro-methylating reagent Cu(O2CCF2SO2F)2, RSC Adv., 2016, 6, 50250–50254 RSC.
- K. Banert, Reaktionen ungesättigter Azide, 8. Azidobutatrien und Azidobutenine, Chem. Ber., 1989, 122, 1175–1178 CrossRef CAS.
- J. R. Alexander, P. V. Kevorkian and J. J. Topczewski, Intercepting the Banert cascade with nucleophilic fluorine: direct access to α-fluorinated N,H-1,2,3-triazoles, Chem. Commun., 2021, 57, 5024–5027 RSC.
- J. R. Alexander, M. H. Packard, A. M. Hildebrandt, A. A. Ott and J. J. Topczewski, Divergent Mechanisms of the Banert Cascade with Propargyl Azides, J. Org. Chem., 2020, 85, 3174–3181 CrossRef CAS PubMed.
-
(a) R. Kumar, P. Pradhan and B. Zajc, Facile synthesis of 4-vinyl- and 4-fluorovinyl-1,2,3-triazoles via bifunctional “click-olefination” reagents, Chem. Commun., 2011, 47, 3891–3893 RSC;
(b) R. Kumar, G. Singh, L. J. Todaro, L. Yang and B. Zajc, E- or Z-Selective synthesis of 4-fluorovinyl-1,2,3-triazoles with fluorinated second-generation Julia–Kocienski reagents, Org. Biomol. Chem., 2015, 13, 1536–1549 RSC.
-
(a) C. J. Smedley, M. C. Giel, A. Molino, A. S. Barrow, D. J. D. Wilson and J. E. Moses, 1-Bromoethene-1-sulfonyl fluoride (BESF) is another good connective hub for SuFEx click chemistry, Chem. Commun., 2018, 54, 6020–6023 RSC;
(b) J. Thomas and V. V. Fokin, Regioselective Synthesis of Fluorosulfonyl 1,2,3-Triazoles from Bromovinylsulfonyl Fluoride, Org. Lett., 2018, 20, 3749–3752 CrossRef CAS PubMed.
-
(a) A. S. Barrow, C. J. Smedley, Q. Zheng, S. Li, J. Dong and J. E. Moses, The growing applications of SuFEx click chemistry, Chem. Soc. Rev., 2019, 48, 4731–4758 RSC;
(b) T. Zhong, Z. Chen, J. Yi, G. Lu and J. Weng, Recent progress in the synthesis of sulfonyl fluorides for SuFEx click chemistry, Chin. Chem. Lett., 2021, 32, 2736–2750 CrossRef CAS;
(c) S. W. Lucas, R. Z. Qin, K. P. Rakesh, K. S. S. Kumar and H.-L. Qin, Chemical and biology of sulfur fluoride exchange (SuFEx) click chemistry for drug discovery, Bioorg. Chem., 2023, 130, 106227 CrossRef PubMed.
- J. Leng and H.-L. Qin, 1-Bromoethene-1-sulfonyl fluoride (1-Br-ESF), a new SuFEx clickable reagent, and its application for regioselective construction of 5-sulfonylfluoro isoxazoles, Chem. Commun., 2018, 54, 4477–4480 RSC.
- C. J. Smedley, G. Li, A. S. Barrow, T. L. Gialelis, M.-C. Giel, A. Ottonello, Y. Cheng, S. Kitamura, D. W. Wolan, K. B. Sharpless and J. E. Moses, Diversity Oriented Clicking (DOC): Divergent Synthesis of SuFExable Pharmacophores from 2-Substituted-Alkynyl-1-Sulfonyl Fluoride (SASF) Hubs, Angew. Chem., Int. Ed., 2020, 59, 12460–12469 CrossRef CAS PubMed.
- D. Fu, J. Zhang and S. Cao, Copper-mediated trifluoromethylation of 5-iodotriazole with (trifluoromethyl)trimethylsilane promoted by silver carbonate, J. Fluor. Chem., 2013, 156, 170–176 CrossRef CAS.
-
(a) I. Ruppert, K. Schlich and W. Volbach, Die ersten CF3-substituierten organyl(chlor)silane, Tetrahedron Lett., 1984, 25, 2195–2198 CrossRef CAS;
(b) G. K. S. Prakash, R. Krishnamurti and G. A. Olah, Synthetic methods and reactions. 141. Fluoride-induced trifluoromethylation of carbonyl compounds with trifluoromethyltrimethylsilane (TMS-CF3). A trifluoromethide equivalent, J. Am. Chem. Soc., 1989, 111, 393–395 CrossRef CAS.
-
(a) K. P. S. Cheung and G. C. Tsui, Copper(I)-Catalyzed Interrupted Click Reaction with TMSCF3: Synthesis of 5-Trifluoromethyl 1,2,3-Triazoles, Org. Lett., 2017, 19, 2881–2884 CrossRef CAS PubMed;
(b) A. Zhu, X. Xing, S. Wang, D. Yuan, G. Zhu, M. Geng, Y. Guo, G. Zhang and L. Li, Multi-component syntheses of diverse 5-fluoroalkyl-1,2,3-triazoles facilitated by air oxidation and copper catalysis, Green Chem., 2019, 21, 3407–3412 RSC;
(c) Z. Fu, X. Yang and G. C. Tsui, Interrupted Click Reaction with CuCF2CF3 Reagent: Synthesis of 5-Pentafluoroethyl 1,2,3-Triazoles, Org. Lett., 2023, 25, 4945–4949 CrossRef CAS PubMed.
-
(a) L. Li, G. Hao, A. Zhu, X. Fan, G. Zhang and L. Zhang, A Copper(I)-Catalyzed Three-Component Domino Process: Assembly of Complex 1,2,3-Triazolyl-5-Phosphonates from Azides, Alkynes, and H-Phosphates, Chem. – Eur. J., 2013, 19, 14403–14406 CrossRef CAS PubMed;
(b) Z. Zhang, Q. Zhou, F. Ye, Y. Xia, G. Wu, M. L. Hossain, Y. Zhang and J. Wang, Copper(I)-Catalyzed Three-Component Coupling of N-Tosylhydrazones, Alkynes and Azides: Synthesis of Trisubstituted 1,2,3-Triazoles, Adv. Synth. Catal., 2015, 357, 2277–2286 CrossRef CAS.
-
(a) W. Peng and S. Zhu, Efficient synthesis of 5-fluoroalkylated 1H-1,2,3-triazoles and application of the bromodifluoromethylated triazole to the synthesis of novel bicyclic gem-difluorinated 1H-pyrano[3,4-d,][1,2,3]-triazol-4-one compounds, Tetrahedron, 2003, 59, 4395–4404 CrossRef CAS;
(b) Y. A. Rozin, J. Leban, W. Dehaen, V. G. Nenajdenko, V. M. Muzalevskiy, O. S. Eltsov and V. A. Bakulev, Regioselective synthesis of 5-trifluoromethyl-1,2,3-triazoles via CF3-directed cyclization of 1-trifluoromethyl-1,3-dicarbonyl compounds with azides, Tetrahedron, 2012, 68, 614–618 CrossRef CAS;
(c) J. Zhang, G. Jin, S. Xiao, J. Wu and S. Cao, Novel synthesis of 1,4,5-trisubstituted 1,2,3-triazoles via a one-pot three-component reaction of boronic acids, azide, and active methylene ketones, Tetrahedron, 2013, 69, 2352–2356 CrossRef CAS.
- L. J. T. Danence, Y. Gao, M. Li, Y. Huang and J. Wang, Organocatalytic Enamide–Azide Cycloaddition Reactions: Regiospecific Synthesis of 1,4,5-Trisubstituted-1,2,3-Triazoles, Chem. – Eur. J., 2011, 17, 3584–3587 CrossRef CAS PubMed.
- R. T. Iminov, A. V. Mashkov, B. A. Chalyk, P. K. Mykhailiuk, A. V. Tverdokhlebov, A. A. Tolmachev, Y. M. Volovenko, O. V. Shishkin and S. V. Shishkina, A Convenient Route to 1-Alkyl-5-trifluoromethyl-1,2,3-triazole-4-carboxylic Acids Employing a Diazo Transfer Reaction, Eur. J. Org. Chem., 2013, 2891–2897 CrossRef CAS.
- R. Augusti and C. Kascheres, Reactions of 3-diazo-1,3-dihydro-2H-indol-2-one derivatives with enaminones. A novel synthesis of 1,2,3-triazoles, J. Org. Chem., 1993, 58, 7079–7083 CrossRef CAS.
- Y. Ning, H. Wang, P. Sivaguru, S. Li, G. Zanoni, S. P. Nolan and X. Bi, Defluorinative [4 + 1] Annulation of Perfluoroalkyl N-Mesylhydrazones with Primary Amines Provides 5-Fluoroalkyl 1,2,3-Triazoles, Green Chem., 2021, 23, 7976–7981 RSC.
- H. Yang, T.-H. Xu, S.-N. Lu, Z. Chen and X.-F. Wu, Synthesis of 5-trifluoromethyl-1,2,3-triazoles via base-mediated cascade annulation of diazo compounds with trifluoroacetimidoyl chlorides, Org. Chem. Front., 2021, 8, 3440–3445 RSC.
- L. L. Zhang, M. T. Li, L. L. Shen and Q. P. Wu, Efficient Synthesis of 5-Trifluoromethylthio-1,2,3-Triazoles: One-Pot Multicomponent Reaction from Elemental Sulfur and TMSCF3, Synthesis, 2020, 304–310 Search PubMed.
- F. Wei, T. Zhou, Y. Ma, C. H. Tung and Z. Xu, Bench-Stable 5-Stannyl Triazoles by a Copper(I)-Catalyzed Interrupted Click Reaction: Bridge to Trifluoromethyltriazoles and Trifluoromethylthiotriazoles, Org. Lett., 2017, 19, 2098–2101 CrossRef CAS PubMed.
- S. Alazet, L. Zimmer and T. Billard, Base-Catalyzed Electrophilic Trifluoromethylthiolation of Terminal Alkynes, Angew. Chem., Int. Ed., 2013, 52, 10814–10817 CrossRef CAS PubMed.
-
(a) Q. Shena, E.-J. Han, Y.-G. Huang, Q.-Y. Chen and Y. Guo, Synthesis of Fluorinated 1,4,5-Substituted 1,2,3-Triazoles by RuAAC- Reaction, Synthesis, 2015, 3936–3946 Search PubMed;
(b) W. Song, N. Zheng, M. Li, J. He, J. Li, K. Dong, K. Ullah and Y. Zheng, Rhodium(I)-Catalyzed Regioselective Azide-internal Alkynyl Trifluoromethyl Sulfide Cycloaddition and Azide-internal Thioalkyne Cycloaddition under Mild Conditions, Adv. Synth. Catal., 2019, 361, 469–475 CrossRef CAS.
- S. Bräse, C. Gil, K. Knepper and V. Zimmermann, Organic Azides: An Exploding Diversity of a Unique Class of Compounds, Angew. Chem., Int. Ed., 2005, 44, 5188–5240 CrossRef PubMed.
-
(a) J. Das, S. N. Patil, R. Awasthi, C. P. Narasimhulu and S. Trehan, An Easy Access to Aryl Azides from Aryl Amines under Neutral Conditions, Synthesis, 2005, 1801–1806 CrossRef CAS;
(b) K. Barral, A. D. Moorhouse and J. E. Moses, Efficient Conversion of Aromatic Amines into Azides: A One-Pot Synthesis of Triazole Linkages, Org. Lett., 2007, 9, 1809–1811 CrossRef CAS PubMed;
(c) L. M. Jin, X. Xu, H. Lu, X. Cui, L. Wojtas and X. P. Zhang, Effective Synthesis of Chiral N-Fluoroaryl Aziridines through Enantioselective Aziridination of Alkenes with Fluoroaryl Azides, Angew. Chem., Int. Ed., 2013, 52, 5309–5313 CrossRef CAS PubMed;
(d) F. Sebest, L. Casarrubios, H. S. Rzepa, A. J. P. White and S. Díez-González, Thermal azide–alkene cycloaddition reactions: straightforward multi-gram access to Δ2–1,2,3-triazolines in deep eutectic solvents, Green Chem., 2018, 20, 4023–4035 RSC.
-
(a) C.-Z. Tao, X. Cui, J. Li, A.-X. Liu, L. Liu and Q.-X. Guo, Copper-catalyzed synthesis of aryl azides and 1-aryl-1,2,3-triazoles from boronic acids, Tetrahedron Lett., 2007, 48, 3525–3529 CrossRef CAS;
(b) K. D. Grimes, A. Gupte and C. C. Aldrich, Copper(II)-Catalyzed Conversion of Aryl/Heteroaryl Boronic Acids, Boronates, and Trifluoroborates into the Corresponding Azides: Substrate Scope and Limitations, Synthesis, 2010, 1441–1448 CAS;
(c) H. Luo, Y.-F. Lv, H. Zhang, J.-M. Hu, H.-M. Li and S.-J. Liu, Synthesis and Antitumor Activity of 1-Substituted 1,2,3-Triazole-Mollugin Derivatives, Molecules, 2021, 26, 3249 CrossRef CAS PubMed;
(d) M. Payne, A. L. Bottomley, A. Och, H. G. Hiscocks, A. P. Asmara, E. J. Harry and A. T. Ung, Synthesis and biological evaluation of tetrahydroisoquinoline-derived antibacterial compounds, Bioorg. Med. Chem., 2022, 57, 116648 CrossRef CAS PubMed.
-
(a) M. Sundhoro, S. Jeon, J. Park, O. Ramström and M. Yan, Perfluoroaryl Azide Staudinger Reaction: A Fast and Bioorthogonal Reaction, Angew. Chem., Int. Ed., 2017, 56, 12117–12121 CrossRef CAS PubMed;
(b) M. Berta, A. Dancsó, A. Nemes, Z. Pathó, D. Szabó and J. Rábai, Convenient synthesis of pure fluorous alkyl azides at multigram scale, J. Fluor. Chem., 2017, 196, 57–62 CrossRef CAS.
- W. Zhu and D. Ma, Synthesis of aryl azides and vinyl azides via proline-promoted CuI-catalyzed coupling reactions, Chem. Commun., 2004, 888–889 RSC.
- O. Bakhanovich and P. Beier, Synthesis, Stability and Reactivity of α-Fluorinated Azidoalkanes, Chem. – Eur. J., 2020, 26, 773–782 CrossRef CAS PubMed.
- P. Sivaguru, Y. Ning and X. Bi, New Strategies for the Synthesis of Aliphatic Azides, Chem. Rev., 2021, 121, 4253–4307 CrossRef CAS PubMed.
- H. Martinez, A. Rebeyrol, T. B. Nelms and W. R. Dolbier Jr, Impact of fluorine substituents on the rates of nucleophilic aliphatic substitution and β-elimination, J. Fluor. Chem., 2012, 135, 167–175 CrossRef CAS.
- S. Voltrová, J. Filgas, P. Slavíček and P. Beier, Azidofluoromethane: synthesis, stability and reactivity in [3 + 2] cycloadditions, Org. Chem. Front., 2020, 7, 10–13 RSC.
- M. Glaser and E. Årstad, “Click Labeling” with 2-[18F]Fluoroethylazide for Positron Emission Tomography, Bioconjugate Chem., 2007, 18, 989–993 CrossRef CAS PubMed.
-
(a) A. Haslop, A. Gee, C. Plisson and N. Long, Fully automated radiosynthesis of [1-(2-[18F]fluoroethyl),1H[1,2,3]triazole 4-ethylene] triphenylphosphonium bromide as a potential positron emission tomography tracer for imaging apoptosis, J. Labelled Compd. Radiopharm., 2013, 56, 313–316 CrossRef CAS PubMed;
(b) K. Kettenbach, H. Schieferstein and T. L. Ross, 18F-labeling using click cycloadditions, BioMed. Res. Int., 2014, 2014, 361329 Search PubMed;
(c) B. S. Lee, S. Y. Chu, W. J. Jung, H. J. Jeong, K. Lee, M. H. Kim, M. H. Kim, D. Y. Chi, H. Ahn, Y. J. Lee, K. C. Lee and S. M. Lim, 18F-labeled 1,2,3-triazole-linked Glu-urea-Lys-based PSMA ligands have good pharmacokinetic properties for positron emission tomography imaging of prostate cancer, Prostate, 2020, 80, 1383–1393 CrossRef CAS PubMed.
- Y.-M. Wu, J. Deng, X. Fang and Q.-Y. Chen, Regioselective synthesis of fluoroalkylated [1,2,3]-triazoles by Huisgen cycloaddition, J. Fluor. Chem., 2004, 125, 1415–1423 CrossRef CAS.
- J. Tomaszewska, K. Koroniak-Szejn and H. Koroniak, Fluorinated organic azides – their preparation and synthetic application, ARKIVOC, 2017,(part ii), 421–432 Search PubMed.
-
(a) A. Haas, M. Spitzer and M. Lieb, Synthese seitenkettenfluorierter aromatischer Verbindungen und deren chemische Reaktivität, Chem. Ber., 1988, 121, 1329–1340 CrossRef CAS;
(b) W. M. Koppes and M. Chaykovsky, US Pat, 5276171, 1994 Search PubMed;
(c) S. A. Lermontov, A. G. Polivanova and S. B. Shkavrov, Interaction of α,α-difluoroazides with trivalent phosphorus compounds and triphenylantimony, Russ. J. Gen. Chem., 2010, 80, 1646–1651 CrossRef CAS;
(d) W. Zhao, H. Li, J. Zhang and S. Cao, Synthesis of Novel gem-Difluoromethylene-containing 1,2,3-Triazoles via Click Reaction, Chin. J. Chem., 2011, 29, 2763–2768 CrossRef CAS;
(e) M. Mamone, R. S. B. Goncalves, F. Blanchard, G. Bernadat, S. Ongeri, T. Milcent and B. Crousse, N-Difluoromethyl-triazole as a constrained scaffold in peptidomimetics, Chem. Commun., 2017, 53, 5024–5027 RSC;
(f) J. Engel-Andreasen, I. Wellhofer, K. Wich and C. A. Olsen, Backbone-Fluorinated 1,2,3-Triazole-Containing Dipeptide Surrogates, J. Org. Chem., 2017, 82, 11613–11619 CrossRef CAS PubMed;
(g) J. Y. Chai, H. Cha, H. B. Kim and D. Y. Chi, Selective addition reactions of difluoromethyltriazoles to ketones and aldehydes without the formation of difluorocarbene, Tetrahedron, 2020, 76, 131370 CrossRef CAS;
(h) J. Laxio Arenas, Y. Xu, T. Milcent, C. Van Heijenoort, F. Giraud, T. Ha-Duong, B. Crousse and S. Ongeri, Fluorinated Triazole Foldamers: Folded or Extended Conformational Preferences, ChemPlusChem, 2021, 86, 241–251 CrossRef CAS PubMed.
-
(a) E. M. Sletten and C. R. Bertozzi, A Bioorthogonal Quadricyclane Ligation, J. Am. Chem. Soc., 2011, 133, 17570–17573 CrossRef CAS PubMed;
(b) R. van Geel, M. A. Wijdeven, R. Heesbeen, J. M. M. Verkade, A. A. Wasiel, S. S. van Berkel and F. L. van Delft, Chemoenzymatic Conjugation of Toxic Payloads to the Globally Conserved N-Glycan of Native mAbs Provides Homogeneous and Highly Efficacious Antibody–Drug Conjugates, Bioconjugate Chem., 2015, 26, 2233–2242 CrossRef CAS PubMed.
-
(a) T. G. Archibald and K. Baum, Synthesis of fluoro nitro ethers by Michael addition reactions to activated β,β-difluoroolefins, J. Org. Chem., 1990, 55, 3562–3565 CrossRef CAS;
(b) T. Hiramatsu, Y. Guo and T. Hosoya, 3-Azidodifluoromethyl-3H-diazirin-3-yl group as an all-in-one functional group for radioisotope-free photoaffinity labelling, Org. Biomol. Chem., 2007, 5, 2916–2919 RSC.
- S. A. Postovoi, Y. V. Zeifman and I. L. Knunyants, Reaction of vicinal dihalopolyfluoroalkanes with sodium azide, Russ. Chem. Bull., 1986, 35, 1183–1186 CrossRef.
-
(a) I. Rico and C. Wakselman, Condensation of 1,2-dibromotetrafluoroethane with various potassium thiophenoxides and phenoxides, J. Fluor. Chem., 1982, 20, 759–764 CrossRef CAS;
(b) S. A. Postovoi, L. T. Lantseva, Y. V. Zeifman and I. L. Knunyants, Reaction of perfluoro-tert-butyl anion with vicinal dihalopolyfluoroalkanes, Russ. Chem. Bull., 1982, 31, 2233–2236 CrossRef.
- For a related approach through Grignard formation from 1,2-dibromotetrafluoroethane and subsequent decomposition and trapping with azide see: D. Tichý, V. Košťál, V. Motornov, I. Klimánková and P. Beier, Preparation of 1-Azido-2-Bromo-1,1,2,2-Tetrafluoroethane and Its Use in the Synthesis of N-Fluoroalkylated Nitrogen Heterocycles, J. Org. Chem., 2020, 85, 11482–11489 CrossRef PubMed.
- E. Shaitanova, V. Matoušek, T. Herentin, M. Adamec, R. Matyáš, B. Klepetářová and P. Beier, Synthesis and Cycloaddition Reactions of 1-Azido-1,1,2,2-tetrafluoroethane, J. Org. Chem., 2023, 88, 14969–14977 CrossRef CAS PubMed.
- J. Dai, Z. Li, T. Wang and R. Bai, A highly stable and versatile heterobifunctional fluoroalkylation reagent for preparation of fluorinated organic compounds, Org. Biomol. Chem., 2016, 14, 4382–4386 RSC.
- J. Dai, Z. Li, T. Wang, W. Bai and R. Bai, A Diheteroatom Fluoroalkylation Reagent for Preparation of S- and N-Containing Fluoroalkyl Compounds and Sulfonic Acid Polymer, Org. Lett., 2017, 19, 1418–1421 CrossRef CAS PubMed.
- C. G. Krespan, F. A. Van-Catledge and B. E. Smart, Generation and capture of functionalized fluorocarbanions, J. Am. Chem. Soc., 1984, 106, 5544–5546 CrossRef CAS.
- C. G. Krespan and B. E. Smart, Fluorocarbanion chemistry. A versatile synthesis of functionalized fluoro ketones, J. Org. Chem., 1986, 51, 320–326 CrossRef CAS.
- V. A. Ginsburg, P. O. Gitel and I. M. Didina, SU Pat, 375287A1, 1973 Search PubMed.
- H. Bock and R. Dammel, Gas-phase reactions. 53. Pyrolysis of difluoromethyl azide, Inorg. Chem., 1985, 24, 4427–4429 CrossRef CAS.
- S. Voltrová, I. Putovný, V. Matoušek, B. Klepetářová and P. Beier, Reintroducing Azidodifluoromethane: Synthesis, Stability and [3 + 2] Cycloadditions, Eur. J. Org. Chem., 2018, 5087–5090 CrossRef.
- Z. E. Blastik, S. Voltrová, V. Matoušek, B. Jurásek, D. W. Manley, B. Klepetrářová and P. Beier, Azidoperfluoroalkanes: Synthesis and Application in Copper(I)-Catalyzed Azide–Alkyne Cycloaddition, Angew. Chem., Int. Ed., 2017, 56, 346–349 CrossRef CAS PubMed.
-
(a) S. P. Makarov, A. Y. Yakubovich, A. S. Filatov, M. A. Englin and T. Y. Nikiforova, Reactions of Trifluoronitrosomethane with Amines. IV. Reactions of Trifluoronitrosomethane with Hydrazine and Hydroxylamine and Their Derivatives, Zh. Obshch. Khim., 1968, 38, 709–715 CAS;
(b) K. O. Christe and C. J. Schack, Properties of azidotrifluoromethane, Inorg. Chem., 1981, 20, 2566–2570 CrossRef CAS.
- S. Voltrová, M. Muselli, J. Filgas, V. Matoušek, B. Klepetrářová and P. Beier, Synthesis of tetrafluoroethylene- and tetrafluoroethyl-containing azides and their 1,3-dipolar cycloaddition as synthetic application, Org. Biomol. Chem., 2017, 15, 4962–4965 RSC.
- M. Ziabko, B. Klepetrářová and P. Beier, Synthesis of Azidodifluoromethyl Phenyl Sulfone and Its Use as a Synthetic Equivalent of the Azidodifluoromethyl Anion, J. Org. Chem., 2023, 88, 6939–6946 CrossRef CAS PubMed.
- J. Sultana, N. D. Khupse, S. Chakrabarti, P. Chattopadhyay and D. Sarma, Ag2CO3-catalyzed cycloaddition of organic azides onto terminal alkynes: A green and sustainable protocol accelerated by aqueous micelles of CPyCl, Tetrahedron Lett., 2019, 60, 1117–1121 CrossRef CAS.
-
(a) A. Montagu, V. Roy, J. Balzarini, R. Snoeck, G. Andrei and L. A. Agrofoglio, Synthesis of new C5-(1-substituted-1,2,3-triazol-4 or 5-yl)-2′-deoxyuridines and their antiviral evaluation, Eur. J. Med. Chem., 2011, 46, 778–786 CrossRef CAS PubMed;
(b) T. Irie, I. Fujii and M. Sawa, Design and combinatorial synthesis of a novel kinase-focused library using click chemistry-based fragment assembly, Bioorg. Med. Chem. Lett., 2012, 22, 591–596 CrossRef CAS PubMed;
(c) A. Torrens-Jover, N. Jagerovic and C. Almansa-Rosales, WO Pat, 2017178515A1, 2017 Search PubMed.
-
(a) L. Maugeri, T. Lébl, D. B. Cordes, A. M. Z. Slawin and D. Philp, Cooperative Binding in a Phosphine Oxide-Based Halogen Bonded Dimer Drives Supramolecular Oligomerization, J. Org. Chem., 2017, 82, 1986–1995 CrossRef CAS PubMed;
(b) A. Docker, C. H. Guthrie, H. Kuhn and P. D. Beer, Modulating Chalcogen Bonding and Halogen Bonding Sigma-Hole Donor Atom Potency and Selectivity for Halide Anion Recognition, Angew. Chem., Int. Ed., 2021, 60, 21973–21978 CrossRef CAS PubMed;
(c) F. Ostler, D. G. Piekarski, T. Danelzik, M. S. Taylor and O. Garcia Mancheno, Neutral Chiral Tetrakis-Iodo-Triazole Halogen-Bond Donor for Chiral Recognition and Enantioselective Catalysis, Chem. – Eur. J., 2021, 27, 2315–2320 CrossRef CAS PubMed;
(d) T. Bunchuay, A. Docker, N. G. White and P. D. Beer, A new halogen bonding 1,2-iodo-triazolium-triazole benzene motif for anion recognition, Polyhedron, 2021, 209, 115482 CrossRef CAS;
(e) A. Docker, X. Shang, D. Yuan, H. Kuhn, Z. Zhang, J. J. Davis, P. D. Beer and M. J. Langton, Halogen Bonding Tetraphenylethene Anion Receptors: Anion-Induced Emissive Aggregates and Photoswitchable Recognition, Angew. Chem., Int. Ed., 2021, 60, 19442–19450 CrossRef CAS PubMed;
(f) L. E. Bickerton, A. Docker, A. J. Sterling, H. Kuhn, F. Duarte, P. D. Beer and M. J. Langton, Highly Active Halogen Bonding and Chalcogen Bonding Chloride Transporters with Non-Protonophoric Activity, Chem. – Eur. J., 2021, 27, 11738–11745 CrossRef CAS PubMed;
(g) R. Hein, A. Docker, J. J. Davis and P. D. Beer, Redox-Switchable Chalcogen Bonding for Anion Recognition and Sensing, J. Am. Chem. Soc., 2022, 144, 8827–8836 CrossRef CAS PubMed;
(h) A. Docker, Y. C. Tse, H. M. Tay, A. J. Taylor, Z. Zhang and P. D. Beer, Anti-Hofmeister Anion Selectivity via a Mechanical Bond Effect in Neutral Halogen-Bonding [2]Rotaxanes, Angew. Chem., Int. Ed., 2022, 61, e202214523 CrossRef CAS PubMed;
(i) A. Docker, I. Marques, H. Kuhn, Z. Zhang, V. Felix and P. D. Beer, Selective Potassium Chloride Recognition, Sensing, Extraction, and Transport Using a Chalcogen-Bonding Heteroditopic Receptor, J. Am. Chem. Soc., 2022, 144, 14778–14789 CrossRef CAS PubMed;
(j) L. E. Bickerton and M. J. Langton, Controlling transmembrane ion transport via photo-regulated carrier mobility, Chem. Sci., 2022, 13, 9531–9536 RSC;
(k) A. C. Keuper, K. Fengler, F. Ostler, T. Danelzik, D. G. Piekarski and O. G. Mancheno, Fine-Tuning Substrate–Catalyst Halogen–Halogen Interactions
for Boosting Enantioselectivity in Halogen-Bonding Catalysis, Angew. Chem., Int. Ed., 2023, 62, e202304781 CrossRef CAS PubMed;
(l) K. M. Bąk, I. Marques, H. Kuhn, K. E. Christensen, V. Félix and P. D. Beer, Fullerene-Functionalized Halogen-Bonding Heteroditopic Hosts for Ion-Pair Recognition, J. Am. Chem. Soc., 2023, 145, 27367–27379 CrossRef PubMed;
(m) T. G. Johnson, A. Docker, A. Sadeghi-Kelishadi and M. J. Langton, Halogen Bonding Relay and Mobile Anion Transporters with Kinetically Controlled Chloride Selectivity, Chem. Sci., 2023, 14, 5006–5013 RSC.
-
(a) L. Zeng, J. Li and S. Cui, Rhodium-Catalyzed Atroposelective Click Cycloaddition of Azides and Alkynes, Angew. Chem., Int. Ed., 2022, 61, e202205037 CrossRef CAS PubMed;
(b) L. Zhou, Y. Li, S. Li, Z. Shi, X. Zhang, C.-H. Tung and Z. Xu, Asymmetric rhodium-catalyzed click cycloaddition to access C–N axially chiral N-triazolyl indoles, Chem. Sci., 2023, 14, 5182–5187 RSC;
(c) L. Zeng, F. Zhang and S. Cui, Construction of Axial Chirality via Click Chemistry: Rh-Catalyzed Enantioselective Synthesis of 1-Triazolyl-2-Naphthylamines, Org. Lett., 2023, 25, 443–448 CrossRef CAS PubMed.
- D. Bhatt, P. R. Singh, P. Kalaramna, K. Kumar and A. Goswami, An Atom-Economical Approach to 2-Triazolyl Thio-/Seleno Pyridines via Ruthenium-Catalyzed One-pot [3 + 2]/[2 + 2 + 2] Cycloadditions, Adv. Synth. Catal., 2019, 361, 5483–5489 CrossRef CAS.
- X. Zhang, S. Li, W. Yu, Y. Xie, C.-H. Tung and Z. Xu, Asymmetric Azide–Alkyne Cycloaddition with Ir(I)/Squaramide Cooperative Catalysis: Atroposelective Synthesis of Axially Chiral Aryltriazoles, J. Am. Chem. Soc., 2022, 144, 6200–6207 CrossRef CAS PubMed.
-
(a) A. K. Feldman, B. Colasson and V. V. Fokin, One-Pot Synthesis of 1,4-Disubstituted 1,2,3-Triazoles from In Situ Generated Azides, Org. Lett., 2004, 6, 3897–3899 CrossRef CAS PubMed;
(b) J. Andersen, S. Bolvig and X. Liang, Efficient One-Pot Synthesis of 1-Aryl 1,2,3-Triazoles from Aryl Halides and Terminal Alkynes in the Presence of Sodium Azide, Synlett, 2005, 2941–2947 CAS.
- For examples starting from polyfluoroaryl boronic acids see:
(a) B. Kaboudin, R. Mostafalu and T. Yokomatsu, Fe3O4 nanoparticle-supported Cu(II)-β-cyclodextrin complex as a magnetically recoverable and reusable catalyst for the synthesis of symmetrical biaryls and 1,2,3-triazoles from aryl boronic acids, Green Chem., 2013, 15, 2266–2274 RSC;
(b) P. K. Chinthakindi, P. L. Sangwan, S. Farooq, R. R. Aleti, A. Kaul, A. K. Saxena, Y. L. N. Murthy, R. A. Vishwakarma and S. Koul, Diminutive effect on T and B-cell proliferation of non-cytotoxic α-santonin derived 1,2,3-triazoles: A report, Eur. J. Med. Chem., 2013, 60, 365–375 CrossRef CAS PubMed;
(c) A. S. Kumar, M. A. Reddy, M. Knorn, O. Reiser and B. Sreedhar, Magnetically Recoverable CuFe2O4 Nanoparticles: Catalyzed Synthesis of Aryl Azides and 1,4-Diaryl-1,2,3-triazoles from Boronic Acids in Water, Eur. J. Org. Chem., 2013, 4674–4680 CrossRef CAS;
(d) B. S. P. A. Kumar, K. H. V. Reddy, K. Karnakar, G. Satish and Y. V. D. Nageswar, Copper on chitosan: an efficient and easily recoverable heterogeneous catalyst for one pot synthesis of 1,2,3-triazoles from aryl boronic acids in water at room temperature, Tetrahedron Lett., 2015, 56, 1968–1972 CrossRef;
(e) C. Hao, C. Zhou, J. Xie, J. Zhang, P. Liu and B. Dai, An Efficient Copper-Catalyzed One-Pot Synthesis of 1-Aryl-1,2,3-triazoles from Arylboronic Acids in Water under Mild Conditions, Chin. J. Chem., 2015, 33, 1317–1320 CrossRef CAS;
(f) R. Nie, R. Sang, X. Ma, Y. Zheng, X. Cheng, W. Li, L. Guo, H. Jin and Y. Wu, Copper-γ-cyclodextrin complexes immobilized on hexagonal boron nitride as an efficient catalyst in the multicomponent synthesis of 1,2,3-triazoles, J. Catal., 2016, 344, 286–292 CrossRef CAS;
(g) J. M. Pérez, P. Crosbie, S. Lal and S. Díez-González, Copper(I)–Phosphinite Complexes in Click Cycloadditions: Three-Component Reactions and Preparation of 5-Iodotriazoles, ChemCatChem, 2016, 8, 2222–2226 CrossRef PubMed;
(h) S. Layek, S. Kumari, Anuradha, B. Agrahari, R. Ganguly and D. D. Pathak, Synthesis, characterization and crystal structure of a diketone based Cu(II) complex and its catalytic activity for the synthesis of 1,2,3-triazoles, Inorg. Chim. Acta, 2016, 453, 735–741 CrossRef CAS;
(i) A. Garg, A. A. Ali, K. Damarla, A. Kumar and D. Sarma, Aqueous bile salt accelerated cascade synthesis of 1,2,3-triazoles from arylboronic acids, Tetrahedron Lett., 2018, 59, 4031–4035 CrossRef CAS;
(j) X.-Y. Huo, L. Guo, X.-F. Chen, Y.-T. Zhou, J. Zhang, X.-Q. Han and B. Dai, Design, Synthesis, and Antifungal Activity of Novel Aryl-1,2,3-Triazole-β-Carboline Hybrids, Molecules, 2018, 23, 1344 CrossRef PubMed.
- For examples starting from polyfluoroaryl iodides see:
(a) L. Ackermann, H. K. Potukuchi, D. Landsberg and R. Vicente, Copper-Catalyzed “Click” Reaction/Direct Arylation Sequence: Modular Syntheses of 1,2,3-Triazoles, Org. Lett., 2008, 10, 3081–3084 CrossRef CAS PubMed;
(b) M. Klein, P. Dinér, D. Dorin-Semblat, C. Doerig and M. Grøtli, Synthesis of 3-(1,2,3-triazol-1-yl)- and 3-(1,2,3-triazol-4-yl)-substituted pyrazolo[3,4-d]pyrimidin-4-amines via click chemistry: potential inhibitors of the Plasmodium falciparum, PfPK7 protein kinase, Org. Biomol. Chem., 2009, 7, 3421–3429 RSC;
(c) C. Proulx and W. D. Lubell, Aza-1,2,3-triazole-3-alanine Synthesis via Copper-Catalyzed 1,3-Dipolar Cycloaddition on Aza-progargylglycine, J. Org. Chem., 2010, 75, 5385–5387 CrossRef CAS PubMed;
(d) T. Kim, J. H. Song, K. H. Jeong, S. Lee and J. Ham, Potassium (1-Organo-1H-1,2,3-triazol-4-yl)trifluoroborates from Ethynyltrifluoro-borate through a Regioselective One-Pot Cu-Catalyzed Azide–Alkyne Cycloaddition Reaction, Eur. J. Org. Chem., 2013, 3992–3996 CrossRef CAS;
(e) P. Dziedzic, J. A. Cisneros, M. J. Robertson, A. A. Hare, N. E. Danford, R. H. G. Baxter and W. L. Jorgensen, Design, Synthesis, and Protein Crystallography of Biaryltriazoles as Potent Tautomerase Inhibitors of Macrophage Migration Inhibitory Factor, J. Am. Chem. Soc., 2015, 137, 2996–3003 CrossRef CAS PubMed;
(f) A. Laurent, M. Proulx, Y. Rose, I. Denissova, K. Dairi, S. Jarvis and J. B. Jaquith, US Pat, 9284350B2, 2016 Search PubMed.
-
(a) V. R. Kamalraj, S. Senthil and P. Kannan, One-pot synthesis and the fluorescent behavior of 4-acetyl-5-methyl-1,2,3-triazole regioisomers, J. Mol. Struct., 2008, 892, 210–215 CrossRef CAS;
(b) F. Stazi, D. Cancogni, L. Turco, P. Westerduin and S. Bacchi, Highly efficient and safe procedure for the synthesis of aryl 1,2,3-triazoles from aromatic amine in a continuous flow reactor, Tetrahedron Lett., 2010, 51, 5385–5387 CrossRef CAS;
(c) L. Wang, S. Peng, L. J. Danence, Y. Gao and J. Wang, Amine-Catalyzed [3 + 2] Huisgen Cycloaddition Strategy for the Efficient Assembly of Highly Substituted 1,2,3-Triazoles, Chem. – Eur. J., 2012, 18, 6088–6093 CrossRef CAS PubMed;
(d) W. Li, Q. Jia, Z. Du and J. Wang, Direct access to triazole-olefins through catalytic cycloaddition of azides to unsaturated aldehydes, Chem. Commun., 2013, 49, 10187–10189 RSC;
(e) H. Singh, J. Sindhu and J. M. Khurana, Efficient, green and regioselective synthesis of 1,4,5-trisubstituted-1,2,3-triazoles in ionic liquid [bmim]BF4 and in task-specific basic ionic liquid [bmim]OH, J. Iran. Chem. Soc., 2013, 10, 883–888 CrossRef CAS;
(f) H. Singh, J. Sindhu and J. M. Khurana, Synthesis of biologically as well as industrially important 1,4,5-trisubstituted-1,2,3-triazoles using a highly efficient, green and recyclable DBU–H2O catalytic system, RSC Adv., 2013, 3, 22360–22366 RSC;
(g) D. B. Ramachary, A. B. Shashank and S. Karthik, An Organocatalytic Azide–Aldehyde [3 + 2] Cycloaddition: High-Yielding Regioselective Synthesis of 1,4-Disubstituted 1,2,3-Triazoles, Angew. Chem., Int. Ed., 2014, 53, 10420–10424 CrossRef CAS PubMed;
(h) A. B. Shashank, S. Karthik, R. Madhavachary and D. B. Ramachary, An Enolate-Mediated Organocatalytic Azide–Ketone [3 + 2]-Cycloaddition Reaction: Regioselective High-Yielding Synthesis of Fully Decorated 1,2,3-Triazoles, Chem. – Eur. J., 2014, 20, 16877–16881 CrossRef CAS PubMed;
(i) S. R. Putapatri, A. Kanwal, S. K. Banerjee and S. Kantevari, Synthesis of novel l-rhamnose derived acyclic C-nucleosides with substituted 1,2,3-triazole core as potent sodium-glucose co-transporter (SGLT) inhibitors, Bioorg. Med. Chem. Lett., 2014, 24, 1528–1531 CrossRef CAS PubMed;
(j) S. Zhou, H. Liao, M. Liu, G. Feng, B. Fu, R. Li, M. Cheng, Y. Zhao and P. Gong, Discovery and biological evaluation of novel 6,7-disubstituted-4-(2-fluorophenoxy)quinoline derivatives possessing 1,2,3-triazole-4-carboxamide moiety as c-Met kinase inhibitors, Bioorg. Med. Chem., 2014, 22, 6438–6452 CrossRef CAS PubMed;
(k) P. M. Krishna, D. B. Ramachary and S. Peesapati, Azide–acetonitrile “click” reaction triggered by Cs2CO3: the atom-economic, high-yielding synthesis of 5-amino-1,2,3-triazoles, RSC Adv., 2015, 5, 62062–62066 RSC;
(l) X. Zhou, X. Xu, K. Liu, H. Gao, W. Wang and W. Li, Organocatalytic 1,3-Dipolar Cyclo-addition Reaction of β-Keto Amides with Azides – Direct Access to 1,4,5-Trisubstituted 1,2,3-Triazole-4-carb-oxamides, Eur. J. Org. Chem., 2016, 1886–1890 CrossRef CAS;
(m) D. B. Ramachary, J. Gujral, S. Peraka and G. S. Reddy, Triazabicyclodecene as an Organocatalyst for the Regiospecific Synthesis of 1,4,5-Trisubstituted N-Vinyl-1,2,3-triazoles, Eur. J. Org. Chem., 2017, 459–464 CrossRef CAS;
(n) D. B. Ramachary, G. S. Reddy, S. Peraka and J. Gujral, Organocatalytic Vinyl Azide-Carbonyl [3 + 2] Cycloaddition: High-Yielding Synthesis of Fully Decorated N-Vinyl-1,2,3-Triazoles, ChemCatChem, 2017, 9, 263–267 CrossRef CAS;
(o) Z. Shi and W. Li, Enamine-Mediated 1,3-Dipolar Cycloaddition Reaction of Curcumin Derivatives with Azides: Direct Access to 1,4,5-Trisubstituted 1,2,3-Triazoles, Synthesis, 2017, 2081–2087 CAS;
(p) D. M. Xavier, B. S. Goldani, N. Seus, R. G. Jacob, T. Barcellos, M. W. Paixão, R. Luque and D. Alves, Sonochemistry in organocatalytic enamine-azide [3 + 2] cycloadditions: A rapid alternative for the synthesis of 1,2,3-triazoyl carboxamides, Ultrason. Sonochem., 2017, 34, 107–114 CrossRef CAS PubMed;
(q) G. S. Reddy, L. M. Reddy, A. S. Kumar and D. B. Ramachary, Organocatalytic Selective [3 + 2] Cycloadditions: Synthesis of Functionalized 5-Arylthiomethyl-1,2,3-triazoles and 4-Arylthio-1,2,3-triazoles, J. Org. Chem., 2020, 85, 15488–15501 CrossRef CAS PubMed;
(r) A. Zhu, L. Li, C. Zhang, Y. Shen, M. Tang, L. Bai, C. Du, S. Zhang and J. Wang, An integrated high-throughput strategy enables the discovery of multifunctional ionic liquids for sustainable chemical processes, Green Chem., 2019, 21, 307–313 RSC;
(s) C. H. A. Rajeena, V. Kamat, V. B. Patil, S. P. Nayak, S. Khanapure, D. A. Barretto and S. K. Vootla, Synthesis and antibacterial evaluation of pyrazolines carrying (benzyloxy)benzaldehyde moiety, J. Iran. Chem. Soc., 2022, 19, 1641–1650 CrossRef CAS;
(t) C. B. Gomes, C. L. Corrêa, D. C. Cabrera, M. G. M. D'Oca, M. Ruiz, T. Collares, L. Savegnago, F. K. Seixas and D. Alves, Organocatalytic Synthesis and Antitumor Activity of Novel 1,2,3-triazoles Derived from Fatty β-ketoesters, Med. Chem., 2022, 18, 463–472 CrossRef CAS PubMed.
-
(a) D. B. Ramachary, P. M. Krishna, J. Gujral and G. S. Reddy, An Organocatalytic Regiospecific Synthesis of 1,5-Disubstituted 4-Thio-1,2,3-triazoles and 1,5-Disubstituted 1,2,3-Triazoles, Chem. – Eur. J., 2015, 21, 16775–16780 CrossRef CAS PubMed;
(b) A. Baykal, D. Zhang, J. Knelles, I. T. Alt and B. Plietker, Nucleophilic Iron Complexes in Proton-Transfer Catalysis: An Iron-Catalyzed Dimroth Cyclocondensation, Chem. – Asian J., 2019, 14, 3003–3010 CrossRef CAS PubMed;
(c) L. Cicco, F. M. Perna, A. Falcicchio, A. Altomare, F. Messa, A. Salomone, V. Capriati and P. Vitale, 1,3-Dipolar Cycloaddition of Alkanone Enolates with Azides in Deep Eutectic Solvents for the Metal-Free Regioselective Synthesis of Densely Functionalized 1,2,3-Triazoles, Eur. J. Org. Chem., 2022, e202200843 CrossRef CAS.
- A. Krasiński, V. V. Fokin and K. B. Sharpless, Direct Synthesis of 1,5-Disubstituted-4-magnesio-1,2,3-triazoles, Revisited, Org. Lett., 2004, 6, 1237–1240 CrossRef PubMed.
-
(a) S. G. Fridman and N. M. Lisovska, Zap. Inst. Khim., Akad. Nauk Ukr. RSR, 1940, 6, 353–360 CAS;
(b) N. M. Boyer, C. H. Mack, N. Goebel and L. R. Morgan Jr., Notes - Reactions of Sodium Phenylacetylide and Sodium Alkoxide with Tosyl and Mesyl Azides, J. Org. Chem., 1958, 23, 1051–1053 CrossRef;
(c) G. S. Akimova, V. N. Chistokletov and A. A. Petrov, Reactions of Iotsich Complexes with Phenyl Azide, Zh. Org. Khim., 1965, 1, 2077 CAS;
(d) G. Akimova, V. Chistokletov and A. Petrov, 1,3-Bipolar Addition to Unsaturated Compounds. XVII. The Reaction of Azides with Iotsich Complexes Obtained from Phenyl- and Alkenylacetylenes, Zh. Obshch. Khim., 1967, 3, 968–974 CAS.
-
(a) S. W. Kwok, J. R. Fotsing, R. J. Fraser, V. O. Rodionov and V. V. Fokin, Transition-Metal-Free Catalytic Synthesis of 1,5-Diaryl-1,2,3-triazoles, Org. Lett., 2010, 12, 4217–4219 CrossRef CAS PubMed;
(b) C. D. Smith and M. F. Greaney, Zinc Mediated Azide–Alkyne Ligation to 1,5- and 1,4,5-Substituted 1,2,3-Triazoles, Org. Lett., 2013, 15, 4826–4829 CrossRef CAS PubMed;
(c) L. Hong, W. Lin, F. Zhang, R. Liua and X. Zhou, Ln[N(SiMe3)2]3-catalyzed cycloaddition of terminal alkynes to azides leading to 1,5-disubstituted 1,2,3-triazoles: new mechanistic features, Chem. Commun., 2013, 49, 5589–5591 RSC;
(d) M. De Tullio, A. M. Borys, A. Hernán-Gómez, A. R. Kennedy and E. Hevia, Regioselective synthesis of 1,5-disubstituted 1,2,3-triazoles catalyzed by cooperative s-block bimetallics, Chem Catal., 2021, 1, 1308–1321 CrossRef CAS;
(e) M. B. Williams, M. L. Martin, S. Wiedmann and A. Boyer, Exploiting 1,1-Dibromoalkenes as Direct Precursors to 5-Substituted 1,2,3-Triazoles, Synthesis, 2023, 3862–3874 CAS.
- F. E. Bugden, G. J. Clarkson and M. D. Greenhalgh, Eur. J. Org. Chem., 2023, e202201459 CrossRef CAS.
-
(a) R. Raap, Reactions of 1-Phenyl-1,2,3-triazolyllithium Compounds; Formation of Ketenimines, Alkynylamines, and Heterocycles from the Ambident (N-Phenyl)phenylketenimine Anion, Can. J. Chem., 1971, 49, 1792–1798 CrossRef CAS;
(b) I. Bae, H. Han and S. Chang, Highly Efficient One-Pot Synthesis of N-Sulfonylamidines by Cu-Catalyzed Three-Component Coupling of Sulfonyl Azide, Alkyne, and Amine, J. Am. Chem. Soc., 2005, 127, 2038–2039 CrossRef CAS PubMed;
(c) M. P. Cassidy, J. Raushel and V. V. Fokin, Practical Synthesis of Amides from In Situ Generated Copper(I) Acetylides and Sulfonyl Azides, Angew. Chem., Int. Ed., 2006, 45, 3154–3157 CrossRef CAS PubMed;
(d) E. J. Yoo, M. Ahlquist, S. H. Kim, I. Bae, V. V. Fokin and K. B. Sharpless, Copper-Catalyzed Synthesis of N-Sulfonyl-1,2,3-triazoles: Controlling Selectivity, Angew. Chem., Int. Ed., 2007, 46, 1730–1733 CrossRef CAS PubMed;
(e) E. J. Yoo, M. Ahlquist, I. Bae, K. B. Sharpless, V. V. Fokin and S. Chang, Mechanistic Studies on the Cu-Catalyzed Three-Component Reactions of Sulfonyl Azides, 1-Alkynes and Amines, Alcohols, or Water: Dichotomy via a Common Pathway, J. Org. Chem., 2008, 73, 5520–5528 CrossRef CAS PubMed.
- A. Budeev, G. Kantin, D. Dar'in and M. Krasavin, Diazocarbonyl and Related Compounds in the Synthesis of Azoles, Molecules, 2021, 26, 2530 CrossRef CAS PubMed.
-
(a) L. Wolff, Ueber Diazoanhydride, Justus Liebigs Ann. Chem., 1902, 325, 129–195 CrossRef;
(b) L. Wolff, Über Diazoanhydride (1,2,3-Oxydiazole oder Diazoxyde) und Diazoketone, Justus Liebigs Ann. Chem., 1912, 394, 23–59 CrossRef CAS.
- A. Safrygin, D. Dar'in, G. Kantin and M. Krasavin, α-Diazo-β-oxosulfones as Partners in the Wolff 1,2,3-Triazole Synthesis and the Wolff Rearrangement in the Presence of Aromatic Amines, Eur. J. Org. Chem., 2019, 4721–4724 CrossRef CAS.
-
(a) S. A. Lermontov, S. V. Shkavrov and A. N. Pushin, The reaction of α,α-difluoroazides with acetylenic compounds, J. Fluor. Chem., 2000, 105, 141–147 CrossRef CAS;
(b) S. A. Lermontov, S. V. Shkavrov, A. N. Pushin and V. V. Tkachev, Unusual Fluorination of Diphenylacetylene with Methyl 3-Azidotetrafluoropropionate, Russ. J. Gen. Chem., 2002, 72, 1289–1290 CrossRef CAS.
- X. Chen, Y. Zheng, W. Song, R. Zheng, D. Wang, L. Kong, B. Lu and H. Lin, CN Pat, 113149916A, 2021 Search PubMed.
-
(a) L. Janecky, A. Markos, B. Klepetářová and P. Beier, Lewis-Acid-Mediated Intramolecular Cyclization of 4-Aryl-5-allyl-1,2,3-triazoles to Substituted Cyclopentene Derivatives, J. Org. Chem., 2023, 88, 1155–1167 CrossRef CAS PubMed;
(b) A. Markos, L. Janecký, T. Chvojka, T. Martinek, H. Martinez-Seara, B. Klepetářová and P. Beier, Haloalkenyl Imidoyl Halides as Multifacial Substrates in the Stereoselective Synthesis of N-Alkenyl Compounds, Adv. Synth. Catal., 2021, 363, 3258–3266 CrossRef CAS.
- Z. E. Blastik, B. Klepetářová and P. Beier, Enamine-Mediated Azide-Ketone [3 + 2] Cycloaddition of Azidoperfluoroalkanes, ChemistrySelect, 2018, 3, 7045–7048 CrossRef CAS.
-
(a) A. Jończyk, E. Nawrot and M. Kisielewski, Reactions of some nitrogen heterocycles with chlorodifluoromethane under conditions of phase-transfer catalysis, J. Fluor. Chem., 2005, 126, 1587–1591 CrossRef;
(b) J. Zheng, Y. Li, L. Zhang, J. Hu, G. J. Meuzelaar and H.-J. Federsel, Chlorodifluoromethyl phenyl sulfone: a novel non-ozone-depleting substance-based difluorocarbene reagent for O- and N-difluoromethylations, Chem. Commun., 2007, 5149–5151 RSC;
(c) J. E. Boehmer and M. M. W. Mclachlan, WO Pat, 2007096576, 2007 Search PubMed;
(d) W. Zhang, F. Wang and J. Hu, N-Tosyl-S-difluoromethyl-S-phenylsulfoximine: A New Difluoromethylation Reagent for S-, N-, and C-Nucleophiles, Org. Lett., 2009, 11, 2109–2112 CrossRef CAS PubMed;
(e) F. Wang, L. Zhang, J. Zheng and J. Hu, Chlorodifluoromethyl aryl ketones and sulfones as difluorocarbene reagents: The substituent effect, J. Fluor. Chem., 2011, 132, 521–528 CrossRef CAS;
(f) J. J. Crawford, D. F. Ortwine, B. Wei and W. B. Young, US Pat, 20130116246A1, 2013 Search PubMed;
(g) L. Li, F. Wang, C. Ni and J. Hu, Synthesis of gem-Difluorocyclopropa(e)nes and O-, S-, N-, and P-Difluoromethylated Compounds with TMSCF2Br, Angew. Chem., Int. Ed., 2013, 52, 12390–12394 CrossRef CAS PubMed;
(h) G. K. S. Prakash, S. Krishnamoorthy, S. K. Ganesh, A. Kulkarni, R. Haiges and G. A. Olah, N-Difluoromethylation of Imidazoles and Benzimidazoles Using the Ruppert–Prakash Reagent under Neutral Conditions, Org. Lett., 2014, 16, 54–57 CrossRef CAS PubMed;
(i) F. Wang, L. Li, C. Ni and J. Hu, Deoxygenative gem-difluoroolefination of carbonyl compounds with (chlorodifluoromethyl)trimethylsilane
and triphenylphosphine, Beilstein J. Org. Chem., 2014, 10, 344–351 CrossRef PubMed;
(j) C. C. McComas, S. D. Kuduk, D. Scott and T. S. Reger, WO Pat, 2014081619A1, 2014 Search PubMed;
(k) C. S. Thomoson, L. Wang and W. R. Dolbier, Use of fluoroform as a source of difluorocarbene in the synthesis of N-CF2H heterocycles and difluoromethoxypyridines, J. Fluor. Chem., 2014, 168, 34–39 CrossRef CAS;
(l) X.-Y. Deng, J.-H. Lin, J. Zheng and J.-C. Xiao, Difluoromethylation and gem-difluorocyclopropenation with difluorocarbene generated by decarboxylation, Chem. Commun., 2015, 51, 8805–8808 RSC;
(m) K. I. Petko, Difluoromethylation of parent azoles, J. Fluor. Chem., 2018, 205, 5–7 CrossRef CAS.
- K. Niedermann, N. Früh, R. Senn, B. Czarniecki, R. Verel and A. Togni, Direct Electrophilic N-Trifluoromethylation of Azoles by a Hypervalent Iodine Reagent, Angew. Chem., Int. Ed., 2012, 51, 6511–6515 CrossRef CAS PubMed.
- R. Z. Zhang, R. X. Zhang, S. Wang, C. Xu, W. Guan and M. Wang, An N-Trifluoromethylation/Cyclization Strategy for Accessing Diverse N-Trifluoromethyl Azoles from Nitriles and 1,3-Dipoles, Angew. Chem., Int. Ed., 2022, 61, e202110749 CrossRef CAS PubMed.
- K. Niedermann, N. Früh, E. Vinogradova, M. S. Wiehn, A. Moreno and A. Togni, A Ritter-Type Reaction: Direct Electrophilic Trifluoromethylation at Nitrogen Atoms Using Hypervalent Iodine Reagents, Angew. Chem., Int. Ed., 2011, 50, 1059–1063 CrossRef CAS PubMed.
- C. Xu, X. Song, J. Guo, S. Chen, J. Gao, J. Jiang, F. Gao, Y. Li and M. Wang, Synthesis of Chloro(phenyl)trifluoromethyliodane and Catalyst-Free Electrophilic Trifluoromethylations, Org. Lett., 2018, 20, 3933–3393 CrossRef CAS PubMed.
- R. Z. Zhang, W. Huang, R. X. Zhang, C. Xu and M. Wang, Synthesis of N–CF3 Amidines/Imidates/Thioimidates via N–CF3 Nitrilium Ions, Org. Lett., 2022, 24, 2393–2398 CrossRef CAS PubMed.
- E. M. Bacon, G. Balan, C.-H. Chou, C. T. Clark, J. J. Cottell, M. Kim, T. A. Kirschberg, J. O. Link, G. Phillips, S. D. Schroeder, N. H. Squires, K. L. Stevens, J. G. Taylor, W. J. Watkins, N. E. Wright and S. M. Zipfel, WO Pat, 2017007689A1, 2017 Search PubMed.
- C. Boss, S. Cren, T. Kinnerlin, C. Lotz-Jenne, J. Pothier and N. Tidten-Luksch, WO Pat, 2019138107A1, 2019 Search PubMed.
- M. Serrano-Wu, B. Hubbard, V. Kaushik and D. Daniels, WO Pat, 2020150474A1, 2020 Search PubMed.
- A. S. Paparella, K. J. Lee, A. J. Hayes, J. Feng, Z. Feng, D. Cini, S. Deshmukh, G. W. Booker, M. C. J. Wilce, S. W. Polyak and A. D. Abell, Halogenation of Biotin Protein Ligase Inhibitors Improves Whole Cell Activity against Staphylococcus aureus, ACS Infect. Dis., 2018, 4, 175–184 CrossRef CAS PubMed.
- J. Liu, W. Zhang, H. Ma, C. Wang and Z. Xie, US Pat, 2023026724A1, 2023 Search PubMed.
- J. L. Arenas, P. Retailleau, J. M. Gillet, N. E. Ghermani, S. Ongeri and B. Crousse, 5-Fluoro-1,2,3-triazole motif in peptides and its electronic properties, Org. Biomol. Chem., 2022, 20, 8410–8414 RSC.
- E. Bourque, R. Heidebrecht and C. P. Hencken, US Pat, 2023045277A1, 2023 Search PubMed.
- X. Zhang, F. Basuli, S. Abdelwahed, T. Begley and R. Swenson, Radiosynthesis of 5-[18F]Fluoro-1,2,3-triazoles through Aqueous Iodine–[18F]Fluorine Exchange Reaction, Molecules, 2021, 26, 5522 CrossRef CAS PubMed.
- X. J. Wang, K. Sidhu, L. Zhang, S. Campbell, N. Haddad, D. C. Reeves, D. Krishnamurthy and C. H. Senanayake, Bromo-Directed N-2 Alkylation of N,H-1,2,3-Triazoles: Efficient Synthesis of Poly-Substituted 1,2,3-Triazoles, Org. Lett., 2009, 11, 5490–5493 CrossRef CAS PubMed.
- Y. Chen, Y. Liu, J. L. Petersen and X. Shi, Conformational control in the regioselective synthesis of N-2-substituted-1,2,3-triazoles, Chem. Commun., 2008, 3254–3256 RSC.
- V. A. Motornov, A. A. Tabolin, R. A. Novikov, N. E. Shepel, V. G. Nenajdenko and S. L. Ioffe, Synthesis of 2,5-diaryl-4-halo-1,2,3-triazoles and comparative study of their fluorescent properties, Tetrahedron, 2018, 74, 3897–3903 CrossRef CAS.
- T. Bunchuay, A. Docker, U. Eiamprasert, P. Surawatanawong, A. Brown and P. D. Beer, Chalcogen Bond Mediated Enhancement of Cooperative Ion-Pair Recognition, Angew. Chem., Int. Ed., 2020, 59, 12007–12012 CrossRef CAS PubMed.
-
(a) N. Umeda, H. Tsurugi, T. Satoh and M. Miura, luorescent Naphthyl- and Anthrylazoles from the Catalytic Coupling of Phenylazoles with Internal Alkynes through the Cleavage of Multiple C–H Bonds, Angew. Chem., Int. Ed., 2008, 47, 4019–4022 CrossRef CAS PubMed;
(b) N. Umeda, K. Hirano, T. Satoh, N. Shibata, H. Sato and M. Miura, Rhodium-Catalyzed Oxidative 1
:
1, 1
:
2, and 1
:
4 Coupling Reactions of Phenylazoles with Internal Alkynes through the Regioselective Cleavages of Multiple C–H Bonds, J. Org. Chem., 2011, 76, 13–24 CrossRef CAS PubMed;
(c) Z. Qi, S. Yu and X. Li, Rh(III)-Catalyzed Oxidative Annulation of 2-Phenylimidazo[1,2-a]pyridines with Alkynes: Mono versus Double C–H Activation, J. Org. Chem., 2015, 80, 3471–3479 CrossRef CAS PubMed. - Z. Shan, M. Peng, H. Fan, Q. Lu, P. Lu, C. Zhao and Y. Chen, Discovery of potent dipeptidyl peptidase IV inhibitors derived from β-aminoamides bearing substituted [1,2,3]-triazolopiperidines for the treatment of type 2 diabetes, Bioorg. Med. Chem. Lett., 2011, 21, 1731–1735 CrossRef CAS PubMed.
- S. K. Avula, A. Khan, S. A. Halim, Z. Al-Abri, M. U. Anwar, A. Al-Rawahi, R. Csuk and A. Al-Harrasi, Synthesis of novel (R,)-4-fluorophenyl-1H-1,2,3-triazoles: A new class of α-glucosidase inhibitors, Bioorg. Chem., 2019, 91, 103182 CrossRef PubMed.
- D. J. Morgans, K. Mellem, H. L. Powers, P. S. T. Less, W. Won and C. J. Sinz, WO Pat, 2022198196A1, 2022 Search PubMed.
- Q. Tang, L. Wang, Y. Tu, W. Zhu, R. Luo, Q. Tu, P. Wang, C. Wu, P. Gong and P. Zheng, Discovery of novel pyrrolo[2,3-b]pyridine derivatives bearing 1,2,3-triazole moiety as c-Met kinase inhibitors, Bioorg. Med. Chem. Lett., 2016, 26, 1680–1684 CrossRef CAS PubMed.
-
(a) Y. Kataoka, T. Mukohara, H. Tomioka, Y. Funakoshi, N. Kiyota, Y. Fujiwara, M. Yashiro, K. Hirakawa, M. Hirai and H. Minami, Foretinib (GSK1363089), a multi-kinase inhibitor of MET and VEGFRs, inhibits growth of gastric cancer cell lines by blocking inter-receptor tyrosine kinase networks, Invest. New Drugs, 2012, 30, 1352–1360 CrossRef CAS PubMed;
(b) M. Zillhardt, S. M. Park, I. L. Romero, K. Sawada, A. Montag, T. Krausz, S. D. Yamada, M. E. Peter and E. Lengyel, Foretinib (GSK1363089), an orally available multi-kinase inhibitor of c-Met and VEGFR-2, blocks proliferation, induces anoikis, and impairs ovarian cancer metastasis, Clin. Cancer Res., 2011, 17, 4042–4051 CrossRef CAS PubMed.
- J. Han, J. X. Ran, X. P. Chen, Z. H. Wang and F. H. Wu, Study on the green click-chemistry synthesis of 4-trifluoroacetyl-1,2,3-triazoles, Tetrahedron, 2018, 74, 6985–6992 CrossRef CAS.
-
(a) Â. M. A. Lima, W. T. de Paula, I. C. H. L. Leite, P. A. R. Gazolla, L. M. de Abreu, V. R. Fonseca, W. Romão, V. Lacerda, V. T. de Queiroz, R. R. Teixeira and A. V. Costa, Synthesis of eugenol-fluorinated triazole derivatives and evaluation of their fungicidalactivity, J. Braz. Chem. Soc., 2022, 33, 1200–1210 Search PubMed;
(b) Â. M. A. Lima, L. C. Moreira, P. R. Gazolla, M. B. Oliveira, R. R. Teixeira, V. T. Queiroz, M. R. Rocha, W. B. Moraes, N. A. dos Santos, W. Romãod, V. Lacerda Jr., P. A. B. Morais, O. V. de Oliveira, W. C. de Jesus Júnior, L. C. A. Barbosa, C. J. Nascimento, J. Junker and A. V. Costa, Design and Synthesis of Eugenol Derivatives Bearing a 1,2,3-Triazole Moiety for Papaya Protection against Colletotrichum gloeosporioides, J. Agric. Food Chem., 2024, 72, 12459–12468 CrossRef PubMed.
-
(a) R. D. Padmaja and K. Chanda, A Short Review on Synthetic Advances toward the Synthesis ofRufinamide, an Antiepileptic Drug, Org. Process Res. Dev., 2018, 22, 457–466 CrossRef CAS;
(b) W. Junmoin, J. Changsoo, C. Kyuyun, K. Kyungchell and Q. Zheshan, Synthesis and anticonvulsant activity of 1-substituted benzyl-N-substituted-1,2,3-trazoole-4-formamodes, Prog. Nat. Sci., 2006, 16, 925–929 CrossRef.
- C. S. Koble, R. G. Davis, E. W. McLean, F. E. Soroko, B. R. Cooper and J. L. Kelley, 1-(Fluorobenzyl)-4-amino-1H-1,2,3-triazolo[4,5-c]pyridines: Synthesis and Anticonvulsant Activity, J. Med. Chem., 1995, 38, 4131–4134 CrossRef PubMed.
- Y. Xu, Y. Xu, S. Biby, B. Kaur, Y. Liu, F. A. Bagdasarian, H. Y. Wey, R. Tanzi, C. Zhang, C. Wang and S. Zhang, Design and Discovery of Novel NLRP3 Inhibitors and PET Imaging Radiotracers Based on a 1,2,3-Triazole-Bearing Scaffold, J. Med. Chem., 2024, 67, 555–571 CrossRef CAS PubMed.
- L. Y. El-Sharkawy, D. Brough and S. Freeman, Inhibiting the NLRP3 Inflammasome, Molecules, 2020, 25, 5533 CrossRef CAS PubMed.
- Y. Guo, J. Hou, H. Wu, Y. Chen, G. Liu, D. Wang, H. Wang, L. Mao, S. Li and T. Wang, Synthesis and discovery of novel 1,2,3-triazole based cabotegravir derivatives with potent anticancer activity, J. Mol. Struct., 2024, 1298, 137042 CrossRef CAS.
- Y. C. Duan, Y. C. Ma, E. Zhang, X. J. Shi, M. M. Wang, X. W. Ye and H. M. Liu, Design and synthesis of novel 1,2,3-triazole-dithiocarbamate hybrids as potential anticancer agents, Eur. J. Med. Chem., 2013, 62, 11–19 CrossRef CAS PubMed.
-
(a) X. J. Wang, H. W. Xu, L. L. Guo, J. X. Zheng, B. Xu, X. Guo, C. X. Zheng and H. M. Liu, Synthesis and in vitro antitumor activity of new butenolide-containing dithiocarbamates, Bioorg. Med. Chem. Lett., 2011, 21, 3074–3077 CrossRef CAS PubMed;
(b) Y. C. Zheng, Y. C. Duan, J. L. Ma, R. M. Xu, X. Zi, W. L. Lv, M. M. Wang, X. W. Ye, S. Zhu, D. Mobley, Y. Y. Zhu, J. W. Wang, J. F. Li, Z. R. Wang, W. Zhao and H. M. Liu, Triazole–Dithiocarbamate Based Selective Lysine Specific Demethylase 1 (LSD1) Inactivators Inhibit Gastric Cancer Cell Growth, Invasion, and Migration, J. Med. Chem., 2013, 56, 8543–8560 CrossRef CAS PubMed.
- P. K. Kadaba, Triazolines. 14. 1,2,3-Triazolines and triazoles. A new class of anticonvulsants. Drug design and structure-activity relationships, J. Med. Chem., 1988, 31, 196–203 CrossRef CAS PubMed.
- B. Yang, D. Semenzin and A. R. Katritzky, (Trifluoroacetyl)benzotriazole: A Convenient Trifluoroacetylating Reagent, J. Org. Chem., 1997, 62, 726–728 CrossRef PubMed.
- H. Y. He, K. Suzuki and A. R. Katritzky, N-Acylbenzotriazoles: Neutral Acylating Reagents for the Preparation of Primary, Secondary, and Tertiary Amides, J. Org. Chem., 2000, 65, 8210–8213 CrossRef PubMed.
-
(a) T. Horneff, S. Chuprakov, N. Chernyak, V. Gevorgyan and V. V. Fokin, Rhodium-Catalyzed Transannulation of 1,2,3-Triazoles with Nitriles, J. Am. Chem. Soc., 2008, 130, 14972–14974 CrossRef CAS PubMed;
(b) B. Chattopadhyay and V. Gevorgyan, Transition-Metal-Catalyzed Denitrogenative Transannulation: Converting Triazoles into Other Heterocyclic Systems, Angew. Chem., Int. Ed., 2012, 51, 862–872 CrossRef CAS PubMed;
(c) H. M. Davies and J. S. Alford, Reactions of metallocarbenes derived from N-sulfonyl-1,2,3-triazoles, Chem. Soc. Rev., 2014, 43, 5151–5162 RSC;
(d) D. Yadagiri, S. Rajasekar and P. Anbarasan, Recent Advances in Transition-Metal-Catalyzed Denitrogenative Transformations of 1,2,3-Triazoles and Related Compounds, Synthesis, 2014, 3004–3023 Search PubMed.
-
(a) V. Motornov, A. Markos and P. Beier, A rhodium-catalyzed transannulation of N-(per)fluoroalkyl-1,2,3-triazoles under microwave conditions – a general route to N-(per)fluoroalkyl-substituted five-membered heterocycles, Chem. Commun., 2018, 54, 3258–3261 RSC;
(b) V. Motornov and P. Beier, Chemoselective Aza-[4 + 3]-annulation of N-Perfluoroalkyl-1,2,3-triazoles with 1,3-Dienes: Access to N-Perfluoroalkyl-Substituted Azepines, J. Org. Chem., 2018, 83, 15195–15201 CrossRef CAS PubMed;
(c) V. Motornov, V. Košťál, A. Markos, D. Täffner and P. Beier, General approach to 2-fluoroalkyl 1,3-azoles via the tandem ring opening and defluorinative annulation of N-fluoroalkyl-1,2,3-triazoles, Org. Chem. Front., 2019, 6, 3776–3780 RSC;
(d) O. Bakhanovich, V. Khutorianskyi, V. Motornov and P. Beier, Synthesis of N-perfluoroalkyl-3,4-disubstituted pyrroles by rhodium-catalyzed transannulation of N-fluoroalkyl-1,2,3-triazoles with terminal alkynes, Beilstein J. Org. Chem., 2021, 17, 504–510 CrossRef CAS PubMed;
(e) O. Bakhanovich, B. Klepetářová and P. Beier, Rhodium(II)-catalyzed transannulation approach to N-fluoroalkylated indoles, Org. Biomol. Chem., 2023, 21, 7924–7927 RSC.
-
(a) A. Markos, S. Voltrová, V. Motornov, D. Tichý, B. Klepetářová and P. Beier, Stereoselective Synthesis of (Z)-β-Enamido Triflates and Fluorosulfonates from N-Fluoroalkylated Triazoles, Chem. – Eur. J., 2019, 25, 7640–7644 CrossRef CAS PubMed;
(b) T. Chvojka, A. Markos, S. Voltrová, R. Pohl and P. Beier, Ligand-dependent stereoselective Suzuki–Miyaura cross-coupling reactions of β-enamido triflates, Beilstein J. Org. Chem., 2021, 17, 2657–2662 CrossRef CAS PubMed.
- A. Markos, L. Janecky, B. Klepetářová, R. Pohl and P. Beier, Stereoselective Synthesis of (Z)-β-Enamido Fluorides from N-Fluoroalkyl- and N-Sulfonyl-1,2,3-triazoles, Org. Lett., 2021, 23, 4224–4227 CrossRef CAS PubMed.
- A. Kubíčková, A. Markos, S. Voltrová, A. Marková, J. Filgas, B. Klepetářová, P. Slavíček and P. Beier, Aza-Wolff rearrangement of N-fluoroalkyl triazoles to ketenimines, Org. Chem. Front., 2023, 10, 3201–3206 RSC.
-
(a) C. Ye, G. L. Gard, R. W. Winter, R. G. Syvret, B. Twamley and J. M. Shreeve, Synthesis of Pentafluorosulfanylpyrazole and Pentafluorosulfanyl-1,2,3-triazole and Their Derivatives as Energetic Materials by Click Chemistry, Org. Lett., 2007, 9, 3841–3844 CrossRef CAS PubMed;
(b) S. Garg and J. M. Shreeve, Trifluoromethyl- or pentafluorosulfanyl-substituted poly-1,2,3-triazole compounds as dense stable energetic materials, J. Mater. Chem., 2011, 21, 4787–4795 RSC.
- S. Beckendorf, S. Asmus, C. Muck-Lichtenfeld and O. G. Mancheño, “Click” Bis-Triazoles as Neutral C–H⋯Anion-Acceptor Organocatalysts, Chem. – Eur. J., 2013, 19, 1581–1585 CrossRef CAS PubMed.
- M. Zurro, S. Asmus, S. Beckendorf, C. Muck-Lichtenfeld and O. G. Mancheño, Chiral Helical Oligotriazoles: New Class of Anion-Binding Catalysts for the Asymmetric Dearomatization of Electron-Deficient N-Heteroarenes, J. Am. Chem. Soc., 2014, 136, 13999–14002 CrossRef CAS PubMed.
-
(a) M. Mahlau and B. List, Asymmetric Counteranion-Directed Catalysis: Concept, Definition, and Applications, Angew. Chem., Int. Ed., 2013, 52, 518–533 CrossRef CAS PubMed;
(b) K. Brak and E. N. Jacobsen, Asymmetric Ion-Pairing Catalysis, Angew. Chem., Int. Ed., 2013, 52, 534–561 CrossRef CAS PubMed.
-
(a) O. G. Mancheño, S. Asmus, M. Zurro and T. Fischer, Highly Enantioselective Nucleophilic Dearomatization of Pyridines by Anion-Binding Catalysis, Angew. Chem., Int. Ed., 2015, 54, 8823–8827 CrossRef PubMed;
(b) T. Fischer, J. Bamberger and O. G. Mancheño, Asymmetric nucleophilic dearomatization of diazarenes by anion-binding catalysis, Org. Biomol. Chem., 2016, 14, 5794–5802 RSC;
(c) M. Zurro, S. Asmus, J. Bamberger, S. Beckendorf and O. G. Mancheño, Chiral Triazoles in Anion-Binding Catalysis: New Entry to Enantioselective Reissert-Type Reactions, Chem. – Eur. J., 2016, 22, 3785–3793 CrossRef CAS PubMed;
(d) T. Fischer, Q. N. Duong and O. G. Mancheño, Triazole-Based Anion-Binding Catalysis for the Enantioselective Dearomatization of N-Heteroarenes with Phosphorus Nucleophiles, Chem. – Eur. J., 2017, 23, 5983–5987 CrossRef CAS PubMed;
(e) T. Fischer, J. Bamberger, M. Gómez-Martínez, D. G. Piekarski and O. G. Mancheño, Helical Multi-Coordination Anion-Binding Catalysts for the Highly Enantioselective Dearomatization of Pyrylium Derivatives, Angew. Chem., Int. Ed., 2019, 58, 3217–3221 CrossRef CAS PubMed;
(f) M. Gómez-Martínez, M. D. C. Pérez-Aguilar, D. G. Piekarski, C. G. Daniliuc and O. G. Mancheño, N,N-Dialkylhydrazones as Versatile Umpolung Reagents in Enantioselective Anion-Binding Catalysis, Angew. Chem., Int. Ed., 2021, 60, 5102–5107 CrossRef PubMed.
- D. G. Piekarski, P. Steinforth, M. Gómez-Martínez, J. Bamberger, F. Ostler, M. Schonhoff and O. G. Mancheno, Insight into the Folding and Cooperative Multi-Recognition Mechanism in Supramolecular Anion-Binding Catalysis, Chem. – Eur. J., 2020, 26, 17598–17603 CrossRef CAS PubMed.
- P. Steinforth, M. Gómez-Martínez, L.-M. Entgelmeier, O. G. Mancheño and M. Schönhoff, Relevance of the Cation in Anion Binding of a Triazole Host: An Analysis by Electrophoretic Nuclear Magnetic Resonance, J. Phys. Chem. B, 2022, 126, 10156–10163 CrossRef CAS PubMed.
- R. Dorel and B. L. Feringa, Stereodivergent Anion Binding Catalysis with Molecular Motors, Angew. Chem., Int. Ed., 2020, 59, 785–789 CrossRef CAS PubMed.
- D. Mungalpara, S. Stegmüller and S. Kubik, A neutral halogen bonding macrocyclic anion receptor based on a pseudocyclopeptide with three 5-iodo-1,2,3-triazole subunits, Chem. Commun., 2017, 53, 5095–5098 RSC.
- T. Bunchuay, A. Docker, A. J. Martinez-Martinez and P. D. Beer, A Potent Halogen-Bonding Donor Motif for Anion Recognition and Anion Template Mechanical Bond Synthesis, Angew. Chem., Int. Ed., 2019, 58, 13823–13827 CrossRef CAS PubMed.
-
(a) X. Wu, L. W. Judd, E. N. W. Howe, A. M. Withecombe, V. Soto-Cerrato, H. Li, N. Busschaert, H. Valkenier, R. Pérez-Tomás, D. N. Sheppard, Y.-B. Jiang, A. P. Davis and P. A. Gale, Nonprotonophoric Electrogenic Cl− Transport Mediated by Valinomycin-like Carriers, Chem, 2016, 1, 127–146 CrossRef CAS;
(b) Y. Yang, X. Wu, N. Busschaert, H. Furuta and P. A. Gale, Dissecting the chloride–nitrate anion transport assay, Chem. Commun., 2017, 53, 9230–9233 RSC.
- S. J. Edwards, I. Marques, C. M. Dias, R. A. Tromans, N. R. Lees, V. Félix, H. Valkenier and A. P. Davis, Tilting and Tumbling in Transmembrane Anion Carriers: Activity Tuning through n-Alkyl Substitution, Chem. – Eur. J., 2016, 22, 2004–2011 CrossRef CAS PubMed.
- H. M. D. Bandara and S. C. Burdette, Photoisomerization in different classes of azobenzene, Chem. Soc. Rev., 2012, 41, 1809–1825 RSC.
- H.-Y. Duan, S.-T. Han, T.-G. Zhan, L.-J. Liu and K.-D. Zhang, Visible-Light-Switchable Tellurium-Based Chalcogen Bonding: Photocontrolled Anion Binding and Anion Abstraction Catalysis, Angew. Chem., Int. Ed., 2023, 62, e202212707 CrossRef CAS PubMed.
- A. Kerckhoffs, I. Moss and M. J. Langton, Photo-switchable anion binding and catalysis with a visible light responsive halogen bonding receptor, Chem. Commun., 2023, 59, 51–54 RSC.
- K. Torita, R. Haraguchi, Y. Morita, S. Kemmochi, T. Komatsu and S. I. Fukuzawa, Lewis acid–base synergistic catalysis of cationic halogen-bonding-donors with nucleophilic counter anions, Chem. Commun., 2020, 56, 9715–9718 RSC.
- J. Bouffard, B. K. Keitz, R. Tonner, G. Guisado-Barrios, G. Frenking, R. H. Grubbs and G. Bertrand, Synthesis of Highly Stable 1,3-Diaryl-1H-1,2,3-triazol-5-ylidenes and Their Applications in Ruthenium-Catalyzed Olefin Metathesis, Organometallics, 2011, 30, 2617–2627 CrossRef CAS PubMed.
|
This journal is © the Partner Organisations 2024 |