DOI:
10.1039/D4QO01368A
(Research Article)
Org. Chem. Front., 2024,
11, 6319-6326
Well-defined chiral dinuclear copper-catalyzed tandem asymmetric propargylic amination–carboxylative cyclization sequence toward chiral 2-oxazolidinone derivatives†
Received
25th July 2024
, Accepted 14th September 2024
First published on 18th September 2024
Abstract
We report a novel strategy for the synthesis of chiral 2-oxazolidinones via a dinuclear copper-catalyzed asymmetric propargylic amination–carboxylative cyclization sequence of propargylic esters with nucleophilic alkyl amines under ambient pressure of carbon dioxide. A variety of chiral 2-oxazolidinones featuring an exocyclic methylene moiety was obtained in good yields with high enantioselectivities via a one-pot operation.
Introduction
Five-membered heterocyclic compounds like 2-oxazolidinones have found wide ranging applications in medicinal chemistry, organic synthesis and agrochemistry (Scheme 1a).1 Moreover, chiral 2-oxazolidinones have been shown to be of great significance in the realm of asymmetric synthesis, serving as a versatile class of chiral auxiliaries or ligands in the stereoselective synthesis of enantioenriched compounds.2 Therefore, the efficient synthesis of chiral 2-oxazolidinones and their derivatives is of great interest in organic chemistry and medicinal sciences.3
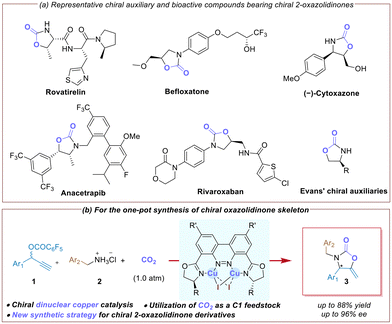 |
| Scheme 1 Asymmetric propargylic amination–carboxylative cyclization sequence toward chiral 2-oxazolidinone derivatives. | |
Utilization of carbon dioxide (CO2) as an inexpensive, nontoxic, and renewable C1 feedstock has attracted attention in the past decades.4 A variety of methods has been developed for the synthesis of achiral 2-oxazolidinones through the cyclization of propargylamines and carbon dioxide.5 However, the use of CO2 in catalytic asymmetric tandem reactions for the synthesis of chiral 2-oxazolidinones in one pot is largely underdeveloped.6,7 In this context, two elegant methods have been developed for the synthesis of chiral N-aryl 2-oxazolidinones based on a tandem process, including the synthesis of chiral propargylamines from an asymmetric aldehyde–alkyne–amine (A3) coupling reaction6 or an asymmetric transfer hydrogenation of alkynyl ketimines,7 followed by a carboxylative cyclization with CO2. However, the synthesis of chiral 2-oxazolidinones bearing an exocyclic methylene motif has been less explored. Therefore, the development of a novel catalytic strategy for the asymmetric synthesis of chiral 2-oxazolidinones derivatives is still highly desirable.3
Although various bi/multi-nuclear copper complexes have been reported,8 their applications in asymmetric catalysis are still much less developed.9 Recently, we disclosed the successful development of a series of binuclear copper catalysts10 based on chiral benzo[c]cinnoline dioxazoline frameworks,11 and their applications in catalytic asymmetric propargylic substitution reactions. Since van Maarseveen and Nishibayashi's pioneering work in 2008,12 copper-catalyzed asymmetric propargylic amination of propargylic esters has been an efficient method for the synthesis of chiral propargyl amines.13 Herein, we report a novel strategy for the synthesis of chiral 2-oxazolidinones via a dinuclear copper-catalyzed asymmetric propargylic amination–carboxylative cyclization sequence of propargylic esters with alkyl amine hydrochlorides under an ambient pressure of carbon dioxide (Scheme 1b).14 A variety of chiral 2-oxazolidinones featuring an exocyclic methylene motif and a N-alkyl group was obtained in good yields with excellent enantioselectivities.
Results and discussion
Reaction development and scope
The studies were initiated by taking the reaction of propargyl carbonate (1a) with benzylamine hydrochloride (2a) under an atm of CO2 as the model reaction and the feasibility of the proposed asymmetric propargylic amination–carboxylative cyclization sequence using the binuclear Cu complexes C1–C8 as catalysts was investigated. A careful survey of the reaction parameters using catalyst C1 revealed that the reaction proceeded well in CF3CH2OH at room temperature for 36 h in the presence of tBuONa (2.0 equiv.) and Ag2CO3 (30 mol%) as additives, affording the desired product 3aa in 51% yield with 73% ee (Table 1, entry 1). Under otherwise identical conditions, other bicopper catalysts, C2–C8, with different substituents on the oxazoline units or the cinnoline backbone of the ligands were subsequently evaluated in the reaction (Table 1, entries 2–8). The reaction catalyzed by C2, with a benzyl group on the oxazoline unit, gave product 3aa in 54% yield with 70% ee (entry 2). To our delight, upon using catalyst C3 with the ligand derived from 2-aminoindanol, the desired product 3aa was obtained with an improved ee value of 79% (entry 3). The reaction using catalyst C4, with increased steric hinderance of the oxazoline units, led to a significant improvement in the ee to 85% (entry 4). Using catalyst C5 featuring further enhanced steric hindrance of the oxazolyl moiety, the ee value of 3aa was increased to 87% (entry 5). Proceeding further by using C6 bearing cyclohexyl groups on the indanyl scaffold as the catalyst, the reaction afforded 3aa in good isolated yield (64%) with excellent enantioselectivity (91% ee, entry 6). However, the introduction of an electron-withdrawing (–Br) group on the indanyl scaffold of catalyst C7 significantly decreased the enantioselectivity of the reaction (entry 7). In addition, catalyst C8, with large steric hinderance on the indenyl scaffold, was also tested, resulting in poor catalytic performance (47% yield, 70% ee) (entry 8). Furthermore, various silver salts had similar impact on the reaction and Ag2CO3 was found to be optimal for the reaction (see the ESI† for reaction details). In addition, free benzylamine was also tested under the optimized conditions (as shown in entry 6), and the desired product was obtained in 74% yield with slightly lower ee (88%). In comparison to free amines, amine hydrochloride salts present a more appealing option due to their widespread commercial availability, cost-effectiveness, and relative stability under atmospheric conditions.15
Table 1 Investigation of the dinuclear copper-catalyzed asymmetric propargylic amination–carboxylative cyclization sequencea
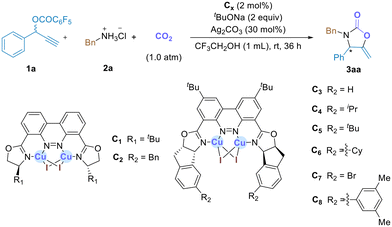
|
Entry |
Cat. |
Yieldb (%) |
eec (%) |
Unless otherwise noted, reaction conditions are as follows: 1a (0.1 mmol), 2a (0.15 mmol), tBuONa (2.0 equiv.), Ag2CO3 (30 mol%), Cx (2 mol%), CF3CH2OH (1 mL), rt, 36 h.
1H NMR yield using mesitylene as the internal standard.
The ee value of 3aa was determined by HPLC on a chiral column IA.
Isolated yield.
|
1 |
C1
|
51 |
73 (S) |
2 |
C2
|
54 |
70 (S) |
3 |
C3
|
49 |
79 (R) |
4 |
C4
|
64 |
85 (R) |
5 |
C5
|
59 |
87 (R) |
6
|
C6
|
70 (64)d
|
91 (R)
|
7 |
C7
|
54 |
65 (R) |
8 |
C8
|
47 |
70 (R) |
Reaction scope
Under the optimized reaction conditions, the scope of propargyl carbonates 1 was first explored in the reaction with benzylamine hydrochloride 2a, and the results are shown in Scheme 2a. The propargyl carbonates bearing either electron-donating (–tBu) or electron-withdrawing (–F, Cl, Br, CF3, –OCF3 and pyridine-containing carbonate) substituents at the para-position of the aryl group reacted smoothly with 2a, affording the corresponding 2-oxazolidinones 3ba–3ha in 42–66% yields with 90–92% ee. Substrates bearing meta-methyl, meta-chloro, and 3,4,5-trimethoxy substituted arenes were also amenable to the procedure, and the reactions provided 3ia–3ka in good yields (40–72%) with 80–91% ee. However, the reactions using the cycloalkyl substituted propargylic carbonate 1l only provided a trace amount of the corresponding product 3la.
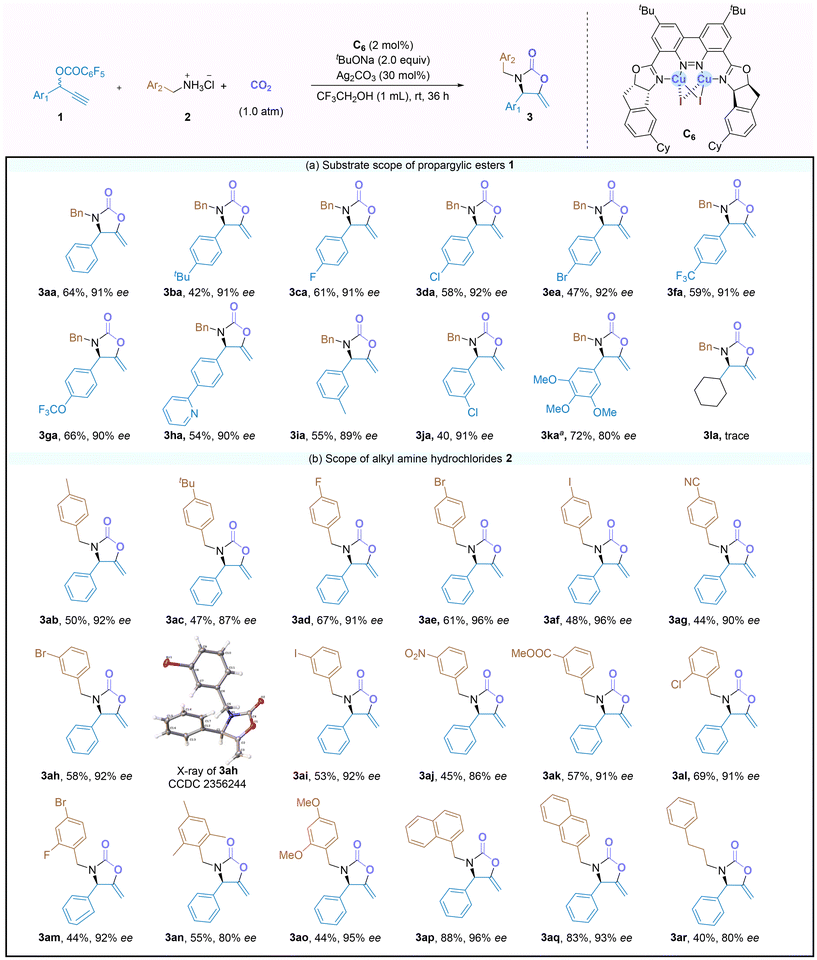 |
| Scheme 2 Substrate scope. Reaction conditions: unless otherwise noted, all reactions were carried out using 1 (0.1 mmol), 2 (0.15 mmol), tBuONa (2.0 equiv.), Ag2CO3 (30 mol%), C6 (2.0 mol%), CF3CH2OH (1.0 mL), rt, 36 h. a Leaving group of the propargyl substrate = OBoc. | |
Subsequently, the scope of alkyl amines hydrochloride 2 was further evaluated in the reactions with propargyl carbonate 1a (Scheme 2b). To our delight, this catalytic system exhibited good tolerance toward various hydrochloride salts of alkyl amines. For instance, the reactions of benzylamines bearing either electronic donating (–Me, and –tBu) or withdrawing (–F, –Br, –I and –CN) groups on the phenyl ring afforded the corresponding products 3ab–3ag in 44–67% yields with good enantioselectivities (87–96% ee). Changing the substituents of the benzylamines from the para- to meta- or ortho-position had no negative effect on the catalysis, smoothly leading to products 3ah–3al in 45–69% yields with 86–92% ee. The absolute configuration of 3ah was established as (R) by X-ray crystallography (CCDC 2356244†), while those of other chiral 2-oxazolidinone products were assigned by analogy. The catalytic system also turned out to be effective for the reactions of poly-substituted benzylamines 2m–2o, giving the corresponding products 3am–3ao in 44–55% yields with 80–95% ee. Furthermore, amine substrates containing an α- or a β-naphthyl group (2p and 2q) were compatible with the protocol, and the reactions gave the corresponding products 3ap and 3aq in 83–88% yields with 93–96% ee. In addition, a primary amine hydrochloride bearing a long alkyl chain, such as 2r, was also a suitable substrate, leading to the formation of the desired 2-oxazolidinone 3ar in 40% yield with 80% ee. Notably, a variety of important functional groups, including –OMe, –F, –Br, –Cl, –I, –CF3, –OCF3, –CO2Me, –CN, and –NO2, was tolerated in the reaction, and the corresponding products showed good compatibility for downstream transformations.
Synthetic applications and control experiments
5-Methylene 2-oxazolidinones are useful chiral building blocks, as demonstrated by a two-step transformation of 3aa to the synthetically useful chiral γ-amino alcohol 4aa without any erosion of ee value (Scheme 3a). In addition, catalytic hydrogenation of the exocyclic C
C double bond of 3aa using Rh(PPh3)3Cl as the catalyst provided (4R,5R)-4ab in high yield (78%) with excellent diastereoselectivity (>95
:
5 dr). To gain an insight into the reaction mechanism, control experiments were carried out (Scheme 3b). Under otherwise standard conditions, the reaction of 1a and 2a in the absence of Ag2CO3 delivered the target product 3aa in a low yield of only 21% with 91% ee, along with the generation of a substantial amount of 3aa′ with similar ee value (66%, 90% ee), suggesting that Ag2CO3 might promote the carboxylative cyclization of 3aa′ in the title reaction. This was confirmed by the reaction of the intermediate 3aa′ with CO2 and without Ag2CO3 or the Cu catalyst; in this case, the cyclization product 3aa was formed in only 19% yield. In contrast, the reaction of intermediate 3aa′ with CO2 in the presence of Ag2CO3 or the binuclear Cu catalyst gave 3aa in significantly improved yields of 74% and 70%, respectively. These results indicated that the title reaction is a tandem binuclear Cu-catalyzed asymmetric propargylic amination and Ag2CO3 promoted the carboxylative cyclization process. The copper catalyst in the first step may also have positive effect on the cyclization process. In addition, the enantioselectivity of 3aa was determined in the propargylic amination step.
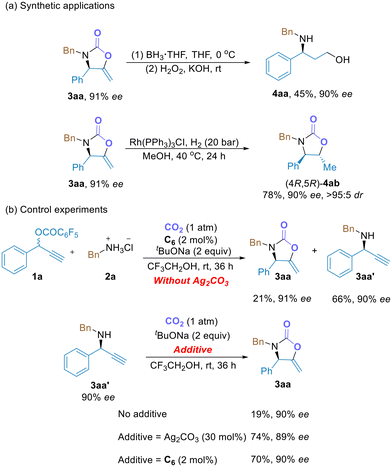 |
| Scheme 3 Synthetic applications and control experiments. | |
Proposed transition state model
Based on the X-ray crystallographic structure of product 3ah and previous computational studies by our group,10 we proposed a stereochemical model to rationalize the observed chiral induction of the system using catalyst C1 (Scheme 4). Due to the large steric bulkiness of the –tBu and the –OCOC6F5 moieties located on the upper part of the ligand plane, the nucleophilic alkyl amines would preferentially attack the terminal carbon of the allene moiety from the bottom side. Subsequent carboxylative cyclization of the resulting chiral propargylamine with CO2 would form the cycloadduct (S)-3aa with retention of the stereochemistry, which is consistent with the results obtained using C1 as the catalyst.
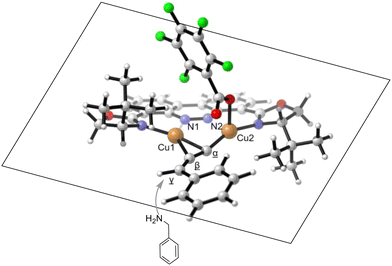 |
| Scheme 4 Proposed chiral induction model based on the catalyst C1. | |
Conclusions
In summary, we have developed an efficient dinuclear copper-catalyzed asymmetric propargylic amination–carboxylative cyclization sequence of propargylic esters with alkyl amine hydrochlorides and CO2, affording a variety of chiral 2-oxazolidinones bearing an exocyclic methylene moiety in good yields with high enantioselectivities.
Author contributions
Y. L., Q. C., and X. W. directed the project; Y. L., P. L., Z. F., L. S. and Q. C. performed all the experiments and analyzed all the data; Y. L., Q. C, and X. W. wrote the manuscript with contributions from all authors.
Data availability
The data supporting this article have been included as part of the ESI.†
Conflicts of interest
There are no conflicts to declare.
Acknowledgements
The authors acknowledge financial support from the National Key R&D Program of China (No. 2022YFA1503200), the Strategic Priority Research Program of the Chinese Academy of Sciences (Grant No. XDB0610000), the National Natural Science Foundation of China (92256303, 22171278, and 21821002), the Shanghai Science and Technology Committee (23ZR1482400), the Natural Science Foundation of Ningbo (2023J034) and Open Research Fund of School of Chemistry and Chemical Engineering, Henan Normal University.
References
-
(a) M. R. Barbachyn and C. W. Ford, Oxazolidinone Structure–Activity Relationships Leading to Linezolid, Angew. Chem., Int. Ed., 2003, 42, 2010–2023 CrossRef PubMed;
(b) T. A. Mukhtar and G. D. Wright, Streptogramins, Oxazolidinones, and Other Inhibitors of Bacterial Protein Synthesis, Chem. Rev., 2005, 105, 529–542 CrossRef PubMed.
-
(a) G. Diaz-Muñoz, I. L. Miranda, S. K. Sartori, D. Cristina Rezende and M. Alves Nogueira Diaz, Use of chiral auxiliaries in the asymmetric synthesis of biologically active compounds: A review, Chirality, 2019, 31, 776–812 CrossRef PubMed;
(b) L. Y. Chen and P. Q. Huang, Evans’ Chiral Auxiliary-Based Asymmetric SyntheticMethodology and Its Modern Extensions, Eur. J. Org. Chem., 2024, 1–25 Search PubMed;
(c) V. Zadsirjan and M. M. Heravi, Oxazolidinones as Chiral Auxiliaries in the Asymmetric 1,4-Conjugate Addition Reaction Applied to the Total Synthesis of Natural Products: A Supplemental Mini-Review, Curr. Org. Synth., 2018, 15, 3–20 CrossRef CAS;
(d) E. Nicolás, K. C. Russell and V. J. Hruby, Asymmetric 1,4-addition of organocuprates to chiral α, β-unsaturated N-acyl-4-phenyl-2-oxazolidinones: a new approach to the synthesis of chiral β-branched carboxylic acids, J. Org. Chem., 1993, 58, 766–770 CrossRef;
(e) M. T. Crimmins and A. L. Choy, An Asymmetric Aldol-Ring-Closing Metathesis Strategy for the Enantioselective Construction of Oxygen Heterocycles: An Efficient Approach to the Enantioselective Synthesis of (+)-Laurencin, J. Am. Chem. Soc., 1999, 121, 5653–5660 CrossRef CAS;
(f) A. Padarti, D. Kim and H. Han, Highly Stereoselective 2-Oxonia-Cope Rearrangement: A Platform Enabling At-Will Control of Regio-, Enantio-, and Diastereoselectivity in the Vinylogous Aldol Reactions of Aldehydes, Org. Lett., 2018, 20, 756–759 CrossRef CAS;
(g) V. Vigneswaran, S. N. MacMillan and D. C. Lacy,
β-Amino Phosphine Mn Catalysts for 1,4-Transfer Hydrogenation of Chalcones and Allylic Alcohol Isomerization, Organometallics, 2019, 38, 4387–4391 CrossRef CAS;
(h) J. M. Fraile, J. I. GarcÌa, C. I. HerrerÌas, J. A. Mayoral, O. Reiser, A. Socuÿllamos and H. Werner, The Role of Binding Constants in the Efficiency of Chiral Catalysts Immobilized by Electrostatic Interactions: The Case of Azabis(oxazoline)–Copper Complexes, Chem. – Eur. J., 2004, 10, 2997–3005 CrossRef CAS PubMed.
-
(a) F. Y. Wang, T. L. Yang, T. Wu, L. S. Zheng, C. C. Yin, Y. J. Shi, X.-Y. Ye, G. Q. Chen and X. M. Zhang, Asymmetric Transfer Hydrogenation of α-Substituted-β-Keto Carbonitriles via Dynamic Kinetic Resolution, J. Am. Chem. Soc., 2021, 143, 2477–2483 CrossRef CAS;
(b) N. S. Barta, D. R. Sidler, K. B. Somerville, S. A. Weissman, R. D. Larsen and P. J. Reider, Practical Modifications and Applications of the Sharpless Asymmetric Aminohydroxylation in the One-Pot Preparation of Chiral Oxazolidin-2-ones, Org. Lett., 2000, 2, 2821–2824 CrossRef CAS PubMed;
(c) C. G. Espino and J. Du Bois, A Rh-Catalyzed C-H Insertion Reaction for the Oxidative Conversion of Carbamates to Oxazolidinones, Angew. Chem., Int. Ed., 2001, 40, 598–600 CrossRef CAS;
(d) L. E. Overman and T. P. Remarchuk, Catalytic Asymmetric Intramolecular Aminopalladation: Enantioselective Synthesis of Vinyl-Substituted 2-Oxazolidinones, 2-Imidazolidinones, and 2-Pyrrolidinones, J. Am. Chem. Soc., 2002, 124, 12–13 CrossRef CAS;
(e) Y. Cui and C. He, A Silver-Catalyzed Intramolecular Amidation of Saturated C-H Bonds, Angew. Chem., Int. Ed., 2004, 43, 4210–4212 CrossRef CAS PubMed;
(f) D. B. Berkowitz and G. Maiti, Following an ISES Lead: The First Examples of Asymmetric Ni (0)-Mediated Allylic Amination, Org. Lett., 2004, 6, 2661–2664 CrossRef CAS;
(g) H. Lebel, K. Huard and S. Lectard,
N-Tosyloxycarbamates as a Source of Metal Nitrenes: Rhodium-Catalyzed C-H Insertion and Aziridination Reactions, J. Am. Chem. Soc., 2005, 127, 14198–14199 CrossRef CAS;
(h) D. N. Barman and K. M. Nicholas, Copper-Catalyzed Intramolecular C–H Amination, Eur. J. Org. Chem., 2011, 908–911 CrossRef;
(i) Y. Fukata, K. Asano and S. Matsubara, Procedure-Controlled Enantioselectivity Switch in Organocatalytic 2-Oxazolidinone Synthesis, J. Am. Chem. Soc., 2013, 135, 12160–12163 CrossRef;
(j) Q. L. Wang, X. F. Tan, Z. Y. Zhu, X. Q. Dong and X. M. Zhang, New synthetic strategy for chiral 2-oxazolidinones derivatives via rhodium-catalyzed asymmetric hydrogenation, Tetrahedron Lett., 2016, 57, 658–662 CrossRef;
(k) W. Li, M. Wollenburg and F. Glorius, Enantioselective synthesis of 2-oxazolidinones by ruthenium(II)–NHC-catalysed asymmetric hydrogenation of 2-oxazolones, Chem. Sci., 2018, 9, 6260–6263 RSC;
(l) Y. H. Liu, Z. Y. Yi, X. L. Yang, H. Wang, C. C. Yin, M. Y. Wang, X. Q. Dong and X. M. Zhang, Efficient Access to Chiral 2-Oxazolidinones via Ni-Catalyzed Asymmetric Hydrogenation: Scope Study, Mechanistic Explanation, and Origin of Enantioselectivity, ACS Catal., 2020, 10, 11153–11161 CrossRef;
(m) P. K. Yu, D. Y. Chen, Y. W. Liu, C. C. Yin, Q. X. Liu and H. F. Zhou, Synthesis of Chiral 2-Oxazolidinones by Ruthenium-Catalyzed Asymmetric Transfer Hydrogenation of 2-Oxazolones, Adv. Synth. Catal., 2023, 365, 1–7 CrossRef.
-
(a) D. M. D'Alessandro, B. Smit and J. R. Long, Carbon Dioxide Capture: Prospects for New Materials, Angew. Chem., Int. Ed., 2010, 49, 6058–6082 CrossRef PubMed;
(b) M. Cokoja, C. Bruckmeier, B. Rieger, W. A. Herrmann and F. E. Kühn, Transformation of Carbon Dioxide with Homogeneous Transition-Metal Catalysts: A Molecular Solution to a Global Challenge?, Angew. Chem., Int. Ed., 2011, 50, 8510–8537 CrossRef CAS;
(c) M. Y. He, Y. H. Sun and B. X. Han, Green Carbon Science: Scientific Basis for Integrating Carbon Resource Processing, Utilization, and Recycling, Angew. Chem., Int. Ed., 2013, 52, 9620–9633 CrossRef CAS;
(d) E. V. Kondratenko, G. Mul, J. Baltrusaitis, G. O. Larrazábal and J. Pérez-Ramírez, Status and perspectives of CO2 conversion into fuels and chemicals by catalytic, photocatalytic and electrocatalytic processes, Energy Environ. Sci., 2013, 6, 3112–3135 RSC;
(e) M. Aresta, A. Dibenedetto and A. Angelini, Catalysis for the Valorization of Exhaust Carbon: from CO2 to Chemicals, Materials, and Fuels. Technological Use of CO2, Chem. Rev., 2014, 114, 1709–1742 CrossRef;
(f) K. Sekine and T. Yamada, Silver-catalyzed carboxylation, Chem. Soc. Rev., 2016, 45, 4524–4532 RSC;
(g) J. H. Ye, T. Ju, H. Huang, L. L. Liao and D. G. Yu, Radical Carboxylative Cyclizations and Carboxylations with CO2, Acc. Chem. Res., 2021, 54, 2518–2531 CrossRef PubMed.
-
(a) S. F. Cai, H. R. Li and L. N. He, Bifunctionalization of unsaturated bonds via carboxylative cyclization with CO2: a sustainable access to heterocyclic compounds, Green Chem., 2021, 23, 9334–9347 RSC;
(b) X. Yang, L. K. Xu, Y. Q. Zhu, S. J. Zhang, G. W. Jia and J. Du, Efficient fabrication of oxazolidinones for the carboxylative cyclization with carbon dioxide, J. CO2 Util., 2023, 74, 102531–102553 CrossRef;
(c) Z. Zhang, J. H. Ye, D. S. Wu, Y. Q. Zhou and D. G. Yu, Synthesis of Oxazolidin-2-ones from Unsaturated Amines with CO2 by Using Homogeneous Catalysis, Chem. – Asian J., 2018, 13, 2292–2306 CrossRef PubMed;
(d) C. K. Ran, H. Huang, X. H. Li, W. Wang, J. H. Ye, S. S. Yan, B. Q. Wang, C. Feng and D. G. Yu, Cu-Catalyzed Selective Oxy-Cyanoalkylation of Allylamines with Cycloketone Oxime Esters and CO2, Chin. J. Chem., 2020, 38, 69–76 CrossRef;
(e) L. Sun, J. H. Ye, W. J. Zhou, X. Zeng and D. G. Yu, Oxy-Alkylation of Allylamines with Unactivated Alkyl Bromides and CO2 via Visible-Light-Driven Palladium Catalysis, Org. Lett., 2018, 20, 3049–3052 CrossRef;
(f) S. Wang and C. J. Xi, Recent advances in nucleophile-triggered CO2-incorporated cyclization leading to heterocycles, Chem. Soc. Rev., 2019, 48, 382–404 RSC;
(g) M. Yoshida, T. Mizuguchi and K. Shishido, Synthesis of Oxazolidinones by Efficient Fixation of Atmospheric CO2 with Propargylic Amines by using a Silver/1,8-Diazabicyclo[5.4.0]undec-7-ene (DBU) Dual-Catalyst System, Chem. – Eur. J., 2012, 18, 15578–15581 CrossRef PubMed;
(h) R. M. Yuan, B. H. Wei and G. Fu, How the Coordinated Structures of Ag(I) Catalysts Affect the Outcomes of Carbon Dioxide Incorporation into Propargylic Amine: A DFT Study, J. Org. Chem., 2017, 82, 3639–3647 CrossRef;
(i) S. Arshadi, E. Vessally, M. Sobati, A. Hosseinian and A. Bekhradnia, Chemical fixation of CO2 to N-propargylamines: A straightforward route to 2-oxazolidinones, J. CO2 Util., 2017, 19, 120–129 CrossRef;
(j) X. Xu, Z. T. Li, H. L. Huang, X. Jing and C. Y. Duan, A novel copper metal–organic framework catalyst for the highly efficient conversion of CO2 with propargylic amines, Inorg. Chem. Front., 2022, 9, 3839–3844 RSC;
(k) J. N. Goswami, D. Saha, U. Saha, A. Bera, M. Ouladsmane, N. Bar, S. Biswas, M. Z. Ansari and M. Dolai, Noble metal free double helix binuclear Cu(I) complex as catalyst for carboxylative cyclization of propargylamines with atmospheric carbon dioxide toward oxazolidinones synthesis under mild conditions, Inorg. Chim. Acta, 2024, 563, 121926–121936 CrossRef;
(l) F. Chen, Sh. Tao, Q. Q. Deng, D. H. Wei, N. Liu and B. Dai, Binuclear Tridentate Hemilabile Copper(I) Catalysts for Utilization of CO2 into Oxazolidinones from Propargylic Amines, J. Org. Chem., 2020, 85, 15197–15212 CrossRef PubMed.
- X. T. Gao, C. C. Gan, S. Y. Liu, F. Zhou, H. H. Wu and J. Zhou, Utilization of CO2 as a C1 Building Block in a Tandem Asymmetric A3 Coupling-Carboxylative Cyclization Sequence to 2-Oxazolidinones, ACS Catal., 2017, 7, 8588–8593 CrossRef.
- Z. Zhang, Z. H. Zhang, F. Zhou and J. Zhou, Catalytic Enantioselective Transfer Hydrogenation−Carboxylative Cyclization to 4-Fluoroalkyl 2-Oxazolidinone with CO2 as the C1 Synthon, Org. Lett., 2021, 23, 2726–2730 CrossRef.
-
(a) C. J. Fahrni, Allylic Oxidation Catalyzed by Chiral Dinuclear Copper Complexes, Tetrahedron, 1998, 54, 5465–5470 CrossRef;
(b) C. J. Fahrni and A. Pfaltz, Synthesis of Chiral C2-Symmetric Binucleating Ligands, Helv. Chim. Acta, 1998, 81, 491–506 CrossRef;
(c) C. J. Fahrni, A. Pfaltz, M. Neuburger and M. Zehnder, Structure and Properties of Transition-Metal Complexes with Chiral C2-Symmetric Binucleating Ligands, Helv. Chim. Acta, 1998, 81, 507–524 CrossRef;
(d) E. Salvadeo, L. Dubois and J. M. Latour, Trinuclear copper complexes as biological mimics: Ligand designs and reactivities, Coord. Chem. Rev., 2018, 374, 345–375 CrossRef;
(e) R. B. Ferreira and L. J. Murray, Cyclophanes as Platforms for Reactive Multimetallic Complexes, Acc. Chem. Res., 2019, 52, 447–455 CrossRef;
(f) A. N. Desnoyer, A. Nicolay, P. Rios, M. S. Ziegler and T. D. Tilley, Bimetallics in a Nutshell: Complexes Supported by Chelating Naphthyridine-Based Ligands, Acc. Chem. Res., 2020, 53, 1944–1956 CrossRef PubMed;
(g) R. Mal, N. Mittal, T. J. Emge and D. Seidel, Facile synthesis of a chiral urea bridged bisoxazoline ligand and structural characterization of its bis-copper(II)-chloride complex, Chem. Commun., 2009, 7309–7311 RSC.
-
(a) J. Gao, J. H. Reibenspies and A. E. Martell, Structurally Defined Catalysts for Enantioselective Oxidative Coupling Reactions, Angew. Chem., Int. Ed., 2003, 42, 6008–6012 CrossRef CAS PubMed;
(b) W. J. Li and S. X. Qiu, A Novel D2-Symmetrical Chiral Tetraoxazoline Ligand for Highly Enantioselective Hydrosilylation of Aromatic Ketones, Adv. Synth. Catal., 2010, 352, 1119–1122 CrossRef;
(c) L. Zhang, G. Yang, C. Shen, S. Arghib and W. Zhang, Chiral dinuclear phthalazine bridged bisoxazoline ligands: synthesis and application in enantioselective Cu-catalyzed conjugate addition of ZnEt2 to enones, Tetrahedron Lett., 2011, 52, 2375–2378 CrossRef;
(d) S. F. Zhu, B. Xu, G. P. Wang and Q. L. Zhou, Well-Defined Binuclear Chiral Spiro Copper Catalysts for Enantioselective N-H Insertion, J. Am. Chem. Soc., 2012, 134, 436–442 Search PubMed.
- Q. L. Cai, H. Q. Rao, S. J. Li, Y. Lan, K. L. Ding and X. M. Wang, Well-defined chiral dinuclear copper complexes in enantioselective propargylic substitution: For a long-standing supposition on binuclear mechanism, Chem, 2024, 10, 265–282 Search PubMed.
-
(a) A. L. Gavrilova and B. Bosnich, Principles of mononucleating and binucleating ligand design, Chem. Rev., 2004, 104, 349–383 Search PubMed;
(b) P. A. Vigato, V. Peruzzo and S. Tamburini, Acyclic and cyclic compartmental ligands: recent results and perspectives, Coord. Chem. Rev., 2012, 256, 953–1114 CrossRef;
(c) A. M. Barrios and S. J. Lippard, Phthalazine-based dinucleating ligands afford dinuclear centers often encountered in metalloenzyme active sites, Inorg. Chem., 2001, 40, 1060–1064 CrossRef PubMed;
(d) S. Brooker, Some copper and cobalt complexes of Schiff-base macrocycles containing pyridazine head units, Eur. J. Inorg. Chem., 2002, 2002, 2535–2547 CrossRef;
(e)
L. Zhang, G. Yang and W. Zhang. China Patent CN102464656A, 2012 Search PubMed;
(f) R. Connon, B. Roche, B. V. Rokade and P. J. Guiry, Further developments and applications of oxazoline-containing ligands in asymmetric catalysis, Chem. Rev., 2021, 121, 6373–6521 CrossRef CAS PubMed;
(g) D. Das, R. Mal, N. Mittal, Z. Zhu, T. J. Emge and D. Seidel, Chiral bisoxazoline ligands designed to stabilize bimetallic complexes, Beilstein J. Org. Chem., 2018, 14, 2002–2011 CrossRef CAS PubMed;
(h) G. Desimoni, G. Faita and K. A. Jørgensen, C2-symmetric chiral bis(oxazoline) ligands in asymmetric catalysis, Chem. Rev., 2006, 106, 3561–3651 CrossRef PubMed;
(i) Y. Z. Zhu, Z. P. Li, J. A. Ma, F. Y. Tang, L. Kang, Q. L. Zhou and A. S. C. Chan, Synthesis of chiral bis(oxazolinyl)bipyridine ligands and related helical metal complexes, Tetrahedron: Asymmetry, 2002, 13, 161–165 CrossRef.
-
(a) R. J. Detz, M. M. E. Delville, H. Hiemstra and J. H. van Maarseveen, Enantioselective Copper-Catalyzed Propargylic Amination, Angew. Chem., Int. Ed., 2008, 47, 3777–3780 CrossRef PubMed;
(b) G. Hattori, H. Matsuzawa, Y. Miyake and Y. Nishibayashi, Copper-Catalyzed Asymmetric Propargylic Substitution Reactions of Propargylic Acetates with Amines, Angew. Chem., Int. Ed., 2008, 47, 3781–3783 CrossRef PubMed.
-
(a) G. Hattori, K. Sakata, H. Matsuzawa, Y. Tanabe, Y. Miyake and Y. Nishibayashi, Copper-Catalyzed Enantioselective Propargylic Amination of Propargylic Esters with Amines: Copper–Allenylidene Complexes as Key Intermediates, J. Am. Chem. Soc., 2010, 132, 10592–10608 CrossRef;
(b) C. Zhang, Y. H. Wang, X. H. Hu, Z. Zheng, J. Xu and X. P. Hu, Chiral Tridentate P,N,N Ligands for Highly Enantioselective Copper-Catalyzed Propargylic Amination with both Primary and Secondary Amines as Nucleophiles, Adv. Synth. Catal., 2012, 354, 2854–2858 CrossRef;
(c) T. Mino, H. Taguchi, M. Hashimoto and M. Sakamoto, Copper-catalyzed asymmetric propargylic amination of propargylic acetates with amines using BICMAP, Tetrahedron: Asymmetry, 2013, 24, 1520–1523 CrossRef;
(d) A. Yoshida, G. Hattori, Y. Miyake and Y. Nishibayashi, Copper-Catalyzed Enantioselective Propargylic Amination of Nonaromatic Propargylic Esters with Amines, Org. Lett., 2011, 13, 2460–2463 CrossRef PubMed;
(e) S. J. Li, J. Huang, J. Y. He, R. J. Zhang, H. D. Qian, X. L. Dai, H. H. Kong and H. Xu, Highly enantioselective copper-catalyzed propargylic amination to access N-tethered 1,6-enynes, RSC Adv., 2020, 10, 38478–38483 RSC;
(f) Z. Zhang, Y. Sun, Y. Gong, D. L. Tang, H. Luo, Z. P. Zhao, F. Zhou, X. Wang and J. Zhou, Enantioselective propargylic amination and related tandem sequences to α-tertiary ethynylamines and azacycles, Nat. Chem., 2024, 16, 521–532 CrossRef PubMed;
(g) J. S. Ma, H. Y. Lu, Y. W. Chen, W. C. Zhao, Y. Z. Sun, R. P. Li, H. X. Wang, G. Q. Lin and Z. T. He, Copper-catalysed convergent regio- and enantioselective alkynylallylic substitution, Nat. Synth., 2023, 2, 37–48 CrossRef;
(h) D. Y. Zhang and X. P. Hu, Recent advances in copper-catalyzed propargylic substitution, Tetrahedron Lett., 2015, 56, 283–295 CrossRef CAS;
(i) C. H. Ding and X. L. Hou, Catalytic asymmetric propargylation, Chem. Rev., 2011, 111, 1914–1937 CrossRef CAS;
(j) N. Ljungdahl and N. Kann, Transitionmetal-catalyzed propargylic substitution, Angew. Chem., Int. Ed., 2009, 48, 642–644 CrossRef CAS;
(k) R. J. Detz, H. Hiemstra and J. H. van Maarseveen, Catalyzed propargylic substitution, Eur. J. Org. Chem., 2009, 6263–6276 CrossRef CAS;
(l) Y. Miyake, S. Uemura and Y. Nishibayashi, Catalytic propargylic substitution reactions, ChemCatChem, 2009, 1, 342–356 CrossRef CAS.
- During the submission of this manuscript, a similar Cu-catalyzed reaction via CO2 shuttling or CO2 fixation was independently reported: H. Li, J. S. Ma, H. Y. Lu, G. Q. Lin and Z. T. He, Asymmetric Multicomponent Propargylations via Carbon Dioxide Shuttling and Fixation, ACS Catal., 2024, 14, 11646–11656 CrossRef.
-
(a) W. J. Yoo and C. J. Li, Highly Efficient Oxidative Amidation of Aldehydes with Amine HydrochlorideSalts, J. Am. Chem. Soc., 2006, 128, 13064–13065 CrossRef CAS PubMed;
(b) S. C. Ghosh, J. S. Y. Ngiam, A. M. Seayad, D. T. Tuan, C. L. L. Chai and A. Chen, Copper-Catalyzed Oxidative Amidation of Aldehydes with Amine Salts: Synthesis of Primary, Secondary, and Tertiary Amides, J. Org. Chem., 2012, 77, 8007–8015 CrossRef CAS PubMed;
(c) W. L. Zhang, Y. J. Yao, Y. L. Xu, X. Y. Zhou and G. Wu, Amine hydrochloride salts as bifunctional reagents for the radical aminochlorination of maleimides, Org. Chem. Front., 2021, 8, 5766–5770 RSC;
(d) J. Huang, H. H. Kong, S. J. Li, R. J. Zhang, H. D. Qian, D. R. Li, J. Y. He, Y. N. Zheng and H. Xu, Asymmetric copper-catalyzed propargylic amination with amine hydrochloride salts, Chem. Commun., 2021, 57, 4674–4677 RSC.
|
This journal is © the Partner Organisations 2024 |
Click here to see how this site uses Cookies. View our privacy policy here.